- College of Agronomy, Sichuan Agricultural University, Chengdu, China
The development of new biological fungicides using plant metabolites has become an important direction for pesticide development, and previous studies found that Radix Aucklandiae had a certain inhibitory effect on plant pathogens. In this study, we systematically studied the antimicrobial activity of extracts of Radix Aucklandiae, and the active compounds were isolated, purified and structurally identified. Ethanol extracts of Radix Aucklandiae had different inhibitory effects on seven common plant-pathogenic fungi, with EC50 (concentration for 50% of maximal effect) values ranging from 114.18 mg/L to 414.08 mg/L. The extract at concentration of 1,000 mg/L had a significant control effect on strawberry grey mould and wheat powdery mildew of more than 90%. Three active compounds were isolated and purified from the extract, which were identified as alantolactone, dehydrocostus lactone and costunolide. All three compounds showed significant inhibitory effects on Botrytis cinerea, and the MIC (minimal inhibitory concentration) values were 15.63 mg/L, 3.91 mg/L and 15.63 mg/L. Dehydrocostus lactone also showed obvious inhibitory effect on Fusarium graminearum with an MIC value of 62.25 mg/L. The extract of Radix Aucklandiae has high antimicrobial activity against some common plant-pathogenic fungi, and the work lays a foundation for the development of extracts of Radix Aucklandiae as botanical fungicides.
Introduction
Plant-pathogenic fungi can cause a decline in crop yield and quality directly or indirectly and secrete a variety of toxins and harmful metabolites (Guo et al., 2021). Therefore, it is necessary to control fungal diseases in agricultural production. At present, chemical fungicides are widely used to control plant diseases. However, chemical fungicide abuse has had a serious negative impact on human health and environmental safety, especially leading to prominent “3R” (Resistance, resurgence, and Residue) problems (Ju, 2011). Therefore, it is urgent to develop environmentally friendly fungicides. Botanical fungicides are pesticides used to control plant diseases with the advantages of high efficiency, low or no toxicity, easy degradation, high selectivity and a low risk of inducing drug resistance (Bhandari et al., 2021). Therefore, the development of new botanical fungicides is a hot spot in the development of new environmentally friendly pesticides. At present, many plant extracts have been proven to have antimicrobial activity. For example, extracts of Sophora flavescens have inhibitory effect on Gibberella zeae, Glomerella cingulata and Botrytis cinerea (Li et al., 2006). Extracts of Syzygium aromaticum (L.) have inhibitory effect on Colletotrichum gloeosporioides and Fusarium oxysporum f. sp. cubense (He et al., 2006).
Radix Aucklandiae (Chinese trade name: Muxiang) is the dried root of Aucklandia lappa Dence. (a perennial herb of the genus Saussurea, family Compositae), which is cultivated in Yunnan and Sichuan Provinces (Shu et al., 2015). It is widely used in clinical medicine because of its anti-inflammatory, anti-ulcer, hepatoprotective, cholagogic, antitumour and other functions (Bocca et al., 2004; Lai et al., 2008; Wang et al., 2008; He et al., 2011; Butturini et al., 2014; Sun et al., 2015). In addition, it has also been proven that Radix Aucklandiae has antimicrobial activity in agricultural production. The water extract of Radix Aucklandiae has inhibitory effect on Botrytis cinerea and Alternaria alternata (Hasi et al., 2009). Its ethanol extract also can inhibit Penicillium italicum Wehmer, Verticillium dahlia, Fusarium oxysporium, Rhizoctonia solani Kuhn and Gloeosporium piperatum (Hu et al., 2009; Liu et al., 2011; Wang et al., 2012; Jin et al., 2019). However, the antimicrobial activity compounds of Radix Aucklandiae is not clear and has not been systematically explored at present.
The inhibitory effect of extracts of Radix Aucklandiae on common plant-pathogenic fungi was systematically studied based on the above, and active compounds of extracts of Radix Aucklandiae were isolated, purified and identified, which laid a foundation for the further development and utilization of the extract as a fungicide.
Materials and Methods
Materials
Radix Aucklandiae was purchased from Bozhou Huakai Electronic Commerce Co., Ltd. The 11 common pathogens including F. graminearum, Blumeria graminis (Bgt), B. cinerea, C. gloeosporioides, Sclerotinia sclerotiorum, F. oxysporum, Fusarium lateritium, A. alternata, Pythium aphanidermatum, D. glomerata and Phytophthora infestans were preserved and provided by the College of Agronomy of Sichuan Agricultural University. The wheat (Triticum aestivum L.) variety Chuannong 30, which is mildew and is grown at the College of Agronomy of Sichuan Agricultural University.
Preparation of Extracts of Radix Aucklandiae
Plant extracts were prepared by solvent extraction (Zhang and Wang, 2011; Chen et al., 2012). Radix Aucklandiae was dried in an electrothermal constant temperature blast drying oven at 55°C, crushed into dry powder with a tissue grinder and stored in a sealed fresh-storage bag away from light. Dry powder (2 kg) was extracted by soaking in 5 times volume ethanol while avoiding light. The extract was filtered out, and the same amount of ethanol was added after 3 days. The extract was filtered out after 2 days, and the two parts of the filtrate were combined. The filtrate was concentrated to paste at 50–60°C by a rotary evaporator and stored in a refrigerator at 4°C for later use.
Toxicity Determination of Extracts of Radix Aucklandiae
The toxicity was determined by the mycelium growth rate (Wang et al., 2014). The paste extract was dissolved in dimethyl sulfoxide (DMSO) and then prepared into 1,000 mg/L, 500 mg/L, 250 mg/L, 125 mg/L, 62.5 mg/L, and 31.25 mg/L solutions with double distilled water (contain 0.1% Tween 80). The solution (1 ml) was mixed with Potato Dextrose Agar (PDA) medium (containing 1% streptomycin sulfate) (9 ml) and then poured into a sterile Petri dish (9 cm in diameter) to make a medium-filled plate. After the medium was solidified, an agar block (with a diameter of 0.5 cm) containing pathogenic fungi to be tested was placed in each medium plane, and the side of the agar block containing fungi was placed onto the surface of the medium. Each concentration was tested using three biological replicates. Double distilled water (containing 0.1% Tween 80 and 2% DMSO) was used as the negative control. Pathogenic fungi were cultured at 25°C. The diameter of colony growth was measured by the cross method after 2–7 days, and the inhibition rate of mycelium growth was calculated. Then, the toxicity regression equation and EC50 value of the extract were obtained according to the probit analysis method for toxicity.
where CK is the negative control colony growth diameter, and PT is the colony growth diameter under extract solution treatment.
Bioassay of Extracts of Radix Aucklandiae In Vivo
Control Effect on Wheat Powdery Mildew
The paste extract was dissolved in DMSO and then prepared into 1,000 mg/L, 500 mg/L and 250 mg/L solutions with double distilled water (contain 0.1% Tween 80). Wheat seeds were planted in a glass tube (4 cm in diameter), and the tube was sealed with parafilm. Seeds were cultured to the three-leaf stage at 20 ± 1°C and 60–70% humidity with a 16:8 h light/dark photoperiod. Then, wheat leaves were sprayed with 250 mg/L, 500 mg/L and 1,000 mg/L extract solutions with three replications at each concentration. Leaves were also sprayed with 100 mg/L prothioconazole as a positive control and with double distilled water (contain 2% DMSO and 0.1% Tween 80) as a negative control. Fresh spores of Bgt were inoculated onto the plants by shaking over the foliage of the wheat seedlings (Xie et al., 2021). The protective activity was determined by spray application of the extract solution first followed by inoculation with the pathogenic fungi 24 h later; the curative activity was determined by inoculation with the pathogenic fungi first followed by spray application of the extract solution 24 h later. Protective activity and curative activity were reflected by relative disease control efficiency (RDCE). The methods for detecting the protective and curative activities of the subsequent experiment were the same. Wheat was further cultured after treatment, and the disease incidence was investigated 7, 9 and 11 days after treatment. The disease was divided into six grades according to the percentage of lesion area to leaf area (Grade 0: 0%; Grade 1: less than 5%; Grade 3: 6–10%; Grade 5: 11–20%; Grade 7: 21–50%; Grade 9: more than 51%) (Liu et al., 2000). Then disease index and RDCE was calculated using the following formula:
Where, CK is DI of sterile water-treatment; PT is DI of medicament treatment.
Control Effect on Wheat Head Blight
Wheat seeds were planted in pots (15 cm in diameter) and cultured to the earing and flowering stage at 26 ± 2°C and 65–75% humidity in the greenhouse. Then, wheat leaves were sprayed with 400 mg/L and 4,000 mg/L extract solutions with three replications at each concentration. Leaves were also sprayed with 100 mg/L tebuconazole as a positive control and with double distilled water (contain 2% DMSO and 0.1% Tween 80) as a negative control. A spore suspension of F. graminearum (10 μL) was injected into wheat panicles with a microinjector. Spores were observed and counted on a haemocytometer under an optical microscope (4 × 10). The method for adjusting the spore suspension in the later experiment was the same. It is advisable to adjust the spore concentration to 80–100 spores per field. Wheat was further cultured after treatment, and the disease incidence was investigated 7, 9 and 12 days after treatment. The disease was divided into five grades according to the percentage of dry ear area to ear area (Grade 0: 0%; Grade 1: less than 25%; Grade 3: 26–50%; Grade 5: 51–75%; Grade 7: more than 76%) (Zhu et al., 2007). Then the disease index and the RDCE was calculated using the formula in 4.2.1.
Control Effect on Strawberry Grey Mould
Fresh strawberry fruits of uniform size were selected, soaked in 75% ethanol for 2 min, washed with double distilled water and then dried. Then, fruits were sprayed with 500 mg/L and 1,000 mg/L extract solutions with three replications at each concentration. Leaves were also sprayed with 100 mg/L pyraclostrobin as a positive control and with double distilled water (contain 2% DMSO and 0.1% Tween 80) as a negative control. The equator of the strawberry was pricked with a sterile needle of a 1 ml injector, a 2 mm wound was formed, and then a suspension of B. cinerea was evenly spread on the fruit surface (Han et al., 2019). The fruit was placed in a culture plate and stored at 25°C and 95% humidity after treatment. The disease incidence was investigated 3, 5 and 7 days after treatment. The disease was divided into six grades according to the percentage of lesion area to fruit surface area (Grade 0: 0.0%; Grade 1: less than 5.0%; Grade 2: 5.1–15.0%; Grade 3: 15.1–30.0%; Grade 4: 30.1–50.0%; Grade 5: more than 50.1%) (Han et al., 2019), and then the disease index and the RDCE were calculated using the formula in 4.2.1.
Control Effect on Citrus Anthracnose
Fruits of Jincheng orange with consistent appearance and no mechanical damage were selected, soaked in 75% ethanol for 2 min, washed with double distilled water and then dried. Then, the fruits were sprayed with 2000 mg/L and 4,000 mg/L extract solutions. Three biological replicates were performed at each concentration with 10 fruits per treatment. Leaves were also sprayed with 86 mg/L pyraclostrobin as a positive control and with double distilled water (contain 2% DMSO and 0.1% Tween 80) as a negative control. C. gloeosporioides was inoculated by needle puncture. The depth of the pinhole was 2 mm, and a spore suspension (10 μL) was dropped at the pinhole (Tian et al., 2019). The fruits were cultured at 28°C and 95% relative humidity after treatment. The diameters of the lesions were measured by the cross method, and the mycelium growth inhibition rate 7 days after treatment was calculated using the formula in 2.3.
Data Processing and Analysis
The inhibition results of different concentrations of extracts of Radix Aucklandiae against different pathogenic fungi were recorded, and the data were statistically analysed with SPSS Statistics 23.
Isolation, Purification and Structure Identification of Active Compounds From Radix Aucklandiae
Macroporous Resin Isolation
D101 macroporous resin was soaked in absolute ethanol for 24 h. Then, it was loaded into the chromatography column after the resin was fully swollen. The resin was rinsed repeatedly with absolute ethanol until the supernatant was free of white turbidity and then rinsed with distilled water until no ethanol was available. The paste extract of Radix Aucklandiae was evenly dispersed in double distilled water. Then, the treated solution (10 L) was adsorbed statically with D101 macroporous resin (2 kg) for 48 h. Then, resin was loaded into the chromatographic column. The resin was eluted with distilled water (3 times the volume of the column), and the eluent was discarded. Then, the resin was eluted with 70% ethanol (5 times the volume of the column). The eluent was collected and concentrated under pressure to obtain the crude extract of Radix Aucklandiae.
Chromatography Isolation
The crude extract was evenly dispersed in double distilled water. The aqueous solution was extracted with petroleum ether at the same volume 4 times. The organic phase was concentrated under reduced pressure at 45°C to obtain petroleum ether extract. The extract was isolated by normal-phase silica gel chromatography and then eluted with different petroleum ether and ethyl acetate mixtures (50:0, 10:1 and 1:1 by volume). Three fractions (Fr. H1-H3) were isolated and antimicrobial active fractions were traced and selected by the spore germination method using B. cinerea as an indicator pathogen. After further silica gel column chromatography isolation, the fraction Fr.H1-1 was obtained by eluting Fr.H1 with petroleum ether: acetone (15:1 by volume) as the eluent. In addition, the fractions Fr. H2-1 and Fr. H3-1 were obtained by eluting Fr.H2 and Fr. H3 with petroleum ether and ethyl acetate mixtures (15:1, 20:1 and 1:1 by volume) as the eluent.
Preparation of High-Performance Liquid Chromatography (HPLC)
Fractions (Fr. H1-1, Fr. H2-1 and Fr. H3-1) were further isolated through a Phenomenex C18 column (250 × 10 mm, Phenomenex, Aschaffenburg, Germany) and UV detector. Fr. H1-1 was eluted with 30% acetonitrile (containing 0.1% formic acid) to obtain compounds numbered Compound 1 (20 mg), Compound 2 (20 mg) and Compound 3 (5 mg). Fr. H2-1 was eluted with 35% acetonitrile (containing 0.1% formic acid) to obtain compounds numbered Compound 4 (20 mg) and Compound 5 (20 mg). Fr. H3-1 was eluted with 35% acetonitrile (containing 0.1% formic acid) to obtain compounds numbered Compound 6 (20 mg) and Compound 7 (20 mg). The antimicrobial active compound was traced and selected by the spore germination method using B. cinerea as indicator pathogen.
Compound Structure Identification
The purity was determined by HPLC. The mass spectrum, 1H-NMR (nuclear magnetic resonance) and 13C-NMR spectra of the compounds with higher purity were determined (the 1H-NMR spectra were 400 MHz; the 13C-NMR spectra were 100 MHz; and the solvent was CDCl3). The chemical structure of the compound was identified according to spectroscopic data.
Compound Activity Identification
The MIC against the pathogen spores was determined by the microtiter method (Yang et al., 2012). The pure compound was prepared with DMSO in mother liquor at 2000 mg/L. Then, mother liquor was diluted with double distilled water to nine concentrations: 1,000 mg/L, 500 mg/L, 250 mg/L, 125 mg/L, 62.25 mg/L, 31.125 mg/L, 15.625 mg/L, 7.8125 mg/L, 3.906 mg/L, 1.953 mg/L. Compound solutions of different concentrations (5 μL) and PDA (45 μL) were added to each well of a 96-well plate and fully mixed. Spore suspension (10 μL) was added to each well after standing for 10 min, and DMSO solution (10 μL) was added as a negative control. Three biological replicates were performed per treatment. The degree of conidial germination in all wells was observed to determine the MIC value after cultivation at 28°C for 48 h.
Results
Toxicity Determination of Extracts of Radix Aucklandiae
The extract of Radix Aucklandiae showed different degrees of inhibition on the mycelial growth of 10 different plant-pathogenic fungi (Table 1). Among them, the inhibitory effects of the extract on B. cinerea, S. sclerotiorum, C. gloeosporioides, F. oxysporum, A. alternata, F. graminearum and D. glomerata were significant, and the EC50 values were 114.18 mg/L, 142.40 mg/L, 251.87 mg/L, 299.34 mg/L, 315.07 mg/L, 398.74 mg/L and 414.08 mg/L, respectively. The extract had a poor inhibitory effect on P. infestans, F. lateritium and P. aphanidermatum, and the EC50 values were in the range of 500–1,000 mg/L.
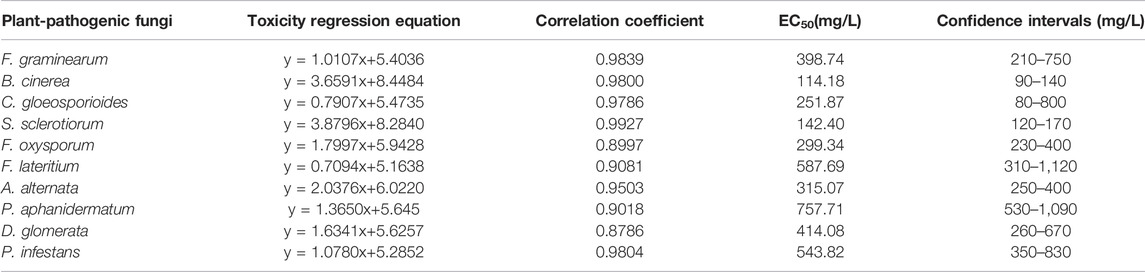
TABLE 1. Toxicity test results of extracts of Radix Aucklandiae against several plant-pathogenic fungi.
Bioassay of Extracts of Radix Aucklandiae In Vivo
Control Effect on Wheat Powdery Mildew
The different concentrations of extracts of Radix Aucklandiae had different effects on the control of wheat powdery mildew (Table 2). With the increase in the concentration of the extract, the control effect on wheat powdery mildew was higher, and the protective activity was better than the curative activity. When the extract concentrations were 1,000 mg/L, the RDCEs of protective and curative acivities were higher than that of the control agent (100 mg/L propiconazole).
Statistical significance was determined using one-way ANOVA. Different lowercase letters in the footnote in the same column showed significant difference in the relative disease control efficiency of different treatment at each time points (p < 0.05).
Control Effect on Wheat Head Blight
Different concentrations of extracts of Radix Aucklandiae had different degrees of control effects on wheat head blight (Table 3). The higher the concentration, the better the control effect, and the protective activity was better than the curative activity, but the control effect of the extract was lower than that of the control agent (86 mg/L Tebuconazole). When the concentration of extracts was 4,000 mg/L, the protective and curative activities of wheat head blight were the best. The RDCEs of the protective activities were 56.26%, 51.98 and 11.90%, and of curative activities were 40.63, 36.39, 11.90% at 7, 9 and 12 days after treatment, respectively.
Statistical significance was determined using one-way ANOVA. Different lowercase letters in the footnote in the same column showed significant difference in the relative disease control efficiency of different treatment at each time points (p < 0.05).
Control Effect on Strawberry Grey Mould
Different concentrations of extracts of Radix Aucklandiae had different degrees of control effects on strawberry grey mould (Table 4). When the concentration of the extract was 500 mg/L, it had obvious protective and curative activitys on strawberry gray mold. The RDCEs of the protective activities were 100.00, 85.71 and 77.78%, and those of the curative activities were 100.00, 75.00, 63.64% at 3, 5 and 7 days after treatment, respectively. When the concentration of extract was 1,000 mg/L, the RDCE of protective and curative activities reached 100%, which can achieve the control effect of the control agent (100 mg/L pyraclostrobin).
Statistical significance was determined using one-way ANOVA. Different lowercase letters in the footnote in the same column showed significant difference in the relative disease control efficiency of different treatment at each time points (p < 0.05).
Control Effect on Citrus Anthracnose
Different concentrations of extracts of Radix Aucklandiae had different degrees of control effects on citrus anthracnose (Table 5). The higher the concentration, the better the control effect, and the protective activity was better than the therapeutic effect, but the control effect of the extract was lower than that of the control agent (86 mg/L Tebuconazole). When the concentration of the extract was 4,000 mg/L, the protective and curative activities on citrus anthracnose were the best. The inhibitory rate for the protective activity was 67.65%, and that of the protective activity was 57.5% at 7 days after treatment.

TABLE 5. Control effect of extracts of Radix Aucklandiae on citrus anthracnose in vivo at 7 days after treatment.
CK is negative control. Statistical significance was determined using one-way ANOVA. Different lowercase letters in the footnote in the same column showed significant difference in the inhibitory rate (p < 0.05).
Chemical Structure Identification of Active Compounds From Radix Aucklandiae
Compound 2
Compound 2 was obtained as white amorphous powder. Its molecular formula was determined to be C15H20O2 based on the HRESIMS data (m/z 233.1540 [M + H]+), indicating six degrees of unsaturation. The 1H-NMR data (Table 6) showed four olefinic protons at δH 6.24 (1H, d, J = 3.6 Hz), 5.51 (1H, d, J = 3.2 Hz), 4.83 (1H, m) and 4.72 (1H, d, J = 9.9 Hz); one oxygen-bearing methine signal at δH 4.55 (1H, dd, 8.8, 9.8), a methine signal at δH 2.55 (1H, m), and two methyl proton signal at δH 1.68 (3H, d, J = 1.3 Hz), 1.40 (3H, s). The 13C-NMR spectrum (Table 6) contained fifteen carbon signals, which were assigned to one lactone carbonyl carbon (δc 170.6), six olefinic carbons (δC 141.4, 140.0, 136.9, 127.2, 127.0, 119.6), one oxy-methine carbon (δC 81.9), one methine carbon (δC 50.3), four methylenes (δC 40.9, 39.4, 28.0, 26.1), and two methyl carbons (δc 17.3, 16.0). These spectral data were elucidated to the published data of Costunolide (Kaur et al., 2017; Chacon-Morales et al., 2020). Therefore, compound 2 was identified as costunolide and its molecular formula is shown in Figure 1. MS diagrams and 1H-NMR and 13C-NMR spectra were shown in Figures S1, S2 and S3, respectively.
Compound 5
Compound 5 was obtained as white amorphous powder. Its molecular formula was determined to be C15H20O2 based on the ESIMS data (m/z 233.1 [M + H]+), indicating six degrees of unsaturation. The 1H-NMR data (Table 6) showed three olefinic protons [δH 6.18 (1H, d, J = 1.9 Hz), 5.61 (1H, d, J = 1.6 Hz), 5.14 (1H, d, J = 4.1 Hz)]; one oxygen-bearing methine signal at δH 4.81 (1H, dt, 3.0, 6.5), three methine signals at δH 3.56 (1H, m), 2.43 (1H, m), and two methyl proton signal at δH 1.18 (3H, s), 1.08 (3H, d, J = 7.6 Hz),. The 13C-NMR spectrum (Table 6) contained fifteen carbon signals, which were assigned to one lactone carbonyl carbon (δc 170.6), four olefinic carbons (δc 149.2, 140.0, 121.8, 118.9), one oxy-methine carbon (δc 76.6), two methine carbons (δC 39.6, 37.7), four methylenes (δC 42.8, 41.9, 32.8, 16.9), and two methyl carbons (δC 28.7, 22.7). These spectral data were elucidated to the published data of Alantolactone (Neves et al., 1999; Yuuya et al., 1999). Therefore, compound 5 was identified as dehydrocostus lactone and its molecular formula is shown in Figure 2. MS diagrams and 1H-NMR and 13C-NMR spectra were shown in Supplementary Figures S4–S6, respectively.
Compound 6
Compound 6 was obtained as white amorphous powder. Its molecular formula was determined to be C15H20O2 based on the ESIMS data (m/z 233.1 [M + H]+), indicating six degrees of unsaturation. The 1H-NMR data (Table 6) showed three olefinic protons [δH 6.18 (1H, d, J = 1.9 Hz), 5.61 (1H, d, J = 1.6 Hz), 5.14 (1H, d, J = 4.1 Hz)]; one oxygen-bearing methine signal at δH 4.81 (1H, dt, 3.0, 6.5), three methine signals at δH 3.56 (1H, m), 2.43 (1H, m), and two methyl proton signal at δH 1.18 (3H, s), 1.08 (3H, d, J = 7.6 Hz),. The 13C-NMR spectrum (Table 6) contained fifteen carbon signals, which were assigned to one lactone carbonyl carbon (δC 170.6), four olefinic carbons (δC 149.2, 140.0, 121.8, 118.9), one oxy-methine carbon (δC 76.6), two methine carbons (δC 39.6, 37.7), four methylenes (δC 42.8, 41.9, 32.8, 16.9), and two methyl carbons (δC 28.7, 22.7). These spectral data were elucidated to the published data of Alantolactone (Ming et al., 1989; Dereli et al., 2020). Therefore, compound 6 was identified as alantolactone and its molecular formula is shown in Figure 3. MS diagrams and 1H-NMR and 13C-NMR spectra were shown in Supplementary Figures S7–S9, respectively.
Antifungal Activity of Active Compoundsactive Ingredients
Alantolactone, dehydrocostus lactone and costunolide isolated from extracts of Radix Aucklandiae had inhibitory effects on the spore germination of F. graminearum, B. cinerea, C. gloeosporioides and F. oxysporum (Table 7). The MIC values of alantolactone against B. cinerea and F. graminearum were 15.63 mg/L and 250 mg/L, and those against C. gloeosporioides and F. oxysporum were both more than 1,000 mg/L. The MIC values of dehydrocostus lactone against F. graminearum, B. cinerea, C. gloeosporioides and F. oxysporum were 3.91 mg/L, 62.25 mg/L, 125 mg/L, 250 mg/L, respectively. The MIC values of costunolide against B. cinerea and F. graminearum were 15.625 mg/L and 1,000 mg/L, and those against C. gloeosporioides and F. oxysporum were both more than 1,000 mg/L.
Discussion
Radix Aucklandiae is the dried root of Aucklandia lappa Dence. (Genus Saussurea, family Compositae). There are a few reports about the antimicrobial activity of the extract in medicine and agriculture. Research has reported that the extract has antimicrobial activity against Helicobacter pylori and Streptococcus in medicine (Yu et al., 2007; Han et al., 2011). The extract also has antimicrobial activity against B. cinerea, A. alternata, Penicillium italicum Wehmer, Verticillium dahliae and F. oxysporum (Hasi et al., 2009; Hu et al., 2009; Wang et al., 2012; Jin et al., 2019). In this study, extracts of Radix Aucklandiae had significant inhibitory effects on B. cinerea, S. sclerotiorum and C. gloeosporioides, and had obvious control effects on wheat powdery mildew, wheat head blight, strawberry grey mould and citrus anthracnose. Therefore, the extract has potential development and application value as a botanical fungicide.
The chemical composition of Radix Aucklandiae is diverse. At present, there have been many reports on the chemical composition of extracts of Radix Aucklandiae. For example, more than 200 compounds, such as sesquiterpene lactones, monoterpenes, phenylpropanoids, lignans, flavonoids and volatile oils, have been isolated from Radix Aucklandiae (Mao et al., 2017). In this study, three active compounds were isolated and purified from Radix Aucklandiae, and identified as alantolactone, dehydrocostus lactone and costunolide, which are all terpenoids.
At present, the biological activities of these three compounds have mainly been reported in the context of medicine and are less known in agriculture. In medicine, it was found that these compounds had antimicrobial, antitumor, anti-inflammatory, hepatoprotective and other pharmacological effects. These compounds can inhibit Fusarium solani (Mart.) Sacc., Mycobacterium tuberculosis and Staphylococcus aureus in the human body (Wahab et al., 1979; Cantrell et al., 1999; O’Shea et al., 2009). In agriculture, recent research has also shown that alantolactone has a significant inhibitory effect on Phytophthora nicotianae (Feng et al., 2018). Dehydrocostus lactone and costunolide have inhibitory effects on Cunninghamella echinulata, Colletotrichum acutatum, B. cinerea and F. oxysporum (Barrero et al., 2000; Wedge et al., 2000). In this study, alantolactone, dehydrocostus lactone and costunolide all had different degrees of inhibitory effects on F. graminearum, B. cinerea, C. gloeosporioides and F. oxysporum. Alantolactone have inhibitory effects on F. graminearum and B. cinerea, and costunolide have inhibitory effects on B. cinerea, while dehydrocostus lactone has inhibitory effects on four plant-pathogenic fungi. Among them, dehydrocostus lactone showed the best control effect on plant fungous diseases.
Dehydrocostus lactone is a guaiane-type sesquiterpene isolated from Radix Aucklandiae. Dehydrocostus lactone is mainly extracted by solvent extraction, microwave-assisted extraction, ultrasonic-assisted extraction, and separated and purificated by column chromatography (He et al., 2009; He et al., 2010). There are still difficulties in large-scale extraction. At present, the research on the structural modification and derivation of dehydrocostus lactone mainly focuses on Michael addition reaction at C-13 site and some oxidation reactions (Qian et al., 2012). The erivatives played an important role in tumor therapy. However, whether dehydrocostus lactone can be used as a lead compound in agricultural disease control remains to be verified. This study laid a foundation for the further development and utilization of extracts of Radix Aucklandiae as botanical fungicides.
Data Availability Statement
The raw data supporting the conclusions of this article will be made available by the authors, without undue reservation.
Author Contributions
XC, CY and GQ: Experimental design and the draft writing; MZ and HC: Experimental guidance and article modification; YB, XQ and LL: Participating in part experimental processes.
Funding
This work was supported by the National Key R&D Program of China under Grant Nos. 2018YFD0200500, and the Biotechnology and Medicine of the Major Scientific and Technological Project of Sichuan Province under Grant Nos. 2017NZDZX0003.
Conflict of Interest
The authors declare that the research was conducted in the absence of any commercial or financial relationships that could be construed as a potential conflict of interest.
Publisher’s Note
All claims expressed in this article are solely those of the authors and do not necessarily represent those of their affiliated organizations, or those of the publisher, the editors and the reviewers. Any product that may be evaluated in this article, or claim that may be made by its manufacturer, is not guaranteed or endorsed by the publisher.
Supplementary Material
The Supplementary Material for this article can be found online at: https://www.frontiersin.org/articles/10.3389/fchem.2022.872480/full#supplementary-material
References
Barrero, A. F., Oltra, J. E., Álvarez, M., Raslan, D. S., Saúde, D. A., and Akssira, M. (2000). New Sources and Antifungal Activity of Sesquiterpene Lactones. Fitoterapia 71 (1), 60–64. doi:10.1016/s0367-326x(99)00122-7
Bhandari, S., Yadav, P. K., and T Sarhan, A. (2021). Botanical Fungicides; Current Status, Fungicidal Properties and Challenges for Wide Scale Adoption: A Review. Review.food.agr. 2 (2), 63–68. doi:10.26480/rfna.02.2021.63.68
Bocca, C., Gabriel, L., Bozzo, F., and Miglietta, A. (2004). A Sesquiterpene Lactone, Costunolide, Interacts with Microtubule Protein and Inhibits the Growth of MCF-7 Cells. Chemico-Biological Interactions 147 (1), 79–86. doi:10.1016/j.cbi.2003.10.008
Butturini, E., Di Paola, R., Suzuki, H., Paterniti, I., Ahmad, A., Mariotto, S., et al. (2014). Costunolide and Dehydrocostuslactone, Two Natural Sesquiterpene Lactones, Ameliorate the Inflammatory Process Associated to Experimental Pleurisy in Mice. Eur. J. Pharmacol. 730 (1), 107–115. doi:10.1016/j.ejphar.2014.02.031
Cantrell, C. L., Abate, L., Fronczek, F. R., Franzblau, S. G., Quijano, L., and Fischer, N. H. (1999). Antimycobacterial Eudesmanolides from Inula Helenium and Rudbeckia Subtomentosa. Planta Med. 65 (4), 351–355. doi:10.1055/s-1999-14001
Chacón-Morales, P. A., Dugarte, C. S., and Amaro-Luis, J. M. (2020). Helenin from Stevia Lucida. The First Report of This Natural Eudesmanolide Mixture in Eupatorieae Tribe. Nat. Product. Res. 35, 4139–4142. doi:10.1080/14786419.2020.1739677
Chen, X., Pan, R., and Gai, Y. (2012). Antimicrobial Activities in Methanol Extracts of 31 Plant Species against Peronophythora Litchii and Colletotrichum Gloeosporioides. Guangdong Agric. Sci. 39 (3), 1–3. doi:10.16768/j.issn.1004-874x.2012.03.045
Feng, C., Zhan, H., Cui, M., Xu, C., Wang, X., and Chen, D. (2018). Chemical Identification of Tobacco Root Exudates and Their Effect on Phytophthora Nicotianae. J. Anhui Agric. Sci. 46 (17), 148–152. doi:10.13989/j.cnki.0517-6611.2018.17.044
Guo, Y., Chen, J., Ren, D., Du, B., Wu, L., Zhang, Y., et al. (2021). Synthesis of Osthol-Based Botanical Fungicides and Their Antifungal Application in Crop protection. Bioorg. Med. Chem. 40, 116184. doi:10.1016/j.bmc.2021.116184
Gürağaç Dereli, F. T., Ilhan, M., and Küpeli Akkol, E. (2020). Identification of the Main Active Antidepressant Constituents in A Traditional Turkish Medicinal Plant, Centaurea Kurdica Reichardt. J. Ethnopharmacology 249, 112373. doi:10.1016/j.jep.2019.112373
Han, Y., Chen, C., Li, J., and Yan, Z. (2019). Effects of Tea Tree Oil Treatment on Grey Mold Disease in Postharvest Strawberry Fruit. J. Xinjiang Agric. Univ. 42 (4), 249–260. doi:10.16021/j.cnki.1007-8622.2011.06.013
Han, Y., Zhang, Y., Liu, X., Tan, H., Du, X., and Yang, Y. (2011). Helicobacter pylori Eradication with Extractive of 20 Species of Medicinal Plants. Med. J. Natl. Defending Forces Northwest China 32 (6), 413–415.
Hasi, G., Ai, Q., Wei, Y., Wu, Z., and Zhang, X. (2009). Antimicrobial Activity of Chinese Herbal Medicine Extracts against Two Postharvest Pathogenic Fungi from Vegetables. J. Beijing Agric. Coll. 24 (01), 20–23. doi:10.13473/j.cnki.issn.1002-3186.2009.01.009
He, Y., Hu, H., Fu, C., Huo, Y., Zhang, J., and Xu, L. (2011). Mechanism of Acetic Ether from Vlaimiria Souliei on Pyloric-Ligation Ulcet Model. J. Chengdu Univ. TCM 34 (3), 72–74.
He, Y., Zhan, R., and Zhao, Y. (2006). Inhibtion Effects of Syzygium Aromaticum (L.) Extracts against Colletotrichum Gloeosporioides and Fusarium Oxysporum f.sp.Cubense. J. Sichuan Agric. Univ. 24 (4), 394–397+404.
He, Z., Fu, D., Wang, H., Yang, S., and Tan, W. (2009). Study on Microwave Extraction of Costunolide and Dehydrocostuslactone from Radix Valdimiriae. Appl. Chem. Industry 38 (6), 878–879.
He, Z., Li, Y., Fu, D., Zhou, S., and Tan, W. (2010). Study on Ultrasonic Power Extraction of Costunolide and Dehydrocostuslactone from Radix Valdimiriae. J. Anhui Agric. Sci. 38 (35), 20040–20042.
Hu, M., Chen, G., and Du, G. (2009). Inhibition of 20 Kinds of Chinese Herbs Extracts against a Rot-Causing Fungus in Postharvest Citrus Fruits. Tianjin Agric. Sci. 15 (2), 56–58.
Jin, L., Yin, H., Wan, P., Huang, W., Xu, D., and Huang, N. (2019). Antimicrobial Activity of 25 Kinds of Chinese Herbal Extract against Verticillium Dahlia. Plant Prot. 45 (2), 234–237+246.
Ju, X. (2011). Connotation and Denotation of Chemical and Biological Pesticides. Agrochemicals 50 (2), 153–154. doi:10.16820/j.cnki.1006-0413.2011.02.024
Kaur, R., Chahal, K. K., and Bhardwaj, U. (2017). Sesquiterpenolides from Inula Racemosa and Their Chemical Transformations. Asian J. Chem. 29 (3), 568–570. doi:10.14233/ajchem.2017.20254
Lai, X., Meng, B., Jang, Z., Song, Y., and Wu, Q. (2008). Effects of Radix Vladimiriae on Experimental Gastric Ulcer in Rats. Prog. Mod. Biomeicine 8 (1), 34–36. doi:10.13241/j.cnki.pmb.2008.01.019
Li, L., Ji, M., and Su, Z. (2006). Research Advances in Use of the Agricultural Fungicide. Sophora flavescensAgrochemicals 45 (9), 581–583. doi:10.1016/j.meatsci.2005.08.008
Liu, J., Xue, G., Wang, X., Song, M., Wang, L., and Fan, H. (2011). Study on the Antibacterial Activity of Ten Ultrasound Extracts from Medicinal Plants. Hubei Agric. Sci. 50 (9), 1809–1811. doi:10.14088/j.cnki.issn0439-8114.2011.09.034
Liu, N., Wu, X., Gu, B., and Zhu, Q. (2000). GB/T 17980-22-2000 Pesticide-Guidelines for the Field Eficacy Trials (1)-Fungicides against Cereal Powdery Mildew. Bejjing: Standards Press of China, 90–93p.
Mao, J., Wang, G., Yi, M., Huang, Y., and Chen, M. (2017). Research Progress on Chemical Constituents in Vladimiriae Radix and Their Pharmacological Activities. Chin. Traditional Herbal Drugs 48 (22), 4797–4803.
Neves, M., Morais, R., Gafner, S., Stoeckli-Evans, H., and Hostettmann, K. (1999). New Sesquiterpene Lactones from the Portuguese Liverwort Targionia Lorbeeriana. Phytochemistry 50 (6), 967–972. doi:10.1016/s0031-9422(98)00648-7
O’Shea, S., Lucey, B., and Cotter, L. (2009). In Vitro activity of Inula Helenium against Clinical Staphylococcus aureus Strains Including MRSA. Br. J. Biomed. Sci. 66 (4), 186–189. doi:10.1080/09674845.2009.11730271
Qian, W., Xu, Y., Wang, C., Mu, X., Zhang, Q., and Zhang, Z. (20121874). Researching Progress of Aucklandiae Radix about its Chemical Compositions and Structural Modification. Nat. Product. Res. Develop. 24 (12), 1857–1865. doi:10.16333/j.1001-6880.2012.12.034
Shum, K. C., Chen, F., Li, S. L., Wang, J., But, P. P., and Shaw, P. C. (2015). Authentication of Radix Aucklandiae and its Substitutes by GC-MS and Hierarchical Clustering Analysis. J. Sep. Sci. 30 (18), 3233–3239. doi:10.1002/jssc.200700232
Sun, W., Zhang, X., Huang, Z., Chen, T., Kang, C., Gao, J., et al. (2015). The Protective Activity of Dehydrocostus Lactone on Liver Injury in Laboratory Rats. World Chin. Med. 10 (3), 399–401. doi:10.3969/j.issn.1673-7202.2015.03.025
Tian, L., Peng, R., Yao, S., Deng, L., and Zeng, K. (2019). Pichia Membranaefaciens Combined with Chitosan Treatment Induces Resistance to Anthracnose in Citrus Fruit. Food Sci. 40 (7), 228–237. doi:10.7506/spkx1002-6630-20180913-120
Wahab, S., Lal, B., Jacob, Z., Pandey, V. C., and Srivastava, O. P. (1979). Studies on a Strain of Fusarium Solani (Mart.) Sacc. Isolated from a Case of Mycotic Keratitis. Mycopathologia 68 (1), 31–38. doi:10.1007/bf00490388
Wang, L., Liu, J., Wang, D., Li, J., and Fan, H. (2012). Inhibition Effect of Four Plants Compound Fungicides against Fusarium Oxysporum. China Vegetables 1 (16), 80–85.
Wang, L., Zhao, F., He, E., Wang, S., Xu, B., and Liu, K. (2008). Effects of Eighteen Sesquiterpenes from Saussurea Lappa on the Proliferation of Six Human Cancer Cell Lines. Nat. Prod. Res. Deo 20 (5), 808–812. doi:10.16333/j.1001-6880.2008.05.022
Wang, M., Shen, H., and Zhou, X. (2014). Laboratory Guide for Chemical Protection of Plant. Beijing: Peking University Press, 113–115p.
Wedge, D. E., Galindo, J. C. G., and Macı́as, F. A. (2000). Fungicidal Activity of Natural and Synthetic Sesquiterpene Lactone Analogs. Phytochemistry 53 (7), 747–757. doi:10.1016/s0031-9422(00)00008-x
Wei Ming, C., Mayer, R., Zimmermann, H., and Rücker, G. (1989). A Non-oxidized Melampolide and Other Germacranolides from Aristolochia Yunnanensis. Phytochemistry 28 (11), 3233–3234. doi:10.1016/0031-9422(89)80315-2
Xie, D., Cai, X., Yang, C., Xie, L., Qin, G., Zhang, M., et al. (2021). Studies on the Control Effect of Bacillus Subtilis on Wheat Powdery Mildew. Pest Manag. Sci. 77 (10), 4375–4382. doi:10.1002/ps.6471
Yang, X., Wang, M., Shen, R., and Liu, F. (2012). Microtiter Method to Test the Sensitivity of Botrytis Cinerea to Fungicides. Scientia Agricultura Sinica 45 (15), 3075–3082. doi:10.3864/j.issn.0578-1752.2012.15.008
Yu, H.-H., Lee, J.-S., Lee, K.-H., Kim, K.-Y., and You, Y.-O. (2007). Saussurea Lappa Inhibits the Growth, Acid Production, Adhesion, and Water-Insoluble Glucan Synthesis of Streptococcus Mutans. J. Ethnopharmacology 111 (2), 413–417. doi:10.1016/j.jep.2006.12.008
Yuuya, S., Hagiwara, H., Suzuki, T., Ando, M., Yamada, A., Suda, K., et al. (1999). Guaianolides as Immunomodulators. Synthesis and Biological Activities of Dehydrocostus Lactone, Mokko Lactone, Eremanthin, and Their Derivatives. J. Nat. Prod. 62 (1), 22–30. doi:10.1021/np980092u
Zhang, T., and Wang, G. (2011). Research Advances in Extraction of Antimicrobial Activity Components from the Plant Materials. Guangdong Agric. Sci. 38 (13), 59–62. doi:10.16768/j.issn.1004-874x.2011.13.006
Keywords: Radix Aucklandiae, antimicrobial activity, alantolactone, dehydrocostus lactone, costunolide
Citation: Cai X, Yang C, Qin G, Zhang M, Bi Y, Qiu X, Lu L and Chen H (2022) Antimicrobial Effects and Active Compounds of the Root of Aucklandia Lappa Decne (Radix Aucklandiae). Front. Chem. 10:872480. doi: 10.3389/fchem.2022.872480
Received: 17 February 2022; Accepted: 03 March 2022;
Published: 06 April 2022.
Edited by:
Pei Li, Kaili University, ChinaReviewed by:
Zhaonong Hu, Northwest A&F University, ChinaHua Fang, Zhejiang University, China
Wenwen Peng, Jiangxi Agricultural University, China
Copyright © 2022 Cai, Yang, Qin, Zhang, Bi, Qiu, Lu and Chen. This is an open-access article distributed under the terms of the Creative Commons Attribution License (CC BY). The use, distribution or reproduction in other forums is permitted, provided the original author(s) and the copyright owner(s) are credited and that the original publication in this journal is cited, in accordance with accepted academic practice. No use, distribution or reproduction is permitted which does not comply with these terms.
*Correspondence: Huabao Chen, Y2hlbmh1YWJhbzEyQDE2My5jb20=
†These authors have contributed equally to this work and share first authorship