- 1Tianjin Key Laboratory for Modern Drug Delivery and High-Efficiency, School of Pharmaceutical Science and Technology, Tianjin University, Tianjin, China
- 2Hebei Key Laboratory of State Key Laboratory of Metastable Materials Science and Technology, Yanshan University, Qinhuangdao, China
The application of PhICl2/NH4SCN and PhICl2/KSeCN reagent systems to the synthesis of the biologically active S/SeCN-containing isocoumarins via a process involving thio/selenocyanation, enabled by thio/selenocyanogen chloride generated in situ, followed with an intramolecular lactonization was realized. Gram-scale synthesis, further derivatization to access C4 thio/selenocyanated Xyridin A and anti-tumor activities of the obtained products highlight the potential use of this method.
Introduction
Organosulfur and selenium compounds have been widely used in organic and biological chemistry (Parnham and Graf, 1991; Ip and Ganther, 1992; Ji et al., 1999; Mugesh et al., 2001; Yao and Larock, 2003; Borges et al., 2005; Mehta et al., 2009; Santos et al., 2009; Sperança et al., 2011; Wilkins et al., 2016; Glenadel et al., 2018; Lin et al., 2020; Mampuys et al., 2020). Among them, organic thiocyanated compounds and their selenylated analogs have attracted continuous attention of organic and medicinal chemists. They can be used as versatile building blocks to achieve various useful synthetic transformations since their thiocyanato and selenocyanato moieties can be readily converted to other sulfur and selenium-containing functional groups (Castanheiro et al., 2016; Zhang et al., 2018; Wu et al., 2021; Yuan et al., 2021). In the meanwhile, naturally occurring or pharmaceutically interesting organothio/selenocyanates have been reported to show a broad spectrum of bioactivities (Chen et al., 2007). For instances, the SCN-containing 4-phenoxyphenoxyethyl thiocyanate (Elhalem et al., 2002), psammaplin B (Piña et al., 2003), and cavernothiocyanate (Hirota et al., 1996) have been evaluated as antiparasitic agents, HDAC enzyme inhibitor and antifouling agents, respectively (Figure 1). Furthermore, it is reported that some NSAID selenocyanated derivatives exert promising activities in reducing the viability of certain type of cancer cell lines (Plano et al., 2016; Liu et al., 2018) (Figure 1). In these regards, direct or late-stage introduction of thio/selenocyanato functional groups into bioactive compounds is of great significance in organic and medicinal chemistry.
The chemistry that describes the preparation of 4-chalcogen isocoumain through the cyclization of 2-alkynylaryl esters promoted by electrophilic chalcogen species has been well documented (Yao and Larock, 2003; Mehta et al., 2009; Sperança et al., 2011; Wilkins et al., 2016; Glenadel et al., 2018; Lin et al., 2020). On the other aspect, thio/selenocyanation of alkenes (Yang et al., 2015; Zhang et al., 2018; Ye et al., 2019; Meng et al., 2020; Nadiveedhi et al., 2020) and hetero/aromatics (Feng et al., 2018; Rezayati and Ramazani, 2020) has also been extensively investigated. However, the thio/selenocyantion of alkynes, a straightforward and versatile route to construct Cvinyl–S(e) CN bond with the concomitant incorporation of a second functionality into the substrate, has remained less exploited. For examples, only dithio/selenocyanation (Prakash et al., 2001; Lu et al., 2018), hydrothiocyanation (Jiang et al., 2017; Wu et al., 2018), and iodothiocyanation (Zeng and Chen, 2018) of alkynes have been established so far. The thiocyanation and especially selenocyanation of alkynes coupled with an intramolecular functionalization, which could afford structurally diverse heterocycles remain unexplored. Our literature survey showed that the existing approaches include a metal-free synthesis of thiocyanato-containing azaspirotrienediones via photocatalytic carbothiocyanation of N-phenylpropynamides (Chen et al., 2019), a visible light-promoted carbon nitride-catalyzed thiocyanation of methylthiolated alkynones with NH4SCN affording the corresponding thiocyanated thioflavone products (Zeng et al., 2021), and a TCCA/NH4SCN-mediated cyclization/thiocyanation of alkynyl aryl ketones enabling the synthsis of 3-thiocyanated chromones (Xiao et al., 2021). Most recently, Zhou and co-workers reported the synthesis of isoquinolylsenocyanates and quinolylsenlenocyanates via electrophilic selenocyanogen cyclization induced by pseudohalogen (SeCN)2 generated in situ (Wang et al., 2022). Each of the above methods has its merits in preparing the corresponding S/SeCN-containing heterocycles. However, owing to the importance of the SCN/SeCN-containing heterocycles, it is still highly desirable to develop alternative and innovative approaches to realize the assemblage of versatile SCN/SeCN-containing heterocyclic framework.
PhICl2, the first hypervalent iodine reagent discovered in 1886 (Willgerodt, 1886), has found wide application in various organic transformations (Stang and Zhdankin, 1996). In 2019, we reported that PhICl2 could enable halolactonization of ortho-alkynylbenzoates, resulting in the formation of a series of functionalized 4-chloroisocoumarins under metal free conditions (Scheme 1A) (Xing et al., 2019b). By using PhICl2/NH4SCN system, we also realized the synthesis of the C5 thiocyanated 2-pyridones from pyridin-2(1H)-ones, demonstrating the efficiency of the reagents system in direct C-H functionalization/thiocyanation (Scheme 1B) (Tao et al., 2021). However, to the best of our knowledge, this oxidative PhICl2/NH4SCN system has never been used to the synthesis of thiocyanated heterocycles via intramolecular oxidative cyclization/oxythiocyanation of alkyne compounds, a strategy that is different from the above direct C–H functionalization/thiocyanation approach. In current work, we describe that by adopting PhICl2/NH4SCN reagents system, the biologically interesting C4-thiocyanated isocoumarins could be regioselectively achieved via oxythiocyanation of o-alkynylbenzoates. Furthermore, the protocol could be extended to the synthesis of C4-selenocyanated isocoumarins by using PhICl2/KSeCN, which is applied in organic synthesis for the first time (Scheme 1C).
Results and Discussion
Optimization of Reaction Conditions
At the outset of our studies, we were interested to investigate whether the PhICl2/NH4SCN reagent system could be applied to the intramolecular cyclization as well as oxythio/selenocyanation of o-alkynylbenzoates, in hope of achieving the biologically interesting C4-thio/selenocyanated isocoumarins. Our study on condition optimization was commenced with the simplest o-alkynylbenzoate 1a. To our delight, the desired C4-thiocyanated isocoumarin 2a could be obtained in 86% yield from the reaction of 1a with 2 equivalents of PhICl2 and 2 equivalents of NH4SCN in DCM for 12 h at room temperature (Table 1, entry 1). The solvents screening showed DCE to be superior to other commonly used solvents, including DCM, MeOH, EtOAc, toluene, and MeCN (Table 1, entries 2–6). Neither increasing nor decreasing the dosage of PhICl2/NH4SCN were beneficial for improving the yield of the product (Table 1, entries 7–8). The other oxidants including PIDA, PIFA, PhIO, I2, and NBS were also applied to take the place of PhICl2, however, lower yield, no reaction or none desired product were observed in these cases (Table 1, entries 9–13). The other SCN-containing inorganic salts including NaSCN, KSCN, AgSCN and CuSCN have also been investigated, but none of them provided better outcome than NH4SCN (SI, Supplementary Table S1, entries 14–17). We have also found that the reaction temperature had an obvious influence on the outcome of the reaction. Performing the reaction of 1a (0.20 mmol), PhICl2 (0.4 mmol) and NH4SCN (0.4 mmol) in DCE at 50°C not only improved the reaction yield to 96%, but also shorten the reaction time to 2 h (Table 1, entry 14). However, when the reaction temperature was further elevated to 60°C, it was found that product 2a was obtained in relatively lower yield, with the starting substrate 1a recovered in a yield of 5% (SI, Supplementary Table S1, entry 19). Furthermore, the reaction does not need a N2 atmosphere (Table 1, entry 14 vs 15). Based on the outcomes of the above screening experiments, the best yield of product 2a (96%) could be obtained by subjecting NH4SCN (2 equiv), PhICl2 (2 equiv) to DCE at 50°C for 2 h (Table 1, entry 14).
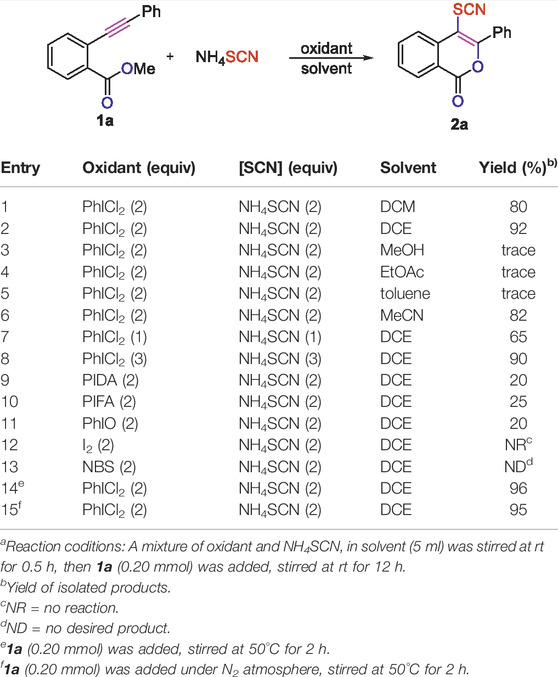
TABLE 1. Optimization on the reaction conditionsa.
Scope Exploration of Substrates
With the optimized conditions in hand, we came to investigate the substrate scope of this reaction by subjecting various o-alkynylbenzoates to the standard condition (Table 2). First, the electronic effect of R1 substituent on the phenyl ring of o-alkynylbenzoates was explored. Substrates with either electron-donating or -withdrawing groups on the phenyl ring were found to well participate the cyclization/thiocyanation reaction and the desired C4 thiocyanated ioscoumarins 2b-f were obtained in good to excellent yields. Moreover, it is worth noting that substrates bearing electron-donating groups (1a-c) exhibited better performance than those bearing electron-withdrawing substituents (1d-f). For instances, when R1 is a strong electron-withdrawing -NO2 group, the reaction smoothly afforded the corresponding isocoumarin 2d in 56% yield. Replacement of the -NO2 group with -Cl or -F groups provided better results, with corresponding products 2e-f obtained in 87 and 85%, respectively. The electronic effect of substituents on the alkyne motif was carefully tested next. To our satisfaction, this transformation was applicable to alkynoates bearing diverse substituents ranging from phenyl, naphthyl, thienyl to alkyl, with the corresponding C4 thiocyanated products 2g-t accomplished in good to excellent yields. Most strikingly, TMS functionality in the substrate was also well tolerated under the reaction conditions and the target product 2r was obtained in 80% yield. The method was also applicable to terminal alkyne, which could afford the corresponding C3-unsubstituted isocoumarin 2s in 84% yield.
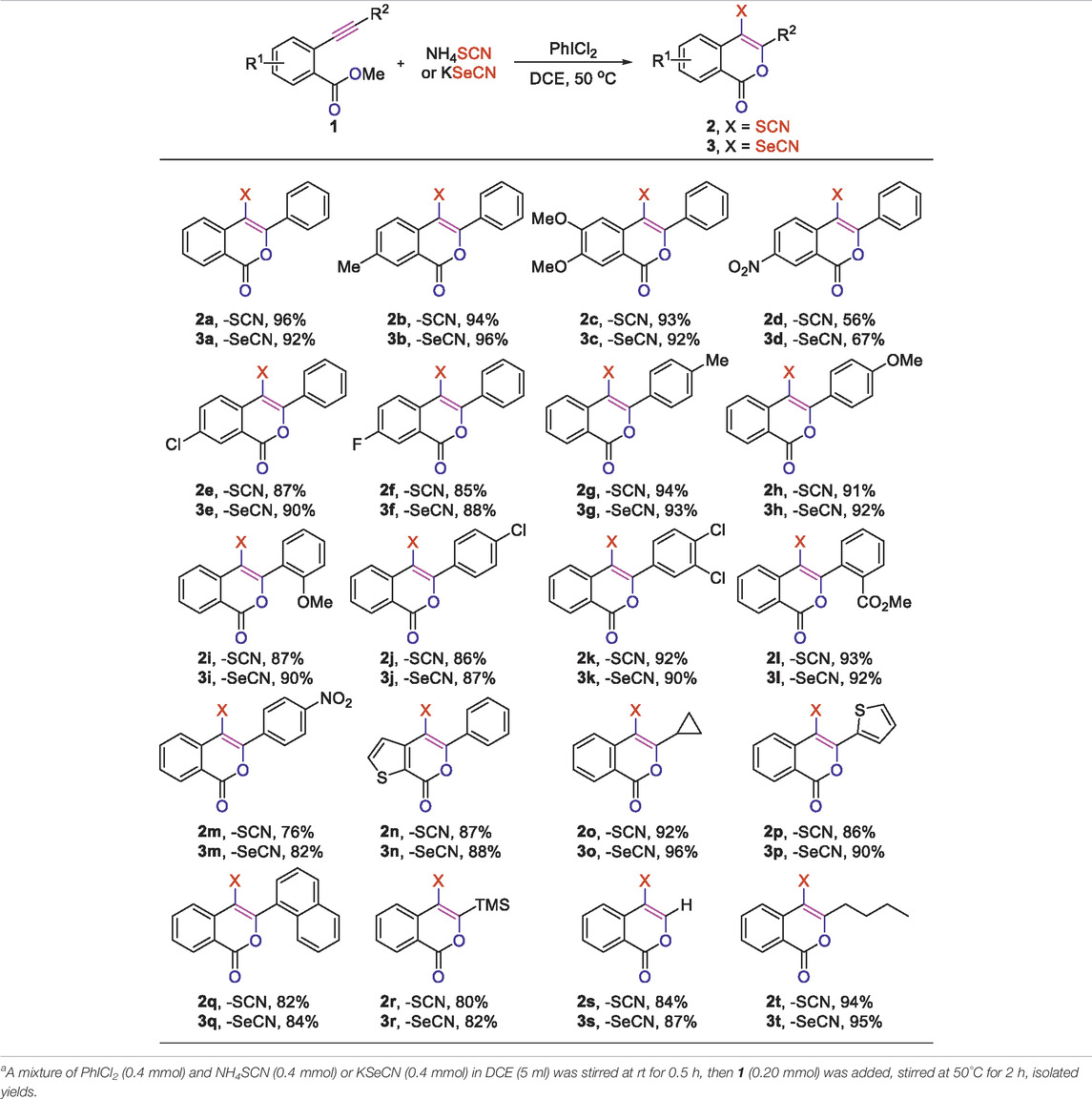
TABLE 2. Electrophilic thio/selenocyanation of o-alkynylbenzoatea.
Encouraged by the feasibility of oxythiocyanation of o-alkynylbenzoates, we further explored the synthesis of C4 selenocyanated isocoumarins, a closely related analogue of thiocyanated isocoumarins. Since the NH4SeCN was not commercially available, we had to resort to other selenocyanate sources (SI, Supplementary Table S2). To our delight, when KSeCN was applied, the reaction worked equally well as the oxythiocyanation and the corresponding selenocyanated product 3a was obtained in 92% yield. Under the adjusted conditions, a series of o-alkynylbenzoates were converted to the corresponding selenocyanated analogs 3b-m in high to excellent yields, regardless of the electronic effect of substituents on the phenyl ring. Notably, functional groups such as -F, -Cl, -NO2, and -CO2Me remained intact during the transformation, thereby facilitating late-stage functionalization of the obtained products. Furthermore, heterocycle-fused substrate 1n also reacted smoothly under the newly optimized reaction conditions, affording the corresponding product 3n in good yield. Finally, good to excellent yields were observed for substrates with R2 being substituents ranging from aliphatic, hetero/aromatic ring to TMS functionality (3o-t). The structure of 2h (CCDC: 2126012) and 3h (CCDC: 2126018) were unambiguously confirmed through X-ray crystallographic analysis, for details, see supporting information.
Synthetic Applications
The isocoumarin skeleton commonly exists in many naturally-occurring compounds and potent pharmaceutical agents displaying anti-tumor, antifungal, and anti-inflammatory properties (Pal and Pal, 2019). For instance, Xyridin A (Ruangrungsi et al., 1995) was isolated in 1995 from Xyris indica, and was found to possess antibacterial activity against various bacteria (Saeed, 2003). To further demonstrate the synthetic utility of our method, a gram-scale reaction was performed with substrate 1u under standard conditions and the corresponding C4 thio/selenocyanated Xyridin A derivatives 2u and 3u were obtained in 87 and 90%, respectively (Scheme 2). Ultimately, compounds 2u and 3u could be readily transformed into SCF3- (Liang et al., 2015) and SeCF3-containing Xyridin A derivatives 2v and 3v by treatment with TMSCF3 and Cs2CO3 in acetonitrile. Moreover, [3 + 2] cycloaddition of compounds 2u/3u with sodium azide was also performed to afford Xyridin A bearing thiotetrazole moiety 2w/3w in 94 and 96% yields (Liang et al., 2015), respectively.
Investigation of Mechanism
We have previously postulated that the reaction of PhICl2 with NH4SCN could generate PhI(SCN)2 as a reactive hypervalent iodine (III) species via a ligand-exchange process (Scheme 3). In order to further corroborate whether PhI(SCN)2 species was indeed formed, the reaction of TolICl2 with NH4SCN in CDCl3 was carried out and 1H NMR analysis was implemented. The outcome revealed that only the peak of 2.46 ppm (s), which can be attributed to the methyl group of TolICl2, and the peak of 2.29 ppm(s), which can be attributed to the methyl group of p-iodotoluene, were observed throughout the whole reaction process (see SI for details). No signal from PhI(SCN)2 was detected, though. In order to further understand the most appropriate pathway adopted by the reaction between PhICl2 with NH4SCN, a computational study was carried out and the result is shown in Scheme 3. The reaction pathway b involving formation of PhI(SCN)2 obviously requires more energy than other pathways. Even though pathway a is also theoretically possible, the 1H NMR experiment result did not support the formation of PhICl(SCN). It is not the reaction pathway with the lowest energy, either. Pathway c with the formation of ClSCN is not only consistent with the 13C NMR experiment (Tao et al., 2021), but also preferred thermodynamically (see SI for details).
Based on these newly gained experimental (control experiments see ESI, Supplementary Scheme S1, SupplementaryFigure S1, S2 and computational calculation) and computational results as well as previous literature reports (Kita et al., 1997; Woon et al., 2006; Ito et al., 2019; Xing et al., 2019a; An et al., 2020), a possible mechanistic pathway for the formation of the C4 thiocyanated isocumarins was proposed (Scheme 4). Differing from the previous mechanism (Kita et al., 1997), we tentatively proposed that intermediate A, an ionic form of PhICl2, reacted with thiocyanate directly to give the reactive thiocyanogen chloride, which could be supported by the observation of peak of 109.1 ppm in its 13C NMR analysis (Tao et al., 2021). Then the reaction of thiocyanate with thiocyanogen chloride provides (SCN)2, which further reacts with the oxidative PhICl2 to give thiocyanogen chloride (Tao et al., 2021). Next, electrophilic addition between the reactive thiocyanogen chloride with substrate 1a gave rise to intermediate B (Xing et al., 2019a; An et al., 2020; Hellwig et al., 2020; Lynch and Scanlan, 2020; Sun et al., 2020; Jurinic et al., 2021; Slivka and Onysko, 2021). Due to the presence of the adjacent electron-withdrawing methoxycarbonyl group which makes the C (sp2) connecting with the Ph substituent more electron-deficient, a favored intramolecular 6-exo cyclization occurred in intermediate B, leading to formation of the cyclic intermediate C. Finally, removal of the methyl group by the nucleophilic attack of chloride ion gave the title product 2a (Scheme 4).
CCK-8 Assay
Finally, those synthesized 4-thio/selenocyanated isocoumarins were screened in vitro for antitumor activity test using CCK-8 assay against HCT 116 and MCF 7 cell lines (for details, see the ESI, Supplementary Figure S5). The results in Figure 2 indicated that these compounds showed moderate activity against HCT 116 after 48 h exposure. Compound 2l was found to be the most potent candidate of the series, which exerted 69% inhibition at 10 μM concentration against HCT 116. Moreover, the screening results revealed that those compounds were active against MCF 7 cell line. Especially, compound 2f, 3h, and 3r seem to be equally potent with 84, 82, and 81% antiproliferative inhibition at 10 μM concentration against MCF 7 cell line, respectively.
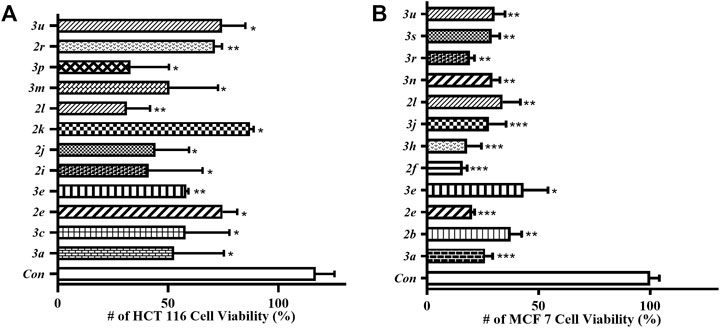
FIGURE 2. Antitumor activity of synthesized 4-thio/selenocyanated isocoumarins (10 μM) against HCT 116 (A) and MCF 7 (B) cell lines, determined by a CCK-8 assay.
Conclusion
In summary, we have realized an alternative synthesis of C4 thio/selenocyanated isocoumarins in a highly regioselective manner with good to excellent yields. Compared with the previous direct C–H functionalization/thiocyanation of a heterocyclic skeleton, this method realized the construction of the functionalized isocoumarin framework via a hypervalent iodine-mediated electrophilic thio/selenocyanation approach. In addition to the features of metal-free conditions, mild reaction conditions, high-yielding of products and broad tolerance of functional groups, the obtained thio/selenocyanated isocoumarins were found to possess anti-tumor activities and have been proven to be useful building blocks, as the functionalized Xyridin A could be converted to other pharmaceutically interesting Xyridin A derivatives.
Materials and Methods
Reagents and solvents were purchased as reagent grade and were used without further purification. PhICl2 (Zhao and Zhang, 2007) were prepared according to literature methods. All reactions were performed in standard glassware, heated at 70°C for 3 h before use. Flash column chromatography was performed over silica gel (200–300°mesh) using a mixture of ethyl acetate (EtOAc), and petroleum ether (PE).
Experimental Details General Procedure for tbl2
To an oven-dried 25 ml round-bottom flask were added NH4SCN or KSeCN (0.4 mmol), PhICl2 (0.4 mmol) and DCE (5 ml). The mixture was stirred at rt for 30 min. Then, substrate 1 (0.2 mmol) in DCE (5 ml) was added to the reaction mixture in one portion. The reaction was heated to 50°C in an aluminum heating block and stirred for another 2 h, poured into the saturated brine solution (20 ml). The product was extracted with DCM (20 ml), dried with Na2SO4 and concentrated. The crude product was purified using silica gel column chromatography.Table 2
Data Availability Statement
The original contributions presented in the study are included in the article/Supplementary Material, further inquiries can be directed to the corresponding authors.
Author Contributions
YD and JY conceived the research and supervised the whole work. ST performed synthesis and characterization of the target compounds and evaluated the anti-antitumor activity test using CCK-8 assay against HCT 116 and MCF 7 cell lines. AH and JY performed the DFT computation of possible reaction pathways to (SCN)2. YG and XZ particopated in data analysis. All authors contributed to the manuscripted writing and editing, and approved the submitted version.
Funding
This research work was financially supported by a grant from the National Natural Science Foundation of China (#22071175), the Department of Education of Hebei Province Foundation (No. QN2019220).
Conflict of Interest
The authors declare that the research was conducted in the absence of any commercial or financial relationships that could be construed as a potential conflict of interest.
Publisher’s Note
All claims expressed in this article are solely those of the authors and do not necessarily represent those of their affiliated organizations, or those of the publisher, the editors and the reviewers. Any product that may be evaluated in this article, or claim that may be made by its manufacturer, is not guaranteed or endorsed by the publisher.
Supplementary Material
The Supplementary Material for this article can be found online at: https://www.frontiersin.org/articles/10.3389/fchem.2022.859995/full#supplementary-material
References
An, X., Zhang, B., Li, X., Du, T., Ai, Z., Zhang, C., et al. (2020). Construction of 4-(Methylthio)isochromenones Skeleton through Regioselective Intramolecular Cyclization of 2-Alkynylbenzoate Mediated by DMSO/[D6 ]DMSO and SOCl2. Eur. J. Org. Chem. 2020, 852–859. doi:10.1002/ejoc.201901723
Borges, V. C., Rocha, J. B. T., and Nogueira, C. W. (2005). Effect of Diphenyl Diselenide, Diphenyl Ditelluride and Ebselen on Cerebral Na+, K+-ATPase Activity in Rats. Toxicology 215, 191–197. doi:10.1016/j.tox.2005.07.002
Castanheiro, T., Suffert, J., Donnard, M., and Gulea, M. (2016). Recent Advances in the Chemistry of Organic Thiocyanates. Chem. Soc. Rev. 45, 494–505. doi:10.1039/C5CS00532A
Chen, K.-M., Spratt, T. E., Stanley, B. A., De Cotiis, D. A., Bewley, M. C., Flanagan, J. M., et al. (2007). Inhibition of Nuclear Factor-Κb DNA Binding by Organoselenocyanates through Covalent Modification of the P50 Subunit. Cancer Res. 67, 10475–10483. doi:10.1158/0008-5472.Can-07-2510
Chen, Y., Chen, Y.-J., Guan, Z., and He, Y.-H. (2019). Visible-Light-Mediated Selective Thiocyanation/ipso-Cyclization/Oxidation Cascade for the Synthesis of Thiocyanato-Containing Azaspirotrienediones. Tetrahedron 75, 130763. doi:10.1016/j.tet.2019.130763
Elhalem, E., Bailey, B. N., Docampo, R., Ujváry, I., Szajnman, S. H., and Rodriguez, J. B. (2002). Design, Synthesis, and Biological Evaluation of Aryloxyethyl Thiocyanate Derivatives against Trypanosoma Cruzi. J. Med. Chem. 45, 3984–3999. doi:10.1021/jm0201518
Feng, C., Peng, Y., Ding, G., Li, X., Cui, C., and Yan, Y. (2018). Catalyst and Additive-free Regioselective Oxidative C-H Thio/selenocyanation of Arenes and Heteroarenes with Elemental Sulfur/selenium and TMSCN. Chem. Commun. 54, 13367–13370. doi:10.1039/C8CC07905F
Glenadel, Q., Ismalaj, E., and Billard, T. (2018). A Metal-free Route to Heterocyclic Trifluoromethyl- and Fluoroalkylselenolated Molecules. Org. Lett. 20, 56–59. doi:10.1021/acs.orglett.7b03338
Hellwig, P. S., Peglow, T. J., Penteado, F., Bagnoli, L., Perin, G., and Lenardão, E. J. (2020). Recent Advances in the Synthesis of Selenophenes and Their Derivatives. Molecules 25, 5907. doi:10.3390/molecules25245907
Hirota, H., Tomono, Y., and Fusetani, N. (1996). Terpenoids with Antifouling Activity against Barnacle Larvae from the Marine Sponge Acanthella Cavernosa. Tetrahedron 52, 2359–2368. doi:10.1016/0040-4020(95)01079-3
Ip, C., and Ganther, H. E. (1992). Comparison of Selenium and Sulfur Analogs in Cancer Prevention. Carcinogenesis 13, 1167–1170. doi:10.1093/carcin/13.7.1167
Ito, Y., Touyama, A., Uku, M., Egami, H., and Hamashima, Y. (2019). Thiocyanation of Aromatic and Heteroaromatic Compounds with 1-Chloro-1,2-Benziodoxol-3-(1h)-One and (Trimethylsilyl)Isothiocyanate. Chem. Pharm. Bull. 67, 1015–1018. doi:10.1248/cpb.c19-00352
Ji, Y., Akerboom, T. P. M., Sies, H., and Thomas, J. A. (1999). S-nitrosylation and S-Glutathiolation of Protein Sulfhydryls byS-Nitroso Glutathione. Arch. Biochem. Biophys. 362, 67–78. doi:10.1006/abbi.1998.1013
Jiang, G., Zhu, C., Li, J., Wu, W., and Jiang, H. (2017). Silver-Catalyzed Regio- and Stereoselective Thiocyanation of Haloalkynes: Access to (Z)-Vinyl Thiocyanates. Adv. Synth. Catal. 359, 1208–1212. doi:10.1002/adsc.201601142
Kita, Y., Takeda, Y., Okuno, T., Egi, M., Iio, K., Kawaguchi, K.-i., et al. (1997). An Efficient P-Thiocyanation of Phenols and Naphthols Using a Reagent Combination of Phenyliodine Dichloride and Lead(II) Thiocyanate. Chem. Pharm. Bull. 45, 1887–1890. doi:10.1248/cpb.45.1887
Liang, Z., Wang, F., Chen, P., and Liu, G. (2015). Copper-Catalyzed Intermolecular Trifluoromethylthiocyanation of Alkenes: Convenient Access to CF3-Containing Alkyl Thiocyanates. Org. Lett. 17, 2438–2441. doi:10.1021/acs.orglett.5b00939
Lin, X., Fang, Z., Zeng, C., Zhu, C., Pang, X., Liu, C., et al. (2020). Continuous Electrochemical Synthesis of Iso‐Coumarin Derivatives from O ‐(1‐Alkynyl) Benzoates under Metal‐ and Oxidant‐Free. Chem. Eur. J. 26, 13738–13742. doi:10.1002/chem.202001766
Liu, l., Li, S., Li, X., Zhong, M., Lu, Y., Jiajie, Y., et al. (2018). Synthesis of NSAIDs-Se Derivatives as Potent Anticancer Agents. Med. Chem. Res. 27, 2071–2078. doi:10.1007/s00044-018-2216-7
Lu, L.-H., Zhou, S.-J., He, W.-B., Xia, W., Chen, P., Yu, X., et al. (2018). Metal-Free Difunctionalization of Alkynes Leading to Alkenyl Dithiocyanates and Alkenyl Diselenocyanates at Room Temperature. Org. Biomol. Chem. 16, 9064–9068. doi:10.1039/C8OB02368A
Lynch, D. M., and Scanlan, E. M. (2020). Thiyl Radicals: Versatile Reactive Intermediates for Cyclization of Unsaturated Substrates. Molecules 25, 3094. doi:10.3390/molecules25133094
Mampuys, P., McElroy, C. R., Clark, J. H., Orru, R. V. A., and Maes, B. U. W. (2020). Thiosulfonates as Emerging Reactants: Synthesis and Applications. Adv. Synth. Catal. 362, 3–64. doi:10.1002/adsc.201900864
Mehta, S., Waldo, J. P., and Larock, R. C. (2009). Competition Studies in Alkyne Electrophilic Cyclization Reactions. J. Org. Chem. 74, 1141–1147. doi:10.1021/jo802196r
Meng, F., Zhang, H., He, H., Xu, N., Fang, Q., Guo, K., et al. (2020). Copper‐Catalyzed Domino Cyclization/Thiocyanation of Unactivated Olefins: Access to SCN‐Containing Pyrazolines. Adv. Synth. Catal. 362, 248–254. doi:10.1002/adsc.201901104
Mugesh, G., du Mont, W.-W., and Sies, H. (2001). Chemistry of Biologically Important Synthetic Organoselenium Compounds. Chem. Rev. 101, 2125–2180. doi:10.1021/cr000426w
Nadiveedhi, M. R., Cirandur, S. R., and Akondi, S. M. (2020). Visible-Light-Promoted Photocatalyst- and Additive-free Intermolecular Trifluoromethyl-Thio(Seleno)Cyanation of Alkenes. Green. Chem. 22, 5589–5593. doi:10.1039/D0GC01726D
Pal, S., and Pal, M. (2019). “Chapter 5 - Isocoumarins in Medicinal Chemistry and Organic Synthesis,” in Isocoumarin, Thiaisocoumarin and Phosphaisocoumarin. Editors S. Pal, and M. Pal (Elsevier), 153–176. doi:10.1016/b978-0-12-815411-3.00005-9
Parnham, M. J., and Graf, E. (1991). Pharmacology of Synthetic Organic Selenium Compounds. Prog. Drug Res. 36, 9–47. doi:10.1007/978-3-0348-7136-5_1
Piña, I. C., Gautschi, J. T., Wang, G.-Y. -S., Sanders, M. L., Schmitz, F. J., France, D., et al. (2003). Psammaplins from the Sponge Pseudoceratina Purpurea: Inhibition of Both Histone Deacetylase and DNA Methyltransferase. J. Org. Chem. 68, 3866–3873. doi:10.1021/jo034248t
Plano, D., Karelia, D. N., Pandey, M. K., Spallholz, J. E., Amin, S., and Sharma, A. K. (2016). Design, Synthesis, and Biological Evaluation of Novel Selenium (Se-NSAID) Molecules as Anticancer Agents. J. Med. Chem. 59, 1946–1959. doi:10.1021/acs.jmedchem.5b01503
Prakash, O., Sharma, V., Batra, H., and Moriarty, R. M. (2001). (Dichloroiodo)benzene and Lead(II) Thiocyanate as an Efficient Reagent Combination for Stereoselective 1,2-dithiocyanation of Alkynes. Tetrahedron Lett. 42, 553–555. doi:10.1016/S0040-4039(00)01909-2
Rezayati, S., and Ramazani, A. (2020). A Review on Electrophilic Thiocyanation of Aromatic and Heteroaromatic Compounds. Tetrahedron 76, 131382. doi:10.1016/j.tet.2020.131382
Ruangrungsi, N., Sekine, T., Phadungcharoen, T., Suriyagan, S., and Murakoshi, I. (1995). Isocoumarins from Xyris Indica. Phytochemistry 38, 481–483. doi:10.1016/0031-9422(94)00714-5
Saeed, A. (2003). Synthesis and Bioactivity of Xyridin A and B, Metabolites Fromxyris Indica. J. Heterocycl. Chem. 40, 337–340. doi:10.1002/jhet.5570400222
Santos, D. B., Schiar, V. P. P., Paixão, M. W., Meinerz, D. F., Nogueira, C. W., Aschner, M., et al. (2009). Hemolytic and Genotoxic Evaluation of Organochalcogens in Human Blood Cells In Vitro. Toxicol. Vitro 23, 1195–1204. doi:10.1016/j.tiv.2009.05.010
Schumacher, R. F., Godoi, B., Jurinic, C. K., and Belladona, A. L. (2021). Diorganyl Dichalcogenides and Copper/Iron Salts: Versatile Cyclization System to Achieve Carbo- and Heterocycles from Alkynes. Synthesis 53, 2545–2558. doi:10.1055/a-1463-4098
Slivka, M., and Onysko, M. (2021). The Use of Electrophilic Cyclization for the Preparation of Condensed Heterocycles. Synthesis 53, 3497–3512. doi:10.1055/s-0040-1706036
Sperança, A., Godoi, B., Pinton, S., Back, D. F., Menezes, P. H., and Zeni, G. (2011). Regioselective Synthesis of Isochromenones by Iron(III)/Phseseph-Mediated Cyclization of 2-Alkynylaryl Esters. J. Org. Chem. 76, 6789–6797. doi:10.1021/jo201211s
Stang, P. J., and Zhdankin, V. V. (1996). Organic Polyvalent Iodine Compounds. Chem. Rev. 96, 1123–1178. doi:10.1021/cr940424+
Sun, K., Wang, X., Li, C., Wang, H., and Li, L. (2020). Recent Advances in Tandem Selenocyclization and Tellurocyclization with Alkenes and Alkynes. Org. Chem. Front. 7, 3100–3119. doi:10.1039/D0QO00849D
Tao, S., Xiao, J., Li, Y., Sun, F., and Du, Y. (2021). PhICl 2/NH 4 SCN‐Mediated Oxidative Regioselective Thiocyanation of Pyridin‐2( 1 H )‐ones. Chin. J. Chem. 39, 2536–2546. doi:10.1002/cjoc.202100278
Wang, J., Wang, Y. Z., Liu, Y. J., Yan, X. X., Yan, Y. H., Chao, S. J., et al. (2022). Synthesis of Isoquinolylselenocyanates and Quinolylselenocyanates via Electrophilic Selenocyanogen Cyclization Induced by Pseudohalogen (SeCN) 2 Generated In Situ. Adv. Synth. Catal. 364, 187–192. doi:10.1002/adsc.202101169
Wilkins, L. C., Günther, B. A. R., Walther, M., Lawson, J. R., Wirth, T., and Melen, R. L. (2016). Contrasting Frustrated Lewis Pair Reactivity with Selenium- and Boron-Based Lewis Acids. Angew. Chem. Int. Ed. 55, 11292–11295. doi:10.1002/anie.201605239
Willgerodt, C. (1885). Ueber Einige Aromatische Jodidchloride. J. Prakt. Chem. 33, 154–160. doi:10.1002/prac.18860330117
Woon, E. C. Y., Dhami, A., Mahon, M. F., and Threadgill, M. D. (2006). 5-Nitroisocoumarins from Tandem Castro-Stephens Coupling-6-Endo-Dig Cyclisation of 2-Iodo-3-Nitrobenzoic Acid and Arylethynes and Ring-Closure of Methyl 2-Alkynyl-3-Nitrobenzoates with Electrophiles. Tetrahedron 62, 4829–4837. doi:10.1016/j.tet.2006.03.005
Wu, C., Lu, L.-H., Peng, A.-Z., Jia, G.-K., Peng, C., Cao, Z., et al. (2018). Ultrasound-promoted Brønsted Acid Ionic Liquid-Catalyzed Hydrothiocyanation of Activated Alkynes under Minimal Solvent Conditions. Green. Chem. 20, 3683–3688. doi:10.1039/C8GC00491A
Wu, D., Duan, Y., Liang, K., Yin, H., and Chen, F.-X. (2021). AIBN-initiated Direct Thiocyanation of Benzylic Sp3 C-H with N-Thiocyanatosaccharin. Chem. Commun. 57, 9938–9941. doi:10.1039/d1cc04302a
Xiao, J., Ai, Z., Li, X., Tao, S., Zhao, B., Wang, X., et al. (2021). Synthesis of 3-thiocyanated Chromones via TCCA/NH4SCN-mediated Cyclization/thiocyanation of Alkynyl Aryl Ketones. Green. Synth. Catal. 1, 1. in press. doi:10.1016/j.gresc.2021.12.003
Xing, L., Zhang, Y., Li, B., and Du, Y. (2019a). In Situ Formation of RSCl/ArSeCl and Their Application to the Synthesis of 4-Chalcogenylisocumarins/Pyrones from O-(1-Alkynyl)benzoates and (Z)-2-Alken-4-ynoates. Org. Lett. 21, 3620–3624. doi:10.1021/acs.orglett.9b01046
Xing, L., Zhang, Y., Li, B., and Du, Y. (2019b). Synthesis of 4-Chloroisocoumarins via Intramolecular Halolactonization of O-Alkynylbenzoates: PhICl2-Mediated C-O/C-Cl Bond Formation. Org. Lett. 21, 1989–1993. doi:10.1021/acs.orglett.9b00047
Yang, H., Duan, X.-H., Zhao, J.-F., and Guo, L.-N. (2015). Transition-Metal-Free Tandem Radical Thiocyanooxygenation of Olefinic Amides: A New Route to SCN-Containing Heterocycles. Org. Lett. 17, 1998–2001. doi:10.1021/acs.orglett.5b00754
Yao, T., and Larock, R. C. (2003). Synthesis of Isocoumarins and α-Pyrones via Electrophilic Cyclization. J. Org. Chem. 68, 5936–5942. doi:10.1021/jo034308v
Ye, A.-H., Zhang, Y., Xie, Y.-Y., Luo, H.-Y., Dong, J.-W., Liu, X.-D., et al. (2019). TMSCl-Catalyzed Electrophilic Thiocyano Oxyfunctionalization of Alkenes Using N-Thiocyano-Dibenzenesulfonimide. Org. Lett. 21, 5106–5110. doi:10.1021/acs.orglett.9b01706
Yuan, Y., Li, L.-S., Zhang, L., Wang, F., Jiang, L., Zuo, L., et al. (2021). Electrochemical Synthesis of Versatile Ammonium Oxides under Metal Catalyst-, Exogenous-Oxidant-, and Exogenous-electrolyte-free Conditions. Chem. Commun. 57, 2768–2771. doi:10.1039/d1cc00486g
Zeng, F.-L., Zhu, H.-L., Chen, X.-L., Qu, L.-B., and Yu, B. (2021). Visible Light-Induced Recyclable G-C3n4 Catalyzed Thiocyanation of C(sp2)-H Bonds in Sustainable Solvents. Green. Chem. 23, 3677–3682. doi:10.1039/D1GC00938A
Zeng, X., and Chen, L. (2018). Iodine-Mediated Regio- and Stereoselective Iodothiocyanation of Alkynes in Aqueous Ethanol. Org. Biomol. Chem. 16, 7557–7560. doi:10.1039/C8OB02216J
Zhang, C., and Zhao, X.-F. (2007). Iodobenzene Dichloride as a Stoichiometric Oxidant for the Conversion of Alcohols into Carbonyl Compounds; Two Facile Methods for its Preparation. Synthesis 2007, 551–557. doi:10.1055/s-2007-965889
Keywords: PhICl2, oxythiocyanation, oxyselenocyanation, o-(1-Alkynyl)benzoate, isocoumarin
Citation: Tao S, Huo A, Gao Y, Zhang X, Yang J and Du Y (2022) PhICl2-Mediated Regioselective and Electrophilic Oxythio/Selenocyanation of o-(1-Alkynyl)benzoates: Access to Biologically Active S/SeCN-Containing Isocoumarins. Front. Chem. 10:859995. doi: 10.3389/fchem.2022.859995
Received: 22 January 2022; Accepted: 28 February 2022;
Published: 18 May 2022.
Edited by:
Jian-Wei Han, East China University of Science and Technology, ChinaReviewed by:
Kazuaki Ishihara, Nagoya University, JapanZhiyuan Chen, Jiangxi Normal University, China
Chi Zhang, Nankai University, China
Copyright © 2022 Tao, Huo, Gao, Zhang, Yang and Du. This is an open-access article distributed under the terms of the Creative Commons Attribution License (CC BY). The use, distribution or reproduction in other forums is permitted, provided the original author(s) and the copyright owner(s) are credited and that the original publication in this journal is cited, in accordance with accepted academic practice. No use, distribution or reproduction is permitted which does not comply with these terms.
*Correspondence: Yunfei Du, ZHV5dW5mZWllckB0anUuZWR1LmNu; Jingyue Yang, eWFuZ2ppbmd5dWVAeXN1LmVkdS5jbg==