- 1College of Chemistry and Materials, Fujian Normal University, Fuzhou, China
- 2Fujian Engineering Research Center of New Chinese Lacquer Materials, Minjiang University, Fuzhou, China
- 3Fujian Key Laboratory of Polymer Materials, Fujian Normal University, Fuzhou, China
- 4Fujian Provincial Key Laboratory of Advanced Oriented Chemical Engineering, Fujian Normal University, Fuzhou, China
In this work, urushiol-based polybenzoxazine is cured by the Lewis acid (FeCl3, AlCl3, and CuCl2) at low temperature instead of high thermal curing temperature. The effect of the Lewis acid on structures and properties of the polymers is revealed. The relating urushiol-based benzoxazine monomer (BZ) was synthesized by natural urushiol, formaldehyde, and n-octylamine. The monomer was reacted with the Lewis acid with a molar ratio of 6:1 (Nmonomer: NMetal) at 80°C to obtain films that can be cured at room temperature. The chemical structures of benzoxazine monomers were identified by Fourier-transform infrared spectroscopy (FTIR) and 1H nuclear magnetic resonance spectroscopy (1H-NMR). The interaction between the metal ion and the polymers is revealed by X-ray photoelectron spectroscopy (XPS) and attenuated total reflectance-FTIR (ATR-FTIR). The effect of the Lewis acid on the mechanical properties, wettability, and thermal stability was investigated. The results show that the benzoxazine cured by Cu2+ has a better performance than that cured by Al3+ and Fe3+.
Introduction
Polybenzoxazine resins, as a new type of phenolic resin (Takeichi et al., 2008), have attracted significant attention due to their unique and excellent properties, such as high mechanical strength, high-temperature performance, low volatile formation, near-zero volumetric change upon curing, low surface energy, low flammability, good electrical resistance, low water absorption, and high char yield. They remain stable to moisture, chemicals, and other corrosive materials. Due to their outstanding and versatile properties, polybenzoxazine resins are widely used in aerospace, automotive, electronic manufacturing, and the preparation of high-performance composites (Ishida and Froimowicz, 2017; Mohamed and Kuo 2020; Samy et al., 2020; Mohamed et al., 2021; Chen et al., 2021a; Mohamed et al., 2022).
Benzoxazine monomers are commonly synthesized by primary amine, phenol, and formaldehyde using the Mannich condensation reaction (Tavernier et al., 2020). One of the most attractive features of benzoxazine resins is their molecular structure design flexibility. Introduction of various functional groups at the amine or phenol fragment can bring new functionalities to materials such as self-healing (Arslan et al., 2018), self-cleaning (Bai et al., 2020), shape memory (Zhang et al., 2018; Hombunma et al., 2020), flame-retardant characteristics (Appavoo et al., 2020; Ding et al., 2022), photo-sensing properties (El-Mahdy et al., 2019), etc. On the other hand, bio-based benzoxazines can be prepared by bio-based phenols and amines instead of petroleum-based ones. Until now, bio-based polybenzoxazine (Salum et al., 2018; Zhan et al., 2019; Lu et al., 2020; Zhan et al., 2020; Cai et al., 2021; Machado et al., 2021; Zhang et al., 2022) resins have been successfully prepared by natural phenols (cardanol (Appavoo et al., 2020), urushiol (Chen et al., 2021b), eugenol (Zhu et al., 2019), resorcinol (Arnebold et al., 2014), guaiacol (Phalak et al., 2017), bisguaiacol F (Periyasamy et al., 2016), phloretic acid (Kirubakaran et al., 2020) (Zhang et al., 2019a), and resveratrol (Zhang et al., 2019b)) and natural amines [(Zhu et al., 2020a), stearyl amine (Zhao et al., 2022), and rosin-based amines (Liu et al., 2017) and (Alhwaige et al., 2019)].
Generally, the benzoxazine monomer can be cured by ring-opening polymerization with thermal treatment. However, the curing temperature of most benzoxazine precursors is high (i.e., over 180°C), which greatly limits the application of polybenzoxazine resins. Much efforts have been devoted to reducing the curing temperature via intramolecular interaction [modification of monomer structures by electron-donating or -withdrawing groups, designing of monomer structure to influence the intermolecular packing (rigid groups)] (Xu et al., 2015; Zhang et al., 2019c; Zhu et al., 2020b) or intermolecular interaction cationic initiators including ordinary acids, thiols or elemental sulfur, brønsted acids; catalysts including Lewis acids, amines, latent catalysts, and nanomaterials) (Wang and Ishida 1999; Zhu et al., 2020a; Chen et al., 2021a; Liu et al., 2021; Lochab et al., 2021; Yan et al., 2021). Among these approaches, the introduction of Lewis acid is the most convenient and versatile one. PCl5 (Zhang et al., 2021), PCl3, POCl3, TiCl4, and AlCl3, and transition metal salts (Sudo et al., 2010; Ran et al., 2012; Pei et al., 2021; Guorong et al., 2022) like CuCl2, AgCl, ZnCl2, NiCl2 have been introduced into polybenzozine resins (Zhang et al., 2019a; Zhang et al., 2020). They can reduce the curing temperature to some extent. However, the interaction between the metal ions and the polymers and the effect of the metal ion on the properties of the polymers are still not clear. In this work, urushiol-based polybenzoxazine was cured with the Lewis acid (FeCl3, AlCl3, and CuCl2) at a low temperature (∼80°C). The interaction between the metal ions and the polymers was revealed and the effects of the metal ions on the properties of the polymer resin were investigated.
Experimental Section
Materials
Chinese lacquer was purchased from Xi’an Institute of Lacquer, China. Urushiol was extracted from Chinese lacquer using ethanol according to the literature. Ferric chloride, copper chloride and aluminum chloride, n-octylamine, formaldehyde (37 wt% in H2O), 1,4-dioxane, chloroform, dichloromethane, xylene, methanol, and anhydrous sodium sulfate were obtained from Sinopharm Chemical Reagent Co. All chemicals were used as received without further purification.
Characterization
1H Proton nuclear magnetic resonance (1H-NMR) spectra were recorded on a Bruker AV400 NMR spectrometer using CDCl3 as the solvent and tetramethylsilane (TMS) as the internal standard at a proton frequency of 400 MHz. Fourier transforms infrared (FTIR) spectra were recorded on a United States. Nicolet Magna 5700 spectrometer at room temperature (20°C). The spectra were collected at 32 scans with a spectral resolution of 4 cm−1. Reflex spectra were obtained from the method of ATR. Differential scanning calorimetry (DSC) was performed on a METTLER DSC3 instrument under a nitrogen atmosphere at a heating rate of 10°C min−1 in the range of 30–250°C. Thermogravimetric analysis (TGA) was performed on a METTLER TGA/SD-TA851 instrument under a nitrogen atmosphere at a heating rate of 10°C min−1 in the range of 30–600°C. X-ray photoelectron spectroscopy (XPS) recorded on a VG MultiLab 2000 spectrometer to Mg Kα (1,253.6 eV) as X-ray radiation and by being able to 20 eV, using the surface contamination carbon C1s binding energy (284.8 eV) as the internal standard calibration of other elements binding energy. The peak area was obtained by integrating the spectral characteristics of the elements and the sensitivity factor to calculate the composition of each surface element in the catalyst.
Synthesis of Urushiol-Based Benzoxazine Monomer
To a 100 ml three-necked round bottom flask equipped with a thermometer, a reflux condenser, and a dropping funnel, 10 ml of 1,4-dioxane, formaldehyde (37 wt%) (0.05 mol, 4.05 g), n-octylamine (0.025 mol, 3.23 g), and 10 ml of dioxane were added and stirred at below 4°C for 40 min. After adding a solution of urushiol (0.025 mol, 7.85–8.00 g) in 10 ml 1,4-dioxane dropwise within 20 min, the solution was gradually heated to 90°C, and the brown mixture was refluxed for 5 h. The solvent was removed by distillation under reduced pressure, and the residual was dissolved in 100 ml of dichloromethane. The solution was washed many times with distilled water and dried with anhydrous magnesium sulfate for 12 h. The solvent was removed by rotary evaporation, and the brown product was dried under vacuum at room temperature for 24 h. Further purification was conducted by column chromatography and a light red liquid was obtained (yield 96%).
Synthesis of Urushiol-Based Polybenzoxazine by Metal Ionic Catalyst
A certain amount of urushiol-based benzoxazine monomer (BZ, 0.01 mol) and anhydrous xylene (10 ml) were added to a 100 ml three-necked round bottom flask equipped with a thermometer and a reflux condenser. The solution was stirred under nitrogen for 20 min. Metal chloride (MCl3 = FeCl3, AlCl3 and CuCl2) with different molar ratio to BZ (see Table 1) in anhydrous methanol (5 ml) was added dropwise. After vigorous stirring at 80°C for 3 h, PBZ-M (PBZ-Fe, PBZ-Al, and PBZ-Cu) were obtained. PBZ-M (2 ml) was cast onto the clean glass slide or the iron plate and then cured at ambient temperature for 3 h. PBZ cured without metal ions at 120°C (2 h), 140°C (2 h), 160°C (2 h), and 180°C (2 h) was also prepared for comparison. PBA and PBZ-M membranes with a thickness of ∼60 μm were obtained.
Gel Fraction
To calculate the gel fraction of the polymers, the polymers were immersed in toluene for 2 h and then dried in the oven. The gel fraction was calculated as:
Gel fraction = mt/m0×100%,
where m0 is the initial mass of the polymeric film and mt is the mass after immersion.
Results and Discussion
Synthesis of Urushiol-Based Polybenzoxazine
The synthesis of urushiol-based benzoxazine precursor is shown in Figure 1A. The novel benzoxazine monomer was prepared via the Mannich reaction of urushiol, n-octylamine, and formaldehyde (37 wt% in H2O). The reaction time was determined by thin-layer chromatography (TLC). The benzoxazine monomer was reacted with the Lewis acid (FeCl3, AlCl3, and CuCl2) to obtain PBZ-Fe, PBZ-Al, and PBZ-Cu, respectively. The benzoxazine monomer was also thermally cured (PBZ) for comparison. It is worth noting that the polybenzoxazine/MCl3 can be cured at ambient temperature. For better performance, the molar ratio of the reactants was optimized. The results are shown in Table 1. The amount of MCl3 has a direct impact on the performance of the film. When the amount of MCl3 is large, the product is precipitate which could not be coated. When the amount of MCl3 is small, the product is viscous liquid while the surface drying time is too long. The suitable molar ratio for film coating is preferable from Table 1 when the ratio of BZ and MCl3 including n (BZ): n (FeCl3) = 6:1, n (BZ): n (AlCl3) = 3:1, n (BZ): n (AlCl3) = 6:1, n (BZ): n (CuCl2) = 4:1. The molar ratio of BZ and MCl3 was kept at 6:1 in the following experiments.
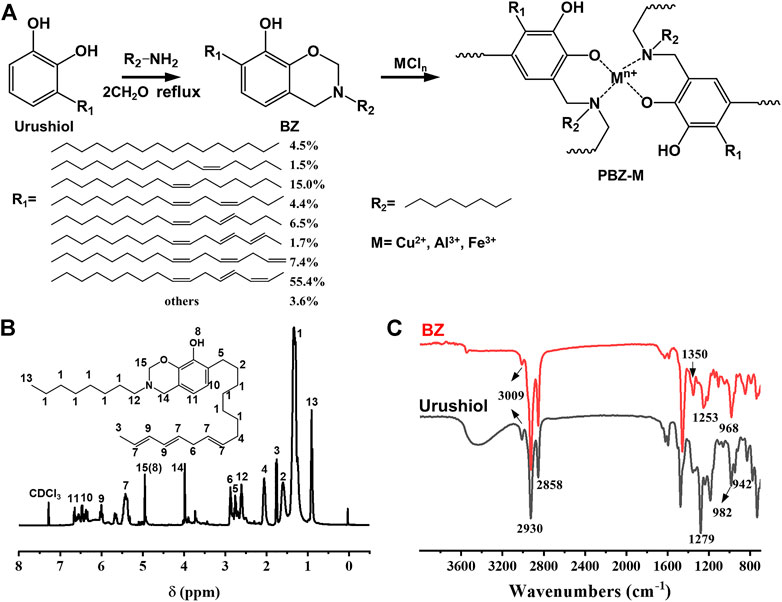
FIGURE 1. (A) Synthesis of BZ and PBZ-M. (B) 1H-NMR spectrum of BZ. (C) FTIR spectrum of urushiol and BZ.
Characterization of the Benzoxazine Monomer
The structure of the benzoxazine monomer was confirmed by 1H-NMR and FTIR. Supplementary Figure S1 shows the 1H-NMR and 13C-NMR spectrum of urushiol. Figure 1B and Supplementary Figure S2 show the 1H-NMR and 13C-NMR spectrum of BZ. The peaks in the range 5.40–6.60 ppm are assigned to the protons in–CH = CH–CH = CH–. The peaks from 1.29 to 3.00 ppm are assigned to protons of the saturated bond in the alkyl side group of the urushiol. Two characteristic resonances centered at 4.01 and 4.99 ppm are attributed to the protons in Ar–CH2–N and O–CH2–N, respectively, which is clear evidence for the formation of benzoxazine. It is worth noting that the proton resonance peak of the oxazine ring (O–CH2–N) is overlapped with the phenol hydroxyl. Figure 1C shows the FTIR spectra (Xu et al., 2012) of urushiol and BZ at room temperature. The bands at 947 and 983 cm−1 in the spectra of BZ and urushiol are assigned to the bending vibration of the conjugated double bond (-CH = CH-CH = CH-), indicating that the side chain of the urushiol was not changed during the reaction. The bands at 1,621 cm−1, 1,595 cm−1 in the spectra of urushiol and BZ are assigned to the skeleton vibration absorption peak of the benzene ring (-C=C-). The band at 3,009 cm−1 is assigned to the stretching vibration of the isolated double bond (-CH = CH-CH2-CH = CH-). The broad band at 3,200–3,600 cm−1 was due to the (–OH) vibration. The band at 1,140 cm−1 was the asymmetric stretching vibration absorption peak of (C-N-C). In comparison with urushiol, the absorption peaks at 983–947 cm−1 shift into one spike, and the absorption is enhanced. BZ exhibited a band at 965 cm−1 corresponding to the out-of-plane (-C-H) vibration of the benzene ring to where the oxazine ring is attached and a band at 1,253 cm−1 due to the asymmetric stretching of (-C-O-C-) of benzoxazine. In the fingerprint area of the spectra, the band 965 cm−1 is the characteristic benzene ring mode of benzoxazine. That broad band at 3,200–3,600 cm−1 assigned to the (–OH) vibration is clearly weakened. Both the 1H-NMR and FTIR spectra indicate the successful synthesis of the urushiol-based benzoxazine monomer.
Thermal Curing Behavior of BZ/MClx
The curing behavior of BZ/MClx was studied by DSC and the curves are shown in Figure 2 and Table 2. As shown in Figure 2A, the exothermic peak temperature (Tp) of BZ is 192.8°C. For PBZ-M, broad exothermic peaks are observed. The exothermic peak temperatures decreased from 192.8 to 134.2°C, 124.5, and 109.4°C for BZ/CuCl2, BZ/FeCl3, and BZ/AlCl3, respectively. The results indicate that MClx can effectively catalyze the polymerization of BZ. Furthermore, no isothermal DSC curves were used to study the curing kinetics of BZ and BZ/MClx at different rates (β = 5, 10, 15°C min−1). The results are shown in Figures 2C,D and Supplementary Figures S3,S4. The activation energy (Ea) is calculated using Kissinger (Kissinger 1957) and Ozawa (Takeo 1965) models. The activation energy of BZ/MClx is dramatically decreased compared with BZ. This means that BZ/MClx is easier to activate and polymerize than BZ.
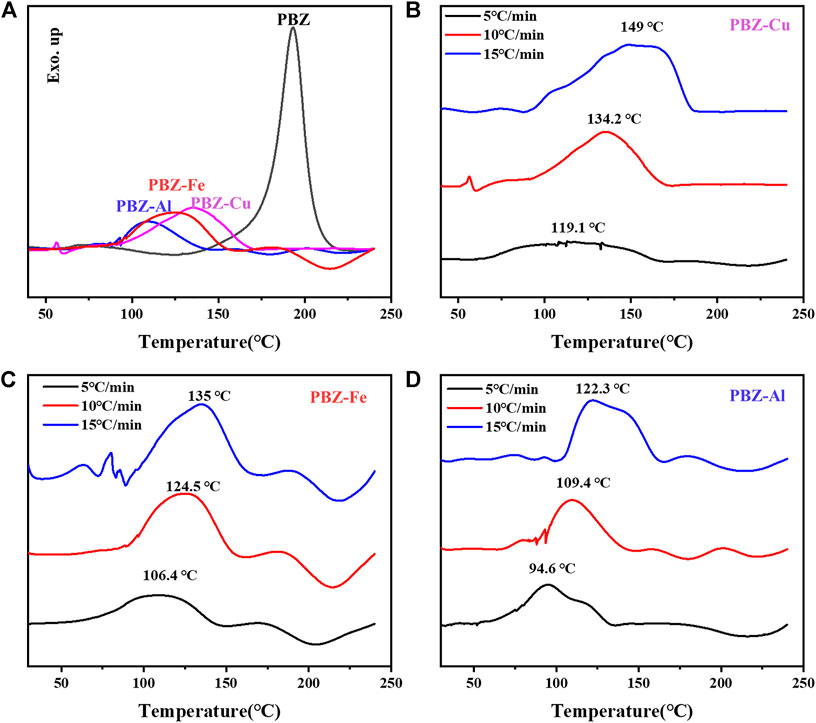
FIGURE 2. (A) Thermal curing behavior of BZ and BZ/MClx. (B–D) the curing kinetics of BZ/MClx at different rates (β = 5, 10, 15°C min−1).
Characterization of Polybenzoxazine
Metal ionic catalytic polymerization of PBZ-M was examined by NMR and ATR-FTIR. Figure 3A and Supplementary Figure S5 show the comparison of NMR spectra of PBZ-M and BZ. Compared to BZ, the peaks assigned to protons in Ar–CH2–N and O–CH2–N in PBZ-M disappeared and a new peak assigned to the Mannich bridge appeared. Figures 3B,C show the ATR-FTIR spectra of PBZ-M, PBZ, and BZ. The band at 968 cm−1, characteristic absorption peaks of benzoxazine oxazine ring, dramatically decreases. In addition, the stretching vibration absorption peak of Ar-O shifts from 1,350 cm−1 to 1,295 cm−1 because of that the structure of Ar-O-C is converted into the structure of Ar-O-H during the ring-opening reaction of the oxazine. The abovementioned changes indicate that the MClx can promote benzoxazine’s ring-opening reaction. The band at 3,009 cm−1 assigned to the stretching vibration of the isolated double bond is weakened, indicating that the isolated double bond in the alkyl side group is also involved in the polymerization reaction. The band at 3,200–3,600 cm−1 assigned to free (–OH) in the monomer is weak. After polymerization, the shoulder at 3,200–3,600 cm−1 is still weak in PBZ-Cu and PBZ-Fe. In contrast, a broad band at 3,200–3,600 cm−1 appeared in PBZ-Al. The possible explanation for this unexpected difference between PBZ-Al and PBZ-Cu and PBZ-Fe is that Cu2+ and Fe3+ are oxidizing agents. The phenolic hydroxyl groups generated during polymerization were oxidized to carbonyl groups. It can also be further demonstrated by the stronger intensity of the peak at 1,630 cm−1 that is assigned to the carbonyl groups in PBZ-M than that of PBZ. Another reason for this difference may be that the coordination situation of the metal and the hydroxyl group in PBZ-M is different. We boldly assume that the Al3+ in PBZ-Al is not mainly coordinated with hydroxyl groups while the Cu2+ and Fe3+ is.
XPS
To reveal the interaction of the metal ion and the polymers, XPS was conducted to show the element distribution in the polymer. Figure 4 shows the XPS spectra of PBZ, PBZ-Al, PBZ-Cu, and PBZ-Fe. In the XPS spectra of PBZ, there are only three peeks relating to O1s (532.1 eV), N1s (399.8 eV), and C1s (284.6 eV). In the XPS spectra of PBZ-Al (Kurdi et al., 2002), there are four peeks relating to O1s (532.1 eV), N1s (400.7 eV), C1s (284.6 eV), and Al2p (74.2 eV). In the XPS spectra of PBZ-Cu(Dong et al., 2011), there are four peeks relating to O1s (532.7 eV), N1s (403.1 eV), C1s (284.6 eV), and Cu2p (932.9 eV). In the XPS spectra of PBZ-Fe (Deng et al., 2017), there are four peeks relating to O1s (532.1 eV), N1s (401.1 eV), C1s (284.6 eV), and Fe2p (711.4 eV). The feature peak position and the change of the polymer electron binding energy of elements are summarized in Table 3. Compared to the binding energy of the metal elements in MClx, the binding energy of the metal elements in PBZ-M are all changed (△Al = −0.6 eV, △Al = −0.2 eV, △Cu = −1.3 eV). It indicates the interaction between metal ions and the main structure of benzoxazine after the polymerization. In addition, the change of the binding energy of Cu2+ is larger than Fe3+ and then Al3+, indicating a stronger interaction between Cu2+ and benzoxazine. The binding energy of N is also changed (△N-PBZ-Al = 1.3 eV, △N-PBZ-Cu = 1.5 eV, and △N-PBZ-Al = 0.9 eV). As for the element of oxygen, the binding energy of PBZ-Al and PBZ-Fe is not changed compared with that of PBZ. The binding energy of PBZ-Cu increased from 532.1 to 532.7 eV (△O-PBZ-Cu = 0.6 eV). The abovementioned analysis indicates Cu2+ is coordinated with both oxygen and nitrogen atoms while the Fe3+ and Al3+ are coordinated with only nitrogen atoms.
Mechanical Performance
The effect of metal ions on the mechanical properties of PBZ-M is shown in Table 4. Overall, PBZ-Cu has better mechanical property (hardness = 2H, impact resistance = 40 cm, flexibility = 1 mm and adhesion = grade 2) than PBZ-Fe and PBZ-Al. The impact resistance and flexibility of PBZ-Fe and PBZ-Al are similar. PBZ-Fe is harder than PBZ-Al, while the adhesion is poorer than PBZ-Al.
Surface’s Wettability
Due to the strong intramolecular hydrogen bonding between the hydroxy groups of polybenzoxazines, its surface energy is even lower than pure polytetrafluoroethylene (PTFE). To reveal the influence of the metal ion on the wettability of the polymer, we measured the water contact angle (WCAs) of PBZ, PBZ-Cu, PBZ-Fe, and PBZ-Al. As shown in Figure 5, compared with PBZ (WCAPBZ = 103° ± 2°), the introduction of Al3+ and Fe3+ reduces the WCA (WCAPBZ-Al = 92° ± 1.9° and WCAPBZ-Fe = 96° ± 1.3°). The reason for the negative effect of Al3+ and Fe3+ on the WCAs of PBZ-Al and PBZ-Fe is the chelation between Al3+ and Fe3+ and the nitrogen atom, destroying the intramolecular hydrogen bonding of the hydroxy groups. In contrast, the intramolecular hydrogen bonding of the hydroxy groups is replaced by the chelation between Cu2+ and the nitrogen atom and oxygen atom. The introduction of Cu2+ has a positive effect on the WCA (WCAPBZ-Cu = 106° ± 2°).
Thermal Stability
The effect of the metal ions catalyst on the thermal stability was studied by using thermogravimetric analysis (TGA). Figure 6 shows the TGA traces and DTG curves of PBZ, PBZ-Fe, PBZ-Al, and PBZ-Cu. The related thermogravimetric results are presented in Table 5. As we can see, the onset degradation temperature of these polymers is almost the same (∼200°C). The char yield of PBZ at 600°C is 19.20%. The char yield of PBZ-M is effectively improved compared to PBZ. The char yields of cured PBZ-Al, PBZ-Fe, and PBZ-Cu are increased from 19.20 to 27.42, 31.23, and 38.34%, respectively. Accordingly, PBZ-Cu exhibits better thermal stability than PBZ-Fe and PBZ-Al as reflected by T10%, T50%, and Tmax values. The effect of metal ions on improving the thermal stability of benzoxazine can be explained as follows: 1) the mechanical properties (hardness, listed in Table 3) and the gel fraction of PBZ-Al (HB, 84.3), PBZ-Fe (H, 85.3) and PBZ-Cu (2H, 87.4) are increased, indicating that the degree of crosslinking is increased. The higher degree of crosslinking, the more stable the PBZ-M is. 2) Cu2+ and Fe3+ are variable valence metal ions while Al3+ is not, indicating that Cu2+ and Fe3+ can oxidize the polybenzoxazine. In the oxidation of polybenzoxazine, the Mannich base transforms into an amide and/or imide-like structure, which is a thermally more stable structure. 3) According to the results of XPS analysis, PBZ-Cu has chelation between Cu2+ and nitrogen (N) and oxygen (O) atoms. PBZ-Fe and PBZ-Al have chelation between M3+ and oxygen (O) atom. The coordination bond strength in PBZ-Cu is higher than that in PBZ-Fe and PBZ-Al. It can be concluded from the abovementioned results that the thermal stability of polybenzoxazines treated with metals can be affected by the chemical structures of the polymers.
Conclusion
The urushiol-based polybenzoxazine was prepared with natural urushiol, formaldehyde, and n-octylamine and then cured with the Lewis acid (FeCl3, AlCl3, and CuCl2) at low temperature. The polymer film can be prepared at room temperature instead of high-temperature treatment. The effect of the Lewis acid on structures and properties of the polymers is revealed. The results indicate that Cu2+ is coordinated with both oxygen and nitrogen atoms while the Fe3+ and Al3+ are coordinated with only nitrogen atoms. The effect of the Lewis acid on the mechanical properties, wettability, and thermal stability was investigated. PBZ-Cu has better mechanical properties than PBZ-Fe and PBZ-Al. The introduction of Cu2+ has a positive effect on the WCA while the introduction of Fe3+ and Al3+ has a negative effect. The thermal stability is significantly improved from 19.2% (PBZ) to 38.34% (PBZ-Cu). We hope that this study will expand the application of polybenzoxazine.
Data Availability Statement
The original contributions presented in the study are included in the article/Supplementary Material, further inquiries can be directed to the corresponding authors.
Author Contributions
YL: article writing and revision; YX: design and guide experiment; WY: experimental operation and data collection; YX: experimental operation and data collection; JC: experimental data collection and analysis; CH: experimental operation and data collection.
Funding
This work was supported by the National Natural Science Foundation of China (22005053, 21978050), the Fujian Provincial Department of Science and Technology (2021J05032), the Department of Education (Fujian province) (JAT190076), and the Open Project Program of Fujian Engineering and Research Center of New Chinese lacquer Materials, Minjiang University, China (No. 323030030702).
Conflict of Interest
The authors declare that the research was conducted in the absence of any commercial or financial relationships that could be construed as a potential conflict of interest.
Publisher’s Note
All claims expressed in this article are solely those of the authors and do not necessarily represent those of their affiliated organizations, or those of the publisher, the editors, and the reviewers. Any product that may be evaluated in this article, or claim that may be made by its manufacturer, is not guaranteed or endorsed by the publisher.
Supplementary Material
The Supplementary Material for this article can be found online at: https://www.frontiersin.org/articles/10.3389/fchem.2022.879605/full#supplementary-material
References
Alhwaige, A. A., Ishida, H., and Qutubuddin, S. (2019). Poly(benzoxazine-f-chitosan) Films: The Role of Aldehyde Neighboring Groups on Chemical Interaction of Benzoxazine Precursors with Chitosan. Carbohydr. Polym. 209, 122–129. doi:10.1016/j.carbpol.2019.01.016
Appavoo, D., Amarnath, N., and Lochab, B. (2020). Cardanol and Eugenol Sourced Sustainable Non-halogen Flame Retardants for Enhanced Stability of Renewable Polybenzoxazines. Front. Chem. 8, 711. doi:10.3389/fchem.2020.00711
Arnebold, A., Schorsch, O., Stelten, J., and Hartwig, A. (2014). Resorcinol-based Benzoxazine with Low Polymerization Temperature. J. Polym. Sci. Part. A: Polym. Chem. 52 (12), 1693–1699. doi:10.1002/pola.27169
Arslan, M., Kiskan, B., and Yagci, Y. (2018). Benzoxazine-based Thermoset with Autonomous Self-Healing and Shape Recovery. Macromolecules 51 (24), 10095–10103. doi:10.1021/acs.macromol.8b02137
Bai, W., Lin, H., Chen, K., Xu, J., Chen, J., Zhang, X., et al. (2020). Eco-friendly Stable Cardanol-Based Benzoxazine Modified Superhydrophobic Cotton Fabrics for Oil-Water Separation. Sep. Purif. Tech. 253, 117545. doi:10.1016/j.seppur.2020.117545
Cai, W., Wang, Z., Shu, Z., Liu, W., Wang, J., and Qiu, J. (2021). Development of a Fully Bio-Based Hyperbranched Benzoxazine. Polym. Chem. 12 (47), 6894–6902. doi:10.1039/D1PY01451J
Chen, C., Cao, Y., Lu, X., Li, X., Yao, H., and Xin, Z. (2021a). Copolymer of Eugenol-Based and Pyrogallol-Based Benzoxazines: Low Curing Temperature and Enhanced Corrosion Resistance. Colloids Surf. A: Physicochemical Eng. Aspects 609. 125605. doi:10.1016/j.colsurfa.2020.125605
Chen, J., Jian, R., Yang, K., Bai, W., Huang, C., Lin, Y., et al. (2021b). Urushiol-based Benzoxazine Copper Polymer with Low Surface Energy, strong Substrate Adhesion and Antibacterial for marine Antifouling Application. J. Clean. Prod. 318. 128527. doi:10.1016/j.jclepro.2021.128527
Deng, Y., Dong, Y., Wang, G., Sun, K., Shi, X., Zheng, L., et al. (2017). Well-Defined ZIF-Derived Fe-N Codoped Carbon Nanoframes as Efficient Oxygen Reduction Catalysts. ACS Appl. Mater. Inter. 9 (11), 9699–9709. doi:10.1021/acsami.6b16851
Ding, H., Wang, X., Song, L., and Hu, Y. (2022). Recent Advances in Flame Retardant Bio-Based Benzoxazine Resins. J. Renew. Mater. 10 (4), 871–895. doi:10.32604/jrm.2022.018150
Dong, Y., Niu, X., Zhu, Y., Yuan, F., and Fu, H. (2011). One-pot Synthesis and Characterization of Cu-Sba-16 Mesoporous Molecular Sieves as an Excellent Catalyst for Phenol Hydroxylation. Catal. Lett. 141 (2), 242–250. doi:10.1007/s10562-010-0490-1
El-Mahdy, A. F. M., Lin, F.-W., Su, W.-H., Chen, T., and Kuo, S.-W. (2019). Photoresponsive Azobenzene Materials Based on Pyridine-Functionalized Benzoxazines as Surface Relief Gratings. ACS Appl. Polym. Mater. 2 (2), 791–804. doi:10.1021/acsapm.9b01079
Guorong, W., Yu, L., Zhiyuan, M., Jie, X., Wei, P., and Qingxin, W. (2022). Curing Mechanism and Kinetics of Benzoxazine Co-catalyzed by Transition Metal Salt and Phenolic Resin. Thermochim. Acta 710, 179182. doi:10.1016/j.tca.2022.179182
Hombunma, P., Parnklang, T., Mora, P., Jubsilp, C., and Rimdusit, S. (2020). Shape Memory Polymers from Bio-Based Benzoxazine/epoxidized Natural Oil Copolymers. Smart Mater. Struct. 29 (1), 015036. doi:10.1088/1361-665X/ab49e5
Ishida, H., and Froimowicz, P. (2017). Advanced and Emerging Polybenzoxazine Science and Technology. Amsterdam: Elsevier.
Kirubakaran, R., Sharma, P., Manisekaran, A., Bijwe, J., and Nebhani, L. (2020). Phloretic Acid: A Smart Choice to Develop Low-Temperature Polymerizable Bio-Based Benzoxazine Thermosets. J. Therm. Anal. Calorim. 142 (3), 1233–1242. doi:10.1007/s10973-019-09228-y
Kissinger, H. E. (1957). Reaction Kinetics in Differential thermal Analysis. Anal. Chem. 29 (11), 1702–1706. doi:10.1021/ac60131a045
Kurdi, J., Ardelean, H., Marcus, P., Jonnard, P., and Arefi-Khonsari, F. (2002). Adhesion Properties of Aluminium-Metallized/ammonia Plasma-Treated Polypropylene: Spectroscopic Analysis (Xps, Exes) of the Aluminium/polypropylene Interface. Appl. Surf. Sci. 189 (1), 119–128. doi:10.1016/S0169-4332(02)00017-X
Liu, X., Zhang, R., Li, T., Zhu, P., and Zhuang, Q. (2017). Novel Fully Biobased Benzoxazines from Rosin: Synthesis and Properties. ACS Sust. Chem. Eng. 5 (11), 10682–10692. doi:10.1021/acssuschemeng.7b02650
Liu, Y., Sheng, W., Yin, R., and Zhang, K. (2021). Propargylamine: An Attractive Amine Source for Designing High-Performance Benzoxazine Resins with Low Polymerization Temperatures. Polym. Chem. 12 (46), 6694–6704. doi:10.1039/D1PY01166A
Lochab, B., Monisha, M., Amarnath, N., Sharma, P., Mukherjee, S., and Ishida, H. (2021). Review on the Accelerated and Low-Temperature Polymerization of Benzoxazine Resins: Addition Polymerizable Sustainable Polymers. Polymers 13 (8), 1260. doi:10.3390/polym13081260
Lu, G., Dai, J., Liu, J., Tian, S., Xu, Y., Teng, N., et al. (2020). A New Sight into Bio-Based Polybenzoxazine: From Tunable thermal and Mechanical Properties to Excellent marine Antifouling Performance. ACS Omega 5 (7), 3763–3773. doi:10.1021/acsomega.0c00025
Machado, I., Hsieh, I., Rachita, E., Salum, M. L., Iguchi, D., Pogharian, N., et al. (2021). A Truly Bio-Based Benzoxazine Derived from Three Natural Reactants Obtained under Environmentally Friendly Conditions and its Polymer Properties. Green. Chem. 23 (11), 4051–4064. doi:10.1039/d1gc00951f
Mohamed, M. G., Chen, T.-C., and Kuo, S.-W. (2021). Solid-state Chemical Transformations to Enhance Gas Capture in Benzoxazine-Linked Conjugated Microporous Polymers. Macromolecules 54 (12), 5866–5877. doi:10.1021/acs.macromol.1c00736
Mohamed, M. G., and Kuo, S.-W. (2020). Crown Ether-Functionalized Polybenzoxazine for Metal Ion Adsorption. Macromolecules 53 (7), 2420–2429. doi:10.1021/acs.macromol.9b02519
Mohamed, M. G., Samy, M. M., Mansoure, T. H., Li, C.-J., Li, W.-C., Chen, J.-H., et al. (2022). Microporous Carbon and Carbon/metal Composite Materials Derived from Bio-Benzoxazine-Linked Precursor for Co2 Capture and Energy Storage Applications. Ijms 23 (1), 347. doi:10.3390/ijms23010347
Ozawa, T. (1965). A New Method of Analyzing Thermogravimetric Data. Bcsj 38 (11), 1881–1886. doi:10.1246/bcsj.38.1881
Pei, L., Zhao, S., Li, H., Zhang, X., Fan, X., Wang, W., et al. (2021). Preparation of Low Temperature Cure Polybenzoxazine Coating with Enhanced thermal Stability and Mechanical Properties by Combustion Synthesis Approach. Polymer 220. 123573. doi:10.1016/j.polymer.2021.123573
Periyasamy, T., Asrafali, S. P., Muthusamy, S., and Kim, S.-C. (2016). Replacing Bisphenol-A with Bisguaiacol-F to Synthesize Polybenzoxazines for a Pollution-free Environment. New J. Chem. 40 (11), 9313–9319. doi:10.1039/c6nj02242a
Phalak, G. A., Patil, D. M., and Mhaske, S. T. (2017). Synthesis and Characterization of Thermally Curable Guaiacol Based Poly(benzoxazine-Urethane) Coating for Corrosion protection on Mild Steel. Eur. Polym. J. 88, 93–108. doi:10.1016/j.eurpolymj.2016.12.030
Ran, Q.-C., Zhang, D.-X., Zhu, R.-Q., and Gu, Y. (2012). The Structural Transformation during Polymerization of Benzoxazine/fecl3 and the Effect on the thermal Stability. Polymer 53 (19), 4119–4127. doi:10.1016/j.polymer.2012.07.033
Salum, M. L., Iguchi, D., Arza, C. R., Han, L., Ishida, H., and Froimowicz, P. (2018). Making Benzoxazines Greener: Design, Synthesis, and Polymerization of a Biobased Benzoxazine Fulfilling Two Principles of green Chemistry. ACS Sust. Chem. Eng. 6 (10), 13096–13106. doi:10.1021/acssuschemeng.8b02641
Samy, M. M., Mohamed, M. G., and Kuo, S.-W. (2020). Pyrene-functionalized Tetraphenylethylene Polybenzoxazine for Dispersing Single-Walled Carbon Nanotubes and Energy Storage. Composites Sci. Tech. 199. 108360. doi:10.1016/j.compscitech.2020.108360
Sudo, A., Hirayama, S., and Endo, T. (2010). Highly Efficient Catalysts-Acetylacetonato Complexes of Transition Metals in the 4th Period for Ring-Opening Polymerization of 1,3-benzoxazine. J. Polym. Sci. A. Polym. Chem. 48 (2), 479–484. doi:10.1002/pola.23810
Takeichi, T., Kawauchi, T., and Agag, T. (2008). High Performance Polybenzoxazines as a Novel Type of Phenolic Resin. Polym. J. 40 (12), 1121–1131. doi:10.1295/polymj.PJ2008072
Tavernier, R., Granado, L., Foyer, G., David, G., and Caillol, S. (2020). Formaldehyde-free Polybenzoxazines for High Performance Thermosets. Macromolecules 53 (7), 2557–2567. doi:10.1021/acs.macromol.0c00192
Wang, Y.-X., and Ishida, H. (1999). Cationic Ring-Opening Polymerization of Benzoxazines. Polymer 40 (16), 4563–4570. doi:10.1016/S0032-3861(99)00074-9
Xu, H., Lu, Z., and Zhang, G. (2012). Synthesis and Properties of Thermosetting Resin Based on Urushiol. RSC Adv. 2 (7), 2768–2772. doi:10.1039/C2RA00829G
Xu, Y., Ran, Q., Li, C., Zhu, R., and Gu, Y. (2015). Study on the Catalytic Prepolymerization of an Acetylene-Functional Benzoxazine and the thermal Degradation of its Cured Product. RSC Adv. 5 (100), 82429–82437. doi:10.1039/c5ra13862k
Yan, H., Zhan, Z., Wang, H., Cheng, J., and Fang, Z. (2021). Synthesis, Curing, and thermal Stability of Low-Temperature-Cured Benzoxazine Resins Based on Natural Renewable Resources. ACS Appl. Polym. Mater. 3 (7), 3392–3401. doi:10.1021/acsapm.1c00361
Zhan, Z. m., Yan, H. q., Yin, P., Cheng, J., and Fang, Z. p. (2019). Synthesis and Properties of a Novel Bio‐based Benzoxazine Resin with Excellent Low‐temperature Curing Ability. Polym. Int. 69 (4), 355–362. doi:10.1002/pi.5957
Zhan, Z., Yan, H., Wang, H., Cheng, J., Ran, S., and Fang, Z. (2020). Novel Full Bio-Based Phloroglucinol Benzoxazine Resin: Synthesis, Curing Reaction and thermal Stability. Polymer 200. 122534. doi:10.1016/j.polymer.2020.122534
Zhang, C., Xue, J., Yang, X., Ke, Y., Ou, R., Wang, Y., et al. (2022). From Plant Phenols to Novel Bio-Based Polymers. Prog. Polym. Sci. 125. 101473. doi:10.1016/j.progpolymsci.2021.101473
Zhang, K., Han, M., Han, L., and Ishida, H. (2019a). Resveratrol-based Tri-functional Benzoxazines: Synthesis, Characterization, Polymerization, and thermal and Flame Retardant Properties. Eur. Polym. J. 116, 526–533. doi:10.1016/j.eurpolymj.2019.04.036
Zhang, K., Tan, X., Wang, Y., and Ishida, H. (2019b). Unique Self-Catalyzed Cationic Ring-Opening Polymerization of a High Performance Deoxybenzoin-Based 1,3-benzoxazine Monomer. Polymer 168, 8–15. doi:10.1016/j.polymer.2019.01.089
Zhang, S., Ran, Q., Fu, Q., and Gu, Y. (2019c). Controlled Polymerization of 3,4-Dihydro-2h-1,3-Benzoxazine and its Properties Tailored by lewis Acids. Reactive Funct. Polym. 139, 75–84. doi:10.1016/j.reactfunctpolym.2019.03.016
Zhang, S., Ran, Q., Fu, Q., and Gu, Y. (2018). Preparation of Transparent and Flexible Shape Memory Polybenzoxazine Film through Chemical Structure Manipulation and Hydrogen Bonding Control. Macromolecules 51 (17), 6561–6570. doi:10.1021/acs.macromol.8b01671
Zhang, S., Ran, Q., and Gu, Y. (2021). Polymerization Mechanism of 1,3-benzoxazine Catalyzed by Pcl5 and Rearrangement of Chemical Structures. Eur. Polym. J. 142. 110133. doi:10.1016/j.eurpolymj.2020.110133
Zhang, T., Bonnaud, L., Raquez, J.-M., Poorteman, M., Olivier, M., and Dubois, P. (2020). Cerium Salts: An Efficient Curing Catalyst for Benzoxazine Based Coatings. Polymers 12 (2), 415. doi:10.3390/polym12020415
Zhao, Y., Yuan, M., Wang, L., Lu, X., and Xin, Z. (2022). Preparation of Bio‐Based Polybenzoxazine/Pyrogallol/Polyhedral Oligomeric Silsesquioxane Nanocomposites: Low Dielectric Constant and Low Curing Temperature. Macro Mater. Eng 307, 2100747–2022747. doi:10.1002/mame.202100747
Zhu, H.-X., Liu, Y., Wu, Y.-X., Qiu, J.-J., and Liu, C.-M. (2020a). Unique Self-Catalyzed Bio-Benzoxazine Derived from Novel Renewable Acid-Containing Diamines Based on Levulinic Acid and Furfurylamine: Synthesis, Curing Behaviors and Properties. Reactive Funct. Polym. 155. 104716. doi:10.1016/j.reactfunctpolym.2020.104716
Zhu, Y., Li, P., Lin, R., and Su, J. (2020b). Promoting Ring-Opening Polymerization of Benzoxazine and its thermal Property through Incorporation of Pyrogallol-Based Benzoxazines. Polym. Bull. 78 (8), 4403–4417. doi:10.1007/s00289-020-03327-9
Keywords: polybenzoxazine, urushiol, metal ions catalyst, curing temperature, metal coordination
Citation: Yang W, Xie Y, Chen J, Huang C, Xu Y and Lin Y (2022) Metal Ion-Catalyzed Low-Temperature Curing of Urushiol-Based Polybenzoxazine. Front. Chem. 10:879605. doi: 10.3389/fchem.2022.879605
Received: 21 February 2022; Accepted: 01 April 2022;
Published: 28 April 2022.
Edited by:
Zhong Jin, Nanjing University, ChinaReviewed by:
Shiao Wei Kuo, National Sun Yat-sen University, TaiwanChunxia Zhao, Southwest Petroleum University, China
Yijiang Liu, Xiangtan University, China
Xiaoyun Liu, East China University of Science and Technology, China
Copyright © 2022 Yang, Xie, Chen, Huang, Xu and Lin. This is an open-access article distributed under the terms of the Creative Commons Attribution License (CC BY). The use, distribution or reproduction in other forums is permitted, provided the original author(s) and the copyright owner(s) are credited and that the original publication in this journal is cited, in accordance with accepted academic practice. No use, distribution or reproduction is permitted which does not comply with these terms.
*Correspondence: Yucai Lin, yucailin@fjnu.edu.cn; Yanlian Xu, ylxu@mju.edu.cn; Jipeng Chen, 1014894694@qq.com