- State Key Laboratory Breeding Base of Green Pesticide and Agricultural Bioengineering, Key Laboratory of Green Pesticide and Agricultural Bioengineering, Ministry of Education, Guizhou University, Guiyang, China
An unprecedented inactivation process of the indanol-derived NHC catalysts bearing N-C6F5 groups is reported. An unexpected multi-cyclic complex product is obtained from the 3-component reaction with the 1-methylcyclopropyl-carbaldehyde, the 2,2,2-trifluoroacetophenone and the NHC catalyst. The absolute structure of the inactivation product is unambiguously assigned via X-ray analysis on its single crystals. The formation of the structurally complex product is rationalized through a multi-step cascade cyclization process.
Introduction
Ever since the first report from Ukai and co-workers on the thiazolium salt promoted benzoin reaction in 1943 (Ukai et al., 1943), N-heterocyclic carbene (NHC) has been developed and used as robust organic catalyst for more than 70 years (Breslow, 1958; Sheehan and Hunneman, 1966; Enders et al., 1995; Rovis et al., 2002). Especially, NHC organocatalysis has seen fantastic development within the past two decades (Enders et al., 2007; Lupton et al., 2013; Glorius et al., 2014; Mahatthananchai and Bode, 2014; Nair et al., 2015; Rovis et al., 2015; Scheidt et al., 2018; Chi et al., 2020; Chi et al., 2021; Wang et al., 2017). Numerous catalytic activation modes have been established within this highly active research field with a huge number of reactions realized for quick and selective access to functional molecules with interesting synthetic or biological applications. Functional molecules such as aldehydes, carboxylic acids and their derivatives, imines, ketenes, and activated ketones can be efficiently activated by NHC organic catalysts via formation of (aza)-Breslow intermediates and go through addition reactions with various electrophiles or nucleophiles through electron-pair-transfer processes (Gu et al., 2017; Yao et al., 2019; Chen et al., 2020; Yao et al., 2020; Fu et al., 2021; Xue and Zheng, 2021). Due to the rich electron densities of the Breslow intermediates formed from the NHC catalysts and the aldehyde substrates, they can be selectively oxidized by external oxidants through single-electron-transfer (SET) processes and furnished radical reactions in both enantioselective and non-chiral fashion. Recently, a couple of carbon- and heteroatom-centered nucleophiles were found to be activated by chiral NHC catalysts via non-covalent interactions and smoothly participate in the enantioselective addition reactions with a diversity of electrophiles (Van Halbeek and Poppe, 1991; Enders et al., 1995; Regitz, 1996; Enders, 2003; Bastin et al., 2019). In all the NHC-catalyzed synthetic transformations we mentioned above, mechanistic studies via both experimental and computational methods have played critical roles in the development and innovations of the activation modes. Therefore, the observation and characterization of the critical intermediates and/or side reaction products to provide evidence for mechanistic studies are of great significance.
Investigations into the cross-interactions between the NHC organic catalysts and the reaction substrates are one of the effective approaches for the mechanistic studies in NHC organocatalytic reactions. Continuous endeavour has been made by organic chemists towards the isolation and characterization of the most basic Breslow intermediates since it was hypothesized by Breslow in 1958 (Breslow, 1958) (Figure 1A). For example, Berkessel and co-workers reported in 2010 the full NMR spectra analysis of the ketone form of the Breslow intermediate generated from the triazolium salt-derived NHC catalyst and the propionic aldehyde (Berkessel et al., 2010). They successfully isolated the crystals of the typical Breslow intermediate from an imidazolium-typed NHC catalyst and the benzaldehyde and obtained its X-ray analytical spectrum in 2012 (Berkessel et al., 2012). An advanced reaction intermediate between the α,β-unsaturated Breslow intermediate and the chalcone substrate could also be isolated as stable crystals and their structures were unambiguously assigned via X-ray analysis in 2015 (Berkessel et al., 2015). The single crystals of the aza-analogues of the Breslow intermediate were obtained by Rovis and co-workers from a chiral indanol-derived NHC catalyst and an iminium salt in 2012 (Rovis et al., 2012). They can also apply the aza-Breslow intermediate analogues as the NHC catalyst precursors to promote an intramolecular Stetter reaction in enantioselective fashion.
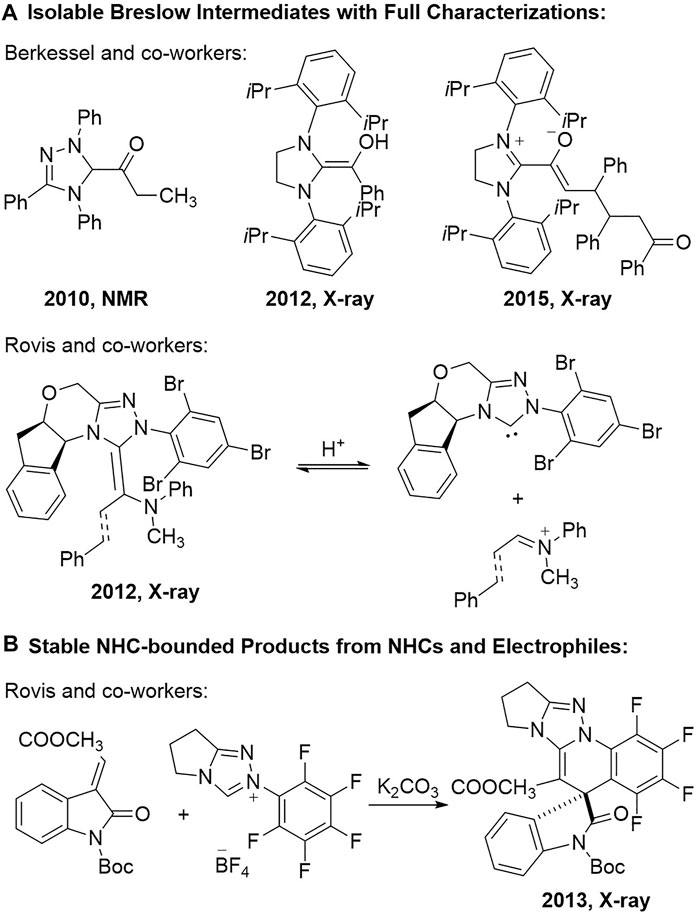
FIGURE 1. Isolation and characterization of NHC-bounded intermediates and products. (A) Isolable breslow intermediates with full characterizations. (B) Stable NHC-bounded products from NHCs and electrophiles.
The isolation and characterization of the NHC-bounded reaction products from the inactivation of the NHC catalysts in the reaction system can also provide significant information on the cross interactions between the NHC catalysts and the reaction substrates. Although the formation of the NHC-bounded side reaction products might be sometimes observed during the investigations of various NHC organocatalytic transformations, to the best of our knowledge, there has been very limited reports on the characterization of those NHC-bounded reaction products (Berkessel et al., 2013; Berden et al., 2021). A representative study was from Rovis and co-workers in 2013, when they reported a cascade cyclization reaction between the triazolium NHC catalyst bearing an N-pentafluorophenyl (N-C6F5) group and the isatin-derived α,β-unsaturated ester substrate (Rovis et al., 2013) (Figure 1B). The spirocyclic product was characterized via X-ray analysis on the product crystals.
We have previously reported an NHC-catalyzed asymmetric (4 + 2) cycloaddition reaction between 1-methylcyclopropyl-carbaldehyde 1 (Chi et al., 2011; Mu et al., 2020; Tong et al., 2021; Wang et al., 2021) and the cyclic sulfonimides 2 (Xu et al., 2013; Wang et al., 2020a; Wang et al., 2020b; Liu et al., 2021) to give a variety of multi-functionalized fused cyclic products 3 in moderate to good yields with generally excellent enantio- and diastereoselectivities (Lv et al., 2021) (Figure 2A). Indanol-derived NHC catalysts bearing electron-deficient N-substituents were found effective for this transformation. However, during the evaluations of different chiral NHC catalysts, we noticed that switching the indanol-derived NHC catalyst A (Rovis et al., 2012) to the NHC catalyst B (Enders and Balensiefer, 2004) bearing an N-C6F5 group resulted in a significant drop of the product yields (from 72% with A to 36% with B), with multiple unidentifiable by-products formed in low yields.
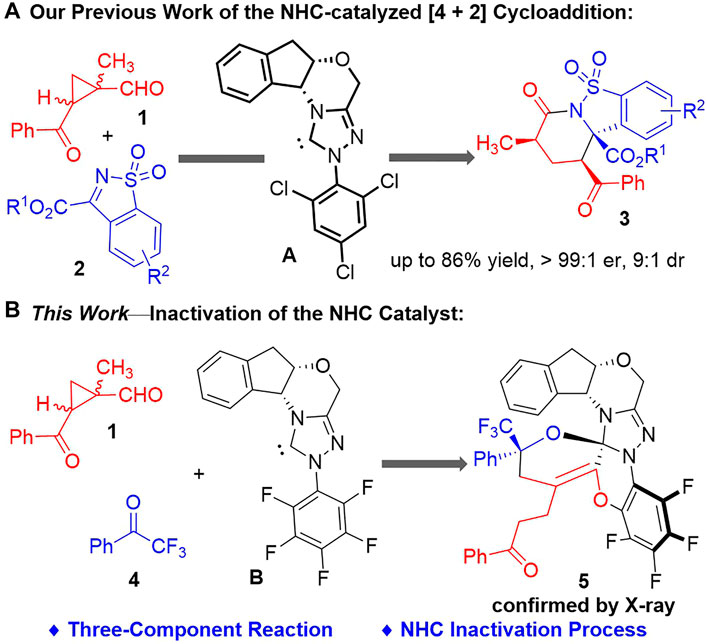
FIGURE 2. Our studies on 1-methylcyclopropylcarbaldehyde activations with NHC organic catalysts. (A) Our previous work of the NHC-catalyzed (4 + 2) cycloaddition. (B) This work—inactivation of the NHC catalyst.
After completion of our studies on the chiral NHC-catalyzed [4 + 2] cycloaddition reactions. We continue to focus on the side products formed with the NHC catalyst B bearing an N-C6F5 group (Figure 2B). To our delight, a crystalline product could be isolated from the reaction system consisted of the 1-methylcyclopropyl-carbaldehyde 1, the 2,2,2-trifluoroaceto-phenone 4 (Su et al., 2017) and the NHC catalyst B. An unexpected crystal structure of 5 was assigned by X-ray analysis, with all the three components combined within one molecule.
Results and Discussion
Having obtained the crystal structure of the NHC-bounded compound 5, we went on to optimize the reaction condition in order to improve the yield of the structural complex product 5 (Table 1). The raw materials of the 1-methylcyclopropyl-carbaldehyde 1, the 2,2,2-trifluoroacetophenone 4 and the NHC catalyst B were initially stirred in THF at room temperature in the presence of a stoichiometric amout of Et3N, with the product of 5 obtained in 12% yield (Table 1, entry 1). The yield of the target product 5 could be improved when switching Et3N in to stronger bases such as DBU and Cs2CO3 (entries 2-3). Solvents other than THF we tested were not effective for this transformation (e.g., entries 4-6). The attempts to improve the reaction yield by adding more or less amount of basic additives were failed (entries 7-8). Further increasing the reaction temperature resulted in the formation of the target product with the same yield.
The formation of the structurally complex product 5 is rationalized through a multi-step cascade cyclization process among the three components of the 1-methylcyclopropyl-carbaldehyde 1, the 2,2,2-trifluoroacetophenone 4 and the NHC catalyst B (Figure 3). After deprotonation of the NHC pre-catalyst, the free NHC B can attack the aldehyde 1 to give the adduct I, which can isomerize to give the Breslow intermediate II via an intramolecular proton shift process. The Breslow intermediate II can go through a ring-opening process to give the zwitter ionic intermediate III that is in equilibrium with the intermediate IV through intramolecular proton transfer processes. The oxide anion of the intermediate IV can attack the electron-deficient pentafluorophenyl group to form a 6-membered ring via an intramolecular O-addition/elimination (SNAr) process to give the intermediate V. The intermediate V bears an α,β-unsaturated iminium ion moiety that can easily be deprotonated by the F− anion to form the dienamine intermediate VI. A dienamine aldol reaction between the intermediate VI and the 2,2,2-trifluoroacetophenone 4 gives the adduct VII, which can further cyclize to afford the final product 5 via an intramolecular oxa-Mannich reaction process.
It is worth noting that the formation of the structurally complex compound 5 is a highly stereospecific process. Only one diastereomer is observed in all the experiments we carried out. This is probably due to the steric match/mismatch effects provided by the chiral NHC scaffold we used in this transformation.
Conclusion
In summary, we have disclosed an unprecedented inactivation process of NHC organic catalysts bearing N-C6F5 groups. A structurally complex multi-cyclic compound was obtained from the 3-component reaction of the 1-methylcyclopropyl-carbaldehyde, the 2,2,2-trifluoroacetophenone and the NHC catalyst bearing an N-C6F5 group. The absolute structure of the complex product was unambiguously assigned via X-ray analysis on its single crystals. The current study can provide novel inspections into the possible pathways that are taking place in the reactions promoted by NHC catalysts bearing N-C6F5 groups. Further investigations into the interactions between the NHC organic catalysts and various reaction substrates are in progress in our laboratories.
Data Availability Statement
The raw data supporting the conclusion of this article will be made available by the authors, without undue reservation.
Author Contributions
YC and JL conducted most of the experiments and contributed equally to this work. XP contributed to some work in manuscript writing. ZJ conceptualized and directed the whole project. ZJ drafted the manuscript. All of the authors contributed in scientific discussions.
Conflict of Interest
The authors declare that the research was conducted in the absence of any commercial or financial relationships that could be construed as a potential conflict of interest.
Publisher’s Note
All claims expressed in this article are solely those of the authors and do not necessarily represent those of their affiliated organizations, or those of the publisher, the editors and the reviewers. Any product that may be evaluated in this article, or claim that may be made by its manufacturer, is not guaranteed or endorsed by the publisher.
Acknowledgments
We acknowledge financial support from the National Natural Science Foundation of China (21961006, 32172459), the Science and Technology Department of Guizhou Province [Qiankehejichu-ZK (2021) Key033], the Program of Introducing Talents of Discipline to Universities of China (111 Program, D20023) at Guizhou University, Frontiers Science Center for Asymmetric Synthesis and Medicinal Molecules, Department of Education, Guizhou Province [Qianjiaohe KY (2020)004] and Guizhou University (China).
Supplementary Material
The Supplementary Material for this article can be found online at: https://www.frontiersin.org/articles/10.3389/fchem.2022.875286/full#supplementary-material
References
Bastin, S., Pallova, L., Morantin, M. E., Lafitte, T., Huynh, M., Barthes, C., et al. (2019). N‐Heterocyclic Carbenes as Key Intermediates in the Synthesis of Fused, Mesoionic, Tricyclic Heterocycles. Chem. Eur. J. 25, 13030–13036. doi:10.1002/chem.201903242
Berden, G., Peckelsen, K., Thomulka, T., Martens, J., Paul, M., Oomens, J., et al. (2021). Breslow Intermediates (Amino Enols) and Their Keto Tautomers: First Gas‐Phase Characterization by IR Ion Spectroscopy. Chem. Eur. J. 27, 2662–2669. doi:10.1002/chem.202003454
Berkessel, A., Elfert, S., Etzenbach-Effers, K., and Teles, J. H. (2010). Aldehyde Umpolung by N-Heterocyclic Carbenes: NMR Characterization of the Breslow Intermediate in its Keto Form, and a Spiro-Dioxolane as the Resting State of the Catalytic System. Angew. Chem. Int. Edition 49, 7120–7124. doi:10.1002/anie.200907275
Berkessel, A., Elfert, S., Yatham, V. R., Neudörfl, J.-M., Schlörer, N. E., and Teles, J. H. (2012). Umpolung by N-Heterocyclic Carbenes: Generation and Reactivity of the Elusive 2,2-Diamino Enols (Breslow Intermediates). Angew. Chem. Int. Ed. 51, 12370–12374. doi:10.1002/anie.201205878
Berkessel, A., Neudörfl, J.-M., Schlörer, N. E., and Yatham, V. R. (2015). Carbene Catalyzed Umpolung of α,β-enals: a Reactivity Study of Diamino Dienols vs. Azolium Enolates, and the Characterization of Advanced Reaction Intermediates. Chem. Sci. 6, 3706–3711. doi:10.1039/c5sc01027f
Berkessel, A., Yatham, V. R., Elfert, S., and Neudörfl, J.-M. (2013). Characterization of the Key Intermediates of Carbene-Catalyzed Umpolung by NMR Spectroscopy and X-ray Diffraction: Breslow Intermediates, Homoenolates, and Azolium Enolates. Angew. Chem. Int. Ed. 52, 11158–11162. doi:10.1002/anie.201303107
Breslow, R. (1958). On the Mechanism of Thiamine Action. IV.1 Evidence from Studies on Model Systems. J. Am. Chem. Soc. 80, 3719–3726. doi:10.1021/ja01547a064
Chen, F., Wang, H. J., Zhang, W., and Tang, P. (2020). Asymmetric Catalytic Hydrogenation of Imines and Enamines in Natural Product Synthesis. Green. Synth. Catal. 1, 2666–5549. doi:10.1016/j.gresc.2020.05.006
Chi, Y. R., Mo, J., Fang, X., and Lv, H. (2011). Formal Diels-Alder Reactions of Chalcones and Formylcyclopropanes Catalyzed by Chiral N-Heterocyclic Carbenes. Org. Lett. 13, 5366–5369. doi:10.1021/ol202250s
Chi, Y. R., Wang, H., Jin, Z., and Chen, X. (2020). N ‐Heterocyclic Carbene Organocatalysis: Activation Modes and Typical Reactive Intermediates. Chin. J. Chem. 38, 1167–1202. doi:10.1002/cjoc.202000107
Chi, Y. R., Wang, H., Jin, Z., and Yang, X. (2021). Development of Green and Low-Cost Chiral Oxidants for Asymmetric Catalytic Hydroxylation of Enals. Green. Synth. Catal. 2, 295–298. doi:10.1016/j.gresc.2021.05.002
Dirocco, D. A., and Rovis, T. (2012). Catalytic Asymmetric α-Acylation of Tertiary Amines Mediated by a Dual Catalysis Mode: N-Heterocyclic Carbene and Photoredox Catalysis. J. Am. Chem. Soc. 134, 8094–8097. doi:10.1021/ja3030164
Enders, D. (2003). Preparation and Application of 1,3,4-Triphenyl-4,5-Dihydro-1h-1,2,4-Triazol-5-Ylidene, A Stable Carbene. Synthesis 2003, 1292–1295. doi:10.1055/s-2003-3940910.1002/chin.200341135
Enders, D., and Balensiefer, T. (2004). Nucleophilic Carbenes in Asymmetric Organocatalysis. Acc. Chem. Res. 37, 534–541. doi:10.1021/ar030050j
Enders, D., Breuer, K., Raabe, G., Runsink, J., Teles, J. H., Melder, J.-P., et al. (1995). Preparation, Structure, and Reactivity of 1,3,4-Triphenyl-4,5-Dihydro-1h-1,2,4-Triazol-5-Ylidene, A New Stable Carbene. Angew. Chem. Int. Ed. Engl. 34, 1021–1023. doi:10.1002/anie.199510211
Enders, D., Niemeier, O., and Henseler, A. (2007). Organocatalysis by N-Heterocyclic Carbenes. Chem. Rev. 107, 5606–5655. doi:10.1021/cr068372z
Fu, Z. Q., Guo, J. C., Huang, J., and Zhang, Y. X. (2021). N-heterocyclic Carbene-Catalyzed [4+2] Annulation of Acetates and β-Silyl Enones: Highly Enantioselective Synthesis of β-Silyl δ-Lactones. Chin. J. Org. Chem. 41, 4467–4475. doi:10.6023/cjoc202105002
Glorius, F., Richter, C., Schedler, M., and Hopkinson, M. N. (2014). An Overview of N-Heterocyclic Carbenes. Nature 510, 485–496. doi:10.1038/nature13384
Gu, C., Du, G., Zhang, J., and Dai, B. (2017). Strecker Reaction of Ethyl Cyanoformate with Aldimines Catalyzed by N-Heterocyclic Carbene. Chin. J. Org. Chem. 37, 914–919. doi:10.6023/cjoc201610029
Liu, P., Li, X., He, J., Li, W., Yang, Z., Wei, Y., et al. (2021). TBAI-catalyzed Ring-Opening Sulfonylations of Benzothiazoles and Arylsulfonyl Hydrazides. Green. Synth. Catal. 2, 381–384. doi:10.1016/j.gresc.2021.08.006
Lupton, D. W., Candish, L., and Ryan, S. J.(2013). Acyl Anion Free N-Heterocyclic Carbene Organocatalysis. Chem. Soc. Rev. 42, 4906–4917. doi:10.1039/c3cs35522e
Lv, J., Xu, J., Pan, X., Jin, Z., and Chi, Y. R. (2021). Carbene-catalyzed Activation of Cyclopropylcarbaldehydes for Mannich Reaction and δ-lactam Formation: Remote Enantioselecitvity Control and Dynamic Kinetic Asymmetric Transformation. Sci. China Chem. 64, 985–990. doi:10.1007/s11426-021-9989-1
Mahatthananchai, J., and Bode, J. W. (2014). On the Mechanism of N-Heterocyclic Carbene-Catalyzed Reactions Involving Acyl Azoliums. Acc. Chem. Res. 47, 696–707. doi:10.1021/ar400239v
Mu, W., Dou, L., and Liu, W. (2020). Recent Progress On[3+2] Ring-Expansion Reaction of Cyclopropane with Unsaturated Compounds. Chin. J. Org. Chem. 40, 1150–1176. doi:10.6023/cjoc201910019
Nair, V., Biju, A. T., and Menon, R. S. (2015). Recent Advances in Employing Homoenolates Generated by N-Heterocyclic Carbene (NHC) Catalysis in Carbon-Carbon Bond-Forming Reactions. Chem. Soc. Rev. 44, 5040–5052. doi:10.1039/c5cs00162e
Regitz, M. (1996). Nucleophile Carbene: Eine Unglaubliche Renaissance. Angew. Chem. 108, 791–794. doi:10.1002/ange.19961080706
Rovis, T., Glover, G. S., Oberg, K. M., Dalton, D. M., and Zhao, X. (2013). SNAr-Derived Decomposition By-Products Involving Pentafluorophenyl Triazolium Carbenes. Synlett 24, 1229–1232. doi:10.1055/s-0033-1338842
Rovis, T., Oberg, K. M., and Dirocco, D. A. (2012). Isolable Analogues of the Breslow Intermediate Derived from Chiral Triazolylidene Carbenes. J. Am. Chem. Soc. 134, 6143–6145. doi:10.1021/ja302031v
Rovis, T., Read de Alaniz, J., and Kerr, M. S. (2002). A Highly Enantioselective Catalytic Intramolecular Stetter Reaction. J. Am. Chem. Soc. 124, 10298–10299. doi:10.1021/ja027411v
Rovis, T., Romanov-Michailidis, F., White, N. A., and Flanigan, D. M. (2015). Organocatalytic Reactions Enabled by N-Heterocyclic Carbenes. Chem. Rev. 115, 9307–9387. doi:10.1021/acs.chemrev.5b00060
Scheidt, K. A., Jaworski, A. A., and Murauski, K. J. R. (2018). A Continuing challenge: N-Heterocyclic Carbene-Catalyzed Syntheses of γ-butyrolactones. Chem. Soc. Rev. 47, 1773–1782. doi:10.1039/c7cs00386b
Sheehan, J. C., and Hunneman, D. H. (1966). Homogeneous Asymmetric Catalysis. J. Am. Chem. Soc. 88, 3666–3667. doi:10.1021/ja00967a049
Su, Y., Wu, L., Chong, S., Zhang, W., Huang, D., Wang, K., et al. (2017). Chiral Thiourea Catalyzed Asymmetric Henry Reaction: Construction of Stereogenic Center Bearing a CF3 Group from 2,2,2-Trifluoroacetophenone Substrates. Chin. J. Org. Chem. 37, 936–942. doi:10.6023/cjoc201612006
Tong, M., Zhang, X., Wang, Y., and Wang, Z. (2021). Advances in Reactions of Iodonium Ylides. Chin. J. Org. Chem. 41, 126–143. doi:10.6023/cjoc202006021
Ukai, T., Tanaka, R., and Dokawa, T. (1943). A New Catalyst for the Acyloin Condensation. J. Pharm. Soc. Jpn. 63, 296–304. doi:10.1248/yakushi1881.63.6-296
Van Halbeek, H., and Poppe, L. (1991). Nuclear Magnetic Resonance of Hydroxyl and Amido, Protons of Oligosaccharides in Aqueous Solution: Evidence for A Strong Intramolecular Hydrogen Bond in Sialic Acid Residues. J. Am. Chem. Soc. 113, 363–365. doi:10.1021/ja00001a055
Wang, A., Zhou, Y., Xu, J., and Liu, H. (2017). Progress of Organic Reactions Catalyzed by N-Heterocyclic Carbenes. Chin. J. Org. Chem. 37, 2590–2608. doi:10.6023/cjoc201702041
Wang, H., Gu, S., Yan, Q., Ding, L., and Chen, F.-E. (2020a). Asymmetric Catalysis in Synthetic Strategies for Chiral Benzothiazepines. Green. Synth. Catal. 1, 12–25. doi:10.1016/j.gresc.2020.05.005
Wang, J., Blaszczyk, S. A., and Zhao, C. (2021). Asymmetric Reactions of N-Heterocyclic Carbene (NHC)-Based Chiral Acyl Azoliums and Azolium Enolates. Green. Synth. Catal. 2, 198–215. doi:10.1016/j.gresc.2021.03.003
Wang, P., Zhao, Q., Xiao, W., and Chen, J. (2020b). Recent Advances in Visible-Light Photoredox-Catalyzed Nitrogen Radical Cyclization. Green. Synth. Catal. 1, 42–51. doi:10.1016/j.gresc.2020.05.003
Xu, M.-H., Jiang, T., and Wang, H. (2013). Simple Branched Sulfur-Olefins as Chiral Ligands for Rh-Catalyzed Asymmetric Arylation of Cyclic Ketimines: Highly Enantioselective Construction of Tetrasubstituted Carbon Stereocenters. J. Am. Chem. Soc. 135, 971–974. doi:10.1021/ja3110818
Xue, X.-S., and Zheng, H. (2021). Mechanism and Selectivity of N-Heterocyclic Carbene-Catalyzed Desymmetrizing [4+1] and [4+2] Annulations+1] and [4+2] Annulations.Chin. Chin. J. Org. Chem. 41, 2530–2531. doi:10.6023/cjoc202100047
Yao, C. S., Yang, W. H., Luo, X., and Li, S. (2019). An Efficient N-Heterocyclic Carbene (NHC)-Catalyzed Synthesis of Polysubstituted Cyclopentene.Chin. J. Org. Chem. 39, 140–1410. doi:10.6023/cjoc201812035
Keywords: catalyst inactivation, N-heterocyclic carbene, organocatalysis, 3-component reaction, multi-step cascade cyclization
Citation: Chen Y, Lv J, Pan X and Jin Z (2022) An Unexpected Inactivation of N-Heterocyclic Carbene Organic Catalyst by 1-Methylcyclopropylcarbaldehyde and 2,2,2-Trifluoroacetophenone. Front. Chem. 10:875286. doi: 10.3389/fchem.2022.875286
Received: 14 February 2022; Accepted: 18 February 2022;
Published: 24 March 2022.
Edited by:
Yaqiong Su, Xi’an Jiaotong University, ChinaCopyright © 2022 Chen, Lv, Pan and Jin. This is an open-access article distributed under the terms of the Creative Commons Attribution License (CC BY). The use, distribution or reproduction in other forums is permitted, provided the original author(s) and the copyright owner(s) are credited and that the original publication in this journal is cited, in accordance with accepted academic practice. No use, distribution or reproduction is permitted which does not comply with these terms.
*Correspondence: Zhichao Jin, zcjin@gzu.edu.cn
†These authors have contributed equally to this work