- Laboratory of Materials Membranes and Environment, Faculty of Sciences and Technologies, University Hassan II Casablanca, Mohammedia, Morocco
A novel electrochemical sensor based on conducting polymer and multi-walled carbon nanotubes was reported for the detection of nitrite ions (NO2−). The hybrid material poly 1,8-Diaminonaphthalene (poly 1,8-DAN)/functionalized multi-walled carbon nanotubes (f-MWCNT) was prepared by using a simple electrochemical approach which is based on the deposition of functionalized multi-walled carbon nanotubes (f-MWCNT) on the surface of the electrode followed by the electropolymerization of 1,8-DAN using cyclic voltammetry. The morphology and the electro-catalytic properties of the obtained electrodes were investigated with Fourier Transform Infrared Spectroscopy (FTIR), Transmission Electron Microscopy (TEM), Cyclic Voltammetry (CV), and Electrochemical Impedance Spectroscopy (EIS) showing an improvement of the electronic transfer due to the synergic effect between the proprieties of poly 1,8-DAN and f-MWCNT. Under the optimum experimental conditions, the poly 1,8-DAN/f-MWCNT/CPE exhibited excellent electro-catalytic activity towards nitrite detection. The nitrite anodic peak potential decreased by 210 mV compared to the bare carbon paste electrode. The calibration plot of nitrite detection was linear in the range of concentration from 300 to 6500 nM with a low detection limit of 75 nM.
Introduction
Nitrite ions; one of the inorganic forms of natural nitrogen; take part of the cycle in which nitrogen takes different forms following several processes such as fixation, assimilation, nitrification, and denitrification (Zhu et al., 2015). It is important to highlight that the industrialization, overpopulation, and the progress of the agri-food sector have led to the accumulation of nitrite in our environment in a very significant way (Berardo et al., 2016). Indeed, it is generated during the decomposition of fertilizers and human waste leading to the contamination of the soil and the groundwater. Nitrite is also used as food preservatives and food coloring under the form of sodium nitrite noted (E250) and constitutes a real threat to the human health. In fact, it has been reported that an excess of this element in the body leads to the conversion of the hemoglobin to methemoglobin, which reduce the amount of oxygen distributed by the blood to the tissues called hypoxia (Katabami et al., 2016). Nitrite ions can also react with amines to form N-nitroamines causing serious health problems such as gastric cancer (Song et al., 2015; Zhang et al., 2019), thyroid cancer (Aschebrook-Kilfoy et al., 2013), and non-Hodgkin's lymphoma (Yu et al., 2020). Moreover, research studies have confirmed a possible relationship between nitrite consumption and neurological damages (Ribeiro et al., 2017). Therefore, there is an urgent need to monitor the presence of nitrite in water and food. For this purpose, the World Health Organization (WHO) has determined a maximum admissible concentration in drinking water of 3 mg/L equivalent to 65.217 µM (Ren et al., 2018). Numerous methods have been developed to detect and quantify nitrite (Wang et al., 2017), such as spectrofluorimetry (Zhan et al., 2019), spectrophotometry (Lo et al., 2017), chemiluminescence (Han and Chen, 2019), chromatography (Lin et al., 2019), capillary electrophoresis (Kalaycıoğlu and Erim, 2016), and electrochemistry (Zhao et al., 2017). The electrochemical techniques possess several advantages such as low cost, rapid response and high sensitivity (Bansod et al., 2017; Oularbi et al., 2019). The electro-oxidation of nitrite leads to the formation of nitrate, unlike its electro-reduction, which can produce several products, and it is limited by the interference of dioxygen (O2) and nitrate reduction (NO3−) (Kesavan et al., 2018). However, the oxidation of nitrite still faces many challenges to overcome, such as the oxidation overpotential, and the slow kinetic of the electronic transfer (Mao et al., 2018). To address this issues, electrochemical sensors and biosensors seem to be a very good alternative. It is, in fact, absolutely necessary to modify the conventional electrodes such as carbon paste (Bijad et al., 2017), screen-printed electrodes (Jaiswal et al., 2017), and glassy carbon electrodes with a suitable agent (Muthumariappan et al., 2017; Velmurugan et al., 2020; Karimi-Maleh et al., 2021). Electrochemical Multi-walled carbon nanotubes (MWCNT), largely used to modify the surface of electrodes for many applications (Mani et al., 2017; Beitollahi et al., 2018; Roy et al., 2018; Rui et al., 2020; Shaikshavali et al., 2020; Baghayeri et al., 2021a); are considered as an ideal choice thanks to their interesting properties. Moreover, they can be combined with metallic nanoparticles, metal organic framework or conducting polymers in order to enhance the sensitivity towards nitrite determination in different matrices (Thirumalraj et al., 2016; Wan et al., 2017; Yu et al., 2019; Baghayeri et al., 2020a; Salagare et al., 2020). Conducting polymers (CPs) constitute a new class of emerging materials and have shown considerable potential for several applications (Gracia and Mecerreyes, 2013; Elbasri and Rhazi, 2015; Salih et al., 2017b; El Rhazi et al., 2018; Salih et al., 2018; Chemchoub et al., 2019; Chemchoub et al., 2020; El Attar et al., 2020; Vagin et al., 2021). They have also shown excellent electrocatalytic activity towards nitrite detection. Recently, several hybrid materials based on CPs have been developed for nitrite sensing (Salhi et al., 2021). Rajalakshmi and John (2015), developed a composite based on 5-amino-1,3,4-thiadiazole-2-thiol and MWCNT for nitrite detection and obtained a very low detection limit of about 0.2 nM. A novel electrochemical approach was also reported in the literature which consists on the combination of poly (3,4-ethylenedioxythiophene) with gold nanoparticles leading to a very good activity toward nitrite oxidation with high stability and selectivity (Lin et al., 2016). Moreover, Shi et al., co-deposited palladium nanoparticles and poly (1.5-Diaminonaphthalene) on MWCNT. The prepared hybrid showed a good electrocatalytic activity towards nitrite oxidation due to its high active surface area and the synergistic effect of Pd nanoparticles, poly 1.5-DAN and MWCNT (Shi et al., 2019). Nevertheless, the main disadvantage of the sensors based on gold and palladium nanoparticles lies in the fact that they are quite expensive.
In this context, we propose a very simple approach which consists on the combination of the two materials (CP and MWCNT) in order to develop an electrochemical sensor; efficient and less expensive; for nitrite determination in water samples. The electropolymerization of 1,8-DAN on carbon nanotubes was carried out using cyclic voltammetry in acidic medium, which allowed us to prepare our working electrodes in less than 1 hour. The morphology and the electrochemical behavior of the modified electrodes were investigated using various techniques such as Fourier transform infrared spectroscopy (FTIR), transmission electron microscopy (TEM), cyclic voltammetry (CV), and electrochemical impedance spectroscopy (EIS). The electrocatalytic activity of poly 1,8-DAN/f-MWCNT/CPE towards nitrite oxidation was examined using both cyclic voltammetry and amperometry.
Experimental
Chemical and Reagents
Graphite powder, paraffin oil, 1,8-Diaminonaphthalene (1,8-DAN), potassium Ferri/Ferrocyanide (K3Fe(CN)6/K4Fe(CN)6.3H2O; ACS reagent >99%), disodium hydrogen phosphate heptahydrate (Na2HPO4.7H2O) and sodium dihydrogen phosphate dihydrate (NaH2PO4.2H2O) were obtained from Sigma Aldrich. Potassium chloride (KCl), hydrochloric acid (HCl, 37%), nitric acid (HNO3, 69%), and sulfuric acid (H2SO4, 98%) were procured from Labo Chimie. Sodium nitrite (NaNO2, ACS reagent >98%) was acquired from Panreac Quimica. Bi-distillated water was used to prepare all the solutions including 0.1 M of phosphate buffer solution (PBS, pH 7.2), which was used as the electrolyte.
Functionalization of Multi Walled Carbon Nanotubes
In order to functionalize multi-walled carbon nanotubes, an appropriate amount of MWCNT was dispersed in a solution of concentred H2SO4 and HNO3, with a volume ratio of 1:3. The suspension was ultrasonicated for a few minutes, then left under magnetic stirring over-night. Afterwards, the functionalized multi walled carbon nanotubes (f-MWCNT) were filtered using a Millipore polycarbonate membrane with a diameter of 0.22 µm, and washed with bi-distillated water until a neutral pH of the filtrate was obtained. Eventually, the f-MWCNT were dried at the oven at 50°C for 5 h.
Preparation of the Modified Electrodes
Firstly, the carbon paste electrode (CPE) was prepared by mixing 1 g of graphite powder with 30% paraffin oil w/w in a mortar until the formation of a homogeneous paste (Salih et al., 2017a; Oularbi et al., 2020; El Attar et al., 2021). Subsequently, the paste was placed in a 3 mm diameter Teflon tube. Then, it was polished until the obtention of a smooth surface. The aqueous suspension of f-MWCNT was prepared by dispersing 1 mg of f-MWCNT in 1 ml of distillated water. Afterward, 20 µL of the suspension was dropped onto the surface of CPE, then dried at the oven at 50°C.
The electrochemical deposition of poly (1,8-DAN) was carried out in a 0.1 M HCl solution containing 5 mM of the monomer (1,8-DAN) using cyclic voltammetry (CV) by sweeping the potential from −0.5 to 1 V vs. Ag/AgCl for 15 cycles at a scan rate of 50 mV/s.
Electrochemical Measurements and Physicochemical Characterization
All the electrochemical measurements including cyclic voltammetry (CV), electrochemical impedance spectroscopy (EIS), and amperometry were performed using a PalmSens4 connected to a PSTrace 5.3 software. A traditional three electrodes system was used, which consists of a modified carbon paste electrode (CPE) as the working electrode, a platinum disk as the counter electrode, and a silver/silver chloride (Ag/AgCl) as the reference electrode.
The CV measurements were carried out in a 0.5 M KCl solution containing 10 mM [Fe(CN)6]3−/4− at an applied potential ranging from −0.4 to 0.8 V vs. Ag/AgCl, and in 0.1 M phosphate buffer (PBS, pH 7.2) containing 0.1 M KCl and 1 mM NO2−, sweeping the potential from −0.2 to 1.1 V vs. Ag/AgCl at different scan rates. Electrochemical impedance spectroscopy (EIS) measurements were performed in 0.1 M PBS (pH 7.2) containing 0.1 M KCl and 1 mM NO2− at a fixed potential of 0.85 V vs. Ag/AgCl, a frequency range from 10 mHz to 63 kHz and an amplitude of 10 mV. The amperometry measurements were conducted in 0.1 M PBS containing 0.1 M KCl at a fixed potential of 0.9 V vs. Ag/AgCl under stirring. It should be noted that all the experiments were performed at room temperature.
Fourier Transform Infrared spectroscopy (FTIR) using an Affinity-1S SHIMADZU spectrometer equipped with a golden gate single reflection attenuated total reflectance (ATR) accessory. FTIR spectra were recorded in the range of 500–4000 cm⁻1 at a resolution of 16 cm⁻1 and were used to determine the structural properties of f-MWCNT and poly 1,8-DAN/f-MWCNT. The morphological properties of the electrocatalysts were characterized using Transmission Electron Microscopy-EDX (TALOS F200S).
Results and Discussions
Polymerization of 1,8-DAN
In order to polymerize 1,8-Diaminonaphthalene onto the surface of CPE and f-MWCNT/CPE, cyclic voltammetry technique was performed in a 0.1 M HCl solution containing 5 mM of the monomer (1,8-DAN) by sweeping the potential from −0.5 to 1 V vs. Ag/AgCl at a scan rate of 50 mV/s for 15 scans. The formation of a brown film on each electrode was observed indicating the formation of poly 1,8-DAN (Oyama et al., 1989). The cyclic voltammograms obtained at CPE and f-MWCNT/CPE were illustrated in Figure 1. The bare electrode (Figure 1A) shows a peak current at +0.5 V vs. Ag/AgCl corresponding to the irreversible electrooxidation of 1,8-DAN which disappears completely after the sixth scan giving rise to new oxidation-reduction peaks which appear at about +0.05 and +0.2 V vs. Ag/AgCl. This is consistent with the previous results found by Majid et al. (2003). However, at the f-MWCNT/CPE as it is shown in Figure 1B, during the first scan, a peak current was observed at + 0.4 V vs. Ag/AgCl which corresponds to the irreversible oxidation of the monomer. The shift of about 100 mV observed on CPE modified by f-MWCNT can be attributed to the 3-dimensional network of f-MWCNT which increases the electrode surface area. It should be noted that a second peak was observed at 0.7 which may correspond to the second oxidation of the amine group. These results are in agreement with the work of Lee et al. (1992), when using platinum electrode for the polymerization of 1,8-DAN. It is important to highlight that after the modification of the electrode with f-MWCNT, the current intensities increased by about 76%. This may be attributed to the higher and good electrical conductivity of f-MWCNT and/or to the 3-dimensional network and large surface area of the f-MWCNT (Guo et al., 2020).
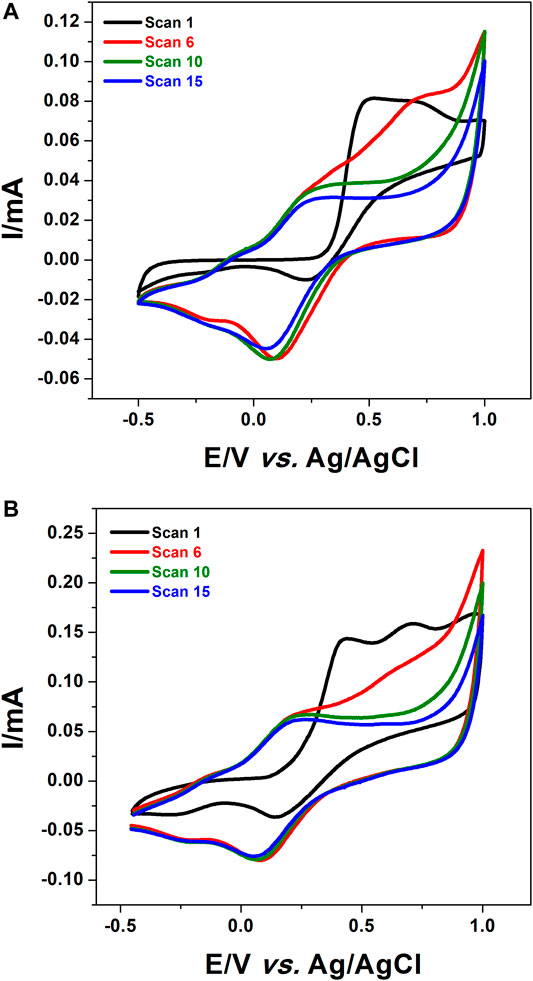
FIGURE 1. CV of (A) CPE and (B) f-MWCNT/CPE in 0.1 M HCl containing 5 mM 1,8-DAN for 15 cycles at a scan rate of 50 mV/s.
In order to check the formation of a stable polymer, the prepared electrode was then placed in a 0.1 M HCl solution free monomer and CV was performed under the potential range of −0.5–1 V at a scan rate of 50 mV/s for 5 cycles. Figure 2 shows the results obtained on poly 1,8-DAN/f-MWCNT/CPE. It can be seen that the voltammograms kept the same shape after consecutive scans revealing the oxidation/reduction peaks of poly 1,8-DAN in acidic medium, which indicates that a stable film of poly 1,8-Diaminonapththalene was synthesized on f-MWCNT/CPE (Salih et al., 2017b).
FTIR and TEM Characterization of Poly 1,8-DAN/f-MWCNT
FTIR spectroscopy was used to confirm the functionalization of MWCNT with carboxylic groups, and also to confirm the deposition of poly 1,8-DAN film (Figure 3A). The FTIR of f-MWCNT (red line) showed two absorption bands at 2334 and 2361 cm−1, which correspond to the hydrogen of the carboxylic group (-COOH). In addition to this, two other bands were noticed at 976 and 1741 cm−1, which are attributed respectively to the C=O and C-O of the -COOH. Another band was observed at 3600 cm−1 indicating the presence of the hydroxylic group -OH of -COOH (Oularbi et al., 2017; Oularbi, 2018; El Attar et al., 2022). These results demonstrated that the MWCNT were successively functionalized. The structural properties of poly 1,8-DAN/f-MWCNT have also been investigated using FTIR spectroscopy (blue line). It can be seen that new peaks appeared after the electrodeposition of the polymer. In fact, the absorption band located in 3309 cm−1 corresponds to the N-H stretching. Whereas, the peaks that appeared at 1490 and 1581 cm−1 are attributed to the C-C of the polymer. Furthermore, the band of 1203 indicates the C-N stretching (Pałys et al., 1997). These results confirm the formation of poly 1,8-DAN on the surface of f-MWCNT/CPE. The morphologies of f-MWCNT/CPE and poly 1,8-DAN/f-MWCNT/CPE were examined using TEM (Figures 3B,C). The corresponding images (Figure 3B) reveal a slight increase of the carbon nanotubes diameter after the electrochemical deposition of poly 1,8-DAN.
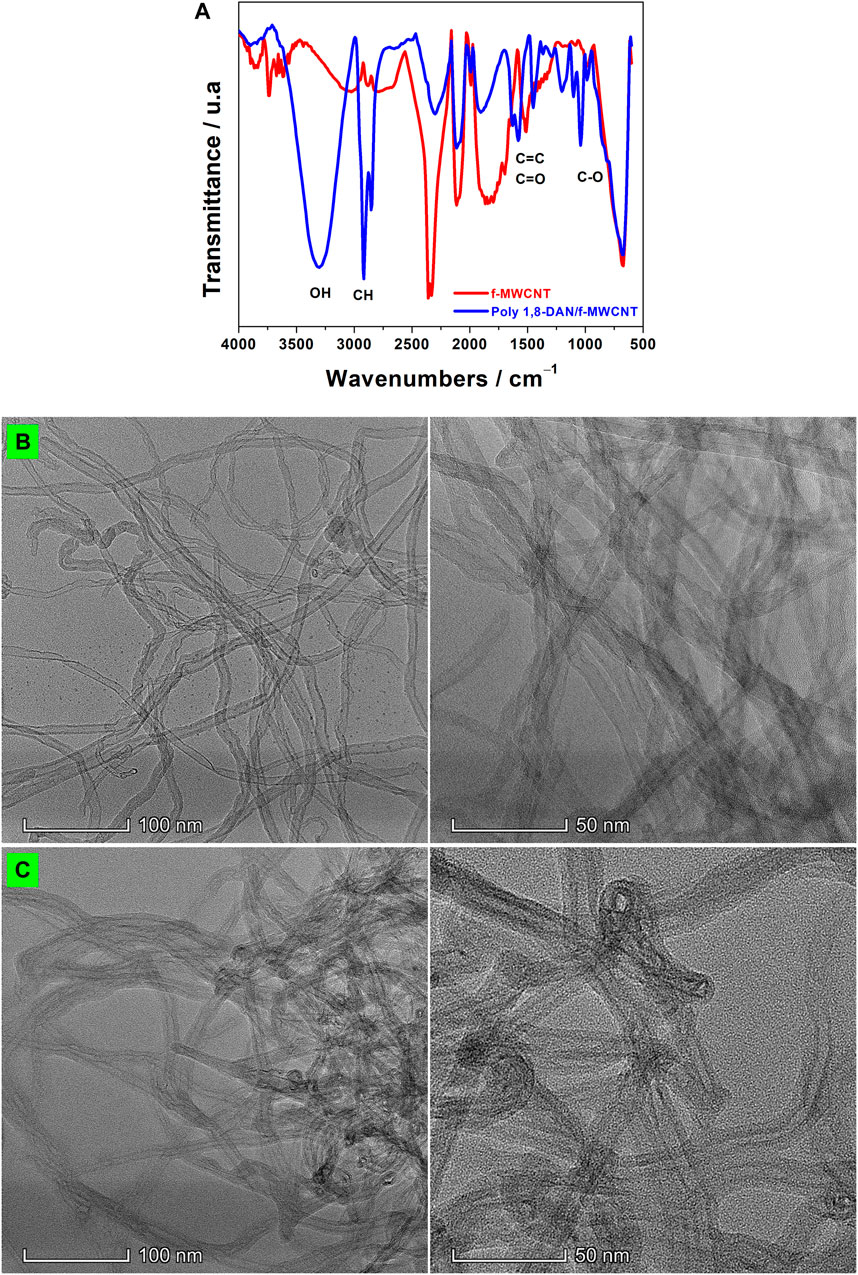
FIGURE 3. (A) FTIR spectra of f-MWCNT (red line), and poly 1,8-DAN/f-MWCNT (blue line). TEM images of (B) f-MWCNT, and (C) poly 1,8-DAN/f-MWCNT.
The thickness of the polymeric film (d) was also determined using Eq. 1 described as follow (Halim et al., 2019):
Where Mw is the molar mass of the monomer (158.2 g/mol), Q is the electrical charge associated to the polymer formation, n is the number of the involved electrons (2) (Lee et al., 1992), F is the Faraday (96485 Q/mol), A is the surface area of the working electrode, and φ is the monomer density (1.12 g/cm3). The thickness of poly 1,8-DAN film deposited on the surface of f-MWCNT/CPE was found to be 22 nm, which indicates the formation of a thin film on the surface. This thickness is in agreement with the results found using the transmission electron microscopy.
Electrochemical Characterization of Poly 1,8-DAN/f-MWCNT
Cyclic Voltammetry Characterization in [Fe(CN)6]3-/4-
The preliminary investigations were aimed towards comparing the electrochemical responses of ferri-ferrocyanide system on different electrodes. In this perspective, CV measurements were performed in 0.5 M KCl containing 10 mM [Fe(CN)6]3−/4− at CPE, f-MWCNT/CPE, poly 1,8-DAN/f-MWCNT/CPE in the potential range from −0.4–0.8 V vs. Ag/AgCl. As can be seen in Figure 4 and Table 1, the peak-to-peak separation on CPE (black line) is equal to 223 mV, which indicate a slow electronic transfer. On the f-MWCNT/CPE, the peak-to-peak separation was reduced to reach the value of 98 mV (red line) and the current intensities increased by about 40%. This behavior could be explained by the large specific surface area and high electrical conductivity offered by f-MWCNT. The same behavior was observed by Oularbi et al. (2017), when using Polypyrrole and Carbon nanofibers. A surprising fact was observed after the electrodeposition of poly 1,8-DAN on f-MWCNT/CPE (blue line). A dramatic decrease of the value of ΔEp was found, around 91 mV, which is about more than 3 times smaller than the bare CPE. The only possible explanation is that the decrease in ΔEp is probably due to the improvement of the electronic transfer at the interface electrode-solution due to the presence of a large amount of amine groups all along the polymer backbone (Wang et al., 2016). Indeed, It has been proved that an amine-rich surface enhances the electronic transfer (Li et al., 2017; Ning et al., 2019). Contrary to what one might expect to find, a low current compared to CPE and f-MWCNT/CPE was obtained due to the low conductivity of poly 1,8-DAN at neutral pH (Majid et al., 2003).
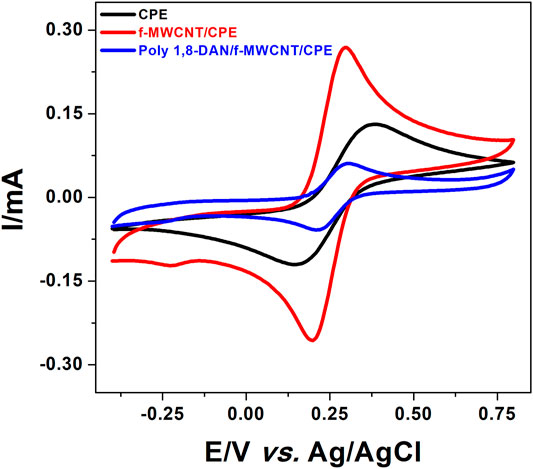
FIGURE 4. CV of CPE (black line), f-MWCNT/CPE (red line), and poly 1,8-DAN/f-MWCNT/CPE (blue line) in 0.5 M of KCl containing 10 mM of [Fe(CN)6]3−/4− at a scan rate of 50 mV/s.
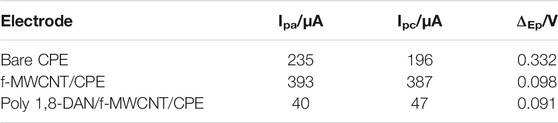
TABLE 1. Comparison between Ipa, Ipc and the peak-to-peak separation (ΔEp) for CPE, f-MWCNT/CPE and 1,8-DAN/f-MWCNT/CPE.
The specific surface area of all the electrodes was calculated using the Randles and Sevcik Eq. 2 (Analytical Electrochemistry, Wang, 2006) as shown in Figure 5:
Where, the number of electrons transferred (n) is equal to 1, the concentration (C) is equal to 10 mM and the diffusion coefficient (D) is equal to 6.7 10–6 cm2/s (Halim et al., 2019). The specific surface areas were found to be 0.073, 0.162, and 0.033 cm2, respectively for CPE, f-MWCNT/CPE, and poly 1,8-DAN/f-MWCNT/CPE. Indeed, the specific surface of f-MWCNT/CPE was about two times higher than the bare CPE. This was due to the large specific surface of multi-walled carbon nanotubes (Shi et al., 2019). It is important to mention that the electrode modified with poly 1,8-DAN and f-MWCNT has the smallest surface area. This phenomena can be explained by the low electrical conductivity of polymer, and the lack of active sites of the poly (1,8-DAN) at neutral pH (Majid et al., 2003).
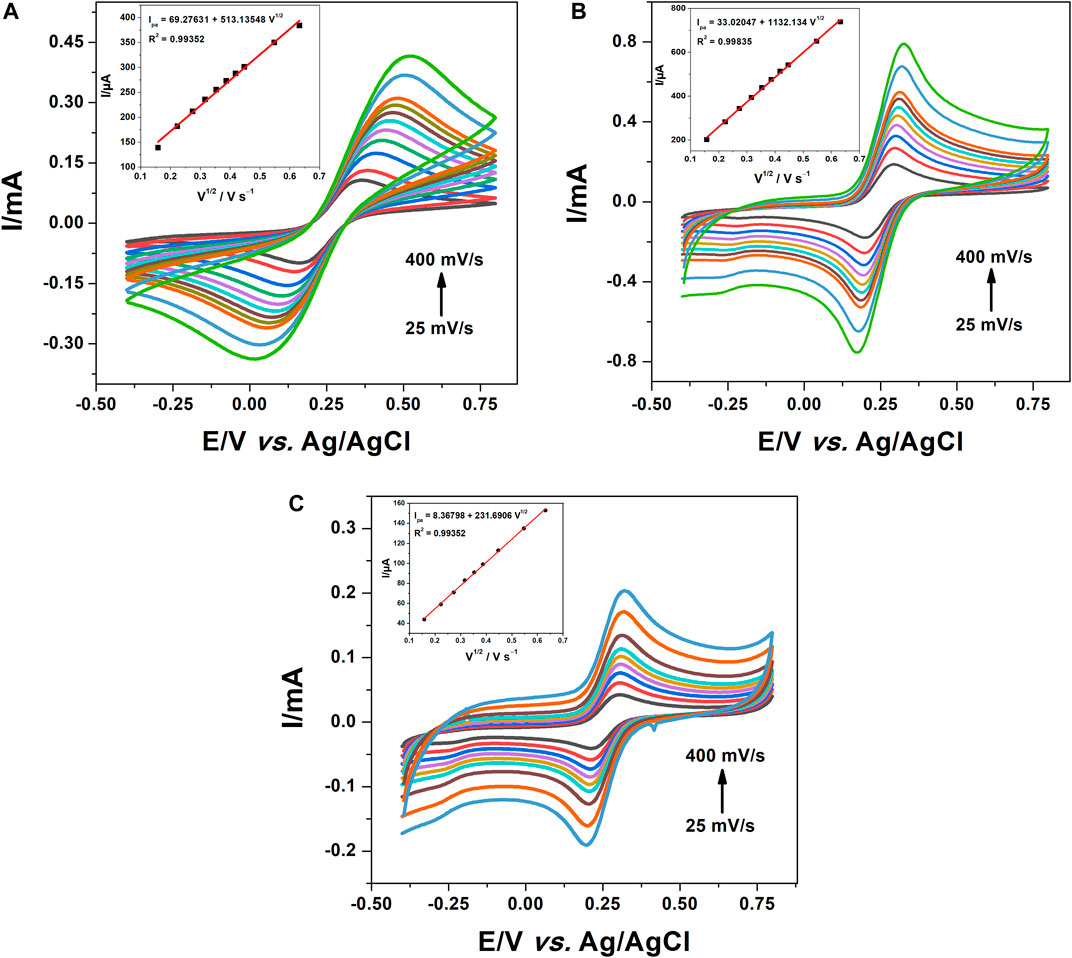
FIGURE 5. CV of (A) CPE, (B) f-MWCNT/CPE, and (C) poly 1,8-DAN/f-MWCNT/CPE in 0.5 M of KCl containing 10 mM of [Fe(CN)6] 3−/4− at different scan rates.
Electrooxidation Behavior of Nitrite on the Poly 1,8-DAN/f-MWCNT
After the characterization of our electrodes, the behavior of the different electrodes in presence of nitrite was investigated using CV in a pH 7.2, 0.1 M phosphate buffer solution containing 0.1 M KCl and 1 mM nitrite. Figure 6 presents the corresponding voltammograms at the bare CPE, f-MWCNT/CPE, and poly 1,8-DAN/f-MWCNT/CPE. An irreversible peak at 1.04 V vs. Ag/AgCl (black line) was obtained at bare carbon paste electrode which corresponds to the irreversible oxidation of nitrite. As expected, the peak potential was shifted to more negative value (210 mV) after the modification of CPE with f-MWCNT (red line) with an important increase in the current intensity. After the electrodeposition of poly 1,8-DAN on the surface of f-MWCNT/CPE (blue line). The same results were observed concerning the peak potential with a further increase of the current intensity, indicating that nitrite is much easier to oxidase on the hybrid material. Similar results were obtained by Huang et al., using gold nanoparticles combined with poly (3-methylthiophene), which was explained by the synergistic effect of combining both gold nanoparticles and conducting polymers. It seems that the carbon nanomaterials combined with conducting polymers enhance the electronic transfer as observed by many authors (Zheng et al., 2009; Rajalakshmi and John, 2015, 2; Arulraj et al., 2018). To better understand this phenomenon, electrochemical impedance spectroscopy (EIS) was conducted in the same solution at an applied potential of 0.85 V vs. Ag/AgCl with a small amplitude of 10 mV. Figure 7 presents the corresponding Nyquist plots. Indeed, it can be seen that the charge transfer resistances (Rct) decreased from 3277 Ω to 11 Ω after the modification of CPE with poly 1,8-DAN/f-MWCNT, which is 300 times smaller. These results are in good agreement with the CV measurements and suggests that the prepared hybrid material promotes the electronic transfer between the supporting electrolyte and the electrode.
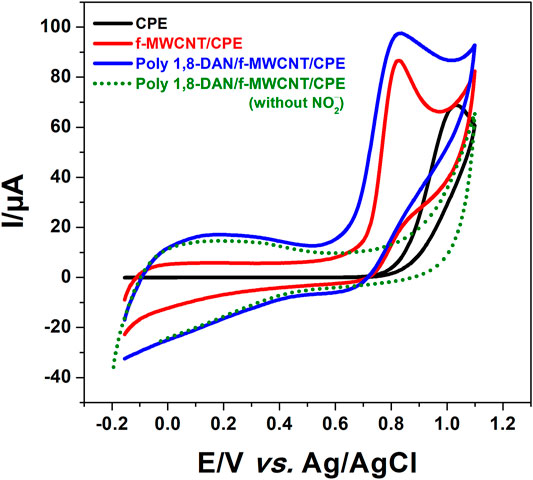
FIGURE 6. CV of CPE (balck line), f-MWCNT/CPE (red line), and poly 1,8-DAN/f-MWCNT/CPE in 0.1 M PBS containing 0.1 M KCl (green dots) and 1 mM NO2− (blue line) at pH 7, 2 and a scanning speed of 50 mV/s.
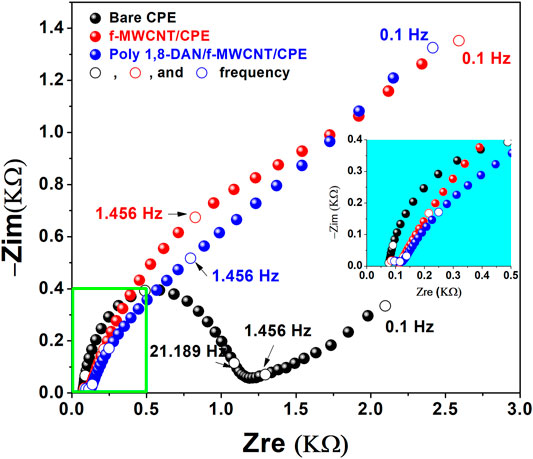
FIGURE 7. Nyquist plots of CPE (black line), f-MWCNT/CPE (red line), and poly 1,8-DAN/f-MWCNT/CPE (blue line) in 0.1 M PBS containing 0.1 M KCl and 1 mM NO2− at pH 7.2 and a fixed Potential of 0.85 V vs. Ag/AgCl.
Electrooxidation Mechanism of Nitrite on the Poly 1,8-DAN/f-MWCNT
In order to establish the reaction mechanism of nitrite oxidation, cyclic voltammetry experiments were recorded on poly 1,8-DAN/f-MWCNT/CPE. The corresponding graphs shown in Figure 8A present the variation of the oxidation current with the square root of the scan rate.
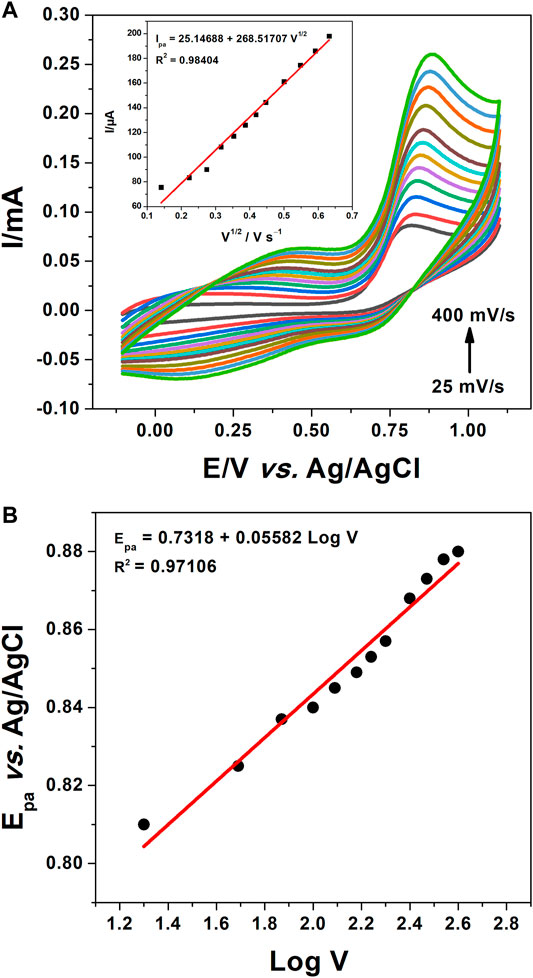
FIGURE 8. (A) CV of poly 1,8-DAN/f-MWCNT/CPE in 0.1 M PBS containing 0.1 M KCl and 1 mM NO2− at pH 7.2 and scanning rates from 25 to 400 mV/s with the plot of nitrite oxidation peak current versus V1/2, (B) the plot of the peak current versus Log(V).
It can be seen that the nitrite oxidation peak increased linearly with the square root of the scan rate according to the equation Ipa = 25.14688 + 268.51707 V1/2 with a correlation coefficient of 0.98404 which suggests that the electrochemical reaction is controlled by a diffusion process (Baghayeri et al., 2020b; Baghayeri et al., 2021b). It should be noted that a shift in the oxidation peak potential (Epa) to more positive values with the scan rates confirms the irreversibility of the nitrite oxidation reaction as mentioned by others authors (Zhao et al., 2017; Shi et al., 2019). The number of the transferred electrons can be calculated by plotting the Epa vs. Log V. Epa varied linearly with the logarithm of the scan rate as described by the equation Epa = 0.7318 + 0.05582 Log V with a correlation coefficient of 0.97106 (Figure 8B). The number of the transferred electrons during the oxidation reaction of nitrite was then calculated according to Laviron’s Eq. 3 (Analytical Electrochemistry, Wang, 2006):
Where n is the number of the transferred electrons, α is the electron transfer coefficient which is taken as 0.5 in a totally irreversible reaction (Shi et al., 2019), v is the scan rate, and E°’ is the formal potential. Whereas R, T and F have their conventional meaning. The number of electron n involved in the oxidation reaction of nitrite was calculated to be about 2 (2.08), which demonstrates that the electrooxidation of nitrite requires the transfer of two electrons according to the following reactions noted (4) and (5). These results are in agreement with the literature (Arulraj et al., 2018):
Calibration Curve and Real Sample Analysis
After the characterization of our sensor, and in order to evaluate the advantages offered by f-MWCNT and poly 1,8-DAN, amperometry was chosen as a sensitive technique to detect nitrite in aqueous solution (Liu et al., 2011; Rajalakshmi and John, 2015; Zhang et al., 2015; Fan et al., 2017). Indeed, amperometry was performed in 0.1 M PBS pH 7.2 containing 0.1 M KCl at a fixed potential of 0.9 V vs. Ag/AgCl under continuous stirring. The corresponding graph shown in Figure 9A illustrates the variation of the current intensities versus time after successive additions of nitrite. The oxidation current increased linearly with nitrite concentration in a range between 300 and 6500 nM according to equation I = −0.0537 + 0.44453 [NO2⁻] with a correlation coefficient of 0.99722, a relative standard deviation (RSD) of 2.36% (n = 3 tests), and a sensitivity of 0.44453 μA/μM. The detection limit was calculated by the equation LOD = 3 × SD/P. where SD is the standard deviation, and P the slope of the calibration curve, and it was found to be 75 nM.
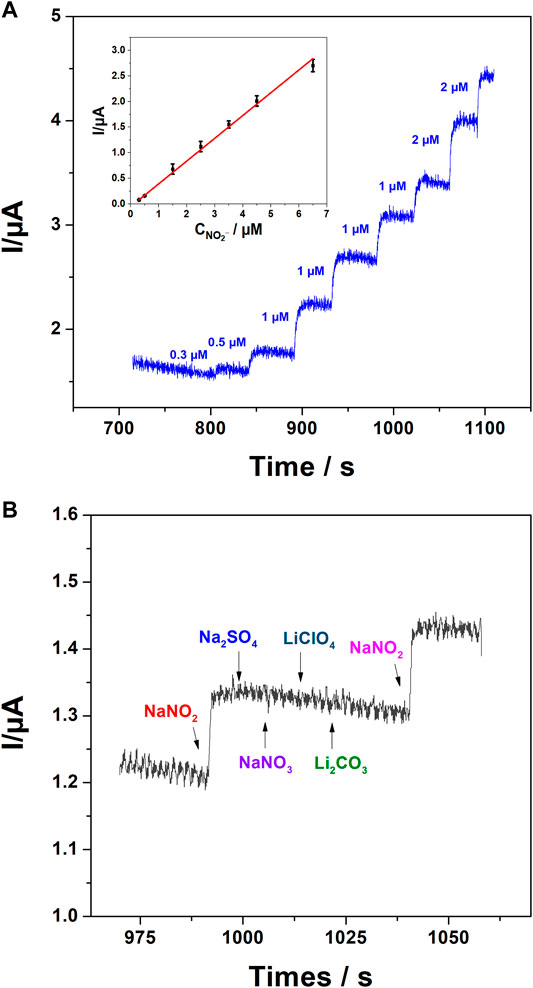
FIGURE 9. (A) Amperometry of poly 1,8-DAN/f-MWCNT/CPE in 0.1 M PBS containing 0.1 M KCl at pH 7.2 with a fixed potential of 0.9 V vs. Ag/AgCl at consecutive additions of nitrite, insert the calibration plot of the current versus nitrite concentrations. (B) Effect of some interference components on nitrite response.
Prior to the application in real samples, the selectivity of the developed sensor was investigated in the presence of some components such as NaNO3, Na2SO4, LiClO4, and Li2CO3. From the obtained results (Figure 9B), we can assume that these components did not interfere with the nitrite response. Subsequently, the effectiveness application of the developed hybrid sensor for detection of nitrite in real samples was tested in tap water. The determination of nitrite was based on the method of dosed additions, and the concentration of nitrite was found to be 0.28667 ± 0.01528 µM with an RSD of about 5.3% (3 tests) which suggests that our sensor can be used directly in real samples, and without any pretreatment of water. The detection limit, linearity range, and the detection technique of different hybrids reported in the literature are summarized in Table 2. Our material seems to be a good choice for nitrite determination in water samples.
Conclusion
In summary, a low-cost sensor based on 1,8-Diaminonaphthalene electrodeposited on f-MWCNT/CPE was developed for a rapid and effective determination of nitrite in aqueous solutions. The characterization of the electrode was performed using different techniques such as FTIR, TEM, CV and EIS. The FTIR and TEM characterizations confirm the presence of the polymer on the surface of our sensor, while the electrochemical measurements revealed that the combination of f-MWCNT and poly 1,8-DAN improves the electronic transfer, and has very good electro-catalytic activity towards nitrite oxidation. The sensor was then applied for detection of nitrite showing a good linearity, selectivity and sensitivity with a very low detection limit of 75 nM. It should be highlighted that the sensor can be used directly and without any pre-treatment of water samples. More studies are undergoing to enhance the linearity range and to extend the applicability of this sensor.
Data Availability Statement
The original contributions presented in the study are included in the article/Supplementary Material, further inquiries can be directed to the corresponding author.
Author Contributions
All authors listed have made a substantial, direct, and intellectual contribution to the work and approved it for publication.
Conflict of Interest
The authors declare that the research was conducted in the absence of any commercial or financial relationships that could be construed as a potential conflict of interest.
Publisher’s Note
All claims expressed in this article are solely those of the authors and do not necessarily represent those of their affiliated organizations, or those of the publisher, the editors and the reviewers. Any product that may be evaluated in this article, or claim that may be made by its manufacturer, is not guaranteed or endorsed by the publisher.
Acknowledgments
This work was supported by MESRSFC (Ministère de l’Enseignement Supérieur et de la Recherche Scientifique et de la Formation des cadres–Morocco) and CNRST (Centre National pour la Recherche Scientifique et Technique-Morocco) (Project number PPR/2015/72).
References
Afkhami, A., Soltani-Felehgari, F., Madrakian, T., and Ghaedi, H. (2014). Surface Decoration of Multi-Walled Carbon Nanotubes Modified Carbon Paste Electrode with Gold Nanoparticles for Electro-Oxidation and Sensitive Determination of Nitrite. Biosens. Bioelectron. 51, 379–385. doi:10.1016/j.bios.2013.07.056
Arulraj, A. D., Sundaram, E., Vasantha, V. S., and Neppolian, B. (2018). Polypyrrole with a Functionalized Multi-Walled Carbon Nanotube Hybrid Nanocomposite: A New and Efficient Nitrite Sensor. New J. Chem. 42, 3748–3757. doi:10.1039/C7NJ04130F
Aschebrook-Kilfoy, B., Shu, X.-O., Gao, Y.-T., Ji, B.-T., Yang, G., Li, H. L., et al. (2013). Thyroid Cancer Risk and Dietary Nitrate and Nitrite Intake in the Shanghai Women's Health Study. Int. J. Cancer 132, 897–904. doi:10.1002/ijc.27659
Baghayeri, M., Amiri, A., Karimabadi, F., Di Masi, S., Maleki, B., Adibian, F., et al. (2021a). Magnetic MWCNT-Dendrimer: A Potential Modifier for Electrochemical Evaluation of as (III) Ions in Real Water Samples. J. Electroanalytical Chem. 888, 115059. doi:10.1016/j.jelechem.2021.115059
Baghayeri, M., Ghanei-Motlagh, M., Tayebee, R., Fayazi, M., and Narenji, F. (2020a). Application of Graphene/zinc-Based Metal-Organic Framework Nanocomposite for Electrochemical Sensing of As(III) in Water Resources. Analytica Chim. Acta 1099, 60–67. doi:10.1016/j.aca.2019.11.045
Baghayeri, M., Nabavi, S., Hasheminejad, E., and Ebrahimi, V. (2021b). Introducing an Electrochemical Sensor Based on Two Layers of Ag Nanoparticles Decorated Graphene for Rapid Determination of Methadone in Human Blood Serum. Top. Catal. 1099, 1–10. doi:10.1007/s11244-021-01483-4
Baghayeri, M., Nodehi, M., Amiri, A., Amirzadeh, N., Behazin, R., and Iqbal, M. Z. (2020b). Electrode Designed with a Nanocomposite Film of CuO Honeycombs/Ag Nanoparticles Electrogenerated on a Magnetic Platform as an Amperometric Glucose Sensor. Analytica Chim. Acta 1111, 49–59. doi:10.1016/j.aca.2020.03.039
Bansod, B., Kumar, T., Thakur, R., Rana, S., and Singh, I. (2017). A Review on Various Electrochemical Techniques for Heavy Metal Ions Detection with Different Sensing Platforms. Biosens. Bioelectron. 94, 443–455. doi:10.1016/j.bios.2017.03.031
Beitollahi, H., Movahedifar, F., Tajik, S., and Jahani, S. (2018). A Review on the Effects of Introducing CNTs in the Modification Process of Electrochemical Sensors. Electroanalysis 31, 1195–1203. doi:10.1002/elan.201800370
Berardo, A., De Maere, H., Stavropoulou, D. A., Rysman, T., Leroy, F., and De Smet, S. (2016). Effect of Sodium Ascorbate and Sodium Nitrite on Protein and Lipid Oxidation in Dry Fermented Sausages. Meat Sci. 121, 359–364. doi:10.1016/j.meatsci.2016.07.003
Bijad, M., Karimi-Maleh, H., Farsi, M., and Shahidi, S.-A. (2017). Simultaneous Determination of Amaranth and Nitrite in Foodstuffs via Electrochemical Sensor Based on Carbon Paste Electrode Modified with CuO/SWCNTs and Room Temperature Ionic Liquid. Food Anal. Methods 10, 3773–3780. doi:10.1007/s12161-017-0933-z
Chemchoub, S., Elbasri, M., Halim, E. M., and El Rhazi, M. (2019). The Electrocatalytic Oxidation of Methanol on a Carbon Paste Electrode Modified by Poly(para-Phenylenediamine) and Nickel Particles. Mater. Today Proc. 13, 720–729. doi:10.1016/j.matpr.2019.04.033
Chemchoub, S., Oularbi, L., El Attar, A., Younssi, S. A., Bentiss, F., Jama, C., et al. (2020). Cost-Effective Non-Noble Metal Supported on Conducting Polymer Composite Such as Nickel Nanoparticles/Polypyrrole as Efficient Anode Electrocatalyst for Ethanol Oxidation. Mater. Chem. Phys. 250, 123009. doi:10.1016/j.matchemphys.2020.123009
El Attar, A., Chemchoub, S., Diallo Kalan, M., Oularbi, L., and El Rhazi, M. (2022). Designing New Material Based on Functionalized Multi-Walled Carbon Nanotubes and Cu(OH)2–Cu2O/Polypyrrole Catalyst for Ethanol Oxidation in Alkaline Medium. Front. Chem. 9, 805654. Available at: https://www.frontiersin.org/article/10.3389/fchem.2021.805654 (Accessed February 6, 2022). doi:10.3389/fchem.2021.805654
El Attar, A., Oularbi, L., Chemchoub, S., and El Rhazi, M. (2021). Effect of Electrochemical Activation on the Performance and Stability of Hybrid (PPy/Cu2O Nanodendrites) for Efficient Ethanol Oxidation in Alkaline Medium. J. Electroanalytical Chem. 885, 115042. doi:10.1016/j.jelechem.2021.115042
El Attar, A., Oularbi, L., Chemchoub, S., and El Rhazi, M. (2020). Preparation and Characterization of Copper Oxide Particles/Polypyrrole (Cu2O/PPy) via Electrochemical Method: Application in Direct Ethanol Fuel Cell. Int. J. Hydrogen Energ. 45, 8887–8898. doi:10.1016/j.ijhydene.2020.01.008
El Rhazi, M., Majid, S., Elbasri, M., Salih, F. E., Oularbi, L., and Lafdi, K. (2018). Recent Progress in Nanocomposites Based on Conducting Polymer: Application as Electrochemical Sensors. Int. Nano Lett. 8, 79–99. doi:10.1007/s40089-018-0238-2
Elbasri, M., and Rhazi, M. E. (2015). Preparation and Characterization of Carbon Paste Electrode Modified by Poly(1,8-Diaminonaphthalene) and Nickel Ions Particles: Application to Electrocatalytic Oxidation of Methanol. Mater. Today Proc. 2, 4676–4683. doi:10.1016/j.matpr.2015.09.022
Fan, X., Lin, P., Liang, S., Hui, N., Zhang, R., Feng, J., et al. (2017). Gold Nanoclusters Doped Poly(3,4-Ethylenedioxythiophene) for Highly Sensitive Electrochemical Sensing of Nitrite. Ionics 23, 997–1003. doi:10.1007/s11581-016-1865-0
Gracia, R., and Mecerreyes, D. (2013). Polymers with Redox Properties: Materials for Batteries, Biosensors and More. Polym. Chem. 4, 2206–2214. doi:10.1039/C3PY21118E
Guo, H., Fan, T., Yao, W., Yang, W., Wu, N., Liu, H., et al. (2020). Simultaneous Determination of 4-Aminophenol and Acetaminophen Based on High Electrochemical Performance of ZIF-67/MWCNT-COOH/Nafion Composite. Microchemical J. 158, 105262. doi:10.1016/j.microc.2020.105262
Halim, E. M., Elbasri, M., Perrot, H., Sel, O., Lafdi, K., and El Rhazi, M. (2019). Synthesis of Carbon Nanofibers/Poly(Para-Phenylenediamine)/Nickel Particles Nanocomposite for Enhanced Methanol Electrooxidation. Int. J. Hydrogen Energ. 44, 24534–24545. doi:10.1016/j.ijhydene.2019.07.141
Han, S., and Chen, X. (2019). Copper Nanoclusters-Enhanced Chemiluminescence for Folic Acid and Nitrite Detection. Spectrochimica Acta A: Mol. Biomol. Spectrosc. 210, 315–320. doi:10.1016/j.saa.2018.11.051
Jaiswal, N., Tiwari, I., Foster, C. W., and Banks, C. E. (2017). Highly Sensitive Amperometric Sensing of Nitrite Utilizing Bulk-Modified MnO 2 Decorated Graphene Oxide Nanocomposite Screen-Printed Electrodes. Electrochimica Acta 227, 255–266. doi:10.1016/j.electacta.2017.01.007
Jian, J.-M., Fu, L., Ji, J., Lin, L., Guo, X., and Ren, T.-L. (2018). Electrochemically Reduced Graphene Oxide/Gold Nanoparticles Composite Modified Screen-Printed Carbon Electrode for Effective Electrocatalytic Analysis of Nitrite in Foods. Sensors Actuators B: Chem. 262, 125–136. doi:10.1016/j.snb.2018.01.164
Jiao, S., Jin, J., and Wang, L. (2015). One-Pot Preparation of Au-RGO/PDDA Nanocomposites and Their Application for Nitrite Sensing. Sensors Actuators B: Chem. 208, 36–42. doi:10.1016/j.snb.2014.11.020
Kalaycıoğlu, Z., and Erim, F. B. (2016). Simultaneous Determination of Nitrate and Nitrite in Fish Products with Improved Sensitivity by Sample Stacking-Capillary Electrophoresis. Food Anal. Methods 9, 706–711. doi:10.1007/s12161-015-0241-4
Karimi-Maleh, H., Orooji, Y., Karimi, F., Alizadeh, M., Baghayeri, M., Rouhi, J., et al. (2021). A Critical Review on the Use of Potentiometric Based Biosensors for Biomarkers Detection. Biosens. Bioelectron. 184, 113252. doi:10.1016/j.bios.2021.113252
Katabami, K., Hayakawa, M., and Gando, S. (2016). Severe Methemoglobinemia Due to Sodium Nitrite Poisoning. Case Rep. Emerg. Med. 2016, 9013816. doi:10.1155/2016/9013816
Kesavan, S., Kumar, D. R., Baynosa, M. L., and Shim, J.-J. (2018). Potentiodynamic Formation of Diaminobenzene Films on an Electrochemically Reduced Graphene Oxide Surface: Determination of Nitrite in Water Samples. Mater. Sci. Eng. C 85, 97–106. doi:10.1016/j.msec.2017.12.004
Lee, J. W., Park, D. S., Shim, Y. B., and Park, S. M. (1992). Electrochemical Characterization of Poly(1,8‐Diaminonaphthalene): A Functionalized Polymer. J. Electrochem. Soc. 139, 3507–3514. doi:10.1149/1.2069107
Li, L., Liu, D., Wang, K., Mao, H., and You, T. (2017). Quantitative Detection of Nitrite with N-Doped Graphene Quantum Dots Decorated N-Doped Carbon Nanofibers Composite-Based Electrochemical Sensor. Sensors Actuators B: Chem. 252, 17–23. doi:10.1016/j.snb.2017.05.155
Lin, P., Chai, F., Zhang, R., Xu, G., Fan, X., and Luo, X. (2016). Electrochemical Synthesis of Poly(3,4-Ethylenedioxythiophene) Doped with Gold Nanoparticles, and its Application to Nitrite Sensing. Microchim. Acta 183, 1235–1241. doi:10.1007/s00604-016-1751-5
Lin, S.-L., Hsu, J.-W., and Fuh, M.-R. (2019). Simultaneous Determination of Nitrate and Nitrite in Vegetables by Poly(Vinylimidazole-Co-Ethylene Dimethacrylate) Monolithic Capillary Liquid Chromatography with UV Detection. Talanta 205, 120082. doi:10.1016/j.talanta.2019.06.082
Liu, S., Tian, J., Wang, L., Luo, Y., and Sun, X. (2011). Production of Stable Aqueous Dispersion of Poly(3,4-Ethylenedioxythiophene) Nanorods Using Graphene Oxide as a Stabilizing Agent and Their Application for Nitrite Detection. Analyst 136, 4898. doi:10.1039/c1an15799j
Lo, H.-S., Lo, K.-W., Yeung, C.-F., and Wong, C.-Y. (2017). Rapid Visual and Spectrophotometric Nitrite Detection by Cyclometalated Ruthenium Complex. Analytica Chim. Acta 990, 135–140. doi:10.1016/j.aca.2017.07.018
Majid, S., Rhazi, M. E., Amine, A., Curulli, A., and Palleschi, G. (2003). Carbon Paste Electrode Bulk-Modified with the Conducting Polymer Poly(1,8-Diaminonaphthalene): Application to Lead Determination. Microchimica Acta 143, 195–204. doi:10.1007/s00604-003-0058-5
Mani, V., Govindasamy, M., Chen, S.-M., Chen, T.-W., Kumar, A. S., and Huang, S.-T. (2017). Core-Shell Heterostructured Multiwalled Carbon Nanotubes@reduced Graphene Oxide Nanoribbons/Chitosan, a Robust Nanobiocomposite for Enzymatic Biosensing of Hydrogen Peroxide and Nitrite. Sci. Rep. 7, 11910. doi:10.1038/s41598-017-12050-x
Mao, Y., Bao, Y., Han, D.-X., and Zhao, B. (2018). Research Progress on Nitrite Electrochemical Sensor. Chin. J. Anal. Chem. 46, 147–155. doi:10.1016/S1872-2040(17)61066-1
Muthumariappan, A., Govindasamy, M., Chen, S.-M., Sakthivel, K., and Mani, V. (2017). Screen-printed Electrode Modified with a Composite Prepared from Graphene Oxide Nanosheets and Mn3O4 Microcubes for Ultrasensitive Determination of Nitrite. Microchim. Acta 184, 3625–3634. doi:10.1007/s00604-017-2379-9
Ning, X., Li, Y., Ming, J., Wang, Q., Wang, H., Cao, Y., et al. (2019). Electronic Synergism of Pyridinic- and Graphitic-Nitrogen on N-Doped Carbons for the Oxygen Reduction Reaction. Chem. Sci. 10, 1589–1596. doi:10.1039/C8SC04596H
Oularbi, L. (2018). Étude de nanocomposites polypyrrole/nanoparticule de carbone par impédance électrochimique et ac-électrogravimétrie: application aux capteurs électrochimiques. Aux Capteurs ȁlectrochimiques, Ph.D. Thesis, Sorbonne and Hassan II Casablanca Universities, Casablanca, Morocco, June 29, 2018. Thesis `NNT : 2018SORUS110b. `tel-02922320b.
Oularbi, L., Turmine, M., and El Rhazi, M. (2017). Electrochemical Determination of Traces lead Ions Using a New Nanocomposite of Polypyrrole/Carbon Nanofibers. J. Solid State. Electrochem. 21, 3289–3300. doi:10.1007/s10008-017-3676-2
Oularbi, L., Turmine, M., and El Rhazi, M. (2019). Preparation of Novel Nanocomposite Consisting of Bismuth Particles, Polypyrrole and Multi-Walled Carbon Nanotubes for Simultaneous Voltammetric Determination of Cadmium(II) and Lead(II). Synth. Met. 253, 1–8. doi:10.1016/j.synthmet.2019.04.011
Oularbi, L., Turmine, M., Salih, F. E., and El Rhazi, M. (2020). Ionic Liquid/carbon Nanofibers/bismuth Particles Novel Hybrid Nanocomposite for Voltammetric Sensing of Heavy Metals. J. Environ. Chem. Eng. 8, 103774. doi:10.1016/j.jece.2020.103774
Oyama, N., Sato, M., and Ohsaka, T. (1989). Preparation of Thin Polymeric Films on Electrode Surfaces by Electro-Polymerization of Aromatic Compounds with Amino Groups. Synth. Met. 29, 501–506. doi:10.1016/0379-6779(89)90340-8
Pałys, B. J., Skompska, M., and Jackowska, K. (1997). Sensitivity of Poly 1,8-Diaminonaphthalene to Heavy Metal Ions — Electrochemical and Vibrational Spectra Studies. J. Electroanal. Chem. 433, 41–48. doi:10.1016/S0022-0728(97)00144-7
Rajalakshmi, K., and John, S. A. (2015). Highly Sensitive Determination of Nitrite Using FMWCNT-Conducting Polymer Composite Modified Electrode. Sensors Actuators B: Chem. 215, 119–124. doi:10.1016/j.snb.2015.03.050
Ren, H.-H., Fan, Y., Wang, B., and Yu, L.-P. (2018). Polyethylenimine-Capped CdS Quantum Dots for Sensitive and Selective Detection of Nitrite in Vegetables and Water. J. Agric. Food Chem. 66, 8851–8858. doi:10.1021/acs.jafc.8b01951
Ribeiro, M. C., Bezerra, T. D. S., Soares, A. C., Boechat-Ramos, R., Carneiro, F. P., Vianna, L. M. D. S., et al. (2017). Hippocampal and Cerebellar Histological Changes and Their Behavioural Repercussions Caused by Brain Ischaemic Hypoxia Experimentally Induced by Sodium Nitrite. Behav. Brain Res. 332, 223–232. doi:10.1016/j.bbr.2017.06.008
Roy, A., Ray, A., Saha, S., and Das, S. (2018). Investigation on Energy Storage and Conversion Properties of Multifunctional PANI-MWCNT Composite. Int. J. Hydrogen Energ. 43, 7128–7139. doi:10.1016/j.ijhydene.2018.02.153
Rui, M., Jiang, Y., and Zhu, A. (2020). Sub-Micron Calcium Carbonate as a Template for the Preparation of Dendrite-Like PANI/CNT Nanocomposites and its Corrosion protection Properties. Chem. Eng. J. 385, 123396. doi:10.1016/j.cej.2019.123396
Salagare, S., Shivappa Adarakatti, P., and Venkataramanappa, Y. (2020). Designing and Construction of Carboxyl Functionalised MWCNTs/Co-MOFs-Based Electrochemical Sensor for the Sensitive Detection of Nitrite. Int. J. Environ. Anal. Chem. 100, 1–20. doi:10.1080/03067319.2020.1796989
Salhi, O., Ez‐zine, T., and El Rhazi, M. (2021). Hybrid Materials Based on Conducting Polymers for Nitrite Sensing: A Mini Review. Electroanalysis 33, 1681–1690. doi:10.1002/elan.202100033
Salih, F. E., Achiou, B., Ouammou, M., Bennazha, J., Ouarzane, A., Younssi, S. A., et al. (2017a). Electrochemical Sensor Based on Low Silica X Zeolite Modified Carbon Paste for Carbaryl Determination. J. Adv. Res. 8, 669–676. doi:10.1016/j.jare.2017.08.002
Salih, F. E., Ouarzane, A., and El Rhazi, M. (2017b). Electrochemical Detection of lead (II) at Bismuth/Poly(1,8-Diaminonaphthalene) Modified Carbon Paste Electrode. Arabian J. Chem. 10, 596–603. doi:10.1016/j.arabjc.2015.08.021
Salih, F. E., Oularbi, L., Halim, E., Elbasri, M., Ouarzane, A., and El Rhazi, M. (2018). Conducting Polymer/Ionic Liquid Composite Modified Carbon Paste Electrode for the Determination of Carbaryl in Real Samples. Electroanalysis 30, 1855–1864. doi:10.1002/elan.201800152
Shaikshavali, P., Madhusudana Reddy, T., Venu Gopal, T., Venkataprasad, G., Kotakadi, V. S., Palakollu, V. N., et al. (2020). A Simple Sonochemical Assisted Synthesis of Nanocomposite (ZnO/MWCNTs) for Electrochemical Sensing of Epinephrine in Human Serum and Pharmaceutical Formulation. Colloids Surf. Physicochem. Eng. Asp. 584, 124038. doi:10.1016/j.colsurfa.2019.124038
Shi, S., Li, Z., Chen, Y., Yang, J., Xu, H., and Huang, J. (2019). Electrochemically Co-Deposition of Palladium Nanoparticles and Poly(1, 5-Diaminonaphthalene) onto Multiwalled Carbon Nanotubes (MWCNTs) Modified Electrode and its Application for Amperometric Determination of Nitrite. Int. J. Electrochem. Sci. 14, 7983–7994. doi:10.20964/2019.08.22
Song, P., Wu, L., and Guan, W. (2015). Dietary Nitrates, Nitrites, and Nitrosamines Intake and the Risk of Gastric Cancer: A Meta-Analysis. Nutrients 7, 9872–9895. doi:10.3390/nu7125505
Thirumalraj, B., Palanisamy, S., Chen, S.-M., and Zhao, D.-H. (2016). Amperometric Detection of Nitrite in Water Samples by Use of Electrodes Consisting of Palladium-Nanoparticle-Functionalized Multi-Walled Carbon Nanotubes. J. Colloid Interf. Sci. 478, 413–420. doi:10.1016/j.jcis.2016.06.014
Vagin, M., Gueskine, V., Mitraka, E., Wang, S., Singh, A., Zozoulenko, I., et al. (2021). Negatively‐Doped Conducting Polymers for Oxygen Reduction Reaction. Adv. Energ. Mater. 11, 2002664. doi:10.1002/aenm.202002664
Velmurugan, S., Palanisamy, S., and Yang, T. C.-K. (2020). Single-Crystalline SnS2 Nano-Hexagons Based Non-Enzymatic Electrochemical Sensor for Detection of Carcinogenic Nitrite in Food Samples. Sensors Actuators B: Chem. 316, 128106. doi:10.1016/j.snb.2020.128106
Wan, Y., Zheng, Y. F., Wan, H. T., Yin, H. Y., and Song, X. C. (2017). A Novel Electrochemical Sensor Based on Ag Nanoparticles Decorated Multi-Walled Carbon Nanotubes for Applied Determination of Nitrite. Food Control 73, 1507–1513. doi:10.1016/j.foodcont.2016.11.014
Wang, Q.-H., Yu, L.-J., Liu, Y., Lin, L., Lu, R.-G., Zhu, J.-P., et al. (2017). Methods for the Detection and Determination of Nitrite and Nitrate: A Review. Talanta 165, 709–720. doi:10.1016/j.talanta.2016.12.044
Wang, X., Cao, T., Zuo, Q., Wu, S., Uchiyama, S., and Matsuura, H. (2016). Sensitive Nitrite Detection Using a Simple Electrochemically Aminated Glassy Carbon Electrode. Anal. Methods 8, 3445–3449. doi:10.1039/C6AY00015K
Yu, H., Li, R., and Song, K.-l. (2019). Amperometric Determination of Nitrite by Using a Nanocomposite Prepared from Gold Nanoparticles, Reduced Graphene Oxide and Multi-Walled Carbon Nanotubes. Microchim. Acta 186, 624. doi:10.1007/s00604-019-3735-8
Yu, M., Li, C., Hu, C., Jin, J., Qian, S., and Jin, J. (2020). The Relationship between Consumption of Nitrite or Nitrate and Risk of Non-Hodgkin Lymphoma. Sci. Rep. 10, 551. doi:10.1038/s41598-020-57453-5
Zhan, Y., Zeng, Y., Li, L., Luo, F., Qiu, B., Lin, Z., et al. (2019). Ratiometric Fluorescent Hydrogel Test Kit for On-Spot Visual Detection of Nitrite. ACS Sens. 4, 1252–1260. doi:10.1021/acssensors.9b00125
Zhang, F.-X., Miao, Y., Ruan, J.-G., Meng, S.-P., Dong, J.-D., Yin, H., et al. (2019). Association between Nitrite and Nitrate Intake and Risk of Gastric Cancer: A Systematic Review and Meta-Analysis. Med. Sci. Monit. 25, 1788–1799. doi:10.12659/MSM.914621
Zhang, S., Li, B.-Q., and Zheng, J.-B. (2015). An Electrochemical Sensor for the Sensitive Determination of Nitrites Based on Pt-PANI-Graphene Nanocomposites. Anal. Methods 7, 8366–8372. doi:10.1039/C5AY01710F
Zhao, Z., Xia, Z., Liu, C., Huang, H., and Ye, W. (2017). Green Synthesis of Pd/Fe3O4 Composite Based on polyDOPA Functionalized Reduced Graphene Oxide for Electrochemical Detection of Nitrite in Cured Food. Electrochimica Acta 256, 146–154. doi:10.1016/j.electacta.2017.09.185
Zheng, D., Hu, C., Peng, Y., and Hu, S. (2009). A Carbon Nanotube/Polyvanillin Composite Film as an Electrocatalyst for the Electrochemical Oxidation of Nitrite and its Application as a Nitrite Sensor. Electrochimica Acta 54, 4910–4915. doi:10.1016/j.electacta.2009.04.004
Keywords: nitrite detection, conducting polymer, 1,8-Diaminonaphthalene, electrochemical sensor, multi-walled carbon nanotubes
Citation: Salhi O, Ez-zine T, Oularbi L and El Rhazi M (2022) Electrochemical Sensing of Nitrite Ions Using Modified Electrode by Poly 1,8-Diaminonaphthalene/Functionalized Multi-Walled Carbon Nanotubes. Front. Chem. 10:870393. doi: 10.3389/fchem.2022.870393
Received: 06 February 2022; Accepted: 02 March 2022;
Published: 16 March 2022.
Edited by:
Cosimino Malitesta, University of Salento, ItalyReviewed by:
Mehdi Baghayeri, Hakim Sabzevari University, IranMani Govindasamy, National Taipei University of Technology, Taiwan
Copyright © 2022 Salhi, Ez-zine, Oularbi and El Rhazi. This is an open-access article distributed under the terms of the Creative Commons Attribution License (CC BY). The use, distribution or reproduction in other forums is permitted, provided the original author(s) and the copyright owner(s) are credited and that the original publication in this journal is cited, in accordance with accepted academic practice. No use, distribution or reproduction is permitted which does not comply with these terms.
*Correspondence: Mama El Rhazi, elrhazim@hotmail.com