- Chemical Sciences, Faculty of Science, Universiti Brunei Darussalam, Gadong, Brunei
The ideal methods for the preparation of semiconductors should be reproducible and possess the ability to control the morphology of the particles with monodispersity yields. Apart from that, it is also crucial to synthesize a large quantity of desired materials with good control of size, shape, morphology, crystallinity, composition, and surface chemistry at a reasonably low production cost. Metal oxides and chalcogenides with various morphologies and crystal structures have been obtained using different anion metal precursors (and/or different sulfur sources for chalcogenides in particular) through typical synthesis methods. Generally, spherical particles are obtained as it is thermodynamically favorable. However, by changing the anion precursor salts, the morphology of a semiconductor is influenced. Therefore, precursors having different anions show some effects on the final forms of a semiconductor. This review compiled and discussed the effects of anions (NO3−, Cl−, SO42-, CH3COO−, CH(CH3)O−, etc.) and different sources of S2- on the morphology and crystal structure of selected metal oxides and chalcogenides respectively.
Introduction
Heterogeneous photocatalysis using semiconductors has drawn attention following the discovery of the Honda-Fujishima effect in 1972 (Liu et al., 2014). Photocatalysis has also gained remarkable attention due to its potential application for energy shortage and environmental issues which include hydrogen production from water (Shi et al., 2019), carbon dioxide reduction to fuels (M.S et al., 2021), and toxic pollutants removals in the environment (Koutavarapu et al., 2021). Semiconductor consists of a band structure in which the conduction band (CB) is separated from the valence band (VB) by a band gap. This is one of the important properties as it determines the light absorption and the redox capabilities of a semiconductor. Theoretically, in photocatalysis, when the energy of incident light is equal or larger than that of the band gap of a semiconductor, electrons (e−) and holes (h+) are generated in the CB and VB, respectively (Matussin et al., 2020a; Naidi et al., 2021; Rahman et al., 2021). These photogenerated charge carriers may be involved in the following possible processes:
1) Migrate to the surface-active sites of semiconductor
2) Captured by the defect sites in bulk and/or on the surface of the semiconductor
3) Recombine and release energy in the form of heat or photon
The last two processes are, however, considered to be deactivation processes due to these photogenerated e− and h+ would not involve in photocatalytic reactions. A large number of inorganic semiconductors have been explored including metal oxides, IV group, III-V compounds, and metal chalcogenides. Semiconductor oxide nanomaterials-based photocatalysts have been recognized as one of the most promising areas of research and application such as TiO2, ZnO, SnO2, CeO2, etc (Qi et al., 2013; Khan et al., 2017; Kowsari et al., 2017; Parwaiz et al., 2019; Matussin et al., 2020b; Rahman and Khan, 2021). Metal oxides are mainly used as photocatalysts due to their non-toxicity, low cost, stability, and resistance to photocorrosion.
In comparison to most of the metal oxides, semiconductors including III-V compounds, IV group, and metal chalcogenides show narrow band gap, large optical absorption coefficients, and broad-spectrum light collection (Popescu, 2006; Ahluwalia, 2017). They are called narrow-gap semiconductors in which the band gap of these semiconductors is usually less than 2.3 eV. This could allow light absorption at the wavelength of more than 540 nm. Chalcogenides are compounds consisting of at least one chalcogen anion (S2-, Se2- or Te2-) and at least one electropositive element. Chalcogenides have drawn significant attention due to their great and highly demanded properties including narrow band gap energy, non-toxicity, and bio-compatibility.
The performance of a semiconductor is strongly correlated with its size. When the size of materials falls into the nanoscale, materials may exhibit different properties (Navya and Daima, 2016). As the size is reduced, the atoms or ions percentage exposed on the surface increases, resulting in an increase in the surface to volume ratio (Navya and Daima, 2016). Therefore, the number of active sites for catalytic reactions increases. Moreover, the reduction of size might also affect the electronic properties of the material. In particular, as the material size is smaller than its Bohr radius, the movement of the charge carriers is greatly confined in physical size due to the quantum confinements. This results in the discrete electronic band structure, leading to size-dependent electronic and optical properties (Li and Wu, 2015).
Furthermore, the morphology of a catalyst is crucial since factors such as the size and shape of particles, the energy associated with facets, coordination of atoms, and the presence of protective ligands can mainly influence its catalytic efficiency (Cao et al., 2016; Guo et al., 2018; Lin et al., 2019; Mishra and Nanda, 2020). In a recent study, Chiu et al. (2012) conducted facet-dependent catalytic activity of Au nanocubes, octahedral, and rhombic dodecahedra towards 4-nitroaniline. It was reported that anisotropic shape particles can alter the reaction performance due to differences in crystal facets exposed. Therefore, the concept of morphology-dependent catalytic and/or photocatalytic activity of a semiconductor has become a growing topic in catalysis and for the exploration of potential applications nowadays.
Varied shapes and sizes of semiconductors are reported to have been obtained through different synthesis methods for instance hydrothermal, precipitation, sol-gel, microwave, green synthesis, and many others (Qi et al., 2013; Sahay et al., 2013; Soren et al., 2015; Hasnidawani et al., 2016; Yin et al., 2016). Furthermore, counter-anion in the metal salts precursors plays a role in the shape-selective growth of semiconductor nanomaterials. It is said that the inorganic anions themselves might be selectively adsorbed on particular facets and thus greatly affect the size, and morphology of the nanomaterials (Herricks et al., 2004; Qi et al., 2014). To date, the lack of studies on anions effects on the development of metal oxides and chalcogenides have become a challenge to prepare metal oxides and chalcogenides with controlled morphology and size. Moreover, various shaped semiconductors without implementation of agents are somehow in demand to prevent high-cost methods and chemical hazards. Recently, researchers have gradually begun studies on the effects of anions on the production of semiconductors. Therefore, in this review, different morphologies of metal oxides and chalcogenides obtained using different metal salts precursors and their crystal growth are discussed in-depth. To the authors’ knowledge, there has been no review on the development of semiconductors using different metal precursors having different anions. This is the first review and compilation of the role of anions in the synthesis and crystal growth of selected metal oxides and chalcogenides.
Anion Directed Synthesis of Metal Oxides
Metal oxides nanoparticles (NPs) have been widely exploited for many different areas such as toxic pollutants removal (Gowthaman et al., 2020; Yang et al., 2020; Zhou et al., 2021), drug delivery (He et al., 2019; Mallakpour et al., 2022), hydrogen production (Chen et al., 2015; Bhosale et al., 2016; Chen et al., 2018), CO2 reduction (Loh and Kherani, 2019; Sun et al., 2021; Kuan et al., 2022), optoelectronics (C. Nehru et al., 2012; Jayakumar et al., 2022; Wang et al., 2010), etc., Controllable growth of metal oxides NPs with defined morphology such as spherical, rod-like, sheet-like, cubic amongst others have been synthesized and reported to have an influence on their catalytic properties.
Various morphologies of metal oxides have been acquired from different metal precursors salts (Figure 1). For instance, Panda et al. synthesized ZnO nanorods through a sonochemical method using two different Zn precursors namely: Zn(CH3COO)2 and Zn(NO3)2·6H2O dissolved in a basic condition at room temperature using ammonium acetate and ammonia solution (Panda et al., 2013). Flower-like ZnO was obtained when NO3− anion precursor was used, while CH3COO− anion precursor showed a nanorod with an average width size between 150 and 500 nm for both anions. Similarly, Gusatti et al. (2011) prepared ZnO via the sonochemical method. However, Zn(NO3)2·6H2O and ZnCl2 were used. NaOH was added to both the solutions at 90°C resulting in a mixture of short nanoprisms and nanorods of 18.91 nm long and 11.50 nm wide for NO3− anion precursor and nanorods of 23 nm diameter for Cl− anion precursor. High purity ZnO NPs were synthesized using Zn(NO3)2·6H2O, Zn(CH3COO)2, ZnSO4·7H2O and ZnCl2 using a typical precipitation method as reported by Pourrahimi et al. (2014) The precursors’ solutions were stirred at 60°C for 15 min and pre-heated NaOH was added to the solutions yielding star-shaped particle (500 nm) for NO3− anion, cone-shaped particle (25 nm) for CH3COO− anion, petal-like for both SO4− and Cl− anions (80–100 nm).
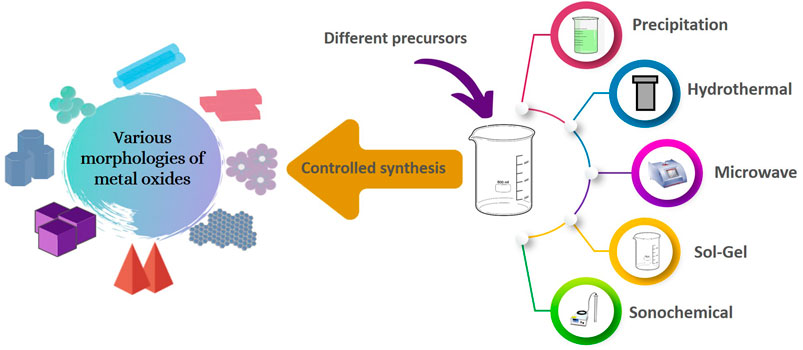
FIGURE 1. Different morphologies of metal oxides synthesized using different precursors having different anions.
The formation of hexamethylenetetramine (HMTA)-mediated ZnO nanoparticles was reported by van Rijt et al. (2020) The ZnO particles were synthesized using Zn(NO3)2·6H2O, Zn(CH3COO)2, ZnSO4·7H2O and ZnCl2 through precipitation method. Hexamine was added to the solutions at 80°C for 6 h. Hexagonal pillar-like shape was obtained when NO3− anion was used, the dumbbell-shaped particle was seen for CH3COO− and hexagonally faceted plate-shaped particles were obtained for both SO4− and Cl− anions. Kaenphakdee et al. (2022) prepared ZnO using Zn(CH3COO)2 and Zn(NO3)2·6H2O. Precipitation method was used in which monoethanolamine in 2-methoxy ethanol was added for CH3COO− anion precursor and hexamethylenetetramine in H2O for NO3− anion precursor at 90°C for 2 h. These resulted in the aggregation of particles which yielded about 400–500 nm for CH3COO− anion precursor and submicron rod-shaped particle at about 3 µm in length and 500 nm in diameter for NO3− anion precursor. Kathalingam et al. (2015) synthesized various morphologies of ZnO by varying the zinc precursors (Zn(NO3)2·6H2O and Zn(CH3COO)2) the precursor’s concentration (10 mM- 0.5 M) as well as the preparation method. It was found that ZnO using NO3− anion precursor shows spherical (45 nm), rod-like (35 nm), plate-like (120 nm), needle-like (32 nm), tube-like (35 nm) ZnO particles. The concentration of the precursor solution was varied leading to different morphologies as well. ZnO particles using CH3COO− anion shows rod-like (15 nm) and wire-like structures (20 nm). Ozel et al. (2016) prepared ZnO particles using Zn(NO3)2·6H2O and ZnCl2 via hydrothermal method. NH4OH was added to the precursor solution at 100°C. Flower-like structure of ZnO was obtained with an average size of 5–7 µm when NO3− anion precursor was used while rod-like ZnO was attained when Cl− anion was used. Dey et al. (2021) reported on the precursor-dependent nanostructures of ZnO. Zn(CH3COO)2, Zn(NO3)2·6H2O and, ZnCl2 were used in the hydrothermal synthesis of ZnO at 120°C. Various morphologies of ZnO were obtained: nano-pencil, nanorods, and no defined shape for CH3COO−, NO3−, and Cl− anions, respectively.
Different morphologies of CeO2 were observed as reported by Wu et al. (2008) CeCl3·7H2O and Ce(NO3)3·6H2O precursors were used in the hydrothermal reaction. The reaction was carried out at 140°C for 48 h producing CeO2 nanorods (15–25 nm in diameter and length up to a few micrometers) and CeO2 nanocubes (8–30 nm) for Cl− and NO3− anions, respectively. Kumar et al. (2017) prepared mesoporous CeO2 using CeCl3·7H2O, Ce(NO3)3·6H2O, (NH4)2Ce(NO3)6 and Ce(CH3COO)3 through hydrothermal reaction. The reaction was carried out at different conditions for each precursor used. L-glycine and Na2(CO2)2 solution were prepared and added to CeCl3 solution and hydrothermally heated at 160°C. In the case of Ce(CH3COO)3, Hexadecylamine in ethanol was added to the solution and stirred at room temperature. It was then placed in an oven at 60°C for 2 days. For Ce(NO3)3, a mixture of CTAB and NaOH was added to Ce(NO3)3 solution and stirred at 90°C and aged at 60°C for 12 h. Acryl amide, glucose, ammonia solution were added to (NH4)2Ce(NO3)6 solution and it was stirred at room temperature for 5 h. Transamidation of acetamide with N-octylamine was carried out and investigated using the CeO2 produced from these methods. It was found that CeO2 with a rod-like structure produced the highest conversion of acetamide.
Samiee and Goharshadi (2012) reported on the effects of different precursors on the properties of CeO2 in which CeO2 was prepared using Ce(NO3)3·6H2O and (NH4)2Ce(NO3)6 in a microwave-assisted synthesis. It was found that CeO2 synthesized using Ce(NO3)3·6H2O showed cubic-shaped particles with an average particle size of 7 nm. Similarly, CeO2 synthesized using (NH4)2Ce(NO3)6 was also showed cubic structure with an average particle size of about 3 nm. Aneggi et al. (2014) reported on the shape-dependent activity of CeO2 in soot combustion. Hydrothermal method was used to synthesize CeO2 in a basic condition using NaOH. Two different precursors were used namely, Ce(NO3)3·6H2O and CeCl3·7H2O in the synthesis producing CeO2 nanocubes and nanorods, respectively. The high stability of CeO2 for the catalytic combustion of chlorobenzene was synthesized using various cerium precursors (Zhang et al., 2021). Ce(NO3)3·6H2O, Ce(CH3COO)3, CeCl3·7H2O, and Ce(SO34)3·8H2O were used in hydrothermal synthesis at 180°C. It was observed that CeO2 synthesized from Ce(NO3)3·6H2O, Ce(CH3COO)3, CeCl3·7H2O and Ce(SO34)3·8H2O show rod-like (5–11 nm in diameter and 40–250 nm in length), lamellar structured particles (3–11 nm), a series of small spherical particles (5–23 nm) and strip structured particles (70–75 nm in width and 70–950 nm in length), respectively. It was found that rod-like CeO2 showed an increase in soot combustion activity.
Zhu et al. (2020) synthesized CeO2 using Ce(NO3)3·6H2O and CeCl3·7H2O in hydrothermal reaction for photocatalytic CO2 reduction. The synthesis was carried out at 140 and 180°C producing CeO2 nanocubes of about 30 nm length and nanorod of 200–400 nm in length and 20 nm in diameter when Ce(NO3)3·6H2O and CeCl3·7H2O were used, respectively. It was observed that CeO2 nanorods showed efficient photocatalytic CO2 reduction. Feng et al. reported on highly reducible nanostructured CeO2 for CO oxidation (Feng et al., 2018). Hydrothermal synthesis reaction was carried out using Ce(NO3)3·6H2O and CeCl3·7H2O at 110 and 160°C, respectively. Tube-like CeO2 was obtained with an average diameter of 30–70 nm and 1–5 µm in length for Ce(NO3)3·6H2O. Meanwhile, rod-like CeO2 at about 300 nm to 1 µm in length and 20–40 nm in diameter was observed for CeCl3·7H2O. The authors found that rod-like CeO2 exhibited the highest activity. Aboul-Gheit et al. (2014) prepared shape-dependent nano-TiO2 for the photodegradation of black b dye in water. TiO2 was synthesized using TiCl4 and Ti(OCH(CH3)2)4 via precipitation method. Semisphere particles of about 20 nm were obtained when TiCl4 was used whereas for the case of Ti(OCH(CH3)2)4, highly agglomerated CeO2 particles were obtained. Singh et al. (2017) synthesized TiO2 via sol-gel method using K2TiO(C2O4)2·2H2O and Ti(OCH(CH3)2)4. NH4OH was added to K2TiO(C2O4)2·2H2O solution and stirred at room temperature and diethanolamine was added in the Ti(OCH(CH3)2)4 solution. Irregular spherical to a mixture of platelet-shaped CeO2 (11–53 nm) and spherical (29–58 nm) for K2TiO(C2O4)2·2H2O and Ti(OCH(CH3)2)4, respectively.
Influence of different anions precursors on the morphologies of Co3O4 was reported by Hussain et al. (2014) Co(NO3)2·6H2O, CoCl2·6H2O, Co(CH3COO)2·4H2O and CoSO4·7H2O were used in the synthesis in a low temperature aqueous chemical growth. It was found that the synthesized Co3O4 showed a honeycomb-like, network of nanowires, grass-like and nanosheets when Co(NO3)2·6H2O, CoCl2·6H2O, Co(CH3COO)2·4H2O and CoSO4·7H2O were used, respectively. Various Fe precursors of Fe were used to produce Fe2O3 as reported by Guru et al. (2016) Microwave synthesis was used at 100°C by mixing ethylene glycol. NaOH, CTAB, and Fe precursors namely: Fe(NO3)3·9H2O, FeSO4·7H2O, Fe2(SO4)3·H2O and FeCl3·6H2O. For all cases, spherical particles were obtained in which the average particle sizes were in the range of 19–80 nm. In another report, α-Fe2O3 was synthesized hydrothermally from three different Fe sources: Fe(NO3)3·9H2O, FeCl3·6H2O and Fe(SO4)2·6H2O (Benhammada et al., 2020). Similarly, for all cases, spherical particles were observed giving an average particle size in the range of 80–110 nm (Sanjini et al., 2017). Microwave synthesized CuO NPs showed various morphologies when three different precursors were used. Spherical-shaped CuO NPs were obtained for the case of CuCl2, needle-shaped CuO NPs were obtained for the case of Cu(NO3)2, and spherical particles for the case of CuSO4.
Counter anions have different abilities to electrostatically stabilize individual nanoparticles into isolated highly crystalline solids during the full course of the reaction as stated by Pourrahimi et al. (2014) In general, the formation of spherical particles is thermodynamically more favorable (Khodashenas and Ghorbani, 2019). Hence, spherical particles have mainly been observed and obtained in the literature. It is well known that the nucleation and growth of nanostructures can be achieved using stabilizing agents with desired thermodynamic and kinetic control. The shape-selectivity of a semiconductor is usually achieved by additional shape-directing agents. These agents absorb preferentially on specific crystallographic planes leading to the change of direction and rate of crystal growth (Jain et al., 2019). Moreover, synthesis methods also play a role in the shape-selectivity of a semiconductor (Figure 2). Although there are many reports on the role of various additives in controlling crystal growth, there are only a few studies reported the influence of inorganic counter ions in shape-selective growth of metal oxide without the involvement of agents (Siegfried and Choi, 2005).
In the case of a typical synthesis of metal oxides using Cl− counter anion precursor, the final shape of a metal oxide (MO) is generally rod-like. This has been illustrated in many literatures as can be seen in Table 1. In general, when Cl− counter anion precursor is used in the synthesis with NaOH, it forms M(OH)3 (M = Zn, Ce, Ti, Co, Fe, and Cu) in which rod-like structure has been obtained. During the dehydration and oxidation process, the rod-like shape is not changed except for the size. It can be said that nanorod-like geometry is the intrinsic formation of the case of Cl− (C. Nehru et al., 2012; Kaenphakdee et al., 2022; Dey et al., 2021). Apart from that, Cl− directs the growth of particles into tube-like or wire-like particles. This is similar to rod-like structure however, the synthesis conditions such as temperature, acidity, and basicity as well as the use of capping agents leads to the distortion of the rod shape of particles (Samiee and Goharshadi, 2012; Zhu et al., 2020; Zhang et al., 2021).
Interestingly, when NO3− salts were introduced, the morphology of the metal oxides was directed into faceted shaped MO (cube, plate-like, hexagonal, honeycomb, etc.) (C. Nehru et al., 2012; Jayakumar et al., 2022; Wang et al., 2010; Panda et al., 2013; Zhu et al., 2020). Typically, metal oxides form polyhedral-kind of shape and in order to tune the surface free energies and induce the anisotropic growth of well-shaped nanostructures, adscititious surfactants are required in which this is the case for most of the reported shapes (Table 1) (Yang and Gao, 2006). However, a dissolution-recrystallization process under the strong basic condition would influence the production of cube-like or faceted metal oxides particles (Yang and Gao, 2006).
Pourrahimi et al. (2014) has conducted studies on the probable “shielding effect” of different counter anions on the particle stabilization. It was found that both Cl− and NO3− ions showed inability to stabilize the particles. Furthermore, nitrate-based precursor has shown to produce smaller particles which was aimed to grow specific directional morphologies in hydroxide solutions (Cho et al., 2008). On the other hand, CH3COO− ions has the strong ability to stabilize as it has been suggested to originate from strong uni- and bi-dentate oxygen coordination bonding of the acetate ions to individual metal atoms, or parallel bridging of the two oxygen atoms in the CH3COO− ions to positively charged metal atoms of the particles (Sun et al., 2007; Segets et al., 2011; Pourrahimi et al., 2014). Moreover, Nicholas et al. (2012) stated that, partially positively charged methyl functional unit of the CH3COO− ions associated with the insufficiently condensed negatively charged metal hydroxide which therefore suggesting the stabilization of growing nanoparticles probably derived from a formed amphiphilic capping layer around the particle (Pourrahimi et al., 2014).
Spherical-shaped CeO2 has shown high efficiency in photocatalysis activities due to its small particle size and high surface areas (Sanjini et al., 2017; Benhammada et al., 2020). However, nanoshaped CeO2 (cube, rod, hexagonal, etc.) are evident to have effects on photocatalysis activities. This is because nanoshaped CeO2 enabled the study of the correlation between exposed surfaces and photocatalytic activities. Anneggi et al. proposed that {100}/{110} exposed surfaces are more reactive in photocatalysis activities, particularly on CO oxidation. This observation can be seen in many studies (Kumar et al., 2017; Feng et al., 2018; Zhu et al., 2020; Zhang et al., 2021).
Anion Directed Synthesis of Chalcogenides
Chalcogenides are narrow-band gap semiconductors consisting of at least one chalcogen anion (sulphide, selenide, or telluride) and at least one more electropositive element (Khan and Khan, 2021; Rahman and Khan, 2021). Unlike metal oxide, researchers have widely explored varying the sulfur precursors for chalcogenides instead of varying the anions of the metal precursors. Table 2 shows some of the reported works on varying the precursors of selected chalcogenides. Over the last decades, many preparation routes have been developed for the synthesis of chalcogenides with different morphologies, particle sizes, and crystal structures that can be obtained from different raw materials through different synthetic pathways (Figure 3). Various authors have investigated the effect of anion on the morphology, particle size, and crystal structure of different chalcogenides. For instance, Gaur and Jeevanandam (2015) investigated the effect of anions (acetate, chloride, nitrate, and sulfate) in diphenyl ether and in solid-state that leads to the formation of CdS nanoparticles with different morphologies. The CdS nanoparticles derived from solid-state thermal decomposition of the cadmium-thiourea complexes with acetate, chloride, nitrate, and sulfate ions exhibited spheres, nanotubes, nanoflowers, and irregular morphologies, respectively. On the other hand, thermal decomposition of the cadmium thiourea complexes with acetate, chloride, and nitrate ions in diphenyl ether results in CdS nanoparticles with microspheres, nanopyramids, and a mixture of nanoparticles and nanorods morphologies, respectively. Amongst the synthesized materials, CdS synthesized from cadmium acetate and thiourea via solid-state exhibited the highest photocatalytic crystal violet degradation of 99.2%.
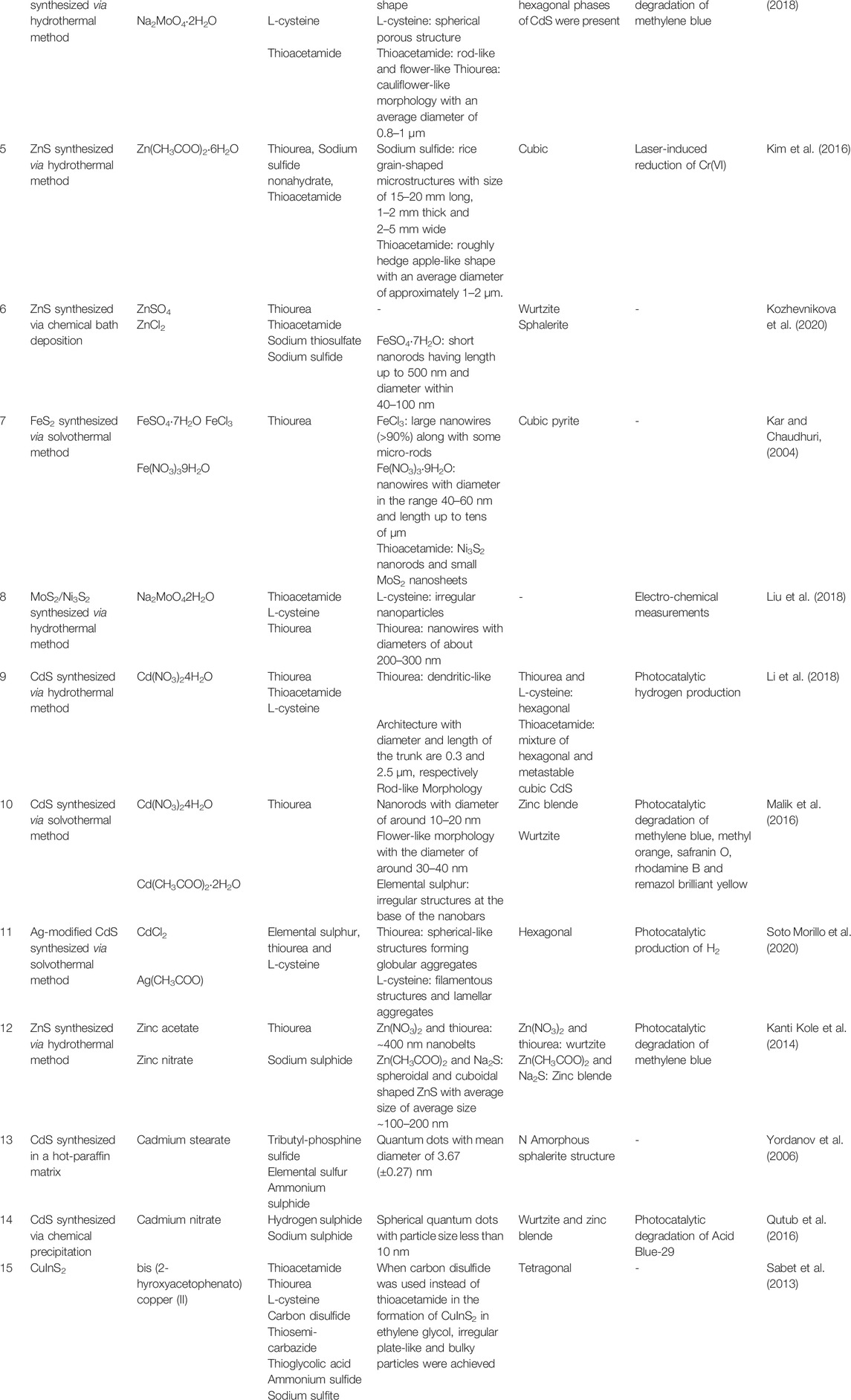
TABLE 2. Summary of previous work on the effect of anions on the morphology, particle size, and crystal structure of various chalcogenides.
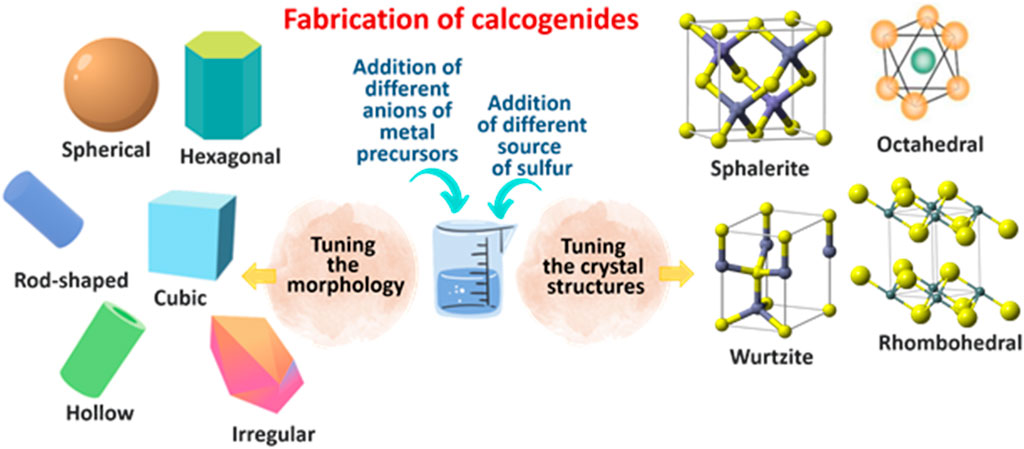
FIGURE 3. Different morphologies and crystal structures of chalcogenides synthesized using different anion metal precursors and different sources of sulfur.
In a different study, two morphologies of MoS2 were obtained by using thiourea and L-cysteine as sulfur sources (Zhang et al., 2017). Zhang et al. reported that MoS2 prepared by using thiourea had a petal-shaped structure, and the crystal size was larger while MoS2 prepared from L-cysteine had a loose structure, and the crystal size was smaller. They also reported that MoS2 prepared from thiourea exhibited better catalytic performance than that from L-cysteine in the hydrodeoxygenation reaction. Hydrothermal assisted synthesis of CdS/MoS2 using three different sulfur sources; thioacetamide, L-cysteine, and thiourea has been reported by Wang et al. (2018) Their results showed that the different sulfur sources induced differences in crystallization, morphology, elemental composition, and absorption in the UV–visible light region. Among the three sulfur sources, CdS/MoS2 prepared using thioacetamide showed excellent adsorption performance and the highest photocatalytic ability to degrade methylene blue with approximately 97% within 120 min under visible light irradiation, much higher than that achieved by CdS/MoS2 prepared using L-cysteine and thiourea.
Kim et al. (2016) have successfully controlled the morphology of ZnS by utilizing anionic precursors via a hydrothermal method for reduction of Cr(VI). The authors reported rate of nucleation is the main factor affecting the morphology variations, and it mainly depends on the rate of release of S2- by the anionic thiourea, thioacetamide, and sodium sulfide precursors. When thiourea and thioacetamide are used as the sulfur sources, the rate of S2- release is slow compared to that achieved with sodium sulfide. The rate of release of S2- from thiourea, in particular, is very slow in comparison to that from thioacetamide because of the strong coupling between the -NH2 group and the nanoparticles. The ZnS nanostructures prepared using thiourea as a sulfur source had a cauliflower-like morphology with an average diameter of 0.8–1 μm. When sodium sulfide was used as the sulfur source, rice grain-shaped microstructures were produced while thioacetamide produces hedge apple-like shape with an average diameter of 1–2 μm.
In another study, Kozhevnikova et al. (2020) have successfully synthesized ZnS using the chemical bath deposition method. In this study, they have used different sources of sulfur including thiourea, thioacetamide, sodium thiosulfate, and sodium sulphide. All the synthesized ZnS exhibited wurtzite and sphalerite ZnS structures. In addition to this, they have also reported that the chemical nature and initial concentrations of ZnSO4 and ZnCl2 salts have no significant effect on particle size, phase composition, and crystal structure of ZnS colloids. FeS2 with different morphologies have been successfully synthesized via solvothermal method as reported by Kar and Chaudhuri (2004) They found that the anions of the iron source (FeSO4·7H2O, FeCl3 and Fe(NO3)3·9H2O), temperature, and the molar concentrations of the precursors in the solvent play an important role in controlling the morphology of the FeS2. When FeSO4·7H2O was used as the iron source, short nanorods having lengths up to 500 nm and diameter within 40–100 nm were produced. When FeCl3 was used, large FeS2 nanowires along with some micro-rods were observed. When Fe(NO3)3·9H2O was used as the precursor, uniform nanowires with diameters in the range 40–60 nm and length up to tens of μm.
Liu et al. (2018) reported that the different sources of sulfur in synthesizing MoS2/Ni3S2 heterostructure have a significant influence on its structures and morphologies. They reported that MoS2/Ni3S2 prepared from thioacetamide showed Ni3S2 nanorods and small MoS2 nanosheets while L-cysteine showed the formation of irregular nanoparticles. In addition, nanowires with diameters of about 200–300 nm were observed when MoS2/Ni3S2 prepared from thiourea. The thioacetamide-assisted synthesis of MoS2/Ni3S2 showed superior H2 evolution reaction activities due to the higher content of MoS2 and it exhibited a larger electrochemically active surface area which provides more active sites for the H2 evolution reaction. Li et al. (2018) have also reported the effects of these sulfur sources (thiourea, thioacetamide, and L-cysteine) on the properties of the resulted CdS including the crystal structure, morphology, and photocatalytic performance for H2 evolution reaction. Based on their study, CdS prepared using thiourea with hexagonal branched dendritic structure has the smallest interfacial electron transfer resistance and the most negative conduction band bottom, and consequently shows the highest H2 evolution reaction. CdS prepared using thioacetamide on the other hand exhibited a mixed phase of hexagonal and cubic which facilitated the recombination of photogenerated charge carriers that leads to a considerably lower H2 evolution performance in comparison to CdS synthesized using thiourea. Moreover, low crystallized hexagonal CdS nanoparticles with no specific morphology were observed for CdS prepared using L-cysteine as the source of sulfur showed the largest interfacial electron transfer resistance and this resulted in the lowest H2 evolution reaction.
Kanti Kole et al. (2014) have been successfully synthesized ZnS nanostructures of different morphologies, such as block-like, belt-like, spheroidal, and cuboidal shaped nanoparticles by using the simple hydrothermal technique. It has been shown that controlling the amount of sulphur precursor or utilizing different types and ratios of zinc and sulphur precursors can easily alter both the phase and morphology of ZnS nanostructures. They also reported that pure phase wurtzite ZnS nanobelts exhibited superior performance for the degradation of methylene blue dye with a degradation efficiency of 98% within 40 min of UV light irradiation. Different crystal structures of CdS nanoparticles prepared via chemical precipitation method using different sulfur sources ((NH4)2S, H2S, Na2S) have been reported by Qutub et al. Their group has studied the effect of different sulfur sources on the size of nanoparticles, respective band gaps, and crystalline structure. Based on their findings, a smaller particles size for CdS prepared using Na2S, followed by H2S and (NH4)2S was observed, and the quantization in the band gap was directly in correlation with decreased particle size effects. Moreover, a mixed-phase of wurtzite and zinc-blende was obtained for CdS synthesized H2S, while the pure phase of zinc-blende and wurtzite was obtained with Na2S and (NH4)2S, respectively. They also reported that CdS synthesized using Na2S with the addition of sodium hydroxide and methanol exhibited the highest activity and almost completely decolorized the derivative Acid Blue-29 under irradiation of visible light within 90 min. Tang et al. (2015) reported a one-pot synthesis of CuInS2 using different anions to engineer their morphology and crystal structure. CuInS2 having chalcopyrite, zinc blende, and wurtzite phases have been successfully synthesized by carefully selecting anions in metal precursors and manipulating reaction parameters such as reactant molar ratios and reaction temperature. They reported that CuInS2 nanoplates with a wurtzite-zinc blende polytypism structure are formed in the presence of Cl− ions. Furthermore, they also reported that the optical absorption measurements of CuInS2 exhibited a strong dependence on the crystal structure and size.
Generally, the preparation methods and the conditions of synthesis are crucial factors for fabricating chalcogenides, and they possess a major role in the chemical as well as structural applications of chalcogenides. In addition to that, the influence of utilizing different anionic metal precursors and/or different sources of sulfur on the structural and morphological properties of chalcogenides was not largely reported in comparison to other semiconductors. Chalcogenides with controllable crystal structures and morphologies have potential applications in various areas as diverse as catalysis, plasmonics, sensing/imaging, spectroscopy, and medicine.
Challenges During the Synthesis and Crystal Growth of Semiconductors
Properties of metal oxides have been considered to be dependent on the morphologies. However, in order to produce targeted shapes of a metal oxide, some agents should be employed in the synthesis. Therefore, metal oxides with different morphologies without the use of agents have become a major challenge. Fabrication of chalcogenides, in particular, can be quite challenging because of their stability. In addition to that, the selection of a suitable precursor is a crucial stage because it will not only have an influence on the physical properties of the materials but also its chemical and optical properties. Moreover, it is also important to avoid the use of toxic precursors, environmentally friendly solvents, keeping the reaction temperature close to room temperature, and also minimizing the quantities of generated by-products are great advantages that make the synthesis of metal oxides and chalcogenides outstanding.
Future Prospects
Controlled crystal growth of semiconductors is crucial for activity efficiency in various applications (biological, environmental, and energy). The controlled crystal growth can be achieved by changing the anion precursor salts and keeping other conditions the same. However, to date, the reports on this matter are still less in number in which some research gaps are yet to be answered. The following are the future prospects that should be considered and addressed:
• Most of the syntheses using different anion precursors to produce different shapes require different synthesis conditions. In order to effectively study the role of anions, one should keep other conditions the same and vary the anion precursors only.
• Most syntheses and studies still require stabilizing and capping agents to aid the formation of different morphologies of a semiconductor.
• In-depth study of crystallographic properties of a semiconductor should be carried out to study the overall effect of different anions on a semiconductor.
• Deeper understanding of the growth mechanisms of the semiconductor via computational simulation would help the researchers to fabricate materials with desired properties more efficiently.
Conclusion
Various morphologies of semiconductors (metal oxides and chalcogenides) have been obtained using different anion precursor salts through typical synthesis methods. Spherical particles are normally observed due to their thermodynamically favorable properties. However, by changing the anion precursor’s salts, the morphology of a semiconductor is affected accordingly. This can be said that the anions have some effects on the final forms of a semiconductor. Nevertheless, in-depth studies are required to investigate the effect of anions on the crystal growth of a semiconductor to get maximum efficiency for the fabricated particles.
Author Contributions
SM: writing–original draft. AR: writing–original draft. MK: supervision, funding acquisition, writing–review and editing.
Funding
The authors would like to acknowledge the FIC block grant UBD/RSCH/1.4/FICBF(b)/2021/035 received from Universiti Brunei Darussalam, Brunei Darussalam.
Conflict of Interest
The authors declare that the research was conducted in the absence of any commercial or financial relationships that could be construed as a potential conflict of interest.
Publisher’s Note
All claims expressed in this article are solely those of the authors and do not necessarily represent those of their affiliated organizations or those of the publisher, the editors, and the reviewers. Any product that may be evaluated in this article, or claim that may be made by its manufacturer, is not guaranteed or endorsed by the publisher.
Acknowledgments
The authors would like to acknowledge the Universiti Brunei Darussalam, Brunei Darussalam for all type of support.
References
Aboul-Gheit, A. K., El-Desouki, D. S., and El-Salamony, R. A. (2014). Different Outlet for Preparing Nano-TiO2 Catalysts for the Photodegradation of Black B Dye in Water. Egypt. J. Pet. 23 (3), 339–348. doi:10.1016/j.ejpe.2014.08.010
Ahluwalia, G. K. (2017). Applications of Chalcogenides: S, Se, and Te. doi:10.1007/978-3-319-41190-3
Aneggi, E., Wiater, D., de Leitenburg, C., Llorca, J., and Trovarelli, A. (2014). Shape-Dependent Activity of Ceria in Soot Combustion. ACS Catal. 4 (1), 172–181. doi:10.1021/cs400850r
Benhammada, A., Trache, D., Kesraoui, M., Tarchoun, A. F., Chelouche, S., and Mezroua, A. (2020). Synthesis and Characterization of α-Fe2O3 Nanoparticles from Different Precursors and Their Catalytic Effect on the thermal Decomposition of Nitrocellulose. Thermochim. Acta 686 (February), 178570. doi:10.1016/j.tca.2020.178570
Bhosale, R., Kumar, A., AlMomani, F., Ghosh, U., Saad Anis, M., Kakosimos, K., et al. (2016). Solar Hydrogen Production via a Samarium Oxide-Based Thermochemical Water Splitting Cycle. Energies 9 (5), 316. doi:10.3390/en9050316
Cao, S., Tao, F., Tang, Y., Li, Y., and Yu, J. (2016). Size- and Shape-dependent Catalytic Performances of Oxidation and Reduction Reactions on Nanocatalysts. Chem. Soc. Rev. 45, 4747–4765. doi:10.1039/c6cs00094k
Chen, C., Zhan, Y., Li, D., Zhang, Y., Lin, X., Jiang, L., et al. (2018). Preparation of CuO/CeO2 Catalyst with Enhanced Catalytic Performance for Water-Gas Shift Reaction in Hydrogen Production. Energy Technol. 6 (6), 1096–1103. doi:10.1002/ente.201700750
Chen, J., Shen, S., Wu, P., and Guo, L. (2015). Nitrogen-doped CeOx Nanoparticles Modified Graphitic Carbon Nitride for Enhanced Photocatalytic Hydrogen Production. Green. Chem. 17 (1), 509–517. doi:10.1039/c4gc01683a
Chiu, C.-Y., Chung, P.-J., Lao, K.-U., Liao, C.-W., and Huang, M. H. (2012). Facet-Dependent Catalytic Activity of Gold Nanocubes, Octahedra, and Rhombic Dodecahedra toward 4-Nitroaniline Reduction. J. Phys. Chem. C 116 (44), 23757–23763. doi:10.1021/jp307768h
Cho, S., Jung, S.-H., and Lee, K.-H. (2008). Morphology-Controlled Growth of ZnO Nanostructures Using Microwave Irradiation: From Basic to Complex Structures. J. Phys. Chem. C 112 (33), 12769–12776. doi:10.1021/jp803783s
C. Nehru, L., Swaminathan, V., and Sanjeeviraja, C. (2012). Photoluminescence Studies on Nanocrystalline Tin Oxide Powder for Optoelectronic Devices. Materials 2 (2), 6–10. doi:10.5923/j.materials.20120202.02
Dey, S., Das, S., and Kar, A. K. (2021). Role of Precursor Dependent Nanostructures of ZnO on its Optical and Photocatalytic Activity and Influence of FRET between ZnO and Methylene Blue Dye on Photocatalysis. Mater. Chem. Phys. 270, 124872. doi:10.1016/j.matchemphys.2021.124872
Feng, G., Han, W., Wang, Z., Li, F., and Xue, W. (2018). Highly Reducible Nanostructured CeO2 for CO Oxidation. Catalysts 8 (11), 535. doi:10.3390/catal8110535
Gaur, R., and Jeevanandam, P. (2015). Effect of Anions on the Morphology of CdS Nanoparticles Prepared via Thermal Decomposition of Different Cadmium Thiourea Complexes in a Solvent and in the Solid State. New J. Chem. 39 (12), 9442–9453. doi:10.1039/C5NJ01605C
Gowthaman, N. S. K., Ngee Lim, H., Balakumar, V., and Shankar, S. (2020). Ultrasonic Synthesis of CeO2@organic Dye Nanohybrid: Environmentally Benign Rabid Electrochemical Sensing Platform for Carcinogenic Pollutant in Water Samples. Ultrason. Sonochem. 61, 104828. doi:10.1016/j.ultsonch.2019.104828
Guo, K., Li, H., and Yu, Z. (2018). Size-Dependent Catalytic Activity of Monodispersed Nickel Nanoparticles for the Hydrolytic Dehydrogenation of Ammonia Borane. ACS Appl. Mater. Inter. 10 (1), 517–525. doi:10.1021/acsami.7b14166
Guru, S., Mishra, D., Singh, M., Amritphale, S. S., and Joshi, S. (2016). Effect of SO4 2-, Cl- and NO3 - Anions on the Formation of Iron Oxide Nanoparticles via Microwave Synthesis. Prot. Met. Phys. Chem. Surf. 52 (4), 627–631. doi:10.1134/S2070205116040146
Gusatti, M., Barroso, G. S., Campos, C. E. M. d., Souza, D. A. R. d., Rosário, J. d. A. d., Lima, R. B., et al. (2011). Effect of Different Precursors in the Chemical Synthesis of ZnO Nanocrystals. Mat. Res. 14 (2), 264–267. doi:10.1590/S1516-14392011005000035
Hasnidawani, J. N., Azlina, H. N., Norita, H., Bonnia, N. N., Ratim, S., and Ali, E. S. (2016). Synthesis of ZnO Nanostructures Using Sol-Gel Method. Proced. Chem. 19, 211–216. doi:10.1016/j.proche.2016.03.095
He, K., Li, J., Shen, Y., and Yu, Y. (2019). pH-Responsive Polyelectrolyte Coated Gadolinium Oxide-Doped Mesoporous Silica Nanoparticles (Gd2O3@MSNs) for Synergistic Drug Delivery and Magnetic Resonance Imaging Enhancement. J. Mater. Chem. B 7 (43), 6840–6854. doi:10.1039/c9tb01654f
Herricks, T., Chen, J., and Xia, Y. (2004). Polyol Synthesis of Platinum Nanoparticles: Control of Morphology with Sodium Nitrate. Nano Lett. 4 (12), 2367–2371. doi:10.1021/nl048570a
Hussain, M., Ibupoto, Z. H., Abbasi, M. A., Nur, O., and Willander, M. (2014). Effect of Anions on the Morphology of Co3O4 Nanostructures Grown by Hydrothermal Method and Their pH Sensing Application. J. Electroanalytical Chem. 717-718 (717), 78–82. doi:10.1016/j.jelechem.2014.01.011
Jain, S., Panigrahi, A., and Sarma, T. K. (2019). Counter Anion-Directed Growth of Iron Oxide Nanorods in a Polyol Medium with Efficient Peroxidase-Mimicking Activity for Degradation of Dyes in Contaminated Water. ACS Omega 4 (8), 13153–13164. doi:10.1021/acsomega.9b01201
Jayakumar, G., Albert Irudayaraj, A., Dhayal Raj, A., John Sundaram, S., and Kaviyarasu, K. (2022). Electrical and Magnetic Properties of Nanostructured Ni Doped CeO2 for Optoelectronic Applications. J. Phys. Chem. Sol. 160 (August), 110369. doi:10.1016/j.jpcs.2021.110369
Kaenphakdee, S., Putthithanas, P., Yodyingyong, S., Leelawattanachai, J., Triampo, W., Sanpo, N., et al. (2022). Zinc Oxide Synthesis from Extreme Ratios of Zinc Acetate and Zinc Nitrate: Synergistic Morphology. Materials 15 (2), 570. doi:10.3390/ma15020570
Kanti Kole, A., Sekhar Tiwary, C., and Kumbhakar, P. (2014). Morphology Controlled Synthesis of Wurtzite ZnS Nanostructures through Simple Hydrothermal Method and Observation of White Light Emission from ZnO Obtained by Annealing the Synthesized ZnS Nanostructures. J. Mater. Chem. C 2 (21), 4338–4346. doi:10.1039/C4TC00091A
Kar, S., and Chaudhuri, S. (2004). Solvothermal Synthesis of Nanocrystalline FeS2 with Different Morphologies. Chem. Phys. Lett. 398 (1–3), 22–26. doi:10.1016/j.cplett.2004.09.028
Kathalingam, A., Park, H.-C., Kim, S.-D., Kim, H.-S., Velumani, S., and Mahalingam, T. (2015). Synthesis of ZnO Nanorods Using Different Precursor Solutions and Their Two Terminal Device Characterization. J. Mater. Sci. Mater. Electron. 26 (8), 5724–5734. doi:10.1007/s10854-015-3129-6
Khan, M. E., Khan, M. M., and Cho, M. H. (2017). Ce3+-ion, Surface Oxygen Vacancy, and Visible Light-Induced Photocatalytic Dye Degradation and Photocapacitive Performance of CeO2-Graphene Nanostructures. Sci. Rep. 7 (1), 1–17. doi:10.1038/s41598-017-06139-6
Khan, M. M. (2021). in Chalcogenide-Based Nanomaterials as Photocatalysts. Editor M. M. Khan (Amsterdam, Netherlands: Elsevier). doi:10.1016/C2019-0-01819-5
Khodashenas, B., and Ghorbani, H. R. (2019). Synthesis of Silver Nanoparticles with Different Shapes. Arabian J. Chem. 12 (8), 1823–1838. doi:10.1016/j.arabjc.2014.12.014
Kim, Y., Amaranatha Reddy, D., Park, H., and Kim, T. K. (2016). Anionic Precursor-Mediated Morphology-Controlled Synthesis of ZnS Nanostructures: Morphology-dependent Tunable Photoluminescence in the Visible Region and Pulsed Laser-Induced Efficient Reduction of Cr(VI). Ceramics Int. 42 (10), 12046–12054. doi:10.1016/j.ceramint.2016.04.132
Koutavarapu, R., Tamtam, M. R., Rao, M. C., Peera, S. G., and Shim, J. (2021). Recent Progress in Transition Metal Oxide/Sulfide Quantum Dots-Based Nanocomposites for the Removal of Toxic Organic Pollutants. Chemosphere 272, 129849. doi:10.1016/j.chemosphere.2021.129849
Kowsari, E., Pradhan, D., and Sohn, Y. (2017). “Carbon-Based Nanocomposites for Visible Light-Induced Photocatalysis,” in Nanocomposites for Visible Light-Induced Photocatalysis, 203–249. doi:10.1007/978-3-319-62446-4_8
Kozhevnikova, N. S., Maskaeva, L. N., Markov, V. F., and Vorokh, A. S. (2020). Effect of Chemical Reaction Mechanism on the Formation of ZnS Colloid Particles with Structure Disordering. Mater. Sci. Semiconductor Process. 113, 105047. doi:10.1016/j.mssp.2020.105047
Kuan, W.-F., Yu, W.-Y., Tu, F.-Y., Chung, C.-H., Chang, Y.-C., Lin, M. M., et al. (2022). Facile Reflux Preparation of Defective Mesoporous Ceria Nanorod with Superior Catalytic Activity for Direct Carbon Dioxide Conversion into Dimethyl Carbonate. Chem. Eng. J. 430 (P1), 132941. doi:10.1016/j.cej.2021.132941
Kumar, A., Sharma, M., Gautam, R. K., Agarwala, P., and Basu, S. (2017). Synthesis of Mesoporous Cerium Oxide (CeO2) Nanoparticles and Effect of Cerium Precursors on Transamidation of Acetamide with N-Octylamine under Solvent-free Conditions. j nanosci nanotechnol 17 (7), 4983–4988. doi:10.1166/jnn.2017.13744
Li, H., Liu, L., Wang, Z., Zheng, X., Meng, S., Chen, S., et al. (2018). Optimizing the Precursor of Sulfur Source for Hydrothermal Synthesis of High Performance CdS for Photocatalytic Hydrogen Production. RSC Adv. 8 (21), 11489–11497. doi:10.1039/C8RA00250A
Li, J., and Wu, N. (2015). Semiconductor-Based Photocatalysts and Photoelectrochemical Cells for Solar Fuel Generation: A Review. Catal. Sci. Technol. 5, 1360–1384. doi:10.1039/c4cy00974f
Lin, C.-Y., Zhang, J., and Xia, Z. (2019). Coordination-Dependent Catalytic Activity and Design Principles of Metal-Organic Frameworks as Efficient Electrocatalysts for Clean Energy Conversion. J. Phys. Chem. C 123 (1), 214–221. doi:10.1021/acs.jpcc.8b10375
Liu, B., Zhao, X., Terashima, C., Fujishima, A., and Nakata, K. (2014). Thermodynamic and Kinetic Analysis of Heterogeneous Photocatalysis for Semiconductor Systems. Phys. Chem. Chem. Phys. 16, 8751–8760. doi:10.1039/c3cp55317e
Liu, X., Wang, P., Zhang, Q., Huang, B., Wang, Z., Liu, Y., et al. (2018). Synthesis of MoS2/Ni3S2 Heterostructure for Efficient Electrocatalytic Hydrogen Evolution Reaction through Optimizing the Sulfur Sources Selection. Appl. Surf. Sci. 459, 422–429. doi:10.1016/j.apsusc.2018.08.024
Loh, J. Y. Y., and Kherani, N. P. (2019). X-Ray Photospectroscopy and Electronic Studies of Reactor Parameters on Photocatalytic Hydrogenation of Carbon Dioxide by Defect-Laden Indium Oxide Hydroxide Nanorods. Molecules 24 (3818), 3818. doi:10.3390/molecules24213818
Malik, R., Singh, C., Garg, R., Kumar, V., and Singhal, S. (2016). Morphology Controlled CdS Nanostructures: A Proficient Visible-Light-Driven Photocatalyst. Anal. Chem. Lett. 6 (5), 492–507. doi:10.1080/22297928.2016.1241718
Mallakpour, S., Nikkhoo, E., and Hussain, C. M. (2022). Application of MOF Materials as Drug Delivery Systems for Cancer Therapy and Dermal Treatment. Coord. Chem. Rev. 451, 214262. doi:10.1016/j.ccr.2021.214262
Matussin, S., Harunsani, M. H., Tan, A. L., and Khan, M. M. (2020). Plant-Extract-Mediated SnO2 Nanoparticles: Synthesis and Applications. ACS Sustain. Chem. Eng. 8 (8), 3040–3054. doi:10.1021/acssuschemeng.9b06398
Matussin, S. N., Harunsani, M. H., Tan, A. L., Mohammad, A., Cho, M. H., and Khan, M. M. (2020). Photoantioxidant Studies of SnO2 Nanoparticles Fabricated Using Aqueous Leaf Extract of Tradescantia Spathacea. Solid State. Sci. 105, 106279. doi:10.1016/j.solidstatesciences.2020.106279
Mishra, S. B., and Nanda, B. R. K. (2020). Facet Dependent Catalytic Activities of Anatase TiO2 for CO2 Adsorption and Conversion. Appl. Surf. Sci. 531, 147330. doi:10.1016/j.apsusc.2020.147330
M.S, R., Shanmuga Priya, S., Freudenberg, N. C., Sudhakar, K., and Tahir, M. (2021). Metal-Organic Framework-Based Photocatalysts for Carbon Dioxide Reduction to Methanol: A Review on Progress and Application. J. CO2 Utilization 43, 101374. doi:10.1016/j.jcou.2020.101374
Naidi, S. N., Khan, F., Tan, A. L., Harunsani, M. H., Kim, Y.-M., and Khan, M. M. (2021). Photoantioxidant and Antibiofilm Studies of green Synthesized Sn-Doped CeO2 Nanoparticles Using Aqueous Leaf Extracts of Pometia Pinnata. New J. Chem. 45 (17), 7816–7829. doi:10.1039/D1NJ00416F
Navya, P. N., and Daima, H. K. (2016). Rational Engineering of Physicochemical Properties of Nanomaterials for Biomedical Applications with Nanotoxicological Perspectives. Nano Convergence 3 (1). doi:10.1186/s40580-016-0064-z
Nicholas, N. J., Franks, G. v., and Ducker, W. A. (2012). Selective Adsorption to Particular Crystal Faces of ZnO. Langmuir 28 (18), 7189–7196. doi:10.1021/la2050674
Ozel, E., Tuncolu, I. G., Tuncolu, I. G., Aciksari, C., and Suvaci, E. (2016). Effect of Precursor Type on Zinc Oxide Formation and Morphology Development during Hydrothermal Synthesis. Hittite J. Sci. Eng. 3 (2), 73–80. doi:10.17350/HJSE19030000034
Panda, N. R., Acharya, B. S., and Nayak, P. (2013). Sonochemical Synthesis of Nitrogen Doped ZnO Nanorods: Effect of Anions on Growth and Optical Properties. J. Mater. Sci. Mater. Electron. 24 (10), 4043–4049. doi:10.1007/s10854-013-1359-z
Parwaiz, S., Khan, M. M., and Pradhan, D. (2019). CeO2-based Nanocomposites: An Advanced Alternative to TiO2 and ZnO in Sunscreens. mat express 9 (3), 185–202. doi:10.1166/mex.2019.1495
Popescu, M. (2006). Chalcogenides - Past, Present, Future. J. Non-Crystalline Sol. 352, 887–891. doi:10.1016/j.jnoncrysol.2005.11.126
Pourrahimi, A. M., Liu, D., Pallon, L. K. H., Andersson, R. L., Martínez Abad, A., Lagarón, J.-M., et al. (2014). Water-based Synthesis and Cleaning Methods for High Purity ZnO Nanoparticles - Comparing Acetate, Chloride, Sulphate and Nitrate Zinc Salt Precursors. RSC Adv. 4 (67), 35568–35577. doi:10.1039/c4ra06651k
Qi, K., Qin, Q., Duan, X., Wang, G., Wu, L., and Zheng, W. (2014). Geometric Matching Principle for Adsorption Selectivity of Ionic Liquids: A Simple Method into the Fascinating World of Shape-Controlled Chemistry. Chem. Eur. J. 20 (29), a–n. doi:10.1002/chem.201400409
Qi, K., Yang, J., Fu, J., Wang, G., Zhu, L., Liu, G., et al. (2013). Morphology-Controllable ZnO Rings: Ionic Liquid-Assisted Hydrothermal Synthesis, Growth Mechanism and Photoluminescence Properties. CrystEngComm 15 (34), 6729–6735. doi:10.1039/c3ce27007f
Qutub, N., Pirzada, B. M., Umar, K., and Sabir, S. (2016). Synthesis of CdS Nanoparticles Using Different Sulfide Ion Precursors: Formation Mechanism and Photocatalytic Degradation of Acid Blue-29. J. Environ. Chem. Eng. 4 (1), 808–817. doi:10.1016/j.jece.2015.10.031
Rahman, A., Harunsani, M. H., Tan, A. L., Ahmad, N., and Khan, M. M. (2021). Antioxidant and Antibacterial Studies of Phytogenic Fabricated ZnO Using Aqueous Leaf Extract of Ziziphus Mauritiana Lam. Chem. Pap. 75, 3295–3308. doi:10.1007/s11696-021-01553-7
Rahman, A., and Khan, M. M. (2021). Chalcogenides as Photocatalysts. New J. Chem. 45, 19622–19635. doi:10.1039/D1NJ04346C
Sabet, M., Salavati-Niasari, M., Ghanbari, D., Amiri, O., and Yousefi, M. (2013). Synthesis of CuInS2 Nanoparticles via Simple Microwave Approach and Investigation of Their Behavior in Solar Cell. Mater. Sci. Semiconductor Process. 16 (3), 696–704. doi:10.1016/j.mssp.2012.12.011
Sahay, P. P., Mishra, R. K., Pandey, S. N., Jha, S., and Shamsuddin, M. (2013). Structural, Dielectric and Photoluminescence Properties of Co-precipitated Zn-Doped SnO2 Nanoparticles. Curr. Appl. Phys. 13 (3), 479–486. doi:10.1016/j.cap.2012.09.010
Samiee, S., and Goharshadi, E. K. (2012). Effects of Different Precursors on Size and Optical Properties of Ceria Nanoparticles Prepared by Microwave-Assisted Method. Mater. Res. Bull. 47 (4), 1089–1095. doi:10.1016/j.materresbull.2011.12.058
Sanjini, N. S., Winston, B., and Velmathi, S. (2017). Effect of Precursors on the Synthesis of CuO Nanoparticles under Microwave for Photocatalytic Activity towards Methylene Blue and Rhodamine B Dyes. J. Nanosci Nanotechnol 17 (1), 495–501. doi:10.1166/jnn.2017.11785
Segets, D., Marczak, R., Schäfer, S., Paula, C., Gnichwitz, J.-F., Hirsch, A., et al. (2011). Experimental and Theoretical Studies of the Colloidal Stability of Nanoparticles−A General Interpretation Based on Stability Maps. ACS Nano 5 (6), 4658–4669. doi:10.1021/nn200465b
Shi, Y., Yang, A.-F., Cao, C.-S., and Zhao, B. (2019). Applications of MOFs: Recent Advances in Photocatalytic Hydrogen Production from Water. Coord. Chem. Rev. 390, 50–75. doi:10.1016/j.ccr.2019.03.012
Siegfried, M. J., and Choi, K.-S. (2005). Directing the Architecture of Cuprous Oxide Crystals during Electrochemical Growth. Angew. Chem. Int. Ed. 44 (21), 3218–3223. doi:10.1002/anie.200463018
Singh, P. K., Mukherjee, S., Ghosh, C. K., and Maitra, S. (2017). Influence of Precursor Type on Structural, Morphological, Dielectric and Magnetic Properties of TiO2 Nanoparticles. Cerâmica 63 (368), 549–556. doi:10.1590/0366-69132017633682145
Soren, S., Jena, S. R., Samanta, L., and Parhi, P. (2015). Antioxidant Potential and Toxicity Study of the Cerium Oxide Nanoparticles Synthesized by Microwave-Mediated Synthesis. Appl. Biochem. Biotechnol. 177 (1), 148–161. doi:10.1007/s12010-015-1734-8
Soto Morillo, E., Mota Toledo, N., García Fierro, J. L., and Navarro Yerga, R. M. (2020). Role of the Sulphur Source in the Solvothermal Synthesis of Ag-CdS Photocatalysts: Effects on the Structure and Photoactivity for Hydrogen Production. Hydrogen 1 (1), 64–89. doi:10.3390/hydrogen1010005
Sun, D., Wong, M., Sun, L., Li, Y., Miyatake, N., and Sue, H.-J. (2007). Purification and Stabilization of Colloidal ZnO Nanoparticles in Methanol. J. Sol-gel Sci. Technol. 43 (2), 237–243. doi:10.1007/s10971-007-1569-z
Sun, Z., Wu, X., Guan, D., Chen, X., Dai, J., Gu, Y., et al. (2021). One Pot-Synthesized Ag/Ag-Doped CeO2 Nanocomposite with Rich and Stable 3D Interfaces and Ce3+ for Efficient Carbon Dioxide Electroreduction. ACS Appl. Mater. Inter. 13, 59993–60001. doi:10.1021/acsami.1c19529
Tang, A., Hu, Z., Yin, Z., Ye, H., Yang, C., and Teng, F. (2015). One-pot Synthesis of CuInS2 Nanocrystals Using Different Anions to Engineer Their Morphology and crystal Phase. Dalton Trans. 44 (19), 9251–9259. doi:10.1039/c5dt01111f
van Rijt, M. M. J., Oosterlaken, B. M., Joosten, R. R. M., Wijkhuijs, L. E. A., Bomans, P. H. H., Friedrich, H., et al. (2020). Counter-Ion Influence on the Mechanism of HMTA-Mediated ZnO Formation. CrystEngComm 22 (35), 5854–5861. doi:10.1039/d0ce00847h
Wang, H., Liu, Y., Li, M., Huang, H., Xu, H. M., Hong, R. J., et al. (2010). Multifunctional TiO2nanowires-Modified Nanoparticles Bilayer Film for 3D Dye-Sensitized Solar Cells. Optoelectronics Adv. Mater. Rapid Commun. 4 (8), 1166–1169. doi:10.1039/b000000x
Wang, Y., Chen, W., Chen, X., Feng, H., Shen, D., Huang, B., et al. (2018). Effect of Sulfur Source on Photocatalytic Degradation Performance of CdS/MoS2 Prepared with One-step Hydrothermal Synthesis. J. Environ. Sci. 65, 347–355. doi:10.1016/j.jes.2017.07.004
Wu, Q., Zhang, F., Xiao, P., Tao, H., Wang, X., Hu, Z., et al. (2008). Great Influence of Anions for Controllable Synthesis of CeO2 Nanostructures: From Nanorods to Nanocubes. J. Phys. Chem. C 112 (44), 17076–17080. doi:10.1021/jp804140e
Yang, L., Chen, C., Rui, Z., and Ji, H. (2020). Anodic Aluminum Oxide Supported Pd@CeO2 Catalyst for Organic Gas Pollutants Removal with an Enhanced Performance. Catal. Today 355 (March), 602–607. doi:10.1016/j.cattod.2019.04.065
Yang, S., and Gao, L. (2006). Controlled Synthesis and Self-Assembly of CeO2 Nanocubes. J. Am. Chem. Soc. 128 (29), 9330–9331. doi:10.1021/ja063359h
Yin, L., Zhang, D., Wang, D., Wang, F., Huang, J., Kong, X., et al. (2016). Controlled Synthesis of Sm2O3 Nano/microstructures with New Morphology by Hydrothermal-Calcination Process. Ceramics Int. 42 (10), 11998–12004. doi:10.1016/j.ceramint.2016.04.126
Yordanov, G. G., Adachi, E., and Dushkin, C. D. (2006). Growth Kinetics and Characterization of Fluorescent CdS Nanocrystals Synthesized with Different Sulfur Precursors in Paraffin Hot-Matrix. Colloids Surf. A: Physicochemical Eng. Aspects 289 (1–3), 118–125. doi:10.1016/j.colsurfa.2006.04.019
Zeng, X., Pramana, S. S., Batabyal, S. K., Mhaisalkar, S. G., Chen, X., and Jinesh, K. B. (2013). Low Temperature Synthesis of Wurtzite Zinc Sulfide (ZnS) Thin Films by Chemical Spray Pyrolysis. Phys. Chem. Chem. Phys. 15 (18), 6763. doi:10.1039/c3cp43470b
Zhang, X., Wei, Y., Song, Z., Liu, W., Gao, C., and Luo, J. (2021). Silicotungstic Acid Modified CeO2 Catalyst with High Stability for the Catalytic Combustion of Chlorobenzene. Chemosphere 263, 128129. doi:10.1016/j.chemosphere.2020.128129
Zhang, Z., Yue, C., and Hu, J. (2017). Fabrication of Porous MoS2 with Controllable Morphology and Specific Surface Area for Hydrodeoxygenation. Nano 12 (09), 1750116. doi:10.1142/S1793292017501168
Zhou, R., Zhou, R., Alam, D., Zhang, T., Li, W., Xia, Y., et al. (2021). Plasmacatalytic Bubbles Using CeO2 for Organic Pollutant Degradation. Chem. Eng. J. 403 (June), 126413. doi:10.1016/j.cej.2020.126413
Keywords: semiconductors, metal oxides, chalcogenides, shaped-dependent properties, Anion directed crystal growth
Citation: Matussin SN, Rahman A and Khan MM (2022) Role of Anions in the Synthesis and Crystal Growth of Selected Semiconductors. Front. Chem. 10:881518. doi: 10.3389/fchem.2022.881518
Received: 22 February 2022; Accepted: 31 March 2022;
Published: 25 April 2022.
Edited by:
Wee-Jun Ong, Xiamen University Malaysia, MalaysiaReviewed by:
Quanjun Xiang, University of Electronic Science and Technology of China, ChinaMetwally Madkour, Kuwait University, Kuwait
Kezhen Qi, Dali University, China
Lutfi Kurnianditia Putri, Monash University Malaysia, Malaysia
Copyright © 2022 Matussin, Rahman and Khan. This is an open-access article distributed under the terms of the Creative Commons Attribution License (CC BY). The use, distribution or reproduction in other forums is permitted, provided the original author(s) and the copyright owner(s) are credited and that the original publication in this journal is cited, in accordance with accepted academic practice. No use, distribution or reproduction is permitted which does not comply with these terms.
*Correspondence: Mohammad Mansoob Khan, mmansoobkhan@yahoo.com, mansoob.khan@ubd.edu.bn