- 1Departamento de Química, Universidade Federal de Santa Catarina—UFSC, Florianópolis, Brazil
- 2Instituto de Química, Universidade Federal do Mato Grosso do Sul.—UFMS, Campo Grande, Brazil
- 3Departamento de Química, Universidade do Estado de Santa Catarina, Joinville, Brazil
- 4Instituto de Química, Universidade Federal de Goiás—UFG, Goiânia, Brazil
- 5Department of Chemical Sciences, Faculty of Science, University of Johannesburg, Doornfontein, South Africa
We report an electrochemical oxidative intramolecular cyclization reaction between 2-alkynylphenol derivatives and different diselenides species to generate a wide variety of substituted-benzo[b]furans. Driven by the galvanostatic electrolysis assembled in an undivided cell, it provided efficient transformation into oxidant-, base-, and metal-free conditions in an open system at room temperature. With satisfactory functional group compatibility, the products were obtained in good to excellent yields.
Introduction
The benzo[b]furan core is present in several derivatives of natural products, containing various types of biological activities (Heravi et al., 2017). Many drugs and candidates for clinical drugs have this nucleus (Miao et al., 2019; Radadiya et al., 2015), such as bufuralol, ailanthoidol, benziodarone, nonekenetin, and cloridarol, as shown in Figure 1 (Asif 2016; Tang et al., 2021). The reported therapeutic activities include antitumor (Romagnoli et al., 2015; Xu et al., 2017), antidepressant (Boukharsa et al., 2016), anti-inflammatory (Xie et al., 2014), antioxidant (Chand et al., 2017), and fungicide (Liang et al., 2016) activities and may also inhibit the formation of amyloid plaques that are characteristic of Alzheimer’s disease (Hiremathad et al., 2018).
Similarly, the construction of the C–Se bond is among the important transformation in organic synthesis (Rafique et al., 2016b; Rafique et al., 2021), mainly due to their properties such as synthetic intermediates in organic transformations (Shao et al., 2019; Arora et al., 2021) and material sciences (Li et al., 2020) as well as in the medicinal chemistry (Nogueria et al., 2021). In the past few decades, these compounds have gained increasing interest, mainly due to their antioxidant (Mugesh and Singh 2000; Botteselle et al., 2021), anti-Alzheimer (Rodrigues et al., 2018; Scheide et al., 2020b; Kumawat et al., 2021), anti-inflammatory (He et al., 2021), antitumor (Spengler et al., 2019; Chen et al., 2020; Dos Santos et al., 2021; Santos et al., 2022), antiviral (Ali et al., 2021), and other biological activities (Wang et al., 2016; Frizon et al., 2020; Rafique et al., 2020; Galant et al., 2021; Martín-Escolano et al., 2021; Veloso et al., 2021).
Considering the biological relevance of benzo[b]furans and the wide spectrum of therapeutic properties of organoselenides, there are few synthetic methods that are available to access organo-selenylbenzo[b]furans. The most frequent approaches are cyclization reactions using 2-alkynylphenols or 2-alkynyl-alkoxybenzenes. In 2005, Larock and co-workers reported the synthesis of disubstituted benzo[b]furans through the cyclization of 2-alkynylanisols in the presence of an electrophilic species of chalcogen (Yue et al., 2005). Zeni and co-workers had developed another approach, which involved cyclization of 2-chalcogenealkynyl anisoles by I2, Br2, and PhSeBr as electrophilic mediators (Manarin et al., 2009). In the same year, Li and co-workers proposed a palladium-promoted annulation reaction of 2-alkynylphenol derivatives with diselenides or disulfides and iodides (Du et al., 2009). In 2010, Zeni and co-workers also reported a FeCl3-diorganyl dichalcogenide-promoted cyclization of 2-alkynylanisoles (Gay et al., 2010). Liu and co-workers proposed the synthesis of 3-selenylbenzo[b]furans via AgNO2-catalyzed radical cyclization of 2-alkynylanisoles or 2-alkynylthioanisoles, elemental Se, and arylboronic acids (An et al., 2019). Zhong and co-workers reported the synthesis of 3-chalcogen-benzo[b]furans via the I2-mediated annulation reaction of 2-alkynylanisoles (Han et al., 2013). Recently, Silva and co-workers reported the synthesis of 3-selenylbenzo[b]furans mediated by the Selectfluor® (Xavier et al., 2020). Arsenyan and co-workers developed the synthesis of benzo[b]furans and indoles bearing short selenocysteine-containing peptides (Lapcinska et al., 2020), and Xu and co-workers described an electrochemical oxidative cyclization of oximes with diselenides (Gao et al., 2021).
In recent years, organic electrochemistry has emerged as an attractive and suitable approach (Martins et al., 2019a; Martins et al., 2020; Scheide et al., 2021; Huang et al., 2022). Such reactions are economically attractive, requiring only an electric current as a redox medium (Cembellín and Cembellín 2021). In this regard, with the use of electrochemistry, alkyne functionalization in single-stage mode and cyclization have been showing high efficiency, being carried out under milder conditions (Martins et al., 2019b).
Thus, in connection with our continuing interest in designing and developing eco-friendly processes (Godoi et al., 2013; Matzkeit et al., 2018; Peterle et al., 2018; Scheide et al., 2020a; Neto et al., 2020; Saba et al., 2020; Franco et al., 2021) and electrochemical selenylation reactions (Meirinho et al., 2019; Lazzaris et al., 2021; Scheide et al., 2021), we report the synthesis of selenylbenzo[b]furan derivatives through an electrochemical oxidative intramolecular cyclization reaction between 2-alkynylphenol derivatives and different diorganyl diselenides. This sustainable approach operates in shorter reaction time, providing the selenylated products in good to excellent yields.
Results and Discussion
Initially, reaction optimization was performed to determine the optimum reaction conditions; the results are presented in Table 1 (see ESI, S1 for complete optimization table). In entries 1–5, different supporting electrolytes (TBAI, TBAPF6, TBABF4, LiClO4, and TBAClO4) were evaluated, in which the most appropriate was tetrabutylammonium perchlorate (TBAClO4). The amount of electrolyte was analyzed, varying from 0.4 equiv. to 0.3 equiv., and a slight decrease in yield was observed (entry 6). In entry 7, the equivalence of diphenyl diselenide (2a) has been reduced by 1 equiv. to 0.75 equiv., and a decrease in yield was obtained.
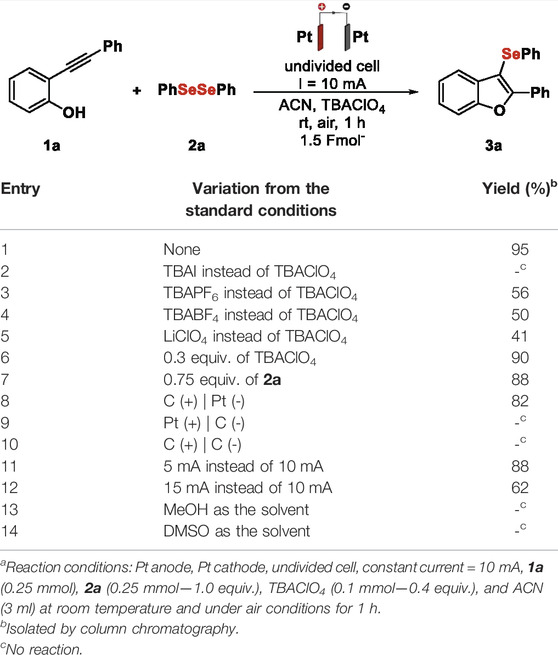
TABLE 1. Optimization of the reaction conditions.a
We emphasize that by applying graphite electrodes, the transformation was not efficient (entries 8–10), obtaining lower yields or no reaction progress. Considering different electrical currents, with 5 mA, a slight reduction in performance was observed (entry 11). Additionally, when the current was increased to 15 mA, a substantial decrease in efficiency was observed (entry 12). Finally, evaluating different solvents, with the application of methanol or dimethyl sulfoxide (entries 13 and 14), in both cases, the reaction did not proceed, and the starting material was completely recovered.
Under the optimal reaction conditions in hand, the substrate scope of intramolecular cyclization between 2-alkynylphenol derivatives and different diselenides was evaluated (Scheme 1). Initially, diphenyl diselenides bearing electron-donor and electron-withdrawing groups as well as aliphatic and thiophene diselenides were subjected to transformation, providing the corresponding product yields up to 98%. The reaction proceeded smoothly for diselenides containing the methoxy group, and derivative 3k was obtained with 50% yield. The method showed great compatibility with the electron-withdrawing groups, being suitable for F, Cl, and CF3 substituents. Substituent groups in the phenolic ring did not affect the reaction progress, delivering the cyclized products in yields of up to 95% (3 g and 3 h). Aliphatic diselenide was used successfully, providing the product 3o with 78% yield. However, with the thiophene diselenide, the yield decreased, affording the selenylated product 3p with 31% yield. However, for the synthesis of product 3q, with the thiophene heterocycle, the reaction proved to be efficient, delivering the product with 91% yield. It was observed that the transformation is not limited only to cyclizations from 2-alkynylphenols (1a–d), and the use of methoxy-2-(phenylethynyl)benzene (1e) was appropriate, providing products 3a–c and 3o with yields of up to 78%, under the same reaction conditions.
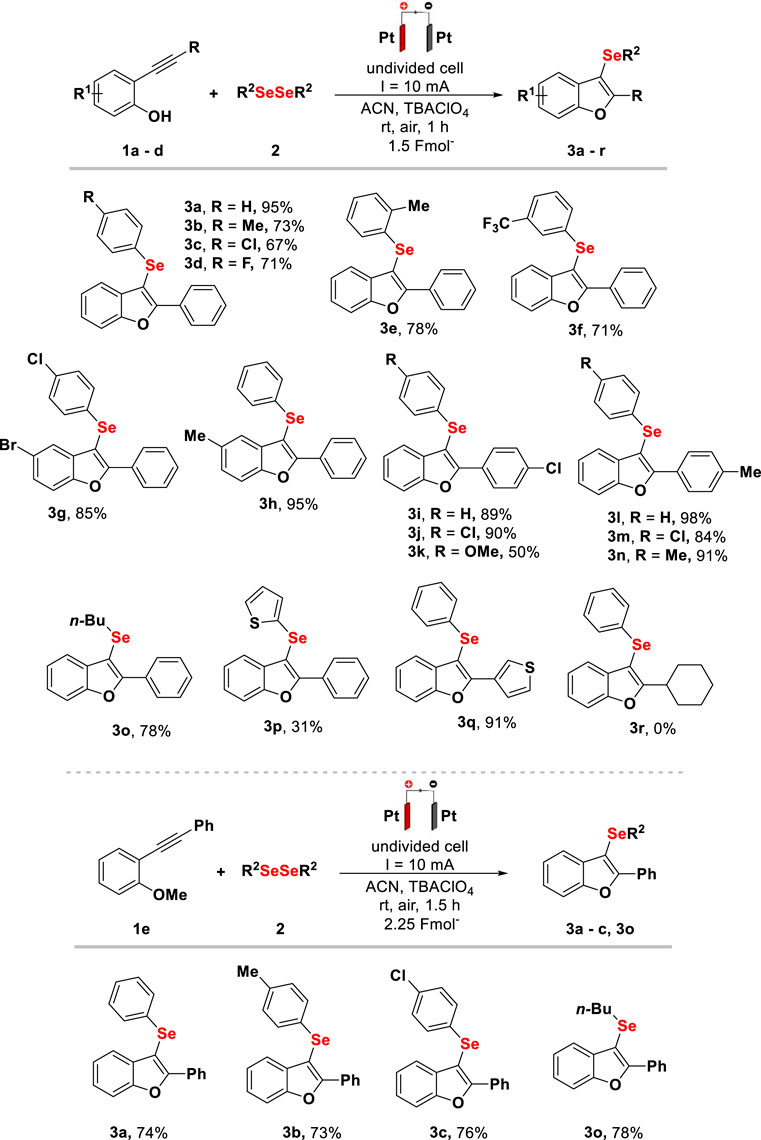
Scheme 1. Scope and limitations for electrochemical synthesis of selenylbenzo[b]furans (3). Reaction conditions: platinum electrodes, constant current (10 mA), 1 (0.25 mmol), 2 (0.25 mmol), TBAClO4 (0.1 mmol), ACN (3 ml), rt, and air. Isolated by column chromatography.
In order to expand the reaction scope, the use of 2-[(trimethylsilyl)ethynyl]phenol (1f) was evaluated, and to our delight, the bis-selenylation product was observed, as shown in Scheme 2, 4a. The need of 1.4 equivalent of diselenide for the complete conversion of 1f into 4a was observed. For a better understanding, the method was extended to the synthesis of different 2,3-bis-organochalcogenyl-benzo [b]chalcogenophenes (4a, 4b, and 4c), varying the diselenides, reaching yields of up to 72% in 1.5 h. Additionally, the use of 2-[(phenylselanyl)ethynyl]phenol (1g) and 2-[(phenylthio)ethynyl]phenol (1h) was evaluated, which provided 2,3-bis-organochalcogenyl-benzo [b]chalcogenophenes (4a’ and 4 days) with yields up to 88%, using 1.0 equivalent of diselenides, in 1 h of reaction time.
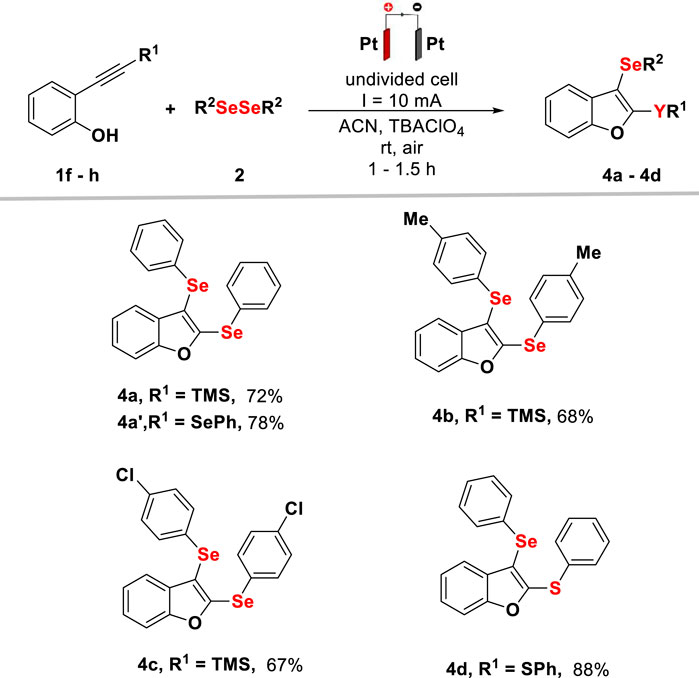
Scheme 2. Scope and limitations for electrochemical synthesis of selenylbenzo[b]furans (4). Reaction conditions: platinum electrodes, constant current (10 mA), 1 (0.25 mmol), 2 (0.35 mmol for R1 = TMS, 0.25 mmol for R1 = SePh and R1 = SPh), TBAClO4 (0.1 mmol), ACN (3 ml), rt, and air. Isolated by column chromatography.
To evaluate the applicability of the present method, the electrochemical intramolecular cyclization of 2-(phenylethynyl)phenol 1a with diphenyl diselenide 2a was carried out in gram-scale synthesis (5 mmol), affording product 3a with 45% yield after 20 h; Scheme 3. The cyclic voltammetry of 3a (ESI S5†) shows an oxidation peak at Epa 1.68 V (vs. NHE), which may be associated with a process of degradation of the selenylated product, resulting in a lower yield for the gram-scale procedure. This suggests that to increase the reaction scale efficiently, it is recommended to enlarge the area of the electrodes, reducing the reaction time.
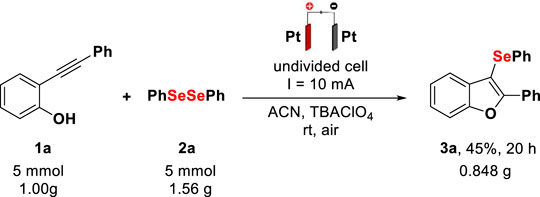
Scheme 3. Gram-scale synthesis. Reaction conditions: platinum electrodes, constant current (10 mA), 1a (5 mmol), 2a (5 mmol), TBAClO4 (2 mmol), ACN (60 ml), rt, and air. Isolated by column chromatography.
For a better understanding of the reaction mechanism, a series of control experiments were performed; Scheme 4. When the radical scavenger TEMPO was used under standard conditions, the reaction was completely inhibited, and no product was observed (A). This observation suggests that a radical is formed in at least one step of the reaction mechanism. The use of an inert atmosphere had no impact on the yield, which implies that atmospheric oxygen does not participate in the reaction mechanism (B). The use of 0.5 equivalent of diphenyl diselenide (2a) proved to be inefficient, delivering product 3a with 75% yield (C). Finally, we applied PhSeBr as a previously synthesized electrophilic source, with the formation of product 3a with only 18% yield after 1 h, without electric current, which suggests that an electrophilic form of organoselenium may be involved in the mechanism (D).
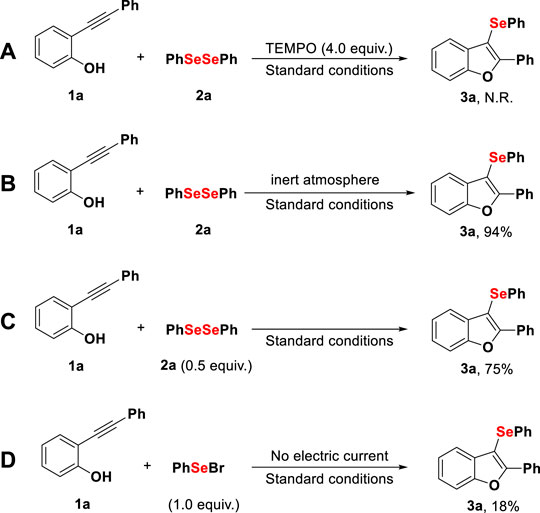
Scheme 4. Control experiments. Standard conditions: platinum electrodes, constant current (10 mA), 1a (0.25 mmol), 2a (0.25 mmol), TBAClO4 (0.1 mmol), ACN (3 ml), 1 h, rt, and air. Isolated by column chromatography. N.R.: no reaction.
Normalized cyclic voltammograms of selected compounds are shown in ESI S5†, and they allowed us to obtain more information regarding the redox potentials involved in the catalytic process studied. Diphenyl diselenide 2a presented an irreversible anodic peak potential (Epa) at 1.55 V in ACN solution that is in line with the study previously reported by Kunai et al (1983), which suggests the formation of radical stages, explaining that the control experiment was carried out, as shown in Scheme 4—A. Recently, Wilken et al (2018) reported that the RSe+ species is not the main catalytically active intermediate in redox reactions using aryl diselenides. This statement is in accordance with a control experiment (D), which under standard conditions without the use of electrical energy, delivered product 3a in a low reaction yield. Additionally, 1a showed an irreversible Epa at 1.60 V, attributed to the deprotonation of phenol or radical formation in oxygen, suggesting the reaction pathway via radical, as previously proposed (Enache et al., 2011).
Although the fine details of the reaction mechanism remain unknown, several aspects observed during the control experiments (Scheme 4), normalized cyclic voltammograms (ESI S5†), and previous reports (Azeredo et al., 2014; Manarin et al., 2009; Nascimento et al., 2012; Rafique et al., 2016a; Saba et al., 2015; Saba et al., 2016; Silveira et al., 2012; Xavier et al., 2020) guided us to propose a plausible mechanism (Scheme 5). Considering this, two reactional pathways can be proposed. Pathway I: it is known that diphenyl diselenide (2a) may be involved in oxidation and reduction processes in the electrocatalytic cycle, suggesting the possibility of the reaction starting with the formation of an intermediate cationic radical A via anodic oxidation. In parallel, the anodic oxidation of 1a would promote the radical species D, which after addition at the sp carbon forms the intermediate E, followed by an addition of B, which delivers the desired product 3a. Moreover, the diselenide can be involved in both processes (oxidation and reduction) under electrochemical conditions, as evidenced in CV (ESI S5†) and in the literature. So, we do not rule out the possibility of the formation of the radical species B via cathodic reduction, as suggested by Guan et al (2019) and Gao et al (2021). Considering the control reactions, it was observed that the medium was completely inhibited by the addition of 4.0 equiv. of the TEMPO radical scavenger (entry A, Scheme 4), indicating that this process possibly occurs via a radical pathway. Pathway II: however, we cannot rule out the pathway through the phenyl selenium cation C. Through the formation of a reversible seleniranium intermediate F, followed by nucleophilic intramolecular attack, product 3a is formed. This pathway was elucidated through control experiments (entry D, Scheme 4).
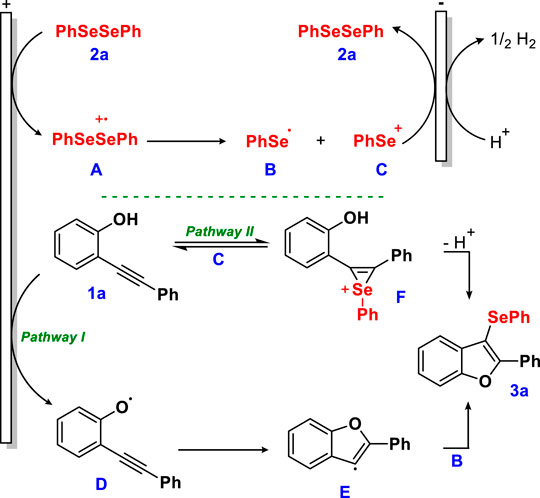
Scheme 5. Proposed mechanism for the electrochemical intramolecular cyclization of 2-alkynylphenols with diselenides.
Considering the importance of selenoxide derivatives, we propose the synthesis of 2-phenyl-3-(phenylseleninyl)benzofuran (5a) starting from 3a, as shown in Scheme 6. NCS was applied as an oxidizer (Weilbeer et al., 2016), and the desired selenoxide product 5a was obtained with 88% yield.
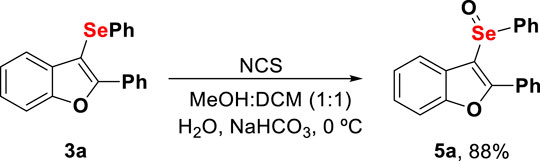
Scheme 6. Functionalization of 3-selenylbenzo[b]furan derivatives. Reaction conditions: 3a (0.5 mmol, 1.0 equiv), NCS (1.05 equiv.), 0.07 M in MeOH/CH2Cl2 (1:1), 0°C, 30 min, NaHCO3 (10 ml/1.0 mmol), 0°C, 15 min, and H2O (15 ml/1.0 mmol), 0°C, 15 min.
Conclusion
In summary, we have developed an efficient regioselective electrochemical synthesis of selenylbenzo[b]furan derivatives through the cyclization of 2-alkynylphenols. This procedure, driven by the galvanostatic electrolysis using platinum electrodes assembled in an undivided cell, provided efficient transformation into oxidant-free, base-free, and transition metal-free conditions in an open system at room temperature. The method was proved to be robust and can be applied at gram-scale. Additionally, a wide applicability of the present method was observed, being able to be applied in the synthesis of 2,3-bis-organochalcogenyl-benzo[b]chalcogenophenes.
Materials and Methods
General Information
1H and 13C NMR spectra were recorded on Bruker 400 and Bruker AC 200 spectrometers, respectively, with the samples dissolved in CDCl3. Chemical shifts are reported in ppm downfield from the signal of TMS, used as the internal standard, and the coupling constants (J) are expressed in Hertz (Hz). The following abbreviations were reported for multiplicity of signal: s (singlet), d (doublet), t (triplet), q (quartet), quint (quintet), sext (sextet), and m (multiplet). High-resolution mass spectroscopy was record on Xevo G2-S QTOF (Waters) on ESI+ and ESI− modes. The reactions were monitored by thin layer chromatography (TLC), and Macherey-Nagel silica gel 818333 of 0.20 mm thickness was used. For visualization, UV fluorescence, an iodine chamber, and acidic methanolic vanillin solution (5% in 10% H2SO4) were used. An Aldrich technical grade silica gel (pore size 60 Å, 230–400 mesh) was used for flash chromatography. The instruments used for electrochemical studies are BK Precision 1739 V/1A DC power supply with 0.1 mA settable resolution. The anode and cathode platinum plate electrodes (1.0 × 1.0 cm2) were used.
General Procedure of the Electrochemical Setup
To a test tube were added 2-(phenylethynyl)phenol (1a, 0.25 mmol), diaryl or dialkyl diselenide (2, 0.25 mmol), TBAClO4 (0.1 mmol), and 3.0 ml CH3CN at room temperature under stirring. The flask was equipped with platinum electrodes (1.0 × 1.0 cm2) as the anode and cathode. The reaction mixture was electrolyzed under a constant current mode (10.0 mA). The reaction progress was monitored by TLC. After the total consumption of starting materials, the solvent was removed under reduced pressure to yield a crude mixture from which the final product was isolated through flash column chromatography with a silica gel as the stationary phase and eluated with a mixture of hexane and ethyl acetate.
2-Phenyl-3-(phenylselanyl)benzofuran (3a) (Xavier et al., 2020)
White solid (84.1 mg, 95% yield): 1H NMR (200 MHz, CDCl3) δ 8.17 (d, J = 7.5 Hz, 2H), 7.46 (d, J = 8.0 Hz, 2H), and 7.40–6.94 (m, 10H). 13C NMR (50 MHz, CDCl3) δ 157.4, 154.3, 132.1, 131.6, 130.3, 129.5, 129.4, 128.6, 128.0, 126.4, 125.4, 123.6, 121.5, 111.4, and 100.0.
2-Phenyl-3-(p-tolylselanyl)benzofuran (3b) (Xavier et al., 2020)
Yellow solid (66.4 mg, 73% yield): 1H NMR (400 MHz, CDCl3) δ 8.32–8.25 (m, 2H), 7.66–7.34 (m, 5H), 7.33–7.22 (m, 3H), 7.04 (d, J = 8.0 Hz, 2H), and 2.30 (s, 3H). 13C NMR (101 MHz, CDCl3) δ 157.0, 154.1, 136.2, 132.0, 130.2, 130.1, 129.6, 129.2, 128.5, 127.8, 127.5, 125.2, 123.4, 121.3, 111.1, 100.2, and 21.0.
3-[(4-Chlorophenyl)selanyl]-2-phenylbenzofuran (3c) (Xavier et al., 2020). White solid (64.3 mg, 67% yield): 1H NMR (400 MHz, CDCl3) δ 8.26–8.18 (m, 2H), 7.60 (d, J = 8.0 Hz, 1H), 7.55–7.35 (m, 5H), and 7.31–7.14 (m, 5H). 13C NMR (100 MHz, CDCl3) δ 157.4, 154.1, 132.3, 131.6, 130.4, 129.9, 129.6, 129.4, 129.4, 128.5, 127.8, 125.4, 123.5, 121.0, 111.3, and 99.4.
3-[(4-Fluorophenyl)selanyl]-2-phenylbenzofuran (3d) (An et al., 2019). White solid (65.3 mg, 71% yield): 1H NMR (400 MHz, CDCl3) δ 8.31–8.15 (m, 2H), 7.59 (d, J = 8.0 Hz, 1H), 7.55–7.21 (m, 8H), and 6.97–6.83 (m, 2H). δ 13C NMR (100 MHz, CDCl3) δ 161.5 (d, JC-F = 246.0 Hz), 157.1, 154.1, 131.7, and 131.4 (d, JC-F = 7.5 Hz), 130.1, 129.4, 128.5, 127.8, and 125.6 (d, JC-F = 3.0 Hz), 125.3, 123.5, 121.1, and 116.5 (d, JC-F = 2.0 Hz), 111.3, and 100.1.
2-Phenyl-3-(o-tolylselanyl)benzofuran (3e). Yellow solid (70.9 mg, 78% yield): 1H NMR (400 MHz, CDCl3) δ 8.29–8.18 (m, 2H), 7.62 (d, J = 8.0 Hz, 1H), 7.56–7.36 (m, 5H), 7.27 (dd, J = 8.0, 6.5 Hz, 1H), 7.21 (d, J = 7.5 Hz, 1H), 7.10 (td, J = 7.5, 1.5 Hz, 1H), 7.04–6.89 (m, 2H), and 2.53 (s, 3H). 13C NMR (100 MHz, CDCl3) δ 157.6, 154.2, 136.7, 132.0, 131.9, 130.2, 130.1, 129.3, 128.5 128.4, 127.8, 126.8, 126.0, 125.2, 123.4, 121.2, 111.2, 99.1, and 21.4. HRMS-ESI [M + H]+ calcd. for C21H17OSe: 365.0445, found 365.0446.
2-Phenyl-3-{[3-(trifluoromethyl)phenyl]selanyl}benzofuran (3f) (Xavier et al., 2020). Yellow solid (74.2 mg, 71% yield): 1H NMR (400 MHz, CDCl3) δ 8.26–8.13 (m, 2H), 7.67–7.57 (m, 2H), 7.58–7.33 (m, 6H), and 7.27 (dt, J = 12.5, 7.5 Hz, 2H). 13C NMR (100 MHz, CDCl3) δ 157.9, 154.3, 135.0, 132.9, 132.2, 131.8, and 131.6 (2xC), 131.5, 131.4, 130.0, 129.8, 129.8, 129.7, 128.7, 127.9, 125.7, 125.7, 125.7, and 125.6 (2x), 125.1, 125.1, 125.0, 125.0, 125.0, 123.8, 123.2, 123.2, 123.2, 123.1, 122.4, 121.0, 111.5, and 98.9.
5-Bromo-3-[(4-chlorophenyl)selanyl]-2-phenylbenzofuran (3g). White solid (98.1 mg, 85% yield): 1H NMR (200 MHz, CDCl3) δ 7.97–7.82 (m, 2H), 7.36–7.35 (m, 1H), 7.26–7.08 (m, 5H), and 7.00–6.78 (m, 4H). 13C NMR (50 MHz, CDCl3) δ 158.6, 152.8, 133.8, 132.6, 130.4, 129.8, 129.5, 129.4, 129.1, 128.5, 128.3, 127.8, 123.6, 116.8, 112.7, and 98.7. HRMS-APCI [M]+ calcd. for C20H12BrClOSe: 461.8925, found 461.8902.
5-Methyl-2-phenyl-3-(phenylselanyl)benzofuran (3h) (Gay et al., 2010). Yellow solid (86.5 mg, 95% yield): 1H NMR (200 MHz, CDCl3) δ 8.12 (dd, J = 7.5, 2.0 Hz, 2H), 7.59–7.44 (m, 2H), 7.37–7.06 (m, 9H), and 2.38 (s, 3H). 13C NMR (50 MHz, CDCl3) δ 157.6, 154.0, 139.4, 132.04, 131.5, 129.2, 129.1, 127.7, 127.3, 126.1, 125.0, 123.37, 121.0, 111.1, 98.9, and 21.4.
2-(4-Chlorophenyl)-3-(phenylselanyl)benzofuran (3i) (Xavier et al., 2020). Yellow solid (85.4 mg, 89% yield): 1H NMR (200 MHz, CDCl3) δ 8.23–8.09 (m, 2H) and 7.60–7.07 (m, 11H). 13C NMR (50 MHz, CDCl3) δ 155.9, 154.0, 135.2, 131.8, 131.1, 129.3, 129.2, 128.9, 128.7, 128.6, 126.4, 125.5, 123.5, 121.2, 111.1, and 100.2.
2-(4-Chlorophenyl)-3-[(4-chlorophenyl)selanyl]benzofuran (3j). White solid (94.0 mg, 90% yield): 1H NMR (200 MHz, CDCl3) δ 7.88 (d, J = 9.0, 2.0 Hz, 2H) and 7.34–6.79 (m, 10H). 13C NMR (50 MHz, CDCl3) δ 156.0, 154.0, 135.4, 132.5, 131.5, 130.5, 129.5, 129.3, 128.8, 128.4, 125.6, 123.7, 121.0, 111.3, and 99.9. HRMS-APCI [M]+ calcd. for C20H12BrClOSe: 461.8925, found 461.8902.
2-(4-Chlorophenyl)-3-[(4-methoxyphenyl)selanyl]benzofuran (3k). White solid (51.6 mg, 50% yield): 1H NMR (200 MHz, CDCl3) δ 8.19 (d, J = 9.0 Hz, 2H), 7.56–7.17 (m, 8H), 6.72 (d, J = 9.0 Hz, 2H), and 3.71 (s, 3H). 13C NMR (50 MHz, CDCl3) δ 158.9, 155.2, 153.9, 135.1, 131.9, 128.8, 128.6, 125.3, 123.4, 121.2, 120.7, 115.1, 111.1, 101.5, and 55.2. HRMS-ESI [M + OH]+ calcd. for C21H16ClO3Se: 430.9953, found 430.9798.
3-(Phenylselanyl)-2-(p-tolyl)benzofuran (3L) (Xavier et al., 2020). White solid (87.0 mg, 98%): 1H NMR (200 MHz, CDCl3) δ 8.10 (d, J = 8.2 Hz, 2H), 7.51 (t, J = 7.6 Hz, 2H), 7.37–7.00 (m, 9H), and 2.38 (s, 3H). 13C NMR (50 MHz, CDCl3) δ 157.6, 154.1, 139.5, 132.0, 131.6, 129.2, 129.1, 127.7, 127.3, 126.2, 124.9, 123.4, 121.1, 111.1, 98.8, and 21.4.
3-[(4-Chlorophenyl)selanyl]-2-(p-tolyl)benzofuran (3m). Yellow solid (84.5 mg, 84%): 1H NMR (200 MHz, CDCl3) δ 8.10 (d, J = 8.0 Hz, 2H), 7.51 (t, J = 7.5 Hz, 2H), 7.37–6.95 (m, 8H), and 2.38 (s, 3H). 13C NMR (50 MHz, CDCl3) δ 157.6, 154.0, 139.5, 132.0, 131.6, 129.2, 127.7, 127.3, 126.2, 124.9, 123.4, 121.1, 111.1, 98.9, and 21.4. HRMS-ESI [M + OH]+ calcd. for C21H16ClO2Se: 415.0004, found 414.9989.
2-(p-Tolyl)-3-(p-tolylselanyl)benzofuran (3n). White solid (85.9 mg, 91%): 1H NMR (200 MHz, CDCl3) δ 8.10 (d, J = 8.0 Hz, 2H), 7.51 (t, J = 6.0 Hz, 2H), 7.40–7.09 (m, 6H), 6.96 (d, J = 8.0 Hz, 2H), 2.39 (s, 3H), and 2.23 (s, 3H). 13C NMR (50 MHz, CDCl3) δ 157.3, 154.0, 139.4, 136.1, 132.1, 130.1, 129.5, 129.2, 127.7, 127.4, 124.9, 123.3, 121.1, 111.0, 99.4, 21.4, and 20.9. EIMS (m/z, rel. int. %) 298 (100), 178 (14), 255 (11), and 378 (28). HRMS not ionized in ESI and APCI.
3-(Butylselanyl)-2-phenylbenzofuran (3o) (Xavier et al., 2020). Yellow oil (64.6 mg, 78% yield): 1H NMR (400 MHz, CDCl3) δ 8.39–8.29 (m, 2H), 7.71 (m, 1H), 7.58–7.27 (m, 6H), 2.82 (t, J = 7.5 Hz, 2H), 1.66–1.53 (m, 2H), 1.42–1.30 (m, 2H), and 0.82 (t, J = 7.5 Hz, 3H). 13C NMR (100 MHz, CDCl3) δ 155.9, 153.9, 132.7, 130.7, 128.9, 128.4, 127.7, 124.9, 123.1, 121.0, 111.1, 100.4, 32.4, 28.3, 22.7, and 13.5.
2-Phenyl-3-(thiophen-2-ylselanyl)benzofuran (3p). Yellow solid (27.6 mg, 31% yield): 1H NMR (400 MHz, CDCl3) δ 8.39–8.19 (m, 2H), 7.77–7.66 (m, 1H), 7.60–7.20 (m, 8H), and 6.98–6.85 (m, 1H). 13C NMR (100 MHz, CDCl3) δ 156.2, 153.9, 133.6, 131.7, 130.2, 129.9, 129.3, 128.5, 128.0, 127.8, 125.2, 123.4, 121.0, 111.2, and 102.1. EIMS (m/z, rel. int. %) 276 (100), 44 (10), 165 (22), and 356 (21). HRMS not ionized in ESI and APCI.
3-(Phenylselanyl)-2-(thiophen-3-yl)benzofuran (3q). Pale yellow solid (80.8 mg, 91% yield): 1H NMR (200 MHz, CDCl3) δ 8.23–8.06 (m, 1H), 7.95 (d, J = 5.0 Hz, 1H), 7.50 (d, J = 8.0 Hz, 2H), and 7.41–6.92 (m, 9H). 13C NMR (50 MHz, CDCl3) δ 154.7, 154.0, 131.9, and 131.4 (2xC), 129.4, 129.3, 126.8, 126.4, 126.0, 125.4, 125.2, 123.6, 121.1, 111.2, and 98.9. HRMS-ESI [M + OH]+ calcd. for C18H13O2SSe: 372.9801, found 372.9798.
2,3-Bis(phenylselanyl)benzofuran (4a, 4a’) (Perin et al., 2019). Yellow solid (77.2 mg, 72% yield): 1H NMR (400 MHz, CDCl3) δ 7.58–7.52 (m, 2H), 7.49 (d, J = 8.0 Hz, 1H), 7.44 (dd, J = 8.0, 1.4 Hz, 1H), and 7.37–7.16 (m, 10H). 13C NMR (100 MHz, CDCl3) δ 157.3, 150.8, 132.8, 130.7, 130.5, 129.4, 129.2, 128.9, 128.9, 127.9, 126.7, 125.3, 123.5, 121.0, 113.7, and 111.4.
2,3-Bis(p-tolylselanyl)benzofuran (4b). Yellow solid (77.6 mg, 68% yield): 1H NMR (400 MHz, CDCl3) δ 7.50–7.40 (m, 4H), 7.32–7.18 (m, 4H), 7.09 (d, J = 8.0 Hz, 2H), 7.00 (d, J = 8.0 Hz, 2H), 2.34 (s, 3H), and 2.29 (s, 3H). 13C NMR (100 MHz, CDCl3) δ 157.1, 138.1, 136.7, 133.3, 130.9, 130.5, 130.2, 130.0, 126.8, 125.1, 123.4, 120.9, 113.4, 111.3, 21.2, and 21.0. HRMS-APCI [M + H]+ calcd. for C22H19OSe2: 458,9766, found 458.9756.
2,3-Bis[(4-chlorophenyl)selanyl]benzofuran (4c). Yellow solid (83.1 mg, 67% yield): 1H NMR (400 MHz, CDCl3) δ 7.50 (d, J = 8.3 Hz, 1H), 7.47–7.41 (m, 2H), 7.34 (td, J = 8.3, 7.2, 1.4 Hz, 1H), 7.29–7.18 (m, 4H), and 7.17–7.10 (m, 1H). 13C NMR (100 MHz, CDCl3) δ 157.3, 134.6, 134.4, 133.1, 131.8, 131.2, 130.2, 129.7, 129.5, 128.9, 126.9, 125.8, 123.9, 120.9, 113.5, and 111.6. HRMS-APCI [M + OH]+ calcd. for C20H13Cl2O2Se2:514.8602, found 514.8602.
3-(Phenylselanyl)-2-(phenylthio)benzofuran (4d). Yellow solid (83.7 mg, 88% yield): 1H NMR (200 MHz, CDCl3) δ 7.45–7.37 (m, 2H) and 7.36–7.11 (m, 12H). 13C NMR (50 MHz, CDCl3) δ 156.4, 152.9, 133.4, 131.0, 130.5, 130.3, 129.3, 127.6, 126.90, 125.9, 123.6, 121.3, 112.8, and 111.6.
2-[(Phenylselanyl)ethynyl]phenol (1g). Brown solid (90.6 mg, 33% yield): 1H NMR (400 MHz, CDCl3) δ 7.60–7.54 (m, 1H), 7.40 (dd, J = 7.5, 1.5 Hz, 1H), 7.36–7.24 (m, 4H), 7.01–6.93 (m, 1H), 6.89 (td, J = 7.5, 1.0 Hz, 1H), and 5.91 (s, 1H). 13C NMR (100 MHz, CDCl3) δ 157.7, 132.5, 131.3, 129.9, 129.4, 128.6, 127.6, 120.5, 115.0, 109.7, 96.7, and 77.2. HRMS-ESI [M + H]+ calcd. for C14H11OSe: 274.9975, found 274.9988.
2-((Phenylthio)ethynyl)phenol (1h). Yellow solid (236.2 mg, 54% yield): 1H NMR (200 MHz, CDCl3) δ 7.58–7.13 (m, 7H), 6.92 (dd, J = 18.5, 8.0 Hz, 2H), and 5.90 (s, 1H). 13C NMR (50 MHz, CDCl3) δ 157.8, 132.9, 132.5, 131.5, 129.6, 127.0, 126.6, 120.6, 115.1, 109.4, 91.6, and 83.4. HRMS-ESI [M-H]- calcd. for C14H9OS: 225.0374, found 225.0370.
2-Phenyl-3-(phenylseleninyl)benzofuran (5a). White solid (160.7 mg, 88% yield): 1H NMR (400 MHz, CDCl3) δ 7.97–7.91 (m, 2H), 7.82 (dd, J = 7.5, 2.0 Hz, 2H), 7.59–7.42 (m, 8H), 7.28 (d, J = 14.5 Hz, 1H), and 7.14–7.06 (m, 1H). 13C NMR (100 MHz, CDCl3) δ 158.6, 154.4, 140.1, 131.3, 130.8, 129.8, 129.3, 128.8, 128.4, 126.8, 126.5, 125.7, 124.0, 121.4, 115.1, and 111.6. HRMS-ESI [M + H]+ calcd. for C20H15O2Se: 367.0237, found 367.0235.
General Procedure for Cyclic Voltammetry
Cyclic voltammograms were obtained using a BAS Epsilon potentiostat/galvanostat. All electrochemical measurements were obtained in acetonitrile solution containing 0.1 molL-1 of TBAClO4 as the supporting electrolyte under an argon atmosphere. The electrochemical cell employed had a three-electrode configuration: platinum (working), platinum wire (counter), and Ag/Ag+ (reference). The Fc+/Fc couple was used as an internal standard (E1/2 = 400 mV vs. NHE).
Data Availability Statement
The original contributions presented in the study are included in the article/Supplementary Material,, further inquiries can be directed to the corresponding authors.
Author Contributions
CD: synthesis and data collection. MRS: synthesis, data collection, and manuscript development. CN: synthesis and data collection. DD: CV and manuscript development. VI: synthesis. MS: synthesis. SM: project contribution and manuscript development. SS: project contribution and manuscript development. JN: conceptualization, synthesis, and project contribution. GM: conceptualization, synthesis, project contribution, manuscript development, and revision. JR: conceptualization, manuscript development, revision, and funding acquisition. AB: supervision, conceptualization, and reviewing. All authors discussed the results and commented on the manuscript.
Funding
The authors gratefully acknowledge CAPES (001), CNPq, UFMS, and INCT-Catálise/FAPESC for financial support. CVD would like to acknowledge CNPq for a doctoral fellowship. We also acknowledge the CAPES–PrInt UFSC Project. JR and SS are grateful to CNPq for funding (315399/2020-1, 422645/2021-4, and 403210/2021-6).
Conflict of Interest
The authors declare that the research was conducted in the absence of any commercial or financial relationships that could be construed as a potential conflict of interest.
Publisher’s Note
All claims expressed in this article are solely those of the authors and do not necessarily represent those of their affiliated organizations, or those of the publisher, the editors, and the reviewers. Any product that may be evaluated in this article, or claim that may be made by its manufacturer, is not guaranteed or endorsed by the publisher.
Acknowledgments
The authors acknowledge LQPN-Farmácia-UFSC for the HRMS analysis.
Supplementary Material
The Supplementary Material for this article can be found online at: https://www.frontiersin.org/articles/10.3389/fchem.2022.880099/full#supplementary-material
References
Ali, W., Benedetti, R., Handzlik, J., Zwergel, C., and Battistelli, C. (2021). The Innovative Potential of Selenium-Containing Agents for Fighting Cancer and Viral Infections. Drug Discov. Today 26, 256–263. doi:10.1016/j.drudis.2020.10.014
An, C., Li, C.-Y., Huang, X.-B., Gao, W.-X., Zhou, Y.-B., Liu, M.-C., et al. (2019). Selenium Radical Mediated Cascade Cyclization: Concise Synthesis of Selenated Benzofurans (Benzothiophenes). Org. Lett. 21, 6710–6714. doi:10.1021/acs.orglett.9b02315
Arora, A., Singh, S., Oswal, P., Nautiyal, D., Rao, G. K., Kumar, S., et al. (2021). Preformed Molecular Complexes of Metals with Organoselenium Ligands: Syntheses and Applications in Catalysis. Coord. Chem. Rev. 438, 213885. doi:10.1016/j.ccr.2021.213885
Asif, M. (2016). Mini Review on Important Biological Properties of Benzofuran Derivatives. J. Anal. Pharm. Res. 3, 1–3. doi:10.15406/japlr.2016.03.00050
Azeredo, J. B., Godoi, M., Martins, G. M., Silveira, C. C., and Braga, A. L. (2014). A Solvent- and Metal-free Synthesis of 3-Chacogenyl-Indoles Employing DMSO/I2 as an Eco-Friendly Catalytic Oxidation System. J. Org. Chem. 79, 4125–4130. doi:10.1021/jo5000779
Botteselle, G. V., Elias, W. C., Bettanin, L., Canto, R. F. S., SalinBarbosa, D. N. O. F. A. R., Barbosa, F. A. R., et al. (2021). Catalytic Antioxidant Activity of Bis-Aniline-Derived Diselenides as GPx Mimics. Molecules 26, 4446. doi:10.3390/molecules26154446
Boukharsa, Y., Meddah, B., Tiendrebeogo, R. Y., Ibrahimi, A., Taoufik, J., Cherrah, Y., et al. (2016). Synthesis and Antidepressant Activity of 5-(benzo[b]furan-2-Ylmethyl)-6-Methylpyridazin-3(2h)-One Derivatives. Med. Chem. Res. 25, 494–500. doi:10.1007/s00044-015-1490-x
Cembellín, S., and Batanero, B. (2021). Organic Electrosynthesis towards Sustainability: Fundamentals and Greener Methodologies. Chem. Rec. 21, 2453–2471. doi:10.1002/tcr.202100128
Chand, K., Rajeshwari, , Hiremathad, A., Hiremathad, A., Singh, M., Santos, M. A., et al. (2017). A Review on Antioxidant Potential of Bioactive Heterocycle Benzofuran: Natural and Synthetic Derivatives. Pharmacol. Rep. 69, 281–295. doi:10.1016/j.pharep.2016.11.007
Chen, Z., Lai, H., Hou, L., and Chen, T. (2020). Rational Design and Action Mechanisms of Chemically Innovative Organoselenium in Cancer Therapy. Chem. Commun. 56, 179–196. doi:10.1039/c9cc07683b
Dos Santos, D. C., Rafique, J., Saba, S., Almeida, G. M., Siminski, T., Pádua, C., et al. (2021). Apoptosis Oxidative Damage-Mediated and Antiproliferative Effect of Selenylated Imidazo[1,2-A]pyridines on Hepatocellular Carcinoma HepG2 Cells and In Vivo. J. Biochem. Mol. Toxicol. 35, e22663. doi:10.1002/jbt.22663
Du, H.-A., Zhang, X.-G., Tang, R.-Y., and Li, J.-H. (2009). PdCl2-Promoted Electrophilic Annulation of 2-Alkynylphenol Derivatives with Disulfides or Diselenides in the Presence of Iodine. J. Org. Chem. 74, 7844–7848. doi:10.1021/jo9016309
Enache, T. A., and Oliveira-Brett, A. M. (2011). Phenol and Para-Substituted Phenols Electrochemical Oxidation Pathways. J. Electroanalytical Chem. 655, 9–16. doi:10.1016/j.jelechem.2011.02.022
Franco, M. S., Saba, S., Rafique, J., and Braga, A. L. (2021). KIO 4 ‐mediated Selective Hydroxymethylation/Methylenation of Imidazo‐Heteroarenes: A Greener Approach. Angew. Chem. Int. Ed. 60, 18454–18460. doi:10.1002/anie.202104503
Frizon, T. E. A., Cararo, J. H., Saba, S., Dal-Pont, G. C., Michels, M., Braga, H. C., et al. (2020). Synthesis of Novel Selenocyanates and Evaluation of Their Effect in Cultured Mouse Neurons Submitted to Oxidative Stress. Oxid. Med. Cel. Longev. 2020, 5417024. doi:10.1155/2020/5417024
Galant, L. S., Rafique, J., Braga, A. L., Braga, F. C., Saba, S., Radi, R., et al. (2021). The Thiol-Modifier Effects of Organoselenium Compounds and Their Cytoprotective Actions in Neuronal Cells. Neurochem. Res. 46, 120–130. doi:10.1007/s11064-020-03026-x
Gao, W., lI, B., Zong, L., Yu, L., Li, X., Li, Q., et al. (2021). Electrochemical Tandem Cyclization of Unsaturated Oximes with Diselenides: A General Approach to Seleno Isoxazolines Derivatives with Quaternary Carbon Center. Euro. J. Org. Chem. 2021, 72431–72435. doi:10.1002/ejoc.202100294
Gay, R. M., Manarin, F., Schneider, C. C., Barancelli, D. A., Costa, M. D., and Zeni, G. (2010). FeCl3-Diorganyl Dichalcogenides Promoted Cyclization of 2-Alkynylanisoles to 3-Chalcogen Benzo[b]furans. J. Org. Chem. 75, 5701–5706. doi:10.1021/jo101126q
Godoi, M., Botteselle, G. V., Rafique, J., Rocha, M. S. T., Pena, J. M., and Braga, A. L. (2013). Solvent-Free Fmoc Protection of Amines under Microwave Irradiation. Asian J. Org. Chem. 2, 746–749. doi:10.1002/ajoc.201300092
Guan, Z., Wang, Y., Wang, H., Huang, Y., Wang, S., Tang, H., et al. (2019). Electrochemical Oxidative Cyclization of Olefinic Carbonyls with Diselenides. Green. Chem. 21, 4976–4980. doi:10.1039/c9gc02665g
Han, J.-S., Shao, Y.-L., Zhang, X.-H., and Zhong, P. (2013). A Green and Efficient Approach for the Synthesis of 3-Chalcogen Benzo[b]Furans via I2-Mediated Cascade Annulation Reaction of 2-Alkynylanisoles at Room Temperature in Water. Phosphorus, Sulfur, Silicon Relat. Elem. 188, 1599–1610. doi:10.1080/10426507.2013.770738
He, X., Nie, Y., Zhong, M., Li, S., Li, X., Guo, Y., et al. (2021). New Organoselenides (NSAIDs-Se Derivatives) as Potential Anticancer Agents: Synthesis, Biological Evaluation and In Silico Calculations. Eur. J. Med. Chem. 218, 113384. doi:10.1016/j.ejmech.2021.113384
Heravi, M. M., Zadsirjan, V., Hamidi, H., and Tabar Amiri, P. H. (2017). Total Synthesis of Natural Products Containing Benzofuran Rings. RSC Adv. 7, 4470–4521. doi:10.1039/c7ra03551a
Hiremathad, A., Chand, K., and Keri, R. S. (2018). Development of Coumarin-Benzofuran Hybrids as Versatile Multitargeted Compounds for the Treatment of Alzheimer's Disease. Chem. Biol. Drug Des. 92, 1497–1503. doi:10.1111/cbdd.13316
Huang, C., Hu, J., Chen, G., Wu, M., Cao, H., and Liu, X. (2022). Electrochemical Oxidative Cyclization of Alkenes, Boronic Acids, and Dichalcogenides to Access Chalcogenated Boronic Esters and 1,3-diols. Org. Chem. Front. 9, 12–18. doi:10.1039/d1qo01175h
Kumawat, A., Raheem, S., Ali, F., Dar, T. A., Chakrabarty, S., and Rizvi, M. A. (2021). Organoselenium Compounds as Acetylcholinesterase Inhibitors: Evidence and Mechanism of Mixed Inhibition. J. Phys. Chem. B 125, 1531–1541. doi:10.1021/acs.jpcb.0c08111
Kunai, A., Harada, J., Izumi, J., Tachihara, H., and Sasaki, K. (1983). Anodic Oxidation of Diphenyldiselenide in Acetonitrile. Electrochimica Acta 28, 1361–1366. doi:10.1016/0013-4686(83)85189-5
Lapcinska, S., and Arsenyan, P. (2020). Selenocystine Peptides Performance in 5-Endo-Dig Reactions. Eur. J. Org. Chem. 2020, 784–795. doi:10.1002/ejoc.201901548
Lazzaris, M. J., Martins, G. M., Xavier, F. R., Braga, A. L., and Mendes, S. R. (2021). Versatile Electrochemical Oxidative C(sp 2 )−H Bond Selenylation of Resveratrol. Eur. J. Org. Chem. 2021, 4411–4416. doi:10.1002/ejoc.202100568
Li, Q., Zhang, Y., Chen, Z., Pan, X., Zhang, Z., Zhu, J., et al. (2020). Organoselenium Chemistry-Based Polymer Synthesis. Org. Chem. Front. 7, 2815–2841. doi:10.1039/d0qo00640h
Liang, Z., Xu, H., Tian, Y., Guo, M., Su, X., and Guo, C. (2016). Design, Synthesis and Antifungal Activity of Novel Benzofuran-Triazole Hybrids. Molecules 21, 732. doi:10.3390/molecules21060732
Manarin, F., Roehrs, J. A., Gay, R. M., Brandão, R., Menezes, P. H., Nogueira, C. W., et al. (2009). Electrophilic Cyclization of 2-Chalcogenealkynylanisoles: Versatile Access to 2-Chalcogen-Benzo[b]furans. J. Org. Chem. 74, 2153–2162. doi:10.1021/jo802736e
Martín-Escolano, R., Etxebeste-Mitxeltorena, M., Martín-Escolano, J., Plano, D., Rosales, M. J., Espuelas, S., et al. (2021). Selenium Derivatives as Promising Therapy for Chagas Disease: In Vitro and In Vivo Studies. ACS Infect. Dis. 7, 1727–1738.
Martins, G. M., Meirinho, A. G., Ahmed, N., Braga, A. L., and Mendes, S. R. (2019a). Recent Advances in Electrochemical Chalcogen (S/Se)‐Functionalization of Organic Molecules. ChemElectroChem 6, 5928–5940. doi:10.1002/celc.201901525
Martins, G. M., Shirinfar, B., Hardwick, T., Murtaza, A., and Ahmed, N. (2019b). Organic Electrosynthesis: Electrochemical Alkyne Functionalization. Catal. Sci. Technol. 9, 5868–5881. doi:10.1039/c9cy01312a
Martins, G. M., Zimmer, G. C., Mendes, S. R., and Ahmed, N. (2020). Electrifying green Synthesis: Recent Advances in Electrochemical Annulation Reactions. Green. Chem. 22, 4849–4870. doi:10.1039/d0gc01324b
Matzkeit, Y. H., Tornquist, B. L., Manarin, F., Botteselle, G. V., Rafique, J., Saba, S., et al. (2018). Borophosphate Glasses: Synthesis, Characterization and Application as Catalyst for Bis(indolyl)methanes Synthesis under Greener Conditions. J. Non-Crystalline Sol. 498, 153–159. doi:10.1016/j.jnoncrysol.2018.06.020
Meirinho, A. G., Pereira, V. F., Martins, G. M., Saba, S., Rafique, J., Braga, A. L., et al. (20192019). Electrochemical Oxidative C(sp2 )-H Bond Selenylation of Activated Arenes. Eur. J. Org. Chem. 2019, 6465–6469. doi:10.1002/ejoc.201900992
Miao, Y.-H., Hu, Y.-H., Yang, J., Liu, T., Sun, J., and Wang, X.-J. (2019). Natural Source, Bioactivity and Synthesis of Benzofuran Derivatives. RSC Adv. 9, 27510–27540. doi:10.1039/c9ra04917g
Mugesh, G., and Singh, H. B. (2000). Synthetic Organoselenium Compounds as Antioxidants: Glutathione Peroxidase Activity. Chem. Soc. Rev. 29, 347–357. doi:10.1039/a908114c
Nascimento, V., Alberto, E. E., Tondo, D. W., Dambrowski, D., Detty, M. R., Nome, F., et al. (2012). GPx-Like Activity of Selenides and Selenoxides: Experimental Evidence for the Involvement of Hydroxy Perhydroxy Selenane as the Active Species. J. Am. Chem. Soc. 134, 138–141. doi:10.1021/ja209570y
Neto, J. S. S., Balaguez, R. A., Franco, M. S., de Sá Machado, V. C., Saba, S., Rafique, J., et al. (2020). Trihaloisocyanuric Acids in Ethanol: an Eco-Friendly System for the Regioselective Halogenation of Imidazo-Heteroarenes. Green. Chem. 22, 3410–3415. doi:10.1039/d0gc00137f
Nogueria, C. W., Barbos, N. V., and Rocha, J. B. T. (2021). Toxicology and Pharmacology of Synthetic Organoselenium Compounds: an Update. Arch. Toxicol. 95, 1179–1226. doi:10.1007/s00204-021-03003-5
Perin, G., Soares, L. K., Hellwig, P. S., Silva, M. S., Neto, J. S. S., Roehrs, J. A., et al. (2019). Synthesis of 2,3-Bis-Organochalcogenyl-Benzo[b]chalcogenophenes Promoted by Oxone. New J. Chem. 43, 6323–6331. doi:10.1039/c9nj00526a
Peterle, M. M., Scheide, M. R., Silva, L. T., Saba, S., Rafique, J., and Braga, A. L. (2018). Copper‐Catalyzed Three‐Component Reaction of Oxadiazoles, Elemental Se/S and Aryl Iodides: Synthesis of Chalcogenyl (Se/S)‐Oxadiazoles. ChemistrySelect 3, 13191–13196. doi:10.1002/slct.201801213
Radadiya, A., and Shah, A. (2015). Bioactive Benzofuran Derivatives: An Insight on lead Developments, Radioligands and Advances of the Last Decade. Eur. J. Med. Chem. 97, 356–376. doi:10.1016/j.ejmech.2015.01.021
Rafique, J., Canto, R. F. S., Saba, S., Barbosa, F. A. R., and Braga, A. L. (2016b). Recent Advances in the Synthesis of Biologically Relevant Selenium-Containing 5-membered Heterocycles. Curr. Org. Chem. 20, 166–188. doi:10.2174/1385272819666150810222057
Rafique, J., Farias, G., Saba, S., Zapp, E., Bellettini, I. C., Momoli Salla, C. A., et al. (2020). Selenylated-oxadiazoles as Promising DNA Intercalators: Synthesis, Electronic Structure, DNA Interaction and Cleavage. Dyes Pigm. 180, 108519. doi:10.1016/j.dyepig.2020.108519
Rafique, J., Rampon, D. S., Azeredo, J. B., Coelho, F. L., Schneider, P. H., and Braga, A. L. (2021). Light‐Mediated Seleno‐Functionalization of Organic Molecules: Recent Advances. Chem. Rec. 21, 2739–2761. doi:10.1002/tcr.202100006
Rafique, J., Saba, S., Rosário, A. R., and Braga, A. L. (2016a). Regioselective, Solvent- and Metal-free Chalcogenation of Imidazo[1,2-A ]pyridines by Employing I2/DMSO as the Catalytic Oxidation System. Chem. Eur. J. 22, 11854–11862. doi:10.1002/chem.201600800
Rodrigures, J., Saba, S., Joussef, A. C., Rafique, J., and Braga, A. L. (2018). KIO3‐catalyzed C(sp2)‐H Bond Selenylation/sulfenylation of (Hetero)arenes: Synthesis of Chalcogenated (Hetero)arenes and Their Evaluation for anti‐Alzheimer Activity. Asian J. Org. Chem. 7, 1819–1824. doi:10.1002/ajoc.201800346
Romagnoli, R., Baraldi, P. G., Salvador, M. K., Prencipe, F., Lopez-Cara, C., Schiaffino Ortega, S., et al. (2015). Design, Synthesis, In Vitro, and In Vivo Anticancer and Antiangiogenic Activity of Novel 3-Arylaminobenzofuran Derivatives Targeting the Colchicine Site on Tubulin. J. Med. Chem. 58, 3209–3222. doi:10.1021/acs.jmedchem.5b00155
Saba, S., Dos Santos, C. R., Zavarise, B. R., Naujorks, A. A. S., Franco, M. S., Schneider, A. R., et al. (2020). Photoinduced, Direct C(sp 2 )−H Bond Azo Coupling of Imidazoheteroarenes and Imidazoanilines with Aryl Diazonium Salts Catalyzed by Eosin Y. Chem. Eur. J. 26, 4461–4466. doi:10.1002/chem.201905308
Saba, S., Rafique, J., and Braga, A. L. (2016). DMSO/iodine-catalyzed Oxidative C-Se/C-S Bond Formation: a Regioselective Synthesis of Unsymmetrical Chalcogenides with Nitrogen- or Oxygen-Containing Arenes. Catal. Sci. Technol. 6, 3087–3098. doi:10.1039/c5cy01503k
Saba, S., Rafique, J., and Braga, A. L. (2015). Synthesis of Unsymmetrical Diorganyl Chalcogenides under Greener Conditions: Use of an Iodine/DMSO System, Solvent- and Metal-free Approach. Adv. Synth. Catal. 357, 1446–1452. doi:10.1002/adsc.201500024
Santos, D. C., Rafique, J., Saba, S., Grinevicius, V. M. A. S., Filho, D. W., Zamoner, A., et al. (2022). IP-Se-06, a Selenylated Imidazo[1,2-A]pyridine, Modulates Intracellular Redox State and Causes Akt/mTOR/HIF-1α and MAPKs Signaling Inhibition Promoting Antiproliferative Effect and Apoptosis in Glioblastoma Cells. Oxid. Med. Cel. Longev. 2022, 3710449. doi:10.1155/2022/3710449
Scheide, M. R., Nicoleti, C. R., Martins, G. M., and Braga, A. L. (2021). Electrohalogenation of Organic Compounds. Org. Biomol. Chem. 19, 2578–2602. doi:10.1039/d0ob02459g
Scheide, M. R., Peterle, M. M., Saba, S., Neto, J. S. S., Lenz, G. F., Cezar, R. D., et al. (2020a). Borophosphate Glass as an Active media for CuO Nanoparticle Growth: an Efficient Catalyst for Selenylation of Oxadiazoles and Application in Redox Reactions. Sci. Rep. 10, 15233. doi:10.1038/s41598-020-72129-w
Scheide, M. R., Schneider, A. R., Jardim, G. A. M., Martins, G. M., Durigon, D. C., Saba, S., et al. (2020b). Electrochemical Synthesis of Selenyl-Dihydrofurans via Anodic Selenofunctionalization of Allyl-Naphthol/phenol Derivatives and Their Anti-alzheimer Activity. Org. Biomol. Chem. 18, 4916–4921. doi:10.1039/d0ob00629g
Shao, L., Li, Y., Lu, J., and Jiang, X. (2019). Recent Progress in Selenium-Catalyzed Organic Reactions. Org. Chem. Front. 6, 2999–3041. doi:10.1039/c9qo00620f
Silveira, C. C., Mendes, S. R., Wolf, L., Martins, G. M., and von Mühlen, L. (2012). Efficient Synthesis of 3-selanyl- and 3-sulfanylindoles Employing Trichloroisocyanuric Acid and Dichalcogenides. Tetrahedron 68, 10464–10469. doi:10.1016/j.tet.2012.09.022
Spengler, G., Gajdács, M., Marć, M., Domínguez-Álvarez, E., and Sanmartín, C. (2019). Organoselenium Compounds as Novel Adjuvants of Chemotherapy Drugs-A Promising Approach to Fight Cancer Drug Resistance. Molecules 24, 336. doi:10.3390/molecules24020336
Tang, Y., Liu, Y., Zhang, Y., Zhang, D., Gong, X., and Zheng, J. (2021). Repurposing a Cardiovascular Disease Drug of Cloridarol as hIAPP Inhibitor. ACS Chem. Neurosci. 12, 1419–1427. doi:10.1021/acschemneuro.1c00091
Veloso, I. C., Delanogare, E., Machado, A. E., Braga, S. P., Rosa, G. K., De Bem, A. F., et al. (2021). A Selanylimidazopyridine (3-SePh-IP) Reverses the Prodepressant- and Anxiogenic-like Effects of a High-Fat/high-Fructose Diet in Mice. J. Pharm. Pharmacol. 73, 673–681. doi:10.1093/jpp/rgaa070
Wang, B., Wang, Z., Chen, H., Lu, C.-J., and Li, X. (2016). Synthesis and Evaluation of 8-hydroxyquinolin Derivatives Substituted with (Benzo[d][1,2]selenazol-3(2h)-One) as Effective Inhibitor of Metal-Induced Aβ Aggregation and Antioxidant. Bioorg. Med. Chem. 24, 4741–4749. doi:10.1016/j.bmc.2016.08.017
Weilbeer, C., Selent, D., Dyballa, K. M., Franke, R., Spannenberg, A., and Börner, A. (2016). Evaluation of Organoselenium Based Compounds as Co-catalysts in Rhodium-Catalyzed Hydroformylation. ChemistrySelect 1, 5421–5429. doi:10.1002/slct.201601215
Wilken, M., Ortgies, S., Breder, A., and Siewert, I. (2018). Mechanistic Studies on the Anodic Functionalization of Alkenes Catalyzed by Diselenides. ACS Catal. 8, 10901–10912. doi:10.1021/acscatal.8b01236
Xavier, M. C. D. F., Sandagorda, E. M. A., Neto, J. S. S., Schumacher, R. F., and Silva, M. S. (2020). Synthesis of 3-selanylbenzo[b]furans Promoted by SelectFluor®. RSC Adv. 10, 13975–13983. doi:10.1039/d0ra01907k
Xie, Y.-S., Kumar, D., Bodduri, V. D. V., Tarani, P. S., Zhao, B.-X., Miao, J.-Y., et al. (2014). Microwave-assisted Parallel Synthesis of Benzofuran-2-Carboxamide Derivatives Bearing Anti-inflammatory, Analgesic and Antipyretic Agents. Tetrahedron Lett. 55, 2796–2800. doi:10.1016/j.tetlet.2014.02.116
Xu, X.-L., Yang, Y.-R., Mo, X.-F., Wei, J.-L., Zhang, X.-J., and You, Q.-d. (2017). Design, Synthesis, and Evaluation of Benzofuran Derivatives as Novel Anti-pancreatic Carcinoma Agents via Interfering the Hypoxia Environment by Targeting HIF-1α Pathway. Eur. J. Med. Chem. 137, 45–62. doi:10.1016/j.ejmech.2017.05.042
Keywords: selenylbenzo[b]furans, seleno-cyclization, electrosynthesis, diselenide, selenium
Citation: Doerner CV, Scheide MR, Nicoleti CR, Durigon DC, Idiarte VD, Sousa MJA, Mendes SR, Saba S, Neto JSS, Martins GM, Rafique J and Braga AL (2022) Versatile Electrochemical Synthesis of Selenylbenzo[b]Furan Derivatives Through the Cyclization of 2-Alkynylphenols. Front. Chem. 10:880099. doi: 10.3389/fchem.2022.880099
Received: 21 February 2022; Accepted: 08 April 2022;
Published: 17 May 2022.
Edited by:
Guigen Li, Texas Tech University, United StatesReviewed by:
Rajasekar Reddy Annapureddy, Ludwig Maximilian University of Munich, GermanySheng Zhang, Anhui University, China
Copyright © 2022 Doerner, Scheide, Nicoleti, Durigon, Idiarte, Sousa, Mendes, Saba, Neto, Martins, Rafique and Braga. This is an open-access article distributed under the terms of the Creative Commons Attribution License (CC BY). The use, distribution or reproduction in other forums is permitted, provided the original author(s) and the copyright owner(s) are credited and that the original publication in this journal is cited, in accordance with accepted academic practice. No use, distribution or reproduction is permitted which does not comply with these terms.
*Correspondence: Guilherme M. Martins, Z3VpbGhlcm1lLm0ubWFydGluczg1QGdtYWlsLmNvbQ==; Jamal Rafique, amFtYWwucmFmaXF1ZUB1Zm1zLmJy, amFtYWwuY2htQGdtYWlsLmNvbQ==; Antonio L. Braga, YnJhZ2EuYW50b25pb0B1ZnNjLmJy