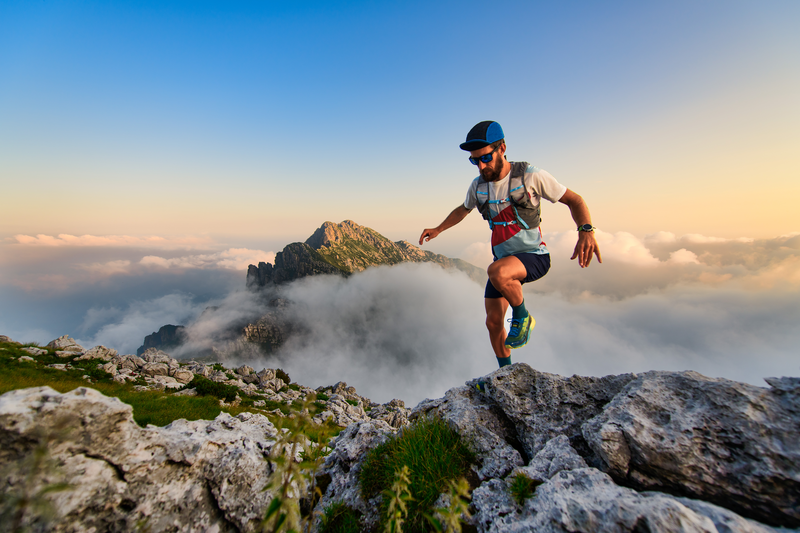
94% of researchers rate our articles as excellent or good
Learn more about the work of our research integrity team to safeguard the quality of each article we publish.
Find out more
ORIGINAL RESEARCH article
Front. Chem. , 07 April 2022
Sec. Organic Chemistry
Volume 10 - 2022 | https://doi.org/10.3389/fchem.2022.879789
This article is part of the Research Topic New Hypervalent Iodine Reagents for Oxidative Coupling - Volume II View all 8 articles
Practical approaches for chemoselective mono-bromination, di-bromination, and tetra-bromination of terminal alkynes to generate 1-bromoalkynes, 1,2-dibromoalkenes, α,α-dibromoketones, and 1,1,2,2-tetrabromoalkanes based on efficient oxidative brominations mediated by a hypervalent iodine reagent have been developed. Chemoselective bromination can be realized under mild conditions by altering the bromine source. The tetrabutylammonium bromide (TBAB)/(diacetoxyiodo)benzene (PIDA) system is specific for mono-bromination to provide 1-bromoalkynes, while the NaBr/PIDA system is selective toward di-bromination to achieve 1,2-dibromoalkenes. When a certain amount of water was added to the NaBr/PIDA system, a different di-bromination product, α,α-dibromo ketones, was generated. Tetra-bromination of terminal alkynes provides an efficient protocol for the synthesis of 1,1,2,2-tetrabromoalkanes in a system with an excess loading of NaBr/PIDA in one pot. This bromination affords good yields (up to 99%) with excellent chemoselectivity (up to 100%). These methods can be applied to the efficient chemoselective synthesis of bromide derivatives, intermediates, and related biologically active compounds.
Halogenated organic compounds play an important role in organic synthesis as synthetic intermediates or key synthetic precursors due to their feasible transformation into a variety of functional compounds for material science, bioactive compounds, or industrial chemicals (Chen et al., 2010; Adimurthym et al., 2013; Wu et al., 2017). For example, monohaloalkynes and α,β-dihaloalkenes have found a variety of application as dual functionalized molecules (Brand and Waser, 2012; Shi and Lei, 2014; Wu and Jang, 2014; Shi, 2015), and α,α-dihaloketones are important building blocks in the synthesis of heterocyclic compounds, unsaturated acids and acetylenic alcohols, and cyclopropanation (Furukawa et al., 1976; Kawabata et al., 1977; Kowalski and Fields, 1982; Zhdankin and Stang, 1993; SooáKim et al., 1995; Madabhushi et al., 2013; Sadhukhan et al., 2020). In addition, α,α-dihaloketones and tetrahalides have been reported to be potent antitumor, antibacterial, antifungal, antineoplastic, antiviral, and antioxidizing agents (Butler and Walker, 1993; Bora et al., 2000; Khazaei et al., 2010). Various synthetic methods for halogenated products have been developed in the past decades by utilizing Cl2/AsF5 or XeF + MF6− (Olah et al., 1998), hydrogen halide (De la Mare and Bolton, 1982; T Becker, 2001), molecular bromine (Chiappe et al., 2001; Ryu et al., 2002), dichlorosulfoxide and m-chloroperoxybenzoic acid (MCPBA) and BCl3 (Larock, 1999), or N-bromosuccinimide (Dalton et al., 1968) as halogen source. Among them, oxidative halogenations mediated by hypervalent iodine compounds (Braddock et al., 2004; Liu et al., 2017), which have the advantages of low toxicity, readily available reagents, mild conditions, excellent selectivity, and a comparable reactivity to alternative methods, have recently attracted considerable attention.
We recently reported our investigation of the oxidative iodination of alkynes mediated by the hypervalent iodine reagent (diacetoxyiodo)benzene (PIDA) (Liu et al., 2017). The chemoselective mono-iodination, di-iodination, and tri-iodination of alkynes were efficiently achieved in good yield with excellent chemoselectivity by altering the iodine source. The system based on hypervalent iodine, i.e., the tetrabutylammonium iodide (TBAI)/PIDA system, is specific for monoiodination, and the KI/PIDA system results in di-iodination. Combining the TBAI/PIDA and KI/PIDA systems in one pot provided the corresponding tri-iodination products efficiently.
Inspired by these observations, and as part of our ongoing interest in constructing practical methods for selective halogenation reactions, we have become keenly interested in the possibility of chemoselective bromination of alkynes mediated by hypervalent iodine reagents. In this context, we wish to report our initial study on the mono-bromination, di-bromination, or tetra-bromination of alkynes to construct 1-bromoalkynes, 1,2-dibromoalkenes, α,α-dibromoketones, and 1,1,2,2-tetrabromoalkanes, respectively, based on a highly chemoselective oxidative bromination method mediated by hypervalent iodine reagents.
To investigate the oxidative bromination of alkynes mediated by PIDA, we initially studied the influence of the bromine source, tetrabutylammonium bromide (TBAB), KBr, NaBr, by using p-tolylethyne as a mode substrate. Acetonitrile (CH3CN) or CH3CN/H2O solvent system was employed in our present bromination study, since these solvents system are optimized for high chemoselective iodination of alkynes mediated by hypervalent iodine reagents in our previous research (Liu et al., 2017). According to our previous results, tetrabutylammonium iodide (TBAI) are specific for the mono-iodination product. Similarly, 1-bromoalkyne 2a (the mono-bromination products of alkyne) was observed as the major product in 76% yield when TBAB was used as the bromine source, under the reaction conditions specified in Table 1, the molar ratio of 2a to 3a to 4a to 5a to 6a is 97:1.5:1.5:0:0 (entry 1), indicating good selectivity toward 2a. Increasing the loading of PIDA (2.0 equiv) is favorable and more efficient for mono-bromination of alkyne, 89% yield of 2a was observed and the molar ratio of 2a to 3a to 4a to 5a to 6a is 94:0:0:6:0 (entry 2). Interestingly, good selectivity toward 2a can still be observed when changing the bromine source to KBr, albeit the yield of 2a was remarkably decrease (entry 3).
Interestingly, changing the bromine source from KBr to NaBr dramatically changed the chemoselectivity to give the di-bromination product of the alkyne, 3a (E-type 1,2-dibromoalkene), as the major product in 66% yield, and 4a (Z-type 1,2-dibromoalkene) as the minor product in 6% yield; the 2a:3a:4a:5a:6a ratio is 5.1:84.6:10.3:0:0 (entry 4). Increasing the loading of PIDA or NaBr can efficiently promote the selectivity of the transformation towards 1,2-dibromoalkene, as observed from the resulting total di-bromination product yields of 90% (entry 5) and 85% (entry 6), respectively.
With these promising results in hand, we further investigated the influence of the solvent on the chemoselectivity of the bromination. In our previous study of the hypervalent iodine-reagent mediated di-iodination of alkynes to construct 1,2-diiodoalkenes, use of the KI/PIDA system in CH3CN/H2O (1:3 v/v) was crucial to achieve this chemoselectivity (Liu et al., 2017). In the present study, however, when the halogen source was changed from KI to KBr, the expected selectivity towards the di-bromination product (1,2-tribromoalkene) was not observed. Interestingly, α,α-dibromoketone 5a was obtained instead in 48% yield with good chemoselectivity; the 2a:3a:4a:5a:6a ratio was 1.6:18:3.3:77.1:0 (entry 7). Further changing the bromine source to NaBr provided 5a with higher selectivity (entry 8). Furthermore, increasing the loading of PIDA (2 equiv) favorably and strongly influenced the transformation towards 5a in 82% yield without loss of chemoselectivity (entry 9). A further increased loading of PIDA (3 equiv) afforded 5a almost exclusively, giving a 2a:3a:4a:5a:6a ratio of 0:0:0:97:3 (entry 10). According to the literatures (Madabhushi et al., 2013; Wu et al., 2017), it’s highly likely that the residual water in solvent participated in the generation of HBrO, which subsequently promoted the generation α,α-dibromoketones under our reaction conditions.
Encouraged by these favorable results, we became interested in the possibility of the direct transformation of 1a into tetra-bromination product 6a mediated by hypervalent iodine reagents. It is worth mentioning that the construction of tetrahalides in good yield and selectivity remains challenging. In our system, the tetra-bromination product (1,1,2,2-tetrabromoalkanes) may be derived from the in-situ generated 1,2-dibromoalkene through addition reaction. To realize the construction of the target molecule, we initially tried increasing the loading of both PIDA (2 equiv) and NaBr (4 equiv). Fortunately, the tetra-bromination product 6a was obtained in 54% yield; the 2a:3a:4a:5a:6a ratio was 0:30.2:15.9:0:53.9 (Table 2, entry 1). To consume the intermediate 1,2-dibromoalkene, we tried using a higher loading of both PIDA (3 equiv) and NaBr (6 equiv). We were delighted to obtain a good yield of 74% and high chemoselectivity toward 6a, with a 2a:3a:4a:5a:6a ratio of 3.8:5.9:3.5:0:86.8 (entry 2). Based on the results from entries 1 and 2, we studied the influence of loading PIDA/NaBr in two batches (in one pot). The result showed that the 1,2-dibromoalkene was fully consumed under the two-batch reaction conditions to afford 6a in good yield and excellent chemoselectivity; the 2a:3a:4a:5a:6a ratio was 0:0:0:9.2:90.8 (entry 3). Further increasing the amounts of both PIDA and NaBr resulted in this transformation almost exclusively, with 93% yield, high chemoselectivity, and a 2a:3a:4a:5a:6a ratio of 0:0:0:6.7:93.3 (entry 4).
Therefore, the optimal conditions for the synthesis of 2a, 3a, 4a, 5a or 6a were established as follows: 1) reaction of the alkyne (1.0 equiv) with TBAB (1.2 equiv) and PIDA (2.0 equiv) in CH3CN at room temperature for 1–3 h affords 2a (method A); 2) reaction of the alkyne (1.0 equiv) with NaBr (4.0 equiv) and PIDA (1.0 equiv) in CH3CN at room temperature for 1–5 h provides 3a/4a (method B); 3) reaction of the alkyne (1.0 equiv) with NaBr (3.0 equiv) and PIDA (3.0 equiv) in CH3CN/H2O (1:3, v/v) at room temperature for 10 h provides 5a (method C); 4) reaction of the alkyne (1.0 equiv) with NaBr (6.0 equiv) and PIDA (4.0 equiv) in CH3CN at room temperature for 3 h, after that, NaBr (3.0 equiv) and PIDA (1.0 equiv) were then added and stirred for 3 h provides 6a (method D); Having established practical methods for the chemoselective mono-bromination, di-bromination, and tri-bromination of p-tolylethyne mediated by hypervalent iodine reagents to furnish 1-bromoalkynes, 1,2-dibromoalkene, α,α-dibromoketone and 1.1,2,2-tetrabromoalkanes, respectively, we next examined the generality and selectivity of the bromination of terminal alkynes (Table 3).
We initially investigated the chemoselective bromination of various types of terminal aromatic alkynes. Our results showed that the mono-bromination of various terminal aromatic alkynes using method A afforded 1-bromoalkynes in good to high yields (69–99%) with excellent chemoselectivity (up to 100% in most cases). The substituents on the aromatic ring of the alkynes did not significantly influence the selectivity (entries 1, 5, 9, 13, 17, 21, 25, 29, 33, and 37). In the di-bromination of various terminal aromatic alkynes using method B, the desired 1,2-dibromoalkenes were obtained in moderate to good yields with the trans-configuration (E-type) products as the major products. Electron-donating groups were tolerated under the applied reaction conditions (entries 2, 6 and 14). However, electron-withdrawing groups resulted in some loss of both yield and selectivity (entries 18, 22, 26, 30, 34, and 38).
Interestingly, the hypervalent iodine-reagent mediated di-bromination of terminal alkynes to furnish α,α-dibromoketone 5 was noticeably affected by the substituents on the aromatic ring of the alkynes. Under the reaction conditions in method C, electron-donating groups were favorable for the synthesis of the corresponding products in good to high yield (72–97%) with very high chemoselectivity (entries 3, 7 and 15). However, halogen substituents on the aromatic ring of the alkynes slightly decreased both the yield and selectivity (entries 23, 27, and 31). The yield and the selectivity decreased dramatically when strongly electron-withdrawing groups were introduced, and the cis-configuration (Z-type) 1,2-dibromoalkenes were unexpectedly obtained as the major products (entries 19, 35, and 39).
With these results in hand, we studied the tetra-bromination of terminal aromatic alkynes utilizing the one-pot system described in Table 2. Substrates with weakly electron-donating groups smoothly furnished the corresponding tetra-bromination products (1,1,2,2-tetrabromoalkanes 6) in up to 93% yield with high chemoselectivity (entries 4, 8, and 12). In contrast, the use of alkynes containing halogen groups resulted in a significant loss of both yield and selectivity (entries 24, 28, and 32). Unfortunately, alkynes with strongly electron-donating or electron-withdrawing groups were unfavorable for the tetra-bromination. For example, only 14 and 18% yields were observed when a trifluoromethyl group (CF3) and nitro group (NO2) were introduced, respectively (entries 36 and 40). The corresponding product was not obtained when a methoxy group (MeO) was introduced, instead, the α,α-dibromoketone was unexpectedly obtained as the major product with high selectivity (entry 16).
In our previous study, terminal aliphatic alkynes were tolerated in the hypervalent iodine-reagent mediated iodination. Therefore, we were also interested in comparing the bromination of terminal aliphatic alkynes with that of terminal aromatic alkynes. Under the applied reaction conditions, an aliphatic terminal alkyne with a hydroxyl group was suitable for mono-bromination, giving 56% yield and high chemoselectivity (entry 41), and for tetra-bromination in a similar yield (entry 44). However, the di-bromination conditions failed to provide either 1,2-dibromoalkenes or α,α-dibromoketone (entries 42 and 43).
In summary, we have demonstrated a practical chemoselective approach for the mono-bromination, di-bromination, or tetra-bromination of terminal alkynes to furnish 1-bromoalkynes, 1,2-dibromoalkenes, α,α-dibromoketones, and 1.1,2,2-tetrabromoalkanes based on efficient oxidative bromination mediated by hypervalent iodine reagents. Practically, chemoselective bromination can be realized under mild conditions by suitable choice of the bromine source. The TBAB/PIDA system, which is specific toward the mono-bromination to provide 1-bromoalkynes, is favorable for both aromatic and aliphatic alkynes. The NaBr/PIDA system is selective toward di-bromination to achieve 1,2-dibromoalkenes from aromatic alkynes. When a certain amount of water was added to the NaBr/PIDA system, a different di-bromination product, α,α-dibromoketones, were successfully generated. Tetra-bromination of terminal alkynes by using the NaBr/PIDA system with an excess loading provided an efficient protocol for the synthesis of 1,1,2,2-tetrabromoalkanes in one pot. Electron-donating groups on the aromatic alkynes showed a positive effect on the chemoselectivity towards α,α-dibromoketones and 1,1,2,2-tetrabromoalkanes, while electron-withdrawing groups had a negative influence. This bromination affords good yields (up to 99%) and proceeds highly selectively (up to 100%) and can thus be applied to the efficient chemoselective synthesis of bromide derivatives, intermediates, and related biologically active compounds.
1H NMR and 13C{1H} NMR spectra were recorded on a Bruker AVANCE III 400 MHz or 500 MHz spectrometer (400 MHz or 500 MHz for 1H NMR, 100 MHz or 125 MHz for 13C NMR). Tetramethylsilane (TMS) was used as an internal standard (0 ppm) for the 1H NMR spectra, and CDCl3 was used as the internal standard (77.0 ppm) for the 13C{1H} NMR spectra. Reactions were monitored by thin-layer chromatography (TLC). Reaction products were purified by column chromatography on silica gel (300–400 mesh). Chemical reagents were purchased from common commercial suppliers and used as received.
General Procedures for the Bromination of Alkynes. Method A. PIDA (193.2 mg, 0.6 mmol) was added in portions over a period of 15 min to a mixture of the alkyne (0.3 mmol) and TBAB (133.0 mg, 0.36 mmol) in CH3CN (3 ml), and the reaction mixture was stirred at room temperature for 1–3 h. The reaction progress was monitored by TLC. Upon completion, the reaction mixture was quenched with saturated aqueous Na2S2O3, washed with brine, extracted with ethyl acetate, and dried over anhydrous Na2SO4. After filtration, the solvent was removed under reduced pressure to afford the crude product, which was purified by column chromatography using hexane or hexane/ethyl acetate and analyzed by 1H and 13C NMR spectroscopy.
Method B. PIDA (96.6 mg, 0.3 mmol) was added in portions over a period of 15 min to a mixture of the alkyne (0.3 mmol) and NaBr (123.5 mg, 1.2 mmol) in CH3CN (3 ml), and the reaction mixture was stirred at room temperature for 1–5 h. The reaction progress was monitored by TLC. Upon completion, the reaction mixture was quenched with saturated aqueous Na2S2O3, washed with brine, extracted with ethyl acetate, and dried over anhydrous Na2SO4. After filtration, the solvent was removed under reduced pressure to afford the crude product, which was purified by column chromatography using hexane or hexane/ethyl acetate and analyzed by 1H and 13C NMR spectroscopy.
Method C. PIDA (289.8 mg, 0.9 mmol) was added in portions over a period of 15 min to a mixture of the alkyne (0.3 mmol) and NaBr (92.6 mg, 0.9 mmol) in CH3CN (1 ml) and H2O (3 ml), and the reaction mixture was stirred at room temperature for 10 h. The reaction progress was monitored by TLC. Upon completion, the reaction mixture was quenched with saturated aqueous Na2S2O3, washed with brine, extracted with ethyl acetate, and dried over anhydrous Na2SO4. After filtration, the solvent was removed under reduced pressure to afford the crude product, which was purified by column chromatography using hexane or hexane/ethyl acetate and analyzed by 1H and 13C NMR spectroscopy.
Method D. PIDA (386.4 mg, 1.2 mmol) was added in portions over a period of 15 min to a mixture of the alkyne (0.3 mmol) and NaBr (185.2.0 mg, 1.8 mmol) in CH3CN (3 ml). After stirring at room temperature for 3 h, NaBr (92.6 mg, 0.9 mmol) were added. More PIDA (96.6 mg, 0.3 mmol) was added in portions over a period of 15 min, and the reaction mixture was stirred at room temperature for 3 h. The reaction progress was monitored by TLC. Upon completion, the reaction mixture was quenched with saturated aqueous Na2S2O3, washed with brine, extracted with ethyl acetate, and dried over anhydrous Na2SO4. After filtration, the solvent was removed under reduced pressure to afford the crude product, which was purified by column chromatography using hexane or hexane/ethyl acetate and analyzed by 1H and 13C NMR spectroscopy.
1-(bromoethynyl)-4-methylbenzene (2a): The crude product was purified by silica gel column chromatography with hexanes as the eluent to give a yellow oil (52.1 mg, 89%). 1H NMR (400 MHz, CDCl3): δ = 7.33 (d, J = 7.6 Hz, 2H), 7.11 (d, J = 8.0 Hz, 2H), 2.34 ppm (s, 3H); 13C{1H} NMR (100 MHz, CDCl3): δ = 138.9, 131.9, 129.2, 119.7, 80.2, 48.9, 21.6 ppm.
(E/Z)-1-(1,2-dibromovinyl)-4-methylbenzene (3a/4a): The crude product was purified by silica gel column chromatography with hexanes as the eluent to give 3a as a yellow oil (63.8 mg, 77%) and 4a as yellow oil (6.6 mg, 8%), respectively. 3a: 1H NMR (400 MHz, CDCl3): δ = 7.41 (d, J = 8.0 Hz, 2H), 7.19 (d, J = 7.6 Hz, 2H), 6.76 (s, 1H), 2.37 ppm (s, 3H); 13C{1H} NMR (100 MHz, CDCl3): δ = 139.6, 134.1, 129.1, 129.0, 121.6, 102.5, 21.5 ppm 4a: 1H NMR (400 MHz, CDCl3): δ = 7.39 (d, J = 8.4 Hz, 2H), 7.15 (d, J = 8.0 Hz, 2H), 7.00 (s, 1H), 2.36 ppm (s, 3H); 13C{1H} NMR (100 MHz, CDCl3): δ = 139.6, 135.7, 131.3, 129.3, 127.6, 108.0, 21.3 ppm.
2,2-dibromo-1-(p-tolyl)ethanone (5a): The crude product was purified by silica gel column chromatography with 50:1 hexanes/EtOAc as the eluent to give a yellow oil (85 mg, 97%). 1H NMR (400 MHz, CDCl3): δ = 7.97 (d, J = 8.0 Hz, 2H), 7.29 (d, J = 8.4 Hz, 2H), 6.74 (s, 1H), 2.43 ppm (s, 3H); 13C{1H} NMR (100 MHz, CDCl3): δ = 185.7, 145.8, 129.8, 129.7, 128.2, 40.2, 21.9 ppm.
1-methyl-4-(1,1,2,2-tetrabromoethyl)benzene (6a): The crude product was purified by silica gel column chromatography with 100:1 hexanes/EtOAc as the eluent to give a yellow oil (121.6 mg, 93%). 1H NMR (400 MHz, CDCl3): δ = 7.74 (d, J = 8.0 Hz, 2H), 7.17 (d, J = 8.0 Hz, 2H), 6.39 (s, 1H), 2.36 ppm (s, 3H); 13C{1H} NMR (100 MHz, CDCl3): δ = 140.3, 137.1, 129.1, 128.6, 73.0, 54.9, 21.2 ppm.
1-(bromoethynyl)-3-methylbenzene (2b): The crude product was purified by silica gel column chromatography with hexanes as the eluent to give a yellow oil (55.6 mg, 95%). 1H NMR (400 MHz, CDCl3): δ = 7.28–7.22 (m, 2H), 7.19 (t, J = 7.6 Hz, 1H), 7.14 (d, J = 7.6 Hz, 1H), 2.31 ppm (s, 3H); 13C{1H} NMR (100 MHz, CDCl3): δ = 138.1, 132.6, 129.6, 129.1, 128.2, 122.5, 80.2, 49.2, 21.2 ppm.
(E/Z)-1-(1,2-dibromovinyl)-3-methylbenzene (3b/4b): The crude product was purified by silica gel column chromatography with hexanes as the eluent to give 3b as a yellow oil (48 mg, 58%) and 4b as yellow oil (2.5 mg, 3%), respectively. 3b: 1H NMR (400 MHz, CDCl3): δ = 7.32–7.29 (m, 2H), 7.26 (d, J = 8.0 Hz, 1H), 7.17 (d, J = 7.2 Hz, 1H), 6.78 (s, 1H), 2.38 ppm (s, 3H); 13C{1H} NMR (100 MHz, CDCl3): δ = 138.1, 137.0, 130.2, 129.6, 128.2, 126.2, 121.6, 102.8, 77.3, 77.0, 76.7, 21.4 ppm 4b: 1H NMR (400 MHz, CDCl3): δ = 7.31–7.28 (m, 2H), 7.22 (t, J = 8.4 Hz, 1H), 7.16 (d, J = 7.2 Hz, 1H), 7.03 (s, 1H), 2.37 ppm (s, 3H); 13C{1H} NMR (100 MHz, CDCl3): δ = 138.5, 138.4, 131.3, 130.2, 128.5, 128.4, 124.9, 108.5, 21.3 ppm.
(Z)-1-(1,2-dibromovinyl)-3-methylbenzene (5b): The crude product was purified by silica gel column chromatography with 50:1 hexanes/EtOAc as the eluent to give a yellow oil (64 mg, 73%). 1H NMR (400 MHz, CDCl3): δ = 7.87–7.86 (m, 2H), 7.44 (d, J = 7.6 Hz, 1H), 7.39 (t, J = 8.0 Hz, 1H), 6.73 ppm (s, 1H); 13C{1H} NMR (100 MHz, CDCl3): δ = 186.2, 139.0, 135.3, 130.9, 130.1, 128.8, 126.8, 39.9, 21.4 ppm.
1-methyl-3-(1,1,2,2-tetrabromoethyl)benzene (6b): The crude product was purified by silica gel column chromatography with 100:1 hexanes/EtOAc as the eluent to give a white solid (83.7 mg, 64%). 1H NMR (400 MHz, CDCl3): δ = 7.66–7.64 (m, 2H), 7.26 (t, J = 7.6 Hz, 1H), 7.17 (d, J = 7.6 Hz, 1H), 6.41 (s, 1H), 2.40 ppm (s, 3H); 13C{1H} NMR (100 MHz, CDCl3): δ = 139.8, 138.2, 130.8, 129.2, 128.3, 125.7, 73.0, 54.7, 21.6 ppm.
(bromoethynyl)benzene (2c): The crude product was purified by silica gel column chromatography with hexanes as the eluent to give a brown oil (45.6 mg, 84%). 1H NMR (400 MHz, CDCl3): δ = 7.44 (d, J = 8.0 Hz, 2H), 7.34–7.27 ppm (m, 3H); 13C{1H} NMR (100 MHz, CDCl3): δ = 132.0, 128.7, 128.4, 122.7, 80.1, 49.7 ppm.
(E/Z)-(1,2-dibromovinyl)benzene (3c/4c): The crude product was purified by silica gel column chromatography with hexanes as the eluent to give 3c as a brown oil (35.4 mg, 45%) and 4c as brown oil (2.4 mg, 3%), respectively. 3c: 1H NMR (400 MHz, CDCl3): δ = 7.50 (d, J = 8.0 Hz, 2H), 7.42–7.34 (m, 3H), 6.79 ppm (s, 1H); 13C{1H} NMR (100 MHz, CDCl3): δ = 136.0, 128.4, 128.1, 127.2, 120.3, 102.0 ppm 4c: 1H NMR (400 MHz, CDCl3): δ = 7.52–7.49 (m, 2H), 7.38–7.32 (m, 3H), 7.06 ppm (s, 1H); 13C{1H} NMR (100 MHz, CDCl3): δ = 137.5, 130.1, 128.4, 127.6, 126.7, 107.8 ppm.
2,2-dibromo-1-phenylethanone (5c): The crude product was purified by silica gel column chromatography with 50:1 hexanes/EtOAc as the eluent to give a brown oil (60 mg, 72%). 1H NMR (400 MHz, CDCl3): δ = 8.08 (d, J = 8.4 Hz, 2H), 7.63 (t, J = 7.2 Hz, 1H), 7.51 (t, J = 8.0 Hz, 2H), 6.72 ppm (s, 1H). 13C{1H} NMR (100 MHz, CDCl3): δ = 184.9, 133.4, 129.9, 128.7, 127.9, 38.7 ppm.
(1,1,2,2-tetrabromoethyl)benzene (6c): The crude product was purified by silica gel column chromatography with 100:1 hexanes/EtOAc as the eluent to give a brown oil (81 mg, 64%). 1H NMR (500 MHz, CDCl3): δ = 7.89–7.87 (m, 2H), 7.40–7.38 (m, 3H), 6.41 ppm (s, 1H); 13C{1H} NMR (125 MHz, CDCl3): δ = 139.8, 130.0, 128.7, 128.4, 72.7, 54.7 ppm.
1-(bromoethynyl)-4-methoxybenzene (2d): The crude product was purified by silica gel column chromatography with 100:1 hexanes/EtOAc as the eluent to give a brown solid (43.7 mg, 69%). 1H NMR (400 MHz, CDCl3): δ = 7.37 (d, J = 8.4 Hz, 1H), 6.82 (d, J = 8.5 Hz, 1H), 3.79 ppm (s, 2H); 13C{1H} NMR (100 MHz, CDCl3): δ = 159.9), 133.5, 114.8, 114.0, 80.0, 55.3, 47.8 ppm.
(E/Z)-1-(1,2-dibromovinyl)-4-methoxybenzene (3d/4d): The crude product was purified by silica gel column chromatography with 100:1 hexanes/EtOAc as the eluent to give 3d as a brown solid (71.8 mg, 82%) and 4d as brown solid (2.6 mg, 3%), respectively. 3d: 1H NMR (400 MHz, CDCl3): δ = 7.48 (d, J = 8.8 Hz, 2H), 6.89 (d, J = 8.8 Hz, 2H), 6.73 (s, 1H), 3.82 ppm (s, 3H); 13C{1H} NMR (100 MHz, CDCl3): δ = 160.2, 130.8, 129.2, 121.5, 113.6, 102.0, 55.4 ppm 4d: 1H NMR (400 MHz, CDCl3): δ = 7.44 (d, J = 8.8 Hz, 2H), 6.94 (s, 1H), 6.86 (d, J = 8.8 Hz, 2H), 3.82 ppm (s, 3H); 13C{1H} NMR (100 MHz, CDCl3): δ = 159.5, 130.1, 129.9, 128.1, 112.9, 106.0, 54.4 ppm.
2,2-dibromo-1-(4-methoxyphenyl)ethanone (5d): The crude product was purified by silica gel column chromatography with 30:1 hexanes/EtOAc as the eluent to give a light brown solid (80.4 mg, 87%). 1H NMR (500 MHz, CDCl3): δ = 8.08 (d, J = 9.0 Hz, 2H), 6.97 (d, J = 8.0 Hz, 2H), 6.68 (s, 1H), 3.90 ppm (s, 3H);13C{1H} NMR (125 MHz, CDCl3): δ = 184.7, 164.6, 132.3, 123.4, 114.2, 55.7, 39.9 ppm.
methyl 4-(bromoethynyl)benzoate (2e): The crude product was purified by silica gel column chromatography with 100:1 hexanes/EtOAc as the eluent to give a light yellow solid (63.1 mg, 88%). 1H NMR (400 MHz, CDCl3): δ = 7.98 (d, J = 8.4 Hz, 2H), 7.50 (d, J = 8.4 Hz, 2H), 3.92 ppm (s, 3H); 13C{1H} NMR (100 MHz, CDCl3): δ = 165.4, 130.9, 129.0, 128.5, 126.3, 78.4, 52.3, 51.3 ppm.
(E/Z)-methyl 4-(1,2-dibromovinyl)benzoate (3e/4e): The crude product was purified by silica gel column chromatography with 100:1 hexanes/EtOAc as the eluent to give 3e as a brownish yellow solid (53.8 mg, 56%) and 4e as brownish yellow solid (mg, 8%), respectively. 3e: mp:73–75°C; 1H NMR (400 MHz, CDCl3): δ = 8.06 (d, J = 8.4 Hz, 2H), 7.58 (d, J = 8.4 Hz, 2H), 6.87 (s, 1H), 3.93 ppm (s, 3H); 13C{1H} NMR (100 MHz, CDCl3): δ = 165.3, 140.3, 129.8, 128.5, 128.2, 119.0, 103.4, 51.3 ppm; IR (KBr): 3,078, 3,069, 2,958, 2,924, 2,853, 1724, 1,603, 1,564, 1,438, 1,430, 1,312, 1,286, 1,182, 1,286, 1,183, 1,110, 1,019, 964, 883, 853, 822, 797, 780, 769, 717, 698, 633, 564 cm−1.4e: mp:77–79°C; 1H NMR (400 MHz, CDCl3): δ = 8.01 (d, J = 8.8 Hz, 2H), 7.57 (d, J = 8.8 Hz, 2H), 7.19 (s, 1H), 3.92 ppm (s, 3H); 13C{1H} NMR (100 MHz, CDCl3): δ = 165.2, 141.3, 129.8, 129.0, 128.8, 126.6, 109.9, 51.3 ppm; IR (KBr): 3,063, 3,039, 3,002, 2,953, 2,853, 1941, 1725, 1,606, 1,584, 1,500, 1,430, 1,407, 1,318, 1,278, 1,218, 1,186, 1,110, 1,020, 970, 895, 862, 801, 769, 745, 697, 652, 542 cm−1.
methyl 4-(2,2-dibromoacetyl)benzoate (5e): The crude product was purified by silica gel column chromatography with 30:1 hexanes/EtOAc as the eluent to give a brown solid (9.1 mg, 9%). Mp: 79–80°C; 1H NMR (400 MHz, CDCl3): δ = 8.16 (s, 4H), 6.66 (s, 1H), 3.97 ppm (s, 3H); 13C{1H} NMR (100 MHz, CDCl3): δ = 184.5, 164.8, 134.0, 133.2, 129.0, 128.7, 51.6, 38.3 ppm; IR (KBr): 2,952, 2,925, 2,854, 1721, 1,690, 1,604, 1,571, 1,528, 1,504, 1,436, 1,406, 1,277, 1,190, 1,108, 1,018, 987, 966, 869, 793, 733, 715, 684, 655, 559 cm−1.
methyl 4-(1,1,2,2-tetrabromoethyl)benzoate (6e): The crude product was purified by silica gel column chromatography with 50:1 hexanes/EtOAc as the eluent to give a brownish yellow solid (21.6 mg, 15%). Mp: 66–68°C; 1H NMR (400 MHz, CDCl3): δ = 8.05 (d, J = 8.8 Hz, 2H), 7.96 (d, J = 8.8 Hz, 2H), 6.41 (s, 1H), 3.94 (s, 3H) ppm; 13C{1H} NMR (100 MHz, CDCl3): δ = 164.9, 143.1, 130.4, 128.5, 127.8, 70.1, 52.7, 51.4 ppm; IR (KBr): 2,994, 2,955, 2,924, 2,853, 1716, 1,608, 1,432, 1,397, 1,313, 1,289, 1,261, 1,184, 1,137, 1,111, 1,018, 960, 863, 840, 766, 736, 707, 691, 671, 629, 607, 570 cm−1.
1-(bromoethynyl)-4-fluorobenzene (2f): The crude product was purified by silica gel column chromatography with hexanes as the eluent to give a yellow oil (58.5 mg, 98%). 1H NMR (400 MHz, CDCl3): δ = 7.44–7.41 (m, 2H), 7.02–6.98 (m, 2H) ppm; 13C{1H} NMR (100 MHz, CDCl3): δ = 162.7(d, J = 248.6 Hz), 133.9 (d, J = 8.4 Hz), 118.8 (d, J = 3.6 Hz), 115.78 (d, J = 22.1 Hz), 79.0, 49.5 ppm.
(E/Z)-1-(1,2-dibromovinyl)-4-fluorobenzene (3f/4f): The crude product was purified by silica gel column chromatography with hexanes as the eluent to give 3f as a yellow oil (46.2 mg, 55%) and 4f as a yellow oil (46.2 mg, 55%) and 4f as yellow oil (5 mg, 6%), respectively. 3f: 1H NMR (400 MHz, CDCl3): δ = 7.52–7.49 (m, 2H), 7.10–7.05 (m, 2H), 6.80 ppm (s, 1H); 13C{1H} NMR (100 MHz, CDCl3): δ = 162.9 (d, J = 248.9 Hz), 133.1 (d, J = 3.5 Hz), 131.3 (d, J = 8.5 Hz), 120.3, 115.5 (d, J = 21.9 Hz), 103.4 ppm 4f: 1H NMR (400 MHz, CDCl3): δ = 7.51–7.47 (m, 2H), 7.07–7.02 (m, 2H), 7.01 ppm (s, 1H); 13C{1H} NMR (100 MHz, CDCl3): δ = 163.2 (d, J = 248.8 Hz), 134.7 (d, J = 3.3 Hz), 129.9, 129.6 (d, J = 8.4 Hz), 115.6 (d, J = 21.8 Hz), 108.7 ppm.
2,2-dibromo-1-(4-fluorophenyl)ethanone (5f): The crude product was purified by silica gel column chromatography with 50:1 hexanes/EtOAc as the eluent to give a yellow oil (69.2 mg, 78%). 1H NMR (400 MHz, CDCl3): δ = 8.17–8.14 (m, 2H), 7.21–7.16 (m, 2H), 6.63 ppm (s, 1H); 13C{1H} NMR (100 MHz, CDCl3): δ = 184.6, 166.4 (d, J = 256.4 Hz), 132.7(d, J = 9.6 Hz), 127.1 (d, J = 3.1 Hz), 116.3 (d, J = 22 Hz), 39.4 ppm.
1-fluoro-4-(1,1,2,2-tetrabromoethyl)benzene (6f): The crude product was purified by silica gel column chromatography with 100:1 hexanes/EtOAc as the eluent to give a yellow oil (69.9 mg, 53%). 1H NMR (400 MHz, CDCl3): δ = 7.91–7.88 (m, 2H), 7.09–7.05 (m, 2H), 6.35 ppm (s, 1H); 13C{1H} NMR (100 MHz, CDCl3): δ = 163.1 (d, J = 250.6 Hz), 135.6 (d, J = 3.1 Hz), 131.0 (d, J = 8.4 Hz), 115.1 (d, J = 21.9 Hz), 71.3, 54.6 ppm.
1-(bromoethynyl)-4-chlorobenzene (2g): The crude product was purified by silica gel column chromatography with hexanes as the eluent to give a white solid (64 mg, 99%). 1H NMR (400 MHz, CDCl3): δ = 7.37 (d, J = 8.8 Hz, 2H), 7.28 ppm (d, J = 8.4 Hz, 2H); 13C{1H} NMR (100 MHz, CDCl3): δ = 134.8, 133.2, 128.7, 121.2, 79.0, 51.0 ppm.
(E/Z)-1-chloro-4-(1,2-dibromovinyl)benzene (3g/4g): The crude product was purified by silica gel column chromatography with hexanes as the eluent to give 3g as a white solid (39.1 mg, 44%) and 4g as white solid (3.6 mg, 4%), respectively. 3g: 1H NMR (400 MHz, CDCl3): δ = 7.45 (d, J = 8.4 Hz, 2H), 7.36 (d, J = 8.8 Hz, 2H), 6.81 ppm (s, 1H); 13C NMR (100 MHz, CDCl3): δ = 135.5, 135.4, 130.6, 128.6, 120.1, 103.8 ppm 4g: 1H NMR (400 MHz, CDCl3): δ = 7.44 (d, J = 8.4 Hz, 2H), 7.33 (d, J = 8.8 Hz, 2H), 7.06 ppm (s, 1H); 13C{1H} NMR (100 MHz, CDCl3): δ = 136.9, 135.5, 129.9, 128.9,128.8, 109.5 ppm.
2,2-dibromo-1-(4-chlorophenyl)ethanone (5g): The crude product was purified by silica gel column chromatography with 50:1 hexanes/EtOAc as the eluent to give a white solid (64.7 mg, 69%). 1H NMR (400 MHz, CDCl3): δ = 8.05 (d, J = 8.8 Hz, 2H), 7.48 (d, J = 8.8 Hz, 2H), 6.62 ppm (s, 1H); 13C{1H} NMR (100 MHz, CDCl3): δ = 184.9, 141.1, 131.2, 129.3, 129.1, 39.3 ppm.
1-chloro-4-(1,1,2,2-tetrabromoethyl)benzene (6g): The crude product was purified by silica gel column chromatography with 100:1 hexanes/EtOAc as the eluent to give a yellow oil (42.4 mg, 31%). 1H NMR (400 MHz, CDCl3): δ = 7.83 (d, J = 8.8 Hz, 2H), 7.36 (d, J = 8.8 Hz, 2H), 6.35 ppm (s, 1H); 13C{1H} NMR (100 MHz, CDCl3): δ = 138.3, 136.2, 130.2, 128.4, 71.1, 54.2 ppm.
bromo-4-(bromoethynyl)benzene (2h): The crude product was purified by silica gel column chromatography with hexanes as the eluent to give a white solid (60.8 mg, 78%). 1H NMR (400 MHz, CDCl3): δ = 7.45 (d, J = 8.8 Hz, 2H), 7.30 ppm (d, J = 8.4 Hz, 2H); 13C{1H} NMR (100 MHz, CDCl3): δ = 133.4, 131.6, 123.0, 121.7, 79.1, 51.2 ppm.
(E/Z)-1-bromo-4-(1,2-dibromovinyl)benzene (3h/4h): The crude product was purified by silica gel column chromatography with hexanes as the eluent to give 3h as a light yellow solid (42.9 mg, 42%) and 4h as light yellow solid (4.1 mg, 4%), respectively. 3 h: 1H NMR (400 MHz, CDCl3): δ = 7.53 (d, J = 8.8 Hz, 2H), 7.38 (d, J = 8.4 Hz, 2H), 6.82 ppm (s, 1H); 13C{1H} NMR (100 MHz, CDCl3): δ = 135.9, 131.6, 130.8, 123.7, 120.1, 103.8 ppm 4h: 1H NMR (400 MHz, CDCl3): δ = 7.49 (d, J = 8.8 Hz, 2H), 7.37 (d, J = 8.4 Hz, 2H), 7.07 (s, 1H); 13C{1H} NMR (100 MHz, CDCl3): δ = 137.4, 131.8, 129.9, 129.2, 123.7, 109.5 ppm.
2,2-dibromo-1-(4-bromophenyl)ethanone (5h): The crude product was purified by silica gel column chromatography with 50:1 hexanes/EtOAc as the eluent to give a light yellow solid (57.8 mg, 54%). 1H NMR (400 MHz, CDCl3): δ = 7.97 (d, J = 8.8 Hz, 2H), 7.66 (d, J = 8.8 Hz, 2H), 6.59 ppm (s, 1H); 13C{1H} NMR (100 MHz, CDCl3): δ = 185.1, 132.3, 131.2, 129.9, 129.5 39.2 ppm.
1-bromo-4-(1,1,2,2-tetrabromoethyl)benzene (6h): The crude product was purified by silica gel column chromatography with 100:1 hexanes/EtOAc as the eluent to give a yellow oil (33 mg, 22%). 1H NMR (400 MHz, CDCl3): δ = 7.76 (d, J = 8.8 Hz, 2H), 7.51 (d, J = 9.2 Hz, 2H), 6.35 ppm (s, 1H); 13C{1H} NMR (100 MHz, CDCl3): δ = 138.8, 131.4, 130.4, 124.5, 71.2, 54.0 ppm.
1-(bromoethynyl)-4-(trifluoromethyl)benzene (2i): The crude product was purified by silica gel column chromatography with hexanes as the eluent to give a brown solid (74 mg, 99%). 1H NMR (400 MHz, CDCl3): δ = 7.59–7.53 ppm (m, 4H); 13C{1H} NMR (100 MHz, CDCl3): δ = 137.5, 132.3, 130.5 (q, J = 32.5 Hz), 125.3 (q, J = 32.5 Hz), 125.2 (q, J = 270.6 Hz), 78.8, 53.0 ppm.
(E/Z)-1-(1,2-dibromovinyl)-4-(trifluoromethyl)benzene (3i/4i): The crude product was purified by silica gel column chromatography with hexanes as the eluent to give 3i as a brown solid (53.5 mg, 54%) and 4i as brown solid (10.9 mg, 11%), respectively. 3i: 1H NMR (400 MHz, CDCl3): δ = 7.67–7.61 (m, 4H), 6.89 (s, 1H); 13C{1H} NMR (100 MHz, CDCl3): δ = 140.6, 131 (q, J = 32.7 Hz), 129.6, 126.4(q, J = 270.6 Hz), 125.4 (q, J = 3.7 Hz), 119.4, 104.7.4i: 1H NMR (400 MHz, CDCl3): δ = 7.66 (s, 4H), 7.17 ppm (s, 1H); 13C{1H} NMR (100 MHz, CDCl3): δ = 141.7, 131.3 (q, J = 32.6 Hz), 129.5, 126.4 (q, J = 270.6 Hz), 125.6 (q, J = 3.8 Hz), 119.7, 111.1 ppm.
2,2-dibromo-1-(4-(trifluoromethyl)phenyl)ethanone (5i): The crude product was purified by silica gel column chromatography with 50:1 hexanes/EtOAc as the eluent to give a brownish yellow solid (9.3 mg, 9%). 1H NMR (400 MHz, CDCl3): δ = 8.23 (d, J = 8.4 Hz, 2H), 7.78 (d, J = 8.4 Hz, 2H), 6.62 ppm (s, 1H); 13C{1H} NMR (100 MHz, CDCl3): δ = 185.0, 135.5 (q, J = 32.8 Hz), 133.7, 130.2, 125.9 (q, J = 3.7 Hz), 126.0 (q, J = 270 Hz), 39.0 ppm.
1-(1,1,2,2-tetrabromoethyl)-4-(trifluoromethyl)benzene (6i): The crude product was purified by silica gel column chromatography with 100:1 hexanes/EtOAc as the eluent to give 6i as a yellow solid (20.6 mg, 14%). Mp: 63–65°C; 1H NMR (400 MHz, CDCl3): δ = 8.03 (d, J = 8.4 Hz, 2H), 7.65 (d, J = 8.4 Hz, 2H), 6.40 ppm (s, 1H); 13C{1H} NMR (100 MHz, CDCl3): δ = 143.3, 131.9 (q, J = 32.8 Hz), 129.3, 125.3 (q, J = 3.7 Hz), 123.5 (q, J = 270.8 Hz), 70.4, 53.6 ppm. IR (KBr): 2,987, 2,920, 2,830, 1,617, 1,408, 1,328, 1,157, 1,136, 1,070, 1,015, 842, 760, 726, 701, 643, 621 cm−1.
1-(bromoethynyl)-4-nitrobenzene (2j): The crude product was purified by silica gel column chromatography with 50:1 hexanes/EtOAc as the eluent to give a brown solid (57.6 mg, 85%). 1H NMR (400 MHz, CDCl3): δ = 8.19 (d, J = 8.8 Hz, 2H), 7.59 ppm (d, J = 8.8 Hz, 2H); 13C{1H} NMR (100 MHz, CDCl3): δ = 146.4, 131.8, 128.5, 122.6, 77.4, 55.3 ppm.
(E/Z)-1-(1,2-dibromovinyl)-4-nitrobenzene (3j/4j): The crude product was purified by silica gel column chromatography with 50:1 hexanes/EtOAc as the eluent to give 3j as a brown solid (35.9 mg, 39%) and 4j as brown solid (9.2 mg, 10%), respectively. 3j: 1H NMR (400 MHz, CDCl3): δ = 8.26 (d, J = 8.8 Hz, 2H), 7.69 (d, J = 8.8 Hz, 2H), 6.95 ppm (s, 1H); 13C{1H} NMR (100 MHz, CDCl3): δ = 146.9, 142.3, 129.3, 122.6, 117.4, 104.8 ppm 4j: 1H NMR (400 MHz, CDCl3): δ = 8.22 (d, J = 8.8 Hz, 2H), 7.69 (d, J = 9.2 Hz, 2H), 7.29 ppm (s, 1H); 13C{1H} NMR (100 MHz, CDCl3): δ = 148.1, 144.1, 128.7, 128.5, 123.9, 112.8 ppm.
2,2-dibromo-1-(4-nitrophenyl)ethanone (5j): The crude product was purified by silica gel column chromatography with 20:1 hexanes/EtOAc as the eluent to give 5j as a brown oil (2 mg, 2%). 1H NMR (400 MHz, CDCl3): δ = 8.25 (d, J = 8.8 Hz, 2H), 7.75 (d, J = 8.8 Hz, 2H), 6.67 ppm (s, 1H); 13C{1H} NMR (100 MHz, CDCl3): δ = 201.7, 145.4, 128.3, 126.7, 123.0, 53.6 ppm.
1-nitro-4-(1,1,2,2-tetrabromoethyl)benzene (6j): The crude product was purified by silica gel column chromatography with 30:1 hexanes/EtOAc as the eluent to give 6j as a brown solid (25.2 mg, 18%). Mp: 77–79°C; 1H NMR (400 MHz, CDCl3): δ = 8.25 (d, J = 9.2 Hz, 2H), 8.10 (d, J = 8.8 Hz, 2H), 6.40 ppm (s, 1H); 13C{1H} NMR (100 MHz, CDCl3): δ = 147.3, 129.1, 123.0, 122.3, 82.7, 52.0 ppm; IR (KBr): 3,103, 3,002, 2,961, 2,924, 2,850, 1,600, 1,513, 1,485, 1,400, 1,346, 1,318, 1,261, 1,166, 1,136, 1,110, 1,014, 867, 845, 815, 765, 752, 705, 676, 608, 570 cm−1.
The original contributions presented in the study are included in the article/Supplementary Material, further inquiries can be directed to the corresponding authors.
YoL, XC, and DH were responsible for designing and performing the experiments. YaL and ZX directed the project and wrote the manuscript.
This work was supported by the National Nature Science Foundation of China (No. 21977019), the Guangdong Provincial Key Laboratory of Plant Resources Biorefinery (No. 2021B1212040011), the Shenzhen Science and Technology Innovation Commission (No. JCYJ20200109110001818).
The authors declare that the research was conducted in the absence of any commercial or financial relationships that could be construed as a potential conflict of interest.
All claims expressed in this article are solely those of the authors and do not necessarily represent those of their affiliated organizations, or those of the publisher, the editors and the reviewers. Any product that may be evaluated in this article, or claim that may be made by its manufacturer, is not guaranteed or endorsed by the publisher.
The Supplementary Material for this article can be found online at: https://www.frontiersin.org/articles/10.3389/fchem.2022.879789/full#supplementary-material
Adimurthym, S., Ranu, B. C., Ramachandraiah, G., Ganguly, B., and Ghosh, P. K. (2013). Bromide Bromate Couple of Varying Ratios for Bromination, Vicinal Functionalization and Oxidation in a Clean Manner. Curr. Org. Synth. 10, 864–884. doi:10.2174/157017941006140206102818
Bora, U., Bose, G., Chaudhuri, M. K., Dhar, S. S., Gopinath, R., Khan, A. T., et al. (2000). Regioselective Bromination of Organic Substrates by Tetrabutylammonium Bromide Promoted by V2O5−H2O2: An Environmentally Favorable Synthetic Protocol. Org. Lett. 2, 247–249. doi:10.1021/ol9902935
Braddock, D. C., Cansell, G., and Hermitage, S. A. (2004). (Diacetoxyiodo)benzene-Lithium Bromide as a Convenient Electrophilic Br+Source. Synlett 2004, 461–464. doi:10.1055/s-2004-815410
Brand, J. P., and Waser, J. (2012). Electrophilic Alkynylation: the Dark Side of Acetylene Chemistry. Chem. Soc. Rev. 41, 4165–4179. doi:10.1039/C2CS35034C
Butler, A., and Walker, J. V. (1993). Marine Haloperoxidases. Chem. Rev. 93, 1937–1944. doi:10.1021/cr00021a014
Chen, Z., Jiang, H., Li, Y., and Qi, C. (2010). Highly Efficient Two-step Synthesis of (Z)-2-halo-1-iodoalkenes from Terminal Alkynes. Chem. Commun. 46, 8049–8051. doi:10.1039/C0CC02156C
Chiappe, C., Capraro, D., Conte, V., and Pieraccini, D. (2001). Stereoselective Halogenations of Alkenes and Alkynes in Ionic Liquids. Org. Lett. 3, 1061–1063. doi:10.1021/ol015631s
Dalton, D. R., Dutta, V. P., and Jones, D. G. (1968). Bromohydrin Formation in Dimethyl Sulfoxide. J. Am. Chem. Soc. 90, 5498–5501. doi:10.1021/ja01022a030
De la Mare, P. B. D., and Bolton, R. (1982). Electrophilic Additions to Unsaturated Systems. 2nd ed. New York: Elsevier.
Furukawa, J., Matsumura, A., Matsuoka, Y., and Kiji, J. (1976). The Reaction of Organicgem-Dihalides with Zerovalent Transition Metal Complexes. An Approach to Organic Synthesis. Bcsj 49, 829–830. doi:10.1246/bcsj.49.829
Kawabata, N., Fujii, T., Naka, M., and Yamashita, S. (1977). Generation of Ketocarbenoid by α-Elimination of ω,ω-Dibromoacetophenone with Copper, and Trapping by Cycloaddition to Olefins. Bcsj 50, 1005–1006. doi:10.1246/bcsj.50.1005
Khazaei, A., Zolfigol, M. A., Kolvari, E., Koukabi, N., Soltani, H., and Bayani, L. S. (2010). Electrophilic Bromination of Alkenes, Alkynes, and Aromatic Amines with Iodic Acid/potassium Bromide under Mild Conditions. Synth. Commun. 40, 2954–2962. doi:10.1080/00397910903349992
Kim, K. S., Cho, I. H., Ahn, Y. H., and Park, J. I. (1995). Synthesis of the 1,4-Diazepan-2-One Moiety of Liposidomycins. J. Chem. Soc. Perkin Trans. 1 1 (14), 1783–1785. doi:10.1039/P19950001783
Kowalski, C. J., and Fields, K. W. (1982). Alkynolate Anions via a New Rearrangement: the Carbon Analog of the Hofmann Reaction. J. Am. Chem. Soc. 104, 321–323. doi:10.1021/ja00365a073
Larock, R. C. (1999). in Comprehensive Organic Transformation: A Guide to Functional Group Preparation (New York: Wiley VCH), 629.
Liu, Y., Huang, D., Huang, J., and Maruoka, K. (2017). Hypervalent Iodine Mediated Chemoselective Iodination of Alkynes. J. Org. Chem. 82, 11865–11871. doi:10.1021/acs.joc.7b01555
Madabhushi, S., Jillella, R., Mallu, K. K. R., Godala, K. R., and Vangipuram, V. S. (2013). A New and Efficient Method for the Synthesis of α,α-dihaloketones by Oxyhalogenation of Alkynes Using Oxone-KX (X = Cl, Br, or I). Tetrahedron Lett. 54, 3993–3996. doi:10.1016/j.tetlet.2013.05.072
Olah, G. A., Laali, K. K., Wang, Q., and Prakash, G. K. S. (1998). Onium Ions. New York: John Wiley & Sons.
Ryu, I., Matsubara, H., Yasuda, S., Nakamura, H., and Curran, D. P. (2002). Phase-vanishing Reactions that Use Fluorous media as a Phase Screen. Facile, Controlled Bromination of Alkenes by Dibromine and Dealkylation of Aromatic Ethers by boron Tribromide. J. Am. Chem. Soc. 124, 12946–12947. doi:10.1021/ja027965y
Sadhukhan, S., Santhi, J., and Baire, B. (2020). The α,α‐Dihalocarbonyl Building Blocks: An Avenue for New Reaction Development in Organic Synthesis. Chem. Eur. J. 26, 7145–7175. doi:10.1002/chem.201905475
Shi, W. (2015). Conjugated Diyne Chemistry: Synthesis, Natural Existence and Applications. Cocat 2, 2–13. doi:10.2174/2213337201666140820225700
Shi, W., and Lei, A. (2014). 1,3-Diyne Chemistry: Synthesis and Derivations. Tetrahedron Lett. 55, 2763–2772. doi:10.1016/j.tetlet.2014.03.022
T Becker, H. G. O. (2001). in Organikum: Organisch-Chemisches Grundpraktikum (Weinheim: Wiley VCH), 299.
Wu, C., Xin, X., Fu, Z.-M., Xie, L.-Y., Liu, K.-J., Wang, Z., et al. (2017). Water-controlled Selective Preparation of α-mono or α,α′-dihalo Ketones via Catalytic cascade Reaction of Unactivated Alkynes with 1,3-Dihalo-5,5-Dimethylhydantoin. Green. Chem. 19, 1983–1989. doi:10.1039/C7GC00283A
Wu, W., and Jiang, H. (2014). Haloalkynes: a Powerful and Versatile Building Block in Organic Synthesis. Acc. Chem. Res. 47, 2483–2504. doi:10.1021/ar5001499
Keywords: bromination, hypervalent iodine reagent, 1-bromoalkynes, 1,2-dibromoalkene, α,α-dibromoketone, tetrabromoalkanes, chemoselectivity, alkyne
Citation: Li Y, Chen X, Huang D, Xie Z and Liu Y (2022) Hypervalent Iodine-Mediated Chemoselective Bromination of Terminal Alkynes. Front. Chem. 10:879789. doi: 10.3389/fchem.2022.879789
Received: 20 February 2022; Accepted: 25 February 2022;
Published: 07 April 2022.
Edited by:
Jian-Wei Han, East China University of Science and Technology, ChinaReviewed by:
Yunfei Du, Tianjin University, ChinaCopyright © 2022 Li, Chen, Huang, Xie and Liu. This is an open-access article distributed under the terms of the Creative Commons Attribution License (CC BY). The use, distribution or reproduction in other forums is permitted, provided the original author(s) and the copyright owner(s) are credited and that the original publication in this journal is cited, in accordance with accepted academic practice. No use, distribution or reproduction is permitted which does not comply with these terms.
*Correspondence: Yan Liu, eWFubGl1QGdkdXQuZWR1LmNu; Zhenming Xie, cmFyZXRlYUAxMjYuY29t
Disclaimer: All claims expressed in this article are solely those of the authors and do not necessarily represent those of their affiliated organizations, or those of the publisher, the editors and the reviewers. Any product that may be evaluated in this article or claim that may be made by its manufacturer is not guaranteed or endorsed by the publisher.
Research integrity at Frontiers
Learn more about the work of our research integrity team to safeguard the quality of each article we publish.