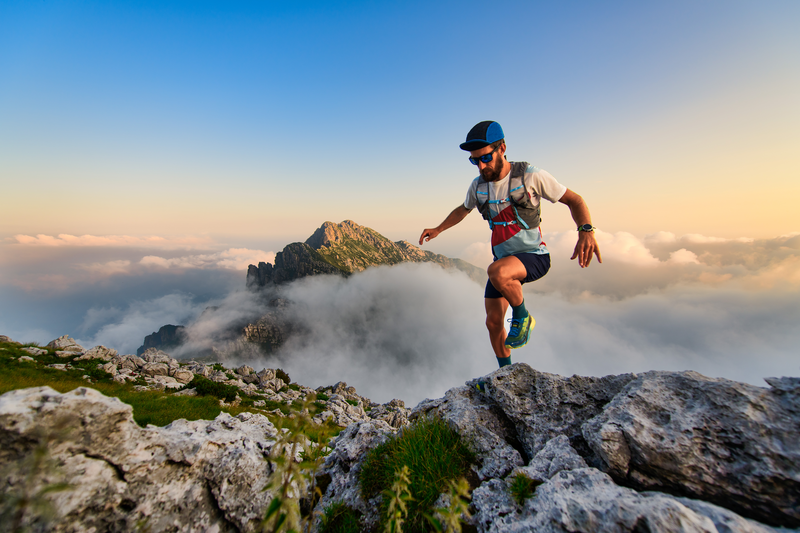
95% of researchers rate our articles as excellent or good
Learn more about the work of our research integrity team to safeguard the quality of each article we publish.
Find out more
ORIGINAL RESEARCH article
Front. Chem. , 11 May 2022
Sec. Organic Chemistry
Volume 10 - 2022 | https://doi.org/10.3389/fchem.2022.865012
A sustainable magnetic core-shell nanocatalyst Fe3O4@C@Fe(III) was successfully applied in the synthesis of a series of 2-nitro-2,3-unsaturated O-glycosides with excellent yields (up to 89%) and high stereoselectivity (α:β > 19:1). The substrate ranges are widely applicable, including different kinds of alcohols and even structurally complex acceptors. In addition, phenols could be applied in good yields. Moreover, the catalyst could be easily separated from the reaction by the application of an external magnetic force and reused a minimum of five times without any significant decrease in catalytic performance.
Amino deoxy sugars are commonly found in bioactive natural products (Wang et al., 2013; Dalziel et al., 2014; Canales et al., 2017; Wild et al., 2018; Shariatinia, 2019; Zhang et al., 2020). In particular, one of the most important amino deoxy sugars, 2-amino-2-deoxy glucose, is an essential unit of glucosaminoglycans (such as heparin and chondroitin sulfate) and many antibiotics (such as lividomycin), as shown in Figure 1. However, 2-amino-2-deoxy sugar–containing pharmaceutical agents with high purity and single stereo configuration are difficult to be extracted from natural sources due to their limited inventories and biosynthetic microheterogeneity (Suzuki et al., 2021; Zeng et al., 2021). Therefore, scientists have been making unremitting efforts to synthesize these compounds through chemical methods (Iglesias-Guerra et al., 1999; Vega-Pérez et al., 1999; Sugita et al., 2014).
2-nitro-2,3 unsaturated glycosides are a kind of important intermediates for the synthesis of 2-amino-2-deoxy sugars. However, so far, there are few reports illustrating efficient ways to obtain these compounds (Scheme 1). In 2013, Dharuman et al. (2013) initially reported their exploration on Ferrier rearrangement of 2-nitro glycals. 4-dimethylaminopyridine, an organic base, was used to successfully catalyze the reaction. However, the number of substrates suitable for this reaction condition was only five, and the yield was generally not very high (57–74%), probably due to the occurrence of deacylation side reaction under alkaline condition. In 2018, Lafuente et al. (2019) promoted the Ferrier rearrangement of 2-nitro glycals with several O-nucleophiles in the presence of CuFe2O4. The catalyst could be separated by an external magnet, while the reaction products were obtained in good yields and high stereoselectivity. However, the preparation process of the Cu–Fe spinel catalyst was complicated, and the conditions were very harsh, so the number of suitable substrates was only eight. Recently, we published an article in which N-heterocyclic carbenes (NHCs) catalyzed Ferrier rearrangement smoothly with the aid of potassium carbonate (Jiang et al., 2021). The reaction conditions were mild, and the range of alcoholic substrates was wide, but the reaction was time-consuming and suffered deacylation as well. On the basis of reports in the literature (Michigami and Hayashi, 2012; Chen and Lin, 2013; Chen et al., 2017; Dong et al., 2019), we concluded that the nitro substituent at the C-2 position has a remarkable effect in the sugar ring and that electron-withdrawing groups reduce the electron density of the oxocarbenium intermediate in the normal Ferrier rearrangement. Consequently, the development of a green and broadly adaptive protocol for the synthesis of 2-nitro-2,3-unsaturated glycosides remains a challenge.
Our research group has been conducting a series of explorations on solid acid catalysts in environmentally benign reactions, such as H2SO4–SiO2, FeCl3·6H2O/C, and FeCl3/C, which have been applied in Ferrier rearrangement reactions with good yield in short time (Zhang et al., 2013; Zhang et al., 2018; Mei et al., 2020). Furthermore, these heterogenous catalysts could usually be easily separated from the reaction media and recycled to enhance their applicability. In addition, we are introducing a nano-magnetic core-shell catalyst into a Ferrier rearrangement of glycals due to their extraordinary noncontact magnetic separation (Wang et al., 2019; Zhang et al., 2021). Previously, Fe3O4@C-SO3H and Fe3O4@C@Fe(Ⅲ) have been studied for the synthesis of O-2,3-unsaturated glycosides (Zhang et al., 2017; Dong et al., 2019; Jiang et al., 2020a). The donors included 3,4,6-tri-O-acetyl-glucal, 3,4,6-tri-O-acetyl-2-haloglucals, and 3,4-di-O-acetyl-L-rhamnal. The acceptors consisted of primary alcohols, secondary alcohols, tert-butanol, unsaturated alcohols, halogenated alcohol, sterol, sugars, and phenols. O-2,3-unsaturated glycosides were obtained rapidly (<3 h) and efficiently (up to 98%) in good α-selectivity (α:β > 5:1 to 19:1). Moreover, the catalyst could be easily separated from the reaction with an external magnetic force and reused several times without any significant decrease in the yields of the products after every recycle, suggesting it to be a promising green catalyst in 2,3-unsaturated glycosides syntheses. Therefore, we would like to continue the research of Fe3O4@C@Fe(III) in the synthesis of 2-nitro unsaturated glycosides, which has not been attempted before.
All reactions were carried out under a dry nitrogen atmosphere. All solvents and reagents were obtained from commercial sources, unless otherwise stated, and were purified according to standard procedures. The removal of the solvent in vacuo refers to distillation using a rotary evaporator attached to an efficient vacuum pump. 1H NMR and 13C NMR spectra were recorded on a Bruker DRX-500 NMR spectrometer in solutions of CDCl3 using tetramethylsilane as the internal standard. Mass spectra were determined on LTQ-XL (Thermo Scientific, United States) with an (ESI) ion trap mass spectrometer.
To a mixture of 2-nitroglycal (63.4 mg, 0.2 mmol), acceptor (0.24 mmol), and the nanomagnetic catalyst Fe3O4@C@Fe(III) (0.06 mmol) was added DCM (2.0 ml) under a nitrogen atmosphere. The reaction mixture was stirred at 40°C and monitored by TLC (PE/EA, 2:1) until the reactant was consumed completely. After completion of the reaction, the catalyst was separated from the reaction with an external magnetic force and washed with dichloromethane. The solvent was removed under reduced pressure to afford a crude product, which was purified by silica gel flash chromatography with a solvent system (PE/EA, 4:1) to yield 2-nitro-2,3-unsaturated glycosides.
[(2R,3S,6S)-3-acetoxy-6-ethoxy-5-nitro-3,6-dihydro-2H-pyran-2-yl] methyl acetate (3a): colorless syrup. 1H NMR (500 MHz, CDCl3) δ 7.16 (d, J = 1.0 Hz, 1H), 5.57 (d, J = 6.7 Hz, 2H), 4.25 (s, 3H), 3.89 (ddd, J = 15.7, 8.3, 4.0 Hz, 1H), 3.76 (ddd, J = 8.7, 7.5, 4.8 Hz, 1H), 2.14 (s, 3H), 2.10 (s, 3H), 1.26 (t, J = 7.1 Hz, 3H). 13C NMR (125 MHz, CDCl3) δ 170.70, 169.91, 148.44, 132.50, 92.30, 66.41, 65.90, 64.59, 62.16, 20.82, 20.82, 15.15. HRMS (ESI): m/z calculated for C12H17NO8Na [M + Na]+326.0846, found 326.0849.
[(2R,3S,6S)-3-acetoxy-6-methoxy-5-nitro-3,6-dihydro-2H-pyran-2-yl] methyl acetate (3b): colorless syrup. 1H NMR (500 MHz, CDCl3) δ 7.19 (d, J = 2.2 Hz, 1H), 5.58 (d, J = 6.7 Hz, 1H), 5.48 (s, 1H), 4.31–4.26 (m, 2H), 4.25–4.20 (m, 1H), 3.56 (s, 3H), 2.15 (s, 3H), 2.12 (s, 3H). HRMS (ESI): m/z calculated for C11H15NO8Na [M + Na]+312.0690, found 312.0694.
[(2R,3S,6S)-3-acetoxy-6-(hexyloxy)-5-nitro-3,6-dihydro-2H-pyran-2-yl] methyl acetate (3c): colorless syrup. 1H NMR (500 MHz, CDCl3) δ 7.16 (d, J = 1.6 Hz, 1H), 5.57 (d, J = 6.7 Hz, 2H), 4.30–4.20 (m, 3H), 3.85–3.78 (m, 1H), 3.69–3.63 (m, 1H), 2.14 (s, 3H), 2.10 (s, 3H), 1.31–1.25 (m, 11H), 0.87 (t, J = 6.8 Hz, 4H). 13C NMR (125 MHz, CDCl3) δ 170.70, 169.91, 148.41, 132.48, 92.48, 70.50, 66.45, 64.58, 62.17, 31.94, 29.54, 29.40, 29.34, 26.16, 22.77, 20.83, 14.21. HRMS (ESI): m/z calculated for C18H29NO8Na [M + Na]+410.1785, found 410.1797.
[(2R,3S,6S)-3-acetoxy-6-(benzyloxy)-5-nitro-3,6-dihydro-2H-pyran-2-yl] methyl acetate (3d): colorless syrup. 1H NMR (500 MHz, CDCl3) δ 7.38–7.33 (m, 5H), 7.20 (d, J = 2.1 Hz, 1H), 5.71 (s, 1H), 5.59 (dd, J = 9.4, 1.4 Hz, 1H), 4.84 (d, J = 11.3 Hz, 1H), 4.76 (d, J = 11.3 Hz, 1H), 4.27 (ddd, J = 19.5, 9.8, 3.4 Hz, 2H), 4.12 (q, J = 7.1 Hz, 1H), 2.13 (s, 3H), 2.11 (s, 3H). HRMS (ESI): m/z calculated for C17H19NO8Na [M + Na]+388.1003, found 388.1013.
[(2R,3S,6S)-3-acetoxy-6-(allyloxy)-5-nitro-3,6-dihydro-2H-pyran-2-yl] methyl acetate(3e): colorless syrup. 1H NMR (500 MHz, CDCl3) δ 7.19 (d, J = 2.1 Hz, 1H), 5.95 (ddt, J = 16.7, 10.5, 5.9 Hz, 1H), 5.64 (s, 1H), 5.60–5.55 (m, 1H), 5.34 (dd, J = 17.2, 1.3 Hz, 1H), 5.30–5.25 (m, 1H), 4.32 (dd, J = 12.5, 5.5 Hz, 1H), 4.27 (d, J = 8.7 Hz, 3H), 4.24–4.20 (m, 1H), 2.15 (s, 3H), 2.11 (s, 3H). HRMS (ESI): m/z calculated for C13H17NO8Na [M + Na]+338.0846, found 338.0855.
[(2R,3S,6S)-3-acetoxy-5-nitro-6-(2,2,2-trichloroethoxy)-3,6-dihydro-2H-pyran-2-yl] methyl acetate (3f): colorless syrup. 1H NMR (500 MHz, CDCl3) δ 7.30 (d, J = 1.6 Hz, 1H), 5.79 (s, 1H), 5.62 (d, J = 9.8 Hz, 1H), 4.38 (d, J = 3.8 Hz, 2H), 4.28 (qd, J = 12.3, 3.5 Hz, 3H), 2.17 (s, 3H), 2.10 (s, 3H). 13C NMR (125 MHz, CDCl3) δ 170.62, 169.85, 146.95, 133.97, 95.43, 92.23, 80.72, 67.43, 64.31, 61.81, 20.82. HRMS (ESI): m/z calculated for C12H14Cl3NO8Na [M + Na]+427.9677, found 427.9686.
{(2R,3S,6S)-3-acetoxy-6-[(5-formylfuran-2-yl) methoxy]-5-nitro-3,6-dihydro-2H-pyran-2-yl} methyl acetate (3g): colorless syrup. 1H NMR (500 MHz, CDCl3) δ 9.64 (s, 1H), 7.23 (s, 2H), 6.61 (d, J = 2.8 Hz, 1H), 5.71 (s, 1H), 5.60 (d, J = 9.2 Hz, 1H), 4.84 (q, J = 13.3 Hz, 2H), 4.26 (dd, J = 11.8, 8.0 Hz, 3H), 2.15 (s, 3H), 2.11 (s, 3H). 13C NMR (125 MHz, CDCl3) δ 177.86, 170.64, 169.86, 156.42, 153.05, 147.51, 133.59, 121.95, 112.26, 100.14, 66.89, 64.37, 63.32, 61.82, 20.84, 20.82. HRMS (ESI): m/z calculated for C16H17NO10Na [M + Na] +406.0745, found 406.0746.
((2R,3S,6S)-3-acetoxy-5-nitro-6-{[(2R,3R,5R,6S)-3,4,5-tris(benzyloxy)-6-methoxytetrahydro-2H-pyran-2-yl] methoxy}-3,6-dihydro-2H-pyran-2-yl) methyl acetate (3h): colorless syrup. 1H NMR (500 MHz, CDCl3) δ 7.39–7.26 (m, 15H), 7.17 (d, J = 1.3 Hz, 1H), 5.73 (s, 1H), 5.56 (d, J = 9.5 Hz, 1H), 4.98 (d, J = 10.9 Hz, 1H), 4.89 (d, J = 11.1 Hz, 1H), 4.80 (d, J = 11.1 Hz, 2H), 4.67 (d, J = 12.0 Hz, 1H), 4.58 (d, J = 11.2 Hz, 2H), 4.19 (s, 3H), 4.01 (t, J = 9.3 Hz, 1H), 3.95 (d, J = 11.5 Hz, 1H), 3.87 (dd, J = 11.7, 4.6 Hz, 1H), 3.78 (dd, J = 9.8, 3.5 Hz, 1H), 3.57 (dd, J = 9.6, 3.4 Hz, 1H), 3.48 (t, J = 9.4 Hz, 1H), 3.36 (s, 3H), 2.14 (s, 3H), 2.04 (s, 3H). 13C NMR (125 MHz, CDCl3) δ 170.65, 169.87, 148.27, 138.84, 138.32, 138.29, 132.83, 128.60, 128.57, 128.53, 128.27, 128.15, 128.04, 127.91, 127.89, 127.75, 98.21, 92.33, 82.03, 80.19, 77.61, 75.87, 75.02, 73.65, 70.48, 67.69, 66.38, 64.49, 61.98, 55.41, 20.84, 20.80. HRMS (ESI): m/z calculated for C38H43NO13Na [M + Na]+744.2627, found 744.2623.
[(2R,3S,6S)-3-acetoxy-6-isopropoxy-5-nitro-3,6-dihydro-2H-pyran-2-yl] methyl acetate(3i): colorless syrup. 1H NMR (500 MHz, CDCl3) δ 7.15 (d, J = 2.1 Hz, 1H), 5.67 (s, 1H), 5.55 (dd, J = 9.4, 2.0 Hz, 1H), 4.32–4.27 (m, 1H), 4.27–4.23 (m, 2H), 4.07 (dd, J = 12.4, 6.2 Hz, 1H), 2.15 (s, 3H), 2.10 (s, 3H), 1.27 (d, J = 6.2 Hz, 3H), 1.24 (d, J = 6.2 Hz, 3H). 13C NMR (125 MHz, CDCl3) δ 170.71, 169.99, 132.28, 100.14, 91.31, 73.32, 66.37, 64.72, 62.32, 23.47, 21.86, 20.86, 20.83. HRMS (ESI): m/z calculated for C13H19NO8Na [M + Na] +340.1003, found 340.1007.
((2R,3S,6S)-3-acetoxy-6-{[(1S,2S,5R)-2-isopropyl-5-methylcyclohexyl]oxy}-5-nitro-3,6-dihydro-2H-pyran-2-yl)methyl acetate (3j): colorless syrup. 1H NMR (500 MHz, CDCl3) δ 7.13 (d, J = 2.1 Hz, 1H), 5.70 (s, 1H), 5.54 (d, J = 9.7 Hz, 1H), 4.31 (d, J = 9.0 Hz, 1H), 4.28–4.18 (m, 2H), 3.57 (t, J = 10.2 Hz, 1H), 2.29 (d, J = 11.8 Hz, 1H), 2.14 (s, 3H), 2.11 (s, 3H), 2.07–2.00 (m, 1H), 1.64 (dd, J = 10.0, 2.7 Hz, 2H), 1.42 (d, J = 2.9 Hz, 1H), 1.21 (d, J = 10.3 Hz, 1H), 1.08–0.98 (m, 2H), 0.93 (d, J = 5.2 Hz, 3H), 0.85 (d, J = 5.8 Hz, 4H), 0.78 (d, J = 5.6 Hz, 3H). 13C NMR (125 MHz, CDCl3) δ 170.79, 169.95, 148.94, 131.89, 93.51, 82.95, 66.38, 64.63, 62.47, 48.63, 43.12, 34.33, 31.72, 25.02, 22.95, 22.59, 21.27, 20.92, 20.86, 15.72. HRMS (ESI): m/z calculated for C20H31NO8Na [M + Na] +436.1942, found 436.1951.
((2R,3S,6S)-3-acetoxy-6-{[(3S,8S,9S,10R,13R,14S,17R)-10,13-dimethyl-17-[(R)-6-methylheptan-2-yl]-2,3,4,7,8,9,10,11,12,13,14,15,16,17-tetradecahydro-1H-cyclopenta[a]phenanthren-3-yl] oxy}-5-nitro-3,6-dihydro-2H-pyran-2-yl)methyl acetate (3k): white solid. 1H NMR (500 MHz, CDCl3) δ 7.16 (d, J = 2.2 Hz, 1H), 5.71 (s, 1H), 5.54 (dd, J = 9.7, 1.5 Hz, 1H), 5.37 (d, J = 5.1 Hz, 1H), 4.34–4.29 (m, 1H), 4.28–4.22 (m, 2H), 3.65 (dd, J = 11.0, 4.9 Hz, 1H), 2.38 (ddd, J = 18.4, 11.7, 9.4 Hz, 2H), 2.14 (s, 3H), 2.10 (s, 3H), 2.04–0.68 (41H, cholesteryl-H). 13C NMR (125 MHz, CDCl3) δ 170.75, 169.95, 148.74, 140.53, 132.33, 122.41, 91.20, 80.36, 66.46, 64.72, 62.37, 56.86, 56.29, 50.27, 42.47, 40.39, 39.88, 39.66, 37.13, 36.82, 36.33, 35.93, 32.09, 32.02, 28.38, 28.16, 27.98, 24.43, 23.97, 22.97, 22.71, 21.19, 20.89, 20.86, 19.49, 18.86, 12.00. HRMS (ESI): m/z calculated for C37H57NO8Na [M + Na] +666.3976, found 666.3987.
[(2R,3S,6R)-3-acetoxy-5-nitro-6-phenoxy-3,6-dihydro-2H-pyran-2-yl] methyl acetate (3l): colorless syrup. 1H NMR (500 MHz, CDCl3) δ 7.35 (dd, J = 11.8, 4.2 Hz, 3H), 7.16 (s, 1H), 7.13 (dd, J = 11.5, 4.1 Hz, 2H), 6.17 (s, 1H), 5.65 (dd, J = 9.3, 1.4 Hz, 1H), 4.46–4.41 (m, 1H), 4.31 (dd, J = 12.4, 5.2 Hz, 1H), 4.26 (dd, J = 12.3, 2.1 Hz, 1H), 2.18 (s, 3H), 2.04 (s, 3H). 13C NMR (125 MHz, CDCl3) δ 170.65, 169.89, 156.99, 155.14, 147.68, 133.71, 129.86, 124.00, 118.00, 99.90, 91.92, 67.30, 64.46, 61.95, 20.85, 20.77. HRMS (ESI): m/z calculated for C16H17NO8Na [M + Na] +374.0846, found 374.0851.
[(2R,3S,6R)-3-acetoxy-6-(4-methoxyphenoxy)-5-nitro-3,6-dihydro-2H-pyran-2-yl] methyl acetate (3m): colorless syrup. 1H NMR (500 MHz, CDCl3) δ7.32 (s, 1H), 7.11 (s, 2H), 6.86 (d, J = 17.1 Hz, 2H), 6.02 (s, 1H), 5.64 (d, J = 9.9 Hz, 1H), 4.46 (s, 1H), 4.30 (s, 2H), 3.79 (d, J = 0.9 Hz, 3H), 2.17 (s, 3H), 2.08 (s, 3H). 13C NMR (126 MHz, CDCl3) δ 170.86, 170.10, 156.52, 151.12, 147.81, 133.76, 119.93, 115.03, 93.21, 77.11, 67.35, 64.73, 62.28, 56.01, 21.05, 21.02. HRMS (ESI): m/z calculated for C17H19NO9Na [M + Na] +404.0952, found 404.0957.
[(2R,3R,6S)-3-acetoxy-6-(benzyloxy)-5-nitro-3,6-dihydro-2H-pyran-2-yl] methyl acetate (3o): colorless syrup. 1H NMR (500 MHz, CDCl3) δ 7.36 (dd, J = 8.7, 4.1 Hz, 5H), 7.29 (d, J = 5.6 Hz, 1H), 5.78 (s, 1H), 5.40 (dd, J = 5.6, 2.8 Hz, 1H), 4.84 (d, J = 11.2 Hz, 1H), 4.73 (d, J = 11.2 Hz, 1H), 4.49–4.45 (m, 1H), 4.28 (dd, J = 11.5, 5.5 Hz, 1H), 4.23 (dd, J = 11.5, 7.3 Hz, 1H), 2.12 (s, 3H), 2.09 (s, 3H).
[(2R,3R,6S)-3-acetoxy-6-methoxy-5-nitro-3,6-dihydro-2H-pyran-2-yl] methyl acetate (3p): colorless syrup. 1H NMR (500 MHz, CDCl3) δ 7.27 (d, J = 4.2 Hz, 1H), 5.55 (s, 1H), 5.39 (dd, J = 5.6, 2.8 Hz, 1H), 4.41–4.36 (m, 1H), 4.29–4.26 (m, 2H), 3.54 (s, 3H), 2.12 (s, 3H), 2.09 (s, 3H).
We first applied different catalysts, including conventional Lewis acid, solid acid, and nanomagnetic catalysts, while the reaction of one equivalent of 3,4,6-tri-O-acetyl-2-deoxy-2-nitro-glucal (1) with 1.2 equivalents of EtOH (2a) was considered the model reaction. As summarized in Table 1, it was found that in DCM, the catalyst and temperature are the key factors through the screening of different catalysts at room temperature (entries 1–7) and 40°C (entries 8–14). Among them, the nanocatalyst Fe3O4@C@Fe(III) gave the best performance at 40°C in DCM with a yield of 72% (entry 10). Next, in order to improve the reaction efficacy, we examined various solvents commonly used in Ferrier reactions (DCE, DCM, MeCN, THF, and 1,4-dioxane) and extended the reaction temperature range to 60°C (entries 16–22). However, we found that the reaction effect was not as good as that of entry 10, despite the fact that the yields increased when the temperature increased in these solvents. Finally, we tried to adjust the catalyst amount (entries 23–26) and found the best catalyst equivalent that could afford the product with excellent yield (84%) was 0.3 (entry 24).
With the optimal conditions established, we investigated the reaction scope using a variety of acceptors. As summarized in Table 2, this magnetic core-shell catalyst could effectively promote the Ferrier rearrangement reaction with different alcohol acceptors (including simple alcohol, branched-chain alcohol, unsaturated alcohol, halogenated alcohol, complex natural alcohol, and sugar acceptors) by the good yield to obtain the corresponding 2-nitro-2,3-unsaturated glycoside. Compared with the literature studies, the yields of compounds 3b (entry 2) and 3e (entry 5) were increased by 20%. The yield of compound 3d (entry 4) increased by 30% (Dharuman et al., 2013). Our previous research found that 2,3-unsaturated glycoside of hydroxymethylfurfural (HMF), which is a significant platform compound and valuable molecule from biomass materials, showed promising antitumor activities (Ding et al., 2018). Therefore, HMF was chosen as a special acceptor. The yield could reach 79% in this system, as shown in entry 7. Not only that, the glycosyl acceptor (entry 9) could be well-applied to the reaction system, and the yield could reach 83%. Moreover, isopropanol gave a fairly good yield in our system compared with that in the reported methods (Jiang et al., 2021). From entry 10 and entry 11, for complex natural alcohols, such as menthol and cholesterol, the reaction time was prolonged, and the yield was obtained with 71–73%. Phenolic acceptors, a type of difficult acceptors in the previous Ferrier rearrangement research, such as phenol and p-methoxyphenol, could also produce the corresponding phenolic glycosides with excellent yield at 0.1 equivalent (Dharuman et al., 2013; Jiang et al., 2021). Compared with the literature studies, the yield of the corresponding product 3m (entry 13) was also increased by 10% (Dharuman et al., 2013). In addition, it could be found from entries 12–14 that the reaction rate of p-methoxyphenol was higher than that of the phenol acceptor, while the reaction rate of p-bromophenol (entry 14) was almost zero, indicating that the electronic effect of the substituent groups on the phenolic acceptor has a significant influence on the reaction results. In addition, the reaction scope was tested with galactose donors 1b, gaining 3o–3p with good yields.
The structure and stereochemistry of the rearrangement products were elucidated by NMR and mass spectroscopy and were subsequently compared with the reported data (Dharuman et al., 2013; Lafuente et al., 2019; Jiang et al., 2021). It was noteworthy that the stereoselectivity of 2-nitro-2,3-unsaturated glycoside compounds was confirmed as α: β > 19:1 by 1H NMR.
As a catalyst with superparamagenetic nano-sized Fe3O4 nuclei, our catalyst is supposed to be recycled efficiently with an external magnet. We tested the recycling performance of the catalyst by using the model reaction to examine whether the ferric ion remained tightly bound to the core-shell Fe3O4@C under heated conditions. As shown in Figure 2, the product was still obtained in 80% yield even after the catalyst had been recycled five times. The catalyst Fe3O4@C@Fe(III) is suitable for the reaction system and has good recycle performance.
FIGURE 2. Recycling experiments of Fe3O4@C@Fe(III). Reaction conditions: 1 (0.05 mmol, 15.85 mg), EtOH (0.06 mmol, 3.6 µL), Fe3O4@C@Fe(III) (34 mg), and DCM (0.5 ml), 6 h, 40°C.
Based on the experimental results and literature studies, a reaction mechanism may be proposed as follows (Figure 3). (Dharuman et al., 2013; Lafuente et al., 2019; Jiang et al., 2020b; Jiang et al., 2021): As we know, a halogen substituent at the C-2 position of the glycals has a remarkable effect, and those electron-withdrawing groups would reduce the electron density of the oxocarbenium intermediate, which is commonly assumed as the intermediate cation in the Ferrier rearrangement (Dong et al., 2019). Thus, under the nanocatalysis of Fe3O4@C@Fe(III), C3 site deacylation in 2-nitro-glucal would occur very slowly and electron transfer would occur in a different way, with the aid of the nucleophiles. At the same time, due to the high steric hindrance of nanocatalysts, the nucleophilic receptor would have to attack the activated 2-nitroglycal from the α side to obtain the α-product via SN2’ mechanism (Lalic et al., 2008). Differing from the usual mechanism (Lalic et al., 2008), there is much fewer oxonium intermediates generated in our system, so the Ferrier-rearranged product (Lalic et al., 2008) was obtained with much higher stereoselectivity than that of the normal glycals and halogenated glycals.
In summary, we have successfully applied a nano-sized magnetic catalyst Fe3O4@C@Fe(III) for Ferrier rearrangement of 2-nitro glucal. This core-shell catalyst successfully solved the problems of catalyst separation, circulation, and substrate suitability. In the reaction system, substrates are widely applicable to various alcohols especially long-chain alcohols, branched-chain alcohols, unsaturated alcohols, halogenated alcohols, cyclic alcohols, steroidal alcohols, and carbohydrate acceptors. In addition, phenols could be applied in good yields. Furthermore, all the products could be achieved with a high stereoselectivity of α: β > 19:1. These results demonstrated that the catalyst and the optimal reaction condition are broadly applicable to different Ferrier rearrangement acceptors and have, thus, built the foundation for the synthesis of natural bioactive molecules and their analogs.
The original contributions presented in the study are included in the article/Supplementary Material, further inquiries can be directed to the corresponding author.
JZ was responsible for designing the experiments. NJ and ZD performed the experimentations and completed data processing. YY and YM analyzed the results and wrote the publication. All authors have given approval for the final version of the manuscript.
The authors declare that the research was conducted in the absence of any commercial or financial relationships that could be construed as a potential conflict of interest.
All claims expressed in this article are solely those of the authors and do not necessarily represent those of their affiliated organizations, or those of the publisher, the editors, and the reviewers. Any product that may be evaluated in this article, or claim that may be made by its manufacturer, is not guaranteed or endorsed by the publisher.
We acknowledge the analytic center of East China Normal University for spectroscopic measurements.
The Supplementary Material for this article can be found online at: https://www.frontiersin.org/articles/10.3389/fchem.2022.865012/full#supplementary-material
Canales, A., Boos, I., Perkams, L., Karst, L., Luber, T., Karagiannis, T., et al. (2017). Breaking the Limits in Analyzing Carbohydrate Recognition by NMR Spectroscopy: Resolving Branch-Selective Interaction of a Tetra-Antennary N -Glycan with Lectins. Angew. Chem. Int. Ed. 56, 14987–14991. doi:10.1002/anie.201709130
Chen, H., Luo, X., Qiu, S., Sun, W., and Zhang, J. (2017). TMSOTf Mediated Stereoselective Synthesis of α-C-glycosides from Unactivated Aryl Acetylenes. Glycoconj. J. 34, 13–20. doi:10.1007/s10719-016-9718-7
Chen, P., and Lin, L. (2013). RuCl3·3H2O as Catalyst for Ferrier Rearrangement: an Efficient Procedure for the Preparation of Pseudoglycosides. Tetrahedron 69, 10045–10051. doi:10.1016/j.tet.2013.09.061
Dalziel, M., Crispin, M., Scanlan, C. N., Zitzmann, N., and Dwek, R. A. (2014). Emerging Principles for the Therapeutic Exploitation of Glycosylation. Science 343, 1235681. doi:10.1126/science.1235681
Dharuman, S., Gupta, P., Kancharla, P. K., and Vankar, Y. D. (2013). Synthesis of 2-Nitroglycals from Glycals Using the Tetrabutylammonium Nitrate-Trifluoroacetic Anhydride-Triethylamine Reagent System and Base-Catalyzed Ferrier Rearrangement of Acetylated 2-Nitroglycals. J. Org. Chem. 78, 8442–8450. doi:10.1021/jo401165y
Ding, Z., Luo, X., Ma, Y., Chen, H., Qiu, S., Sun, G., et al. (2018). Eco-friendly Synthesis of 5-hydroxymethylfurfural (HMF) and its Application to the Ferrier-Rearrangement Reaction. J. Carbohydr. Chem. 37, 81–93. doi:10.1080/07328303.2018.1428990
Dong, Y., Ding, Z., Guo, H., Zhou, L., Jiang, N., Chen, H., et al. (2019). A Highly Efficient Magnetic Iron(III) Nanocatalyst for Ferrier Rearrangements. Synlett 30, 1419–1426. doi:10.1055/s-0037-1611855
Iglesias-Guerra, F., Candela, J. I., Bautista, J., Alcudia, F., and Vega-Pérez, J. M. (1999). Alkylating Agents from Sugars. Alkyl Hexopyranoside Derivatives as Carrier Systems for Chlorambucil. Carbohydr. Res. 316, 71–84. doi:10.1016/S0008-6215(99)00030-0
Jiang, N., Dong, Y., Sun, G., Yang, G., Wang, Q., and Zhang, J. (2020a). Core‐Shell Fe 3 O 4 @Carbon@SO 3 H: A Powerful Recyclable Catalyst for the Synthesis of α‐2‐Deoxygalactosides. ChemistrySelect 5, 1592–1596. doi:10.1002/slct.202000089
Jiang, N., Mei, Y., Yang, Y., Dong, Y., Ding, Z., and Zhang, J. (2021). A General Strategy for the Stereoselective Synthesis of Pyrrole‐Fused Chiral Skeletons: [3+2] Cycloaddition with 2‐Nitro‐2,3‐Unsaturated Glycosides. ChemCatChem 13, 3973–3982. doi:10.1002/cctc.202100795
Jiang, N., Wu, Z., Dong, Y., Xu, X., Liu, X., and Zhang, J. (2020b). Progress in the Synthesis of 2,3-unsaturated Glycosides. Coc 24 (2), 184–199. doi:10.2174/1385272824666200130111142
Lafuente, L., Rochetti, M. F., Bravo, R., Sasiambarrena, L., Santiago, C. C., and Ponzinibbio, A. (2019). Cu-Fe Spinels: First Heterogeneous and Magnetically Recoverable Catalyst for the Ferrier Rearrangement of 2-Nitroglycals. Loc 16, 447–453. doi:10.2174/1570178615666181022145338
Lalic, G., Krinsky, J. L., and Bergman, R. G. (2008). Scope and Mechanism of Formal SN2' Substitution Reactions of a Monomeric Imidozirconium Complex with Allylic Electrophiles. J. Am. Chem. Soc. 130 (13), 4459–4465. doi:10.1021/ja7106096
Mei, Y., Dong, Y., Li, J., Zhang, B., Sun, G., Zhou, J., et al. (2020). FeCl3/C as an Efficient Catalyst for Ferrier Rearrangement of 3,4,6-Tri-O-Benzyl-D-Glucal. J. Carbohydr. Chem. 39, 232–249. doi:10.1080/07328303.2020.1788575
Michigami, K., and Hayashi, M. (2012). O- and N-Glycosidation of D-Glycals Using Ferrier Rearrangement under Mitsunobu Reaction Conditions. Application to N-Nucleoside Synthesis. Tetrahedron 68, 1092–1096. doi:10.1016/j.tet.2011.11.084
Shariatinia, Z. (2019). Pharmaceutical Applications of Chitosan. Adv. Colloid Interf. Sci. 263, 131–194. doi:10.1016/j.cis.2018.11.008
Sugita, K., Tanabe, Y., Kodaira, N., Hirakawa, M., Yamaguchi, S., Shibata, A., et al. (2014). Facile Synthesis of Branched Chitin by Glycosylation of Fully Trimethylsilylated Chitin with a Glucosamine-Derived Oxazoline. Polym. Bull. 71 (4), 965–976. doi:10.1007/s00289-014-1104-7
Suzuki, N., Abe, T., Hanzawa, K., and Natsuka, S. (2021). Toward Robust N-Glycomics of Various Tissue Samples that May Contain Glycans with Unknown or Unexpected Structures. Sci. Rep. 11, 6334. doi:10.1038/s41598-021-84668-x
Vega-Pérez, J. M., Candela, J. I., Blanco, E., and Iglesias-Guerra, F. (1999). Alkylating Agents from Sugars. Stereoselective Synthesis of 2,3-Diaminoglucoses from 2-Nitroalkenes, as Intermediates in the Synthesis of Carriers of Chlorambucil. Tetrahedron 55, 9641–9650. doi:10.1016/S0040-4020(99)00510-4
Wang, Y.-T., Yang, X.-X., Xu, J., Wang, H.-L., Wang, Z.-B., Zhang, L., et al. (2019). Biodiesel Production from Esterification of Oleic Acid by a Sulfonated Magnetic Solid Acid Catalyst. Renew. Energ. 139, 688–695. doi:10.1016/j.renene.2019.02.111
Wang, Z., Chinoy, Z. S., Ambre, S. G., Peng, W., McBride, R., de Vries, R. P., et al. (2013). A General Strategy for the Chemoenzymatic Synthesis of Asymmetrically Branched N -Glycans. Science 341, 379–383. doi:10.1126/science.1236231
Wild, R., Kowal, J., Eyring, J., Ngwa, E. M., Aebi, M., and Locher, K. P. (2018). Structure of the Yeast Oligosaccharyltransferase Complex Gives Insight into Eukaryotic N-Glycosylation. Science 359, 545–550. doi:10.1126/science.aar5140
Zeng, X., Novotny, M. V., Clemmer, D. E., and VTrinidad, J. C. (2022). A Graphical Representation of Glycan Heterogeneity. Glycobiology 32, 201–207. doi:10.1093/glycob/cwab116/6433001
Zhang, J., Sun, G., Qiu, S., Ding, Z., Chen, H., Zhou, J., et al. (2017). Magnetic Core-Shell Fe3O4@C-So3h as an Efficient and Renewable 'Green Catalyst' for the Synthesis of O-2,3-Unsaturated Glycopyranosides. Synlett 28, 347–352. doi:10.1055/s-0036-1588891
Zhang, J., Sun, G., Wu, Y., Liu, A., Qiu, S., Zhang, W., et al. (2018). Substoichiometric FeCl3 Activation of Propargyl Glycosides for the Synthesis of Disaccharides and Glycoconjugates. Synlett 29, 668–672. doi:10.1055/s-0036-1591525
Zhang, J., Zhang, B., Zhou, J., Chen, H., Li, J., Yang, G., et al. (2013). A Facile H2SO4-SiO2-Catalyzed Ferrier Rearrangement of 3,4,6-Tri-O-Benzyl-D-Glucal. J. Carbohydr. Chem. 32, 380–391. doi:10.1080/07328303.2013.809093
Zhang, S., Hedtke, T., Zhu, Q., Sun, M., Weon, S., Zhao, Y., et al. (2021). Membrane-Confined Iron Oxychloride Nanocatalysts for Highly Efficient Heterogeneous Fenton Water Treatment. Environ. Sci. Technol. 55, 9266–9275. doi:10.1021/acs.est.1c01391
Zhang, Y., Zhang, H., Zhao, Y., Guo, Z., and Gao, J. (2020). Efficient Strategy for α-Selective Glycosidation of D-Glucosamine and its Application to the Synthesis of a Bacterial Capsular Polysaccharide Repeating Unit Containing Multiple α-Linked GlcNAc Residues. Org. Lett. 22, 1520–1524. doi:10.1021/acs.orglett.0c00101
Keywords: 2-nitro glycals, Ferrier rearrangement, nanomagnetic catalyst, Fe3O4@C@Fe(III), recyclable, 2-nitro-2, 3 unsaturated glycosides
Citation: Yang Y, Jiang N, Mei Y, Ding Z and Zhang J (2022) Synthesis of 2-Nitro-2,3-Unsaturated Glycosides by a Nanomagnetic Catalyst Fe3O4@C@Fe(III). Front. Chem. 10:865012. doi: 10.3389/fchem.2022.865012
Received: 31 January 2022; Accepted: 28 March 2022;
Published: 11 May 2022.
Edited by:
Zhendong Jin, The University of Iowa, United StatesCopyright © 2022 Yang, Jiang, Mei, Ding and Zhang. This is an open-access article distributed under the terms of the Creative Commons Attribution License (CC BY). The use, distribution or reproduction in other forums is permitted, provided the original author(s) and the copyright owner(s) are credited and that the original publication in this journal is cited, in accordance with accepted academic practice. No use, distribution or reproduction is permitted which does not comply with these terms.
*Correspondence: Jianbo Zhang, amJ6aGFuZ0BjaGVtLmVjbnUuZWR1LmNu
Disclaimer: All claims expressed in this article are solely those of the authors and do not necessarily represent those of their affiliated organizations, or those of the publisher, the editors and the reviewers. Any product that may be evaluated in this article or claim that may be made by its manufacturer is not guaranteed or endorsed by the publisher.
Research integrity at Frontiers
Learn more about the work of our research integrity team to safeguard the quality of each article we publish.