- Key Laboratory for Green Processing of Chemical Engineering of Xin-Jiang Bingtuan, School of Chemistry and Chemical Engineering, Shihezi University, Shihezi, China
The picolinamide-supported tetracoordinated organoboron complexes containing diaryl boronyl segments have been synthesized for the first time. Aryl trifluoroborates were utilized as the BAr2 sources to introduce different aryl motifs with diverse functional groups. The optical experiments discovered these five-membered boron-containing complexes were aggregation-induced emission (AIE) active, thus affording a new class of AIE molecules.
Introduction
Taking advantage of the vacant p-orbitals of the boron atoms, the chelating complexation between π-conjugated ligands and -BR2 units will generate tetracoordinated organoboron complexes containing X→B dative bonds that are able to lock the π-conjugated ligands. These modifications will enhance the molecular rigidity, extend the π-conjugation system, and thus afford molecules with superior photoluminescence properties (Chen et al., 2017; Dou et al., 2017; Mellerup and Wang, 2019; Yan et al., 2019; Huang et al., 2020). Therefore, over the past decades, the synthesis, improvement, and application of these tetracoordinated organoboron complexes have attracted a continuing interest from organic and materials communities. However, the emission of these planar structures in aggregates or the solid-state is usually spoiled due to the aggregation-caused quenching (ACQ) effects. This limits their applications in organic optoelectronic materials or other situations that demand solid-state emissions, such as organic lasers and organic light-emitting diodes (OLEDs), which necessitates the development of new series of these complexes with high solid-state luminescence efficiency.
Since the conceptualization of the aggregation-induced emission (AIE) effect by the Tang group in 2001 (Luo et al., 2001; Mei et al., 2015), many types of AIE-active compounds/units have been developed. In recent years, a number of AIE-active tetracoordinated organoboron complexes have been synthesized and applied as aggregate-state emitters (Virgili et al., 2013; Liu et al., 2019; Nitti et al., 2020; Ito et al., 2021; Yin et al., 2021; Min et al., 2022). These AIE-active complexes, which are usually obtained via linking AIE-active units such as tetraphenylethylene (TPE) or introducing rotational aryls onto the chelating backbones, mostly contain BF2 segments. The introduction of BAr2 segments, which had two bulky aryl rings on boron atoms, has been utilized to disrupt the intermolecular π−π stacking in aggregate states and thus enable more efficient AIE effects (Figure 1). Based on our survey, most of the known AIE-active complexes with BAr2 segments were six-membered. To the best of our knowledge, the only AIE-active five-membered cases have been disclosed very recently by Yang and Zou group (Qi et al., 2021). This was surprising since five-membered tetracoordinated organoboron compounds were very commonly encountered in organoboron-based molecules and materials. Moreover, BAr2 segments were usually introduced via Ar3B or aryl organometallic reagents (Kubota et al., 2012; Yan et al., 2014; Gong et al., 2015; Wang et al., 2015; Wu et al., 2015), limiting the accessibility and diversity of the target molecules (Yang et al., 2021).
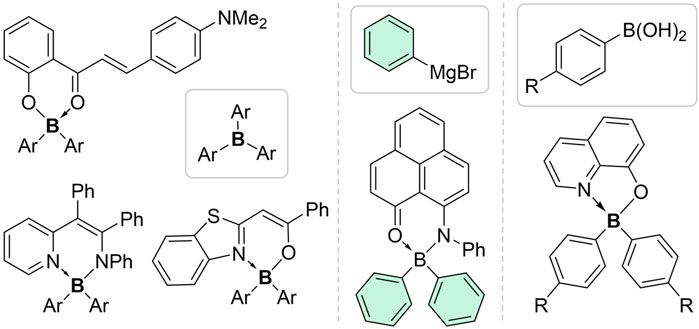
FIGURE 1. The tetracoordinated organoboron complexes containing BAr2 segments with AIE properties. The aryl precursors are indicated in the boxes.
On the other hand, innumerable chelating backbones have been applied in the complexation formation of tetracoordinated organoboron, to fine-tune the desirable luminescence properties. In this regard, it is surprising that picolinamide, one of the most available and widely-used chelating ligands in coordination chemistry, has never been used in the preparation of tetracoordinated organoboron, to the best of our knowledge (Yang et al., 2021). Thus, we hypothesized that complexation between picolinamide and BAr2 segments would afford a new series of tetracoordinated organoboron molecules. These complexes should adopt propeller structures and be consisted of electron-deficient pyridyl, electron-rich anilino groups, and the spiro linker centers of boron atoms, which potentially circumvent the trade-off between the complexation stability and the solid-state luminescence, leading to some special light-emitting molecules (Shiu et al., 2016). The proposal would pose at least two major challenges: 1) to properly introduce the relatively flexible picolinamide ligands and 2) to bypass the traditional organometallic BAr2 incorporation pathways.
Results and Discussion
With these considerations in mind, the investigation was initiated by exploring the reaction between N-phenylpyridinecarboxamide 1a and potassium phenyltrifluoroborate 2a (Sawazaki et al., 2018; Wang et al., 2019), which has been demonstrated to be a competent BPh2 provider recently by Song group (Yang et al., 2018) and our group (Zu et al., 2020) (Table 1). After systematic evaluation of reaction variables, to our delight, under similar reaction conditions with our previous work (Zu et al., 2020), the target product 3a could be isolated in 99% yield by using Mn (1.0 equiv), p-toluenesulfonyl chloride (TsCl, 2.5 equiv) and Na2CO3 (0.5 equiv) in acetonitrile (CH3CN) at 130°C for 24 h (entry 1). The propeller structure of 3a was confirmed unambiguously via X-ray diffraction analysis of its crystals (Table 2).
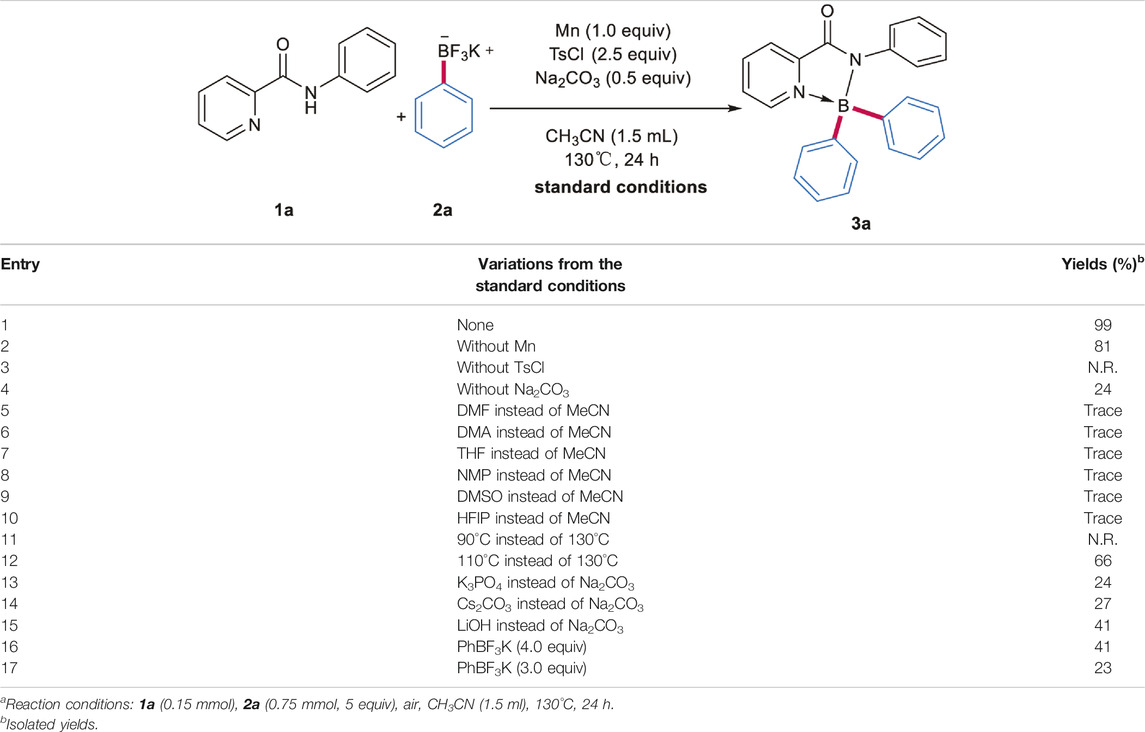
TABLE 1. Optimization of reaction conditionsa.
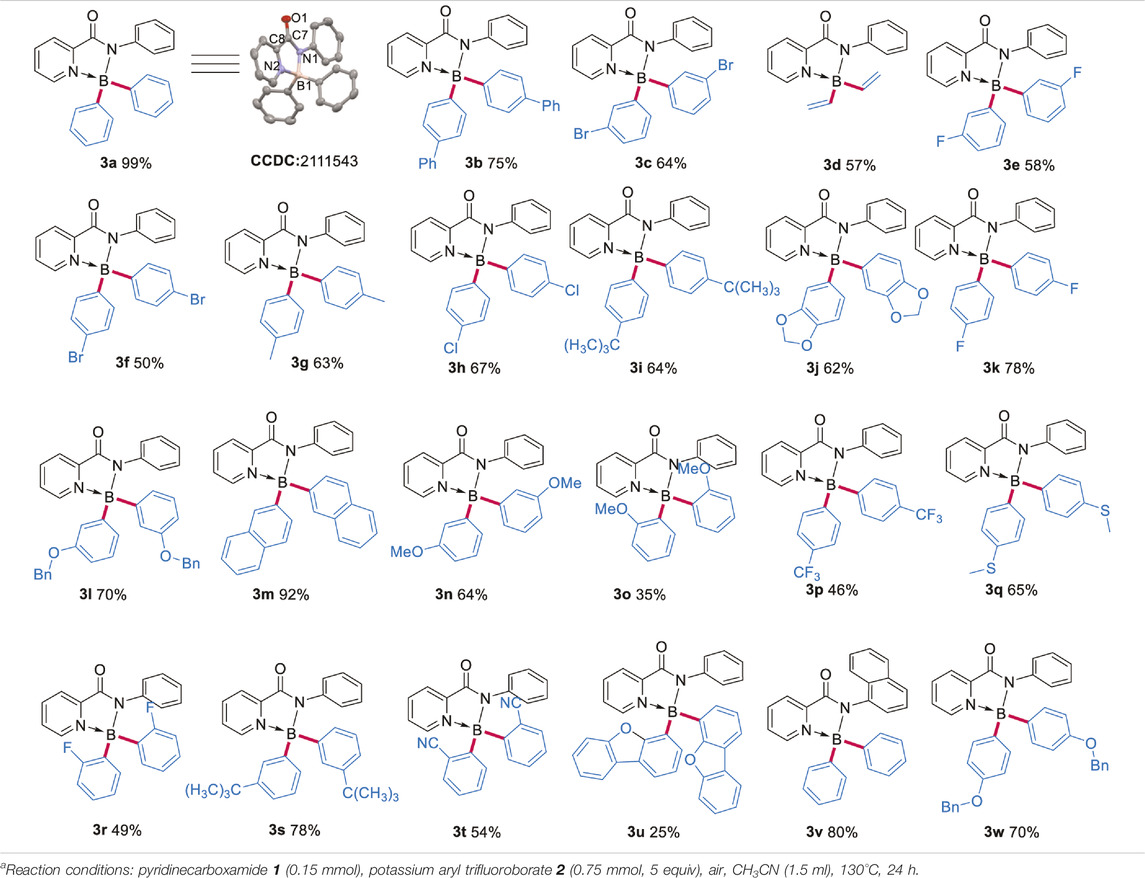
TABLE 2. Optimization of reaction conditionsa.
Subsequently, control experiments were performed to elucidate the function of Mn, TsCl and Na2CO3 in these conditions (entry 2–4). It was found TsCl played a vital role in the formation of 3a, in view that its absence would suppress the reaction totally. Omitting Mn from the conditions, still, 81% isolated yield of 3a could be obtained, while the omission of Na2CO3 led to the formation of 3a in only 24% yield. Further evaluation revealed the choice of solvent was also critical (entry 5–10). Other commonly-used organic solvents, including DMF, THF and DMSO, afforded only a trace amount of product 3a. Moreover, the test of the reaction temperature showed the yield dropped sharply while lowering the temperature (entries 11–12). The investigation of a variety of bases showed that Na2CO3 performed best (entries 13–15). The reduction of the amount of 2a also affected the yields remarkably (entries 16–17).
With the optimal conditions in hand, the substrate scope of this methodology was then investigated (Table 2). A broad scope of aryl trifluoroborates containing para- ortho- and meta-substituents were applied in the reactions successfully. Generally, the ortho-substituted aryl trifluoroborates gave lower isolated yields, in comparison with their para- and meta-substituted analogs, mainly due to the steric effect (3e, 58% vs. 3k, 78% vs. 3r, 49%; 3n, 64% vs. 3o, 35%). The reaction tolerated bromides and chlorides well (3c, 3f, 3h), allowing the formation of halogenated products and thus leaving ample space for further decorations. No matter electron-withdrawing (-CF3, -halides, -CN) or -donating (-OMe, -tBu, -OBn) groups were on the aryl trifluoroborates, the target boronyl complexes could be obtained in moderate to good yields. While aryl substrates containing π-extended systems were applied, products with more congested BAr2 segments, like 3b, 3m and 3u, were obtained. Notably, potassium vinyl fluoroborate proceeded smoothly to provide 3d in moderate yields. When the N-substituent of pyridinecarboxamide was changed to 1-naphthyl, 80% of 3v could be isolated.
Then, photophysical properties of the obtained complexes in solvent and in the solid-state were measured. As shown in Figure 2 and Table 3, the selected complexes possessed very weak luminescence in organic solvent DMF along with very low quantum yield (Φliquid < 0.015), probably due to the non-radiative process in the solvent induced by intramolecular rotation. In contrast, the solid-state spectra of the BAr2-species showed intense emission bands centered around 490 nm with high quantum yield (Φsolid: 31–98%), indicating the existence of AIE phenomenon and the absence of strong intermolecular π-π interactions in the solid-state.
Furthermore, the emission properties of 3a were investigated in detail to prove its AIE behaviors (Figure 3). Firstly, under irradiation with a UV lamp at 365 nm, solid 3a showed macroscopical fluorescence (Figure 3A). Then, 3a was dissolved in H2O/MeOH mixtures with different H2O content (Figure 3C). When the H2O content is lower than 60%, 3a was dissolved totally and the solution was transparent without observable fluorescence. As the water content increased from 80 to 90%, 3a became insoluble in the solvents and began to aggregate. Correspondingly, the turbidity and luminescence (at 365 nm) of the solution increased, which could be observed with the naked eyes. 3a would disperse in the pure water with its aggregation showing the same luminescence as the solid-state. The AIE behavior of 3a was further verified by observing the fluorescent changes during the evaporation of MeOH and THF solution of 3a (Figure 3B). On a thin-layer chromatography plate, as the solvent evaporated and 3a molecules aggregated, a clear increase in the fluorescence was observed under UV irradiation at 365 nm.
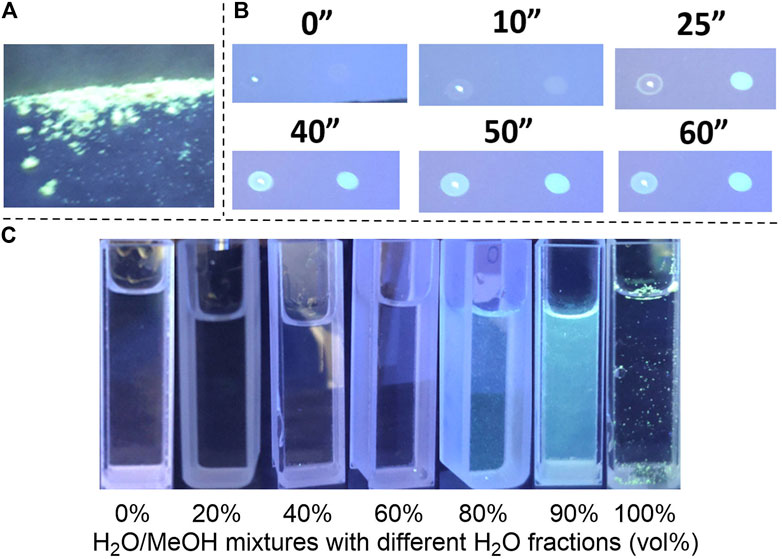
FIGURE 3. AIE behaviours of 3a. (A) 3a solid at 365 nm. (B) Fluorescence comparison of 3a solution at 365 nm at different time. (C) Fluorescence comparison of 3a at 365 nm in different ratios of water/methanol.
In order to better understand the AIE properties of these complexes, X–ray crystallographic analysis of 3a was performed. As shown in Figure 4, the boron atoms of 3a adopted a typical tetrahedral geometry to form N,N–chelated five-membered rings. The pyridyl N→B bond lengths are around 0.03 Å longer than the amide N–B bonds. As anticipated, 3a adopt twisted conformations and the two phenyl rings on the boron atom were not coplanar. The dihedral angles of A and B, A and C, B and C were 76.50°, 78.02° and 61.95°, respectively. As for the packing mode, to take advantage of the capacious space around the planar picolinamide motif, two 3a molecules could assemble in the head-to-tail mode. The aryl hydrogens on the B rings could interact with the carbonyl oxygens of the other molecule to form H-bonding (O1 … H1, 2.85 Å; O1 … H2, 2.75 Å; O2 … H3, 2.91 Å). The aforementioned dimers would interlace zigzag to form weak intermolecular π–π interactions. The shortest distances between the aryl D and E rings were around 3.5 Å. These weak intermolecular interactions, like H-bonding and π–π stacking, were able to fix the molecular conformations in the solid-state, thus inhibiting the internal rotations and non-radiative relaxation and inducing the AIE property.
To gain additional insight, density functional theory (DFT) and time-dependent density functional theory calculations (TD-DFT) (Adamo and Jacquemin, 2013) of 3a were performed with Gaussian09 suite of programs (Frisch et al., 2009). The combination of m062x/6–311 g** was applied in both cases (Zhao and Truhlar, 2008). As shown in Figure 5, both the HOMO and LUMO orbitals were delocalized over the picolinamide backbone. However, the electron density distribution of HOMO and LUMO was mainly localized at acyl aniline motif and pyridyl group, respectively. The electron-density contribution of boron in both orbitals were negligible, being 0.80% (HOMO) and 0.48% (LUMO). The HOMO and LUMO orbitals were spatially separated by the boron atom, rendering limited overlap between these two orbitals. Then, TD-DFT calculations on the DFT-optimized structure suggested that lowest energy transition (S0→S1) involves mostly the HOMO and the LUMO orbitals, indicating a possible ligand-to-ligand charge transfer character.
In conclusion, using readily available and stable aryl trifluoroborates as the BAr2 sources, the picolinamide-based diaryl boronyl complexes have been synthesized for the first time. These propeller-type five-membered complexes exhibit very weak fluorescence in organic solvents and intense fluorescence in their aggregation/solid-state, showing classical AIE properties, which should originate from the confinement of molecular conformations and the inhibited internal rotations when these molecules assemble.
Experimental Section
General Information
Unless otherwise noted, all reactions were carried out under an air atmosphere. Analytical thin-layer chromatography (TLC) was performed on glass plates coated with 0.25 mm 230–400 mesh silica gel containing a fluorescent indicator. Visualization was accomplished by exposure to a UV lamp. All the products in this article are compatible with standard silica gel chromatography. Column chromatography was performed on silica gel (200–300 mesh). Eluent generally contained ethyl acetate (EA), petroleum ether (PE) and triethylamine (TEA).
NMR spectra were measured on a Bruker Ascend 400 spectrometer and chemical shifts (δ) are reported in parts per million (ppm). 1H NMR spectra were recorded at 400 MHz in NMR solvents and referenced internally to corresponding solvent resonance, and 13C NMR spectra were recorded at 101 MHz and referenced to corresponding solvent resonance. Coupling constants are reported in Hz with multiplicities denoted as s (singlet), d (doublet), t (triplet), q (quartet), m (multiplet) and br (broad). Infrared spectra were collected on a Thermo Fisher Nicolet 6700 FT-IR spectrometer using ATR (Attenuated Total Reflectance) method. Absorption maxima (ν max) are reported in wavenumbers (cm−1). High resolution mass spectra (HRMS) were acquired on Thermo Scientific LTQ Orbitrap XL with an ESI source. Melting points were measured with a micro-melting point apparatus.
Commercial reagents, including picolinoyl chloride hydrochloride, aniline, naphthalen-1-amine, Mn, p-toluenesulfonyl chloride, Na2CO3 and All potassium trifluoroborate, were purchased from commercial sources and used as received unless otherwise stated.
Preparation of 2-Pyridinecarboxamide
N-phenylpicolinamide (1a). The target product was prepared according to a literature procedure (Li et al., 2014). The product was isolated by flash chromatography as a white solid (1.64 g, 90%): 1H NMR (400 MHz, CDCl3) δ 10.03 (s, 1H), 8.62 (d, J = 4.4 Hz, 1H), 8.31 (d, J = 7.6 Hz, 1H), 7.96–7.87 (m, 1H), 7.82–7.76 (m, 2H), 7.51–7.45 (m, 1H), 7.43–7.36 (m, 2H), 7.15 (t, J = 7.2 Hz, 1H). 13C{1H} NMR (101 MHz, CDCl3) δ 162.0, 149.9, 148.0, 137.8, 137.7, 129.1, 126.5, 124.3, 122.4, 119.7, 77.4, 77.1, 76.7.
N-(naphthalen-1-yl)picolinamide (1v). The target product was prepared according to a literature procedure.14 The product was isolated by flash chromatography as a white solid (2.03 g, 89%): 1H NMR (400 MHz, CDCl3) δ 10.77 (s, 1H), 8.75–8.70 (m, 1H), 8.46–8.34 (m, 2H), 8.11 (d, J = 8.4 Hz, 1H), 7.98–7.89 (m, 2H), 7.71 (d, J = 8.4 Hz, 1H), 7.63–7.50 (m, 4H). 13C{1H} NMR (101 MHz, CDCl3) δ 162.3, 150.1, 148.2, 137.8, 134.1, 132.4, 128.9, 126.6, 126.4, 126.3, 126.3, 126.0, 126.0, 125.9, 125.1, 122.5, 120.8, 120.5, 119.0, 118.6, 109.7, 77.4, 77.1, 76.7.
Typical Experimental Procedures
General Procedure A
A flame-dried 25 ml vial was placed with a magnetic stir bar. Then, N-phenylpicolinamide (29.7 mg, 0.15 mmol, 1.0 equiv), potassium trifluoro(phenyl)borate (138.0 mg, 0.75 mmol, 5.0 equiv), Mn (82.0 mg, 0.15 mmol, 1.0 equiv), p-toluenesulfonyl chloride (71.5 mg, 0.375 mmol, 2.5 equiv) and Na2CO3 (79.5 mg, 0.075 mmol, 0.50 equiv) as a base, and react with acetonitrile as the reaction solvent at 130°C for 24 h. After the completion of the reaction and concentration, the crude product was purified by column chromatography (silica gel) to give the target product, using PE/EtOAc/DCM the eluent.
Preparation and characterization data for isolated products:
Compounds 3a—3w are unknown compounds. 1H/13C NMR, melting point, IR, data HRMS (ESI) m/z for these compounds are provided herein.
1,1,2-triphenyl-1,2-dihydro-3H-1λ4,8λ4-[1,3,2]diazaborolo[1,5-a]pyridin-3-one (3a). The product was isolated by flash chromatography as a yellow-green solid (53.8 mg, 99%): mp: 186.4–189.4°C; 1H NMR (400 MHz, CDCl3) δ 8.41–8.34 (m, 2H), 8.19 (td, J = 8.0, 1.2 Hz, 1H), 7.58 (dd, J = 12.4, 6.4 Hz, 1H), 7.55–7.51 (m, 2H), 7.37 (dd, J = 7.6, 4.0 Hz, 4H), 7.27–7.20 (m, 6H), 7.16 (t, J = 7.9 Hz, 2H), 7.02 (dd, J = 1.2, 7.6 Hz, 1H). 13C{1H} NMR (101 MHz, CDCl3) δ 161.4, 142.4, 141.6, 140.0, 135.8, 133.7, 128.4, 127.9, 127.3, 127.2, 125.0, 124.8, 122.5, 77.5, 77.2, 76.8. HRMS (ESI), m/z calcd for C24H19BN2ONa+ (M + Na)+ 385.1483, found 385.1485. IR (cm−1): 3,074, 3,009, 1,663, 1,489, 1,348, 762, 702.
1,1-di([1,1′-biphenyl]-4-yl)-2-phenyl-1,2-dihydro-3H-1λ4,8λ4-[1,3,2]diazaborolo[1,5-a]pyridin-3-one (3b). The product was isolated by flash chromatography as a yellow-green solid (57.9 mg, 75%): mp: 289.0–290.7°C; 1H NMR (400 MHz, CDCl3) δ 8.46 (dd, J = 15.6, 5.6 Hz, 2H), 8.28 (t, J = 7.2 Hz, 1H), 7.72–7.68 (m, 1H), 7.65–7.57 (m, 6H), 7.53–7.47 (m, 8H), 7.44–7.39 (m, 4H), 7.34–7.29 (m, 2H), 7.22 (dd, J = 7.6, 2.0 Hz, 2H), 7.06 (t, J = 7.2 Hz, 1H). 13C{1H} NMR (101 MHz, CDCl3) δ 161.3, 148.9, 142.4, 141.6, 141.2, 139.9, 139.8, 134.1, 128.7, 128.4, 127.2, 127.1, 127.0, 126.5, 125.0, 124.6, 122.5, 77.4, 77.2, 76.7. HRMS (ESI), m/z calcd for C36H27BN2ONa+ (M + Na)+ 537.2109, found 537.2111. IR (cm−1): 3,058, 3,015, 1,680, 1,495, 1,348, 740, 686.
1,1-bis(3-bromophenyl)-2-phenyl-1,2-dihydro-3H-1λ4,8λ4-[1,3,2]diazaborolo[1,5-a]pyridin-3-one (3c) The product was isolated by flash chromatography as a yellow-green solid (49.9 mg, 64%): mp: 203.5–204.5°C; 1H NMR (400 MHz, CDCl3) δ 8.48–8.40 (m, 1H), 8.38–8.28 (m, 3H), 7.78–7.72 (m, 2H), 7.43–7.35 (m, 6H), 7.25–7.19 (m, 2H), 7.17–7.08 (m, 3H). 13C{1H} NMR (101 MHz, CDCl3) δ 161.2, 148.6, 143.1, 141.6, 139.0, 135.9, 135.8, 132.0, 130.5, 129.8, 128.6, 127.6, 125.6, 124.9, 122.9, 77.4, 77.1, 76.8. HRMS (ESI), m/z calcd for C24H17BBr2N2ONa+ (M + Na)+ 542.9672, found 542.9680. IR (cm−1): 3,058, 1,674, 1,489, 1,348, 762, 692.
2-phenyl-1,1-divinyl-1,2-dihydro-3H-1λ4,8λ4-[1,3,2]diazaborolo[1,5-a]pyridin-3-one (3d) The product was isolated by flash chromatography as a yellow-green solid (22.4 mg, 57%): mp: 78.9–80.0°C; 1H NMR (400 MHz, CDCl3) δ 8.40 (d, J = 5.6 Hz, 1H), 8.34–8.22 (m, 2H), 7.93–7.85 (m, 2H), 7.75 (t, J = 6.0 Hz, 1H), 7.38–7.31 (m, 2H), 7.14 (t, J = 7.2 Hz, 1H), 6.30 (dd, J = 19.6, 13.2 Hz, 2H), 5.57 (dd, J = 13.2, 3.2 Hz, 2H), 5.32 (dd, J = 19.6, 3.6 Hz, 2H). 13C{1H} NMR (101 MHz, CDCl3) δ 160.6, 149.0, 142.1, 141.0, 140.3, 128.4, 126.5, 124.7, 124.6, 123.7, 122.3, 77.4, 77.1, 76.8. HRMS (ESI), m/z calcd for C16H15BN2ONa+ (M + Na)+ 285.1170, found 285.1173. IR (cm−1): 3,047, 2,928, 1,668, 1,495, 1,360, 757, 686.
1,1-bis(3-fluorophenyl)-2-phenyl-1,2-dihydro-3H-1λ4,8λ4-[1,3,2]diazaborolo[1,5-a]pyridin-3-one (3e) The product was isolated by flash chromatography as a yellow-green solid (34.6 mg, 58%): mp: 152.3–154.6°C; 1H NMR (400 MHz, CDCl3) δ 8.43 (d, J = 8.0 Hz, 1H), 8.37–8.27 (m, 2H), 7.72 (t, J = 6.4 Hz, 1H), 7.44 (d, J = 7.6 Hz, 2H), 7.25–7.16 (m, 4H), 7.13–7.04 (m, 3H), 7.00 (dd, J = 10.4, 1.2 Hz, 2H), 6.92 (td, J = 8.4, 2.0 Hz, 2H). 13C{1H} NMR (101 MHz, CDCl3) δ 164.0, 161.6, 161.2, 148.8, 143.0, 141.5, 139.3, 129.6, 129.5, 128.9, 128.9, 128.5, 127.4, 125.4, 124.8, 122.8, 119.8, 119.6, 114.3, 114.1, 77.4, 77.1, 76.8. 19F NMR (376 MHz, CDCl3) δ -113.78. HRMS (ESI), m/z calcd for C24H17BF2N2ONa+ (M + Na)+ 421.1294, found 421.1297. IR (cm−1): 3,068, 1,680, 1,495, 1,354, 762, 702.
1,1-bis(4-bromophenyl)-2-phenyl-1,2-dihydro-3H-1λ4,8λ4-[1,3,2]diazaborolo[1,5-a]pyridin-3-one (3f) The product was isolated by flash chromatography as a yellow-green solid (39.0 mg, 50%): mp: 250.0–251.3°C; 1H NMR (400 MHz, CDCl3) δ 8.42 (d, J = 8.0 Hz, 1H), 8.34–8.28 (m, 2H), 7.72 (t, J = 6.4 Hz, 1H), 7.47–7.41 (m, 2H), 7.36 (d, J = 8.0 Hz, 4H), 7.23–7.16 (m, 6H), 7.07 (t, J = 7.6 Hz, 1H). 13C{1H} NMR (101 MHz, CDCl3) δ 161.1, 148.8, 142.9, 141.4, 139.3, 135.2, 131.0, 128.5, 127.4, 125.4, 124.6, 122.8, 121.9, 77.4, 77.1, 76.7. HRMS (ESI), m/z calcd for C24H17BBr2N2ONa+ (M + Na)+ 542.9672, found 542.9680. IR (cm−1): 3,068, 1,674, 1,489, 1,354, 762, 698.
2-phenyl-1,1-di-p-tolyl-1,2-dihydro-3H-1λ4,8λ4-[1,3,2]diazaborolo[1,5-a]pyridin-3-one (3g) The product was isolated by flash chromatography as a yellow-green solid (36.9 mg, 63%): mp: 240.9–241.9°C; 1H NMR (400 MHz, CDCl3) δ 8.41–8.35 (m, 2H), 8.24–8.18 m, 1H), 7.64–7.56 (m, 1H), 7.28 (d, J = 8.0 Hz, 4H), 7.20–7.14 (m, 2H), 7.07 (d, J = 7.6 Hz, 4H), 7.05–7.00 (m, 1H), 2.31 (s, 6H). 13C{1H} NMR (101 MHz, CDCl3) δ 161.2, 148.8, 142.2, 141.5, 140.1, 136.6, 133.7, 128.6, 128.2, 127.0, 124.8, 124.6, 122.3, 77.4, 77.1, 76.8, 21.3. HRMS (ESI), m/z calcd for C26H23BN2ONa+ (M + Na)+ 413.1796, found 413.1799. IR (cm−1): 3,020, 1,674, 1,489, 1,348, 768, 686.
1,1-bis(4-chlorophenyl)-2-phenyl-1,2-dihydro-3H-1λ4,8λ4-[1,3,2]diazaborolo[1,5-a]pyridin-3-one (3h) The product was isolated by flash chromatography as yellow-green solid (43.3 mg, 67%): mp: 202.0–206.3°C; 1H NMR (400 MHz, DMSO-d6) δ 8.74 (d, J = 5.6 Hz, 1H), 8.56 (t, J = 7.6 Hz, 1H), 8.42 (d, J = 7.8 Hz, 1H), 7.99 (t, J = 6.4 Hz, 1H), 7.47 (d, J = 8.0 Hz, 2H), 7.35–7.25 (m, 8H), 7.19 (t, J = 7.6 Hz, 2H), 7.02 (t, J = 7.2 Hz, 1H). 13C{1H} NMR (101 MHz, DMSO-d6) δ 161.6, 147.3, 145.0, 142.8, 140.3, 135.5, 132.5, 129.5, 128.7, 128.1, 124.9, 124.1, 123.0, 40.6, 40.4, 40.2, 40.0, 39.8, 39.6, 39.4. HRMS (ESI), m/z calcd for C24H17BCl2N2ONa+ (M + Na)+ 453.0703, found 453.0707. IR (cm−1): 3,080, 1,674, 1,489, 1,360, 1,082, 768.
1,1-bis(4-(tert-butyl)phenyl)-2-phenyl-1,2-dihydro-3H-1λ4,8λ4-[1,3,2]diazaborolo[1,5-a]pyridin-3-one (3i) The product was isolated by flash chromatography as a yellow-green solid (45.5 mg, 64%): mp: 222.6–223.4°C; 1H NMR (400 MHz, CDCl3) δ 8.42–8.34 (m, 2H), 8.17 (td, J = 8.0, 1.2 Hz, 1H), 7.62–7.54 (m, 3H), 7.32 (d, J = 8.4 Hz, 4H), 7.28–7.23 (m, 4H), 7.20–7.13 (m, 2H), 7.05–6.97 (m, 1H), 1.29 (s, 18H). 13C{1H} NMR (101 MHz, CDCl3) δ 161.3, 149.7, 148.7, 142.1, 141.7, 140.2, 133.5, 128.2, 126.9, 124.7, 124.6, 124.6, 122.2, 77.4, 77.1, 76.8, 34.4, 31.4. HRMS (ESI) m/z calcd for C32H35BN2ONa+ (M + Na)+ 497.2735, found 497.2740. IR (cm−1): 3,080, 2,966, 1,674, 1,489, 1,354, 762, 686.
1,1-bis(benzo[d][1,3]dioxol-5-yl)-2-phenyl-1,2-dihydro-3H-1λ4,8λ4-[1,3,2]diazaborolo[1,5-a]pyridin-3-one (3j) The product was isolated by flash chromatography as a yellow-green solid (41.9 mg, 62%): mp: 227.6–229.4°C; 1H NMR (400 MHz, CDCl3) δ 8.37–8.33 (m, 2H), 8.20 (td, J = 8.0, 0.8 Hz, 1H), 7.65–7.59 (m, 1H), 7.58–7.52 (m, 2H), 7.23–7.16 (m, 2H), 7.04 (t, J = 7.6 Hz, 1H), 6.85 (dd, J = 7.6, 1.2 Hz, 2H), 6.79 (d, J = 1.2 Hz, 2H), 6.71 (d, J = 8.0 Hz, 2H), 5.85 (s, 4H). 13C{1H} NMR (101 MHz, CDCl3) δ 161.1, 148.6, 147.3, 146.8, 142.5, 141.5, 139.8, 128.3, 127.1, 125.0, 124.6, 122.4, 113.1, 108.2, 100.4, 77.4, 77.1, 76.8. HRMS (ESI) m/z calcd for C26H19BN2O5Na+ (M + Na)+ 473.1279, found 473.1281. IR (cm−1): 3,063, 2,884, 1,680, 1,484, 1,348, 1,234, 1,039, 768.
1,1-bis(4-fluorophenyl)-2-phenyl-1,2-dihydro-3H-1λ4,8λ4-[1,3,2]diazaborolo[1,5-a]pyridin-3-one (3k) The product was isolated by flash chromatography as a yellow-green solid (46.6 mg, 78%): mp: 176.1–177.9°C; 1H NMR (400 MHz, CDCl3) δ 8.40 (d, J = 7.6 Hz, 1H), 8.33 (d, J = 5.6 Hz, 1H), 8.31–8.24 (m, 1H), 7.71–7.65 (m, 1H), 7.49–7.44 (m, 2H), 7.34–7.27 (m, 4H), 7.22–7.15 (m, 2H), 7.08–7.02 (m, 1H), 6.96–6.90 (m, 4H). 13C{1H} NMR (101 MHz, CDCl3) δ 163.8, 161.3, 161.1, 148.7, 142.7, 141.4, 139.7, 135.2, 135.1, 128.7, 128.4, 127.3, 125.2, 124.6, 122.6, 114.9, 114.7, 77.4, 77.1, 76.8. 19F NMR (376 MHz, CDCl3) δ -113.78. HRMS (ESI) m/z calcd for C24H17BF2N2ONa+ (M + Na)+ 421.1294, found 421.1298. IR(cm−1): 3,042, 1,680, 1,495, 1,364, 1,164, 774.
1,1-bis(3-(benzyloxy)phenyl)-2-phenyl-1,2-dihydro-3H-1λ4,8λ4-[1,3,2]diazaborolo[1,5-a]pyridin-3-one (3l) The product was isolated by flash chromatographyas a yellow-green solid (60.3 mg, 70%): mp: 72.6–74.2°C; 1H NMR (400 MHz, CDCl3) δ 8.36 (d, J = 7.6 Hz, 1H), 8.26 (d, J = 5.6 Hz, 1H), 8.20 (td, J = 8.0, 1.2 Hz, 1H), 7.59–7.50 (m, 3H), 7.38–7.27 (m, 10H), 7.23–7.15 (m, 4H), 7.07 (t, J = 7.2 Hz, 1H), 6.98–6.94 (m, 4H), 6.90–6.84 (m, 2H), 4.97–4.89 (m, 4H). 13C{1H} NMR (101 MHz, CDCl3) δ 161.2, 158.3, 148.7, 142.4, 141.6, 139.9, 137.3, 128.9, 128.5, 128.4, 127.8, 127.6, 127.1, 126.3, 125.1, 124.9, 122.4, 120.0, 113.5, 77.4, 77.1, 76.8, 69.8. HRMS (ESI) m/z calcd for C38H31BN2O3Na+ (M + Na)+ 597.2320, found 597.2322. IR (cm−1): 3,063, 3,030, 1,680, 1,489, 1,348, 1,229, 762, 692.
1,1-di(naphthalen-2-yl)-2-phenyl-1,2-dihydro-3H-1λ4,8λ4-[1,3,2]diazaborolo[1,5-a]pyridin-3-one (3m) The product was isolated by flash chromatography as a yellow-green solid (63.8 mg, 92%): mp: 215.5–217.0°C; 1H NMR (400 MHz, CDCl3) δ 8.44 (dd, J = 4.8, 4.0 Hz, 2H), 8.20 (td, J = 7.6, 0.8 Hz, 1H), 7.98–7.95 (m, 2H), 7.83–7.78 (m, 2H), 7.77–7.71 (m, 4H), 7.65–7.61 (m, 2H), 7.60–7.55 (m, 1H), 7.51 (dd, J = 8.4, 1.2 Hz, 2H), 7.48–7.40 (m, 4H), 7.19–7.12 (m, 2H), 7.05–6.98 (m, 1H). 13C{1H} NMR (101 MHz, CDCl3) δ 161.4, 148.9, 142.5, 141.7, 139.9, 133.5, 133.4, 133.0, 131.0, 128.4, 128.1, 127.6, 127.1,127.1, 125.7, 125.6, 125.1, 124.8, 122.6, 77.4, 77.1, 76.8. HRMS (ESI) m/z calcd for C32H23BN2ONa+ (M + Na)+ 485.1796, found 485.1797. IR (cm−1): 3,047, 1,668, 1,495, 1,354, 762, 469.
1,1-bis(3-methoxyphenyl)-2-phenyl-1,2-dihydro-3H-1λ4,8λ4-[1,3,2]diazaborolo[1,5-a]pyridin-3-one (3n) The product was isolated by flash chromatography as a yellow-green solid (40.5 mg, 64%): mp: 170.9–172.2°C; 1H NMR (400 MHz, CDCl3) δ 8.378 (t, J = 5.2 Hz, 2H), 8.20 (td, J = 400, 1.2 Hz, 1H), 7.64–7.58 (m, 1H), 7.55–7.49 (m, 2H), 7.21–7.15 (m, 4H), 7.07–7.01 (m, 1H), 6.96–6.89 (m, 4H), 6.79–6.75 (m, 2H), 3.67 (s, 6H). 13C{1H} NMR (101 MHz, CDCl3) δ 161.3, 159.1, 148.7, 142.5, 141.7, 139.9, 128.9, 128.3, 127.1, 125.9, 125.1, 124.9, 122.4, 119.4, 112.2, 77.5, 77.1, 76.8, 55.0. HRMS (ESI) m/z calcd for C26H23BN2O3Na+ (M + Na)+ 445.1694, found 445.1699. IR (cm−1): 3,009, 1,668, 1,343, 1,229, 762, 757.
1,1-bis(2-methoxyphenyl)-2-phenyl-1,2-dihydro-3H-1λ4,8λ4-[1,3,2]diazaborolo[1,5-a]pyridin-3-one (3o) The product was isolated by flash chromatography as a yellow-green solid (22.2 mg, 35%): mp: 249.1–250.7°C; 1H NMR (400 MHz, CDCl3) δ 9.13 (d, J = 5.6 Hz, 1H), 8.29 (d, J = 7.6 Hz, 1H), 8.15 (t, J = 6.8 Hz, 1H), 7.62 (d, J = 7.6 Hz, 2H), 7.55 (t, J = 6.0 Hz, 1H), 7.43–7.39 (m, 2H), 7.23–7.18 (m, 2H), 7.12 (t, J = 7.6 Hz, 2H), 6.95 (t, J = 7.6 Hz, 1H), 6.84 (t, J = 7.2 Hz, 2H), 6.76 (d, J = 8.0 Hz, 2H), 3.48 (s, 6H). 13C{1H} NMR (101 MHz, CDCl3) δ 162.7, 162.3, 149.6, 142.7, 141.3, 140.9, 137.2, 128.6, 127.9, 125.9, 124.1, 124.0, 121.3, 120.3, 110.4, 77.4, 77.1, 76.8, 54.8. HRMS (ESI) m/z calcd for C26H23BN2O3Na+ (M + Na)+ 445.1694, found 445.1695. IR (cm−1): 3,058, 2,933, 1,674, 1,495, 1,360, 1,234, 757.
2-phenyl-1,1-bis(4-(trifluoromethyl)phenyl)-1,2-dihydro-3H-1λ4,8λ4-[1,3,2]diazaborolo[1,5-a]pyridin-3-one (3p) The product was isolated by flash chromatography as a yellow-green solid (34.4 mg, 46%): mp: 230.3–232.0°C; 1H NMR (400 MHz, CDCl3) δ 8.47 (d, J = 7.6 Hz, 1H), 8.37 (dd, J = 7.6, 1.2 Hz, 1H), 8.35–8.31 (m, 1H), 7.79–7.74 (m, 1H), 7.50 (d, J = 8.0 Hz, 4H), 7.46–7.40 (m, 6H), 7.24–7.18 (m, 2H), 7.09 (t, J = 7.6 Hz, 1H). 13C{1H} NMR (101 MHz, CDCl3) δ 161.1, 148.9, 143.2, 141.4, 139.1, 133.6, 129.8, 129.4, 129.1, 128.6, 127.5, 125.7, 125.5, 124.6, 124.6, 123.0, 123.0, 77.4, 77.0, 76.7. 19F NMR (376 MHz, CDCl3) δ -62.58. HRMS (ESI) m/z calcd for C26H17BF6N2ONa+ (M + Na)+ 521.1230, found 521.1236. IR (cm−1): 3,074, 3,036, 1,691, 1,327, 1,109, 762.
1,1-bis(4-(methylthio)phenyl)-2-phenyl-1,2-dihydro-3H-1λ4,8λ4-[1,3,2]diazaborolo[1,5-a]pyridin-3-one (3q) The product was isolated by flash chromatography as a yellow-green solid (44.3 mg, 65%): mp: 242.1–243.6°C; 1H NMR (400 MHz, CDCl3) δ 8.37–8.29 (m, 2H), 8.21 (td, J = 7.6, 0.8 Hz, 1H), 7.64–7.57 (m, 1H), 7.53–7.47 (m, 2H), 7.26–7.22 (m, 4H), 7.18–7.12 (m, 2H), 7.11–7.08 (m, 4H), 7.04–6.98 (m, 1H), 2.40 (s, 6H). 13C{1H} NMR (101 MHz, CDCl3) δ 161.2, 148.7, 142.5, 141.4, 139.8, 137.2, 134.1, 128.4, 127.1, 125.9, 125.0, 124.5, 122.5, 77.4, 77.1, 76.8, 15.5. HRMS (ESI) m/z calcd for C26H23BN2OS2Na+ (M + Na)+ 477.1237, found 477.1238. IR (cm−1): 3,063, 2,916, 1,674, 1,495, 1,375, 1,088, 762.
1,1-bis(2-fluorophenyl)-2-phenyl-1,2-dihydro-3H-1λ4,8λ4-[1,3,2]diazaborolo[1,5-a]pyridin-3-one (3r) The product was isolated by flash chromatography as a yellow-green solid (29.3 mg, 49%): mp: 222.9–224.0°C; 1H NMR (400 MHz, CDCl3) δ 8.85 (d, J = 6.0 Hz, 1H), 8.36 (d, J = 7.6 Hz, 1H), 8.25 (td, J = 8.0, 1.2 Hz, 1H), 7.10–766 (m, 1H), 7.65–7.60 (m, 2H), 7.48–7.42 (m, 2H), 7.25–7.20 (m, 2H), 7.20–7.15 (m, 2H), 7.06–7.01 (m, 3H), 6.92–6.86 (m, 2H). 13C{1H} NMR (101 MHz, CDCl3) δ 167.3, 164.9, 161.6, 149.0, 142.5, 142.0, 140.0, 136.9, 136.8, 129.8, 129.7, 128.3, 127.2, 124.6, 123.9, 123.8, 123.8, 122.4, 115.2, 115.0, 77.4, 77.1, 76.7. 19F NMR (376 MHz, CDCl3) δ -103.33. HRMS (ESI) m/z calcd for C24H17BF2N2ONa+ (M + Na)+ 421.1294, found 421.1298. IR (cm−1): 3,068, 1,668, 1,495, 1,354, 762, 746.
1,1-bis(3-(tert-butyl)phenyl)-2-phenyl-1,2-dihydro-3H-1λ4,8λ4-[1,3,2]diazaborolo[1,5-a]pyridin-3-one (3s) The product was isolated by flash chromatography as a yellow-green solid (55.5 mg, 78%): mp: 78.1–79.8°C; 1H NMR (400 MHz, CDCl3) δ 8.45–8.37 (m, 2H), 8.21 (td, J = 7.6, 0.8 Hz, 1H), 7.66–7.55 (m, 3H), 7.51–7.45 (m, 2H), 7.32–7.27 (m, 2H), 7.23–7.14 (m, 4H), 7.08–7.01 (m, 3H), 1.25 (s, 18H).13C{1H} NMR (101 MHz, CDCl3) δ 161.3, 149.9, 148.9, 142.3, 141.7, 140.0, 131.6, 130.2, 128.2, 127.4, 127.0, 125.1, 125.0, 123.9, 122.4, 77.5, 77.1, 76.8, 34.7, 31.5. HRMS (ESI) m/z calcd for C32H35BN2ONa+ (M + Na)+ 497.2735, found 497.2740. IR (cm−1): 2,966, 1,680, 1,489, 1,354, 757, 713.
2,2′-(3-oxo-2-phenyl-2,3-dihydro-1H-1λ4,8λ4-[1,3,2]diazaborolo[1,5-a]pyridine-1,1-diyl)dibenzonitrile (3t) The product was isolated by flash chromatography as a yellow-green solid (33.4 mg, 54%): mp: 252.9–253.7°C; 1H NMR (400 MHz, CDCl3) δ 8.44–8.40 (m, 1H), 8.34–8.29 (m, 2H), 7.75–7.69 (m, 1H), 7.47–7.42 (m, 2H), 7.39–7.34 (m, 4H), 7.22–7.16 (m, 6H), 7.10–7.04 (m, 1H). 13C{1H} NMR (101 MHz, CDCl3) δ 161.0, 148.8, 142.9, 141.4, 139.4, 135.2, 131.0, 128.5, 127.3, 125.3, 124.6, 122.8, 121.9, 77.4, 77.1, 76.7. HRMS (ESI) m/z calcd for C26H18BN4O+ (M + H)+ 413.1568, found 413.1563. IR (cm−1): 3,074, 3,025, 1,674, 1,489, 1,348, 762.
1,1-bis(dibenzo[b,d]furan-4-yl)-2-phenyl-1,2-dihydro-3H-1λ4,8λ4-[1,3,2]diazaborolo[1,5-a]pyridin-3-one (3u) The product was isolated by flash chromatography as a yellow-green solid (20.3 mg, 25%): mp: 240.3–241.9°C; 1H NMR (400 MHz, CDCl3) δ 9.12 (d, J = 5.6 Hz, 1H), 8.55 (d, J = 7.6 Hz, 1H), 8.31 (t, J = 7.6 Hz, 1H), 7.98–7.90 (m, 4H), 7.71–7.64 (m, 4H), 7.60–7.54 (m, 1H), 7.39–7.34 (m, 2H), 7.33–7.29 (m, 3H), 7.29–7.27 (m, 1H), 7.24 (d, J = 7.6 Hz, 2H), 7.15 (t, J = 7.9 Hz, 2H), 7.00 (t, J = 7.2 Hz, 1H).13C{1H} NMR (101 MHz, CDCl3) δ 162.0, 159.6, 155.5, 149.8, 142.6, 142.3, 140.3, 134.7, 128.2, 126.8, 126.5, 124.6, 124.5, 124.3, 123.2, 122.6, 122.4, 122.1, 120.4, 120.2, 111.3, 77.4, 77.1, 76.7. HRMS (ESI) m/z calcd for C36H23BN2O3Na+ (M + Na)+ 565.1694, found 565.1697. IR (cm−1): 3,053, 1,685, 1,446, 1,348, 1,175, 762.
2-(naphthalen-1-yl)-1,1-diphenyl-1,2-dihydro-3H-1λ4,8λ4-[1,3,2]diazaborolo[1,5-a]pyridin-3-one (3v) The product was isolated by flash chromatography as a yellow-green solid (49.5 mg, 80%): mp: 251.2–252.5°C; 1H NMR (400 MHz, CDCl3) δ 8.48 (d, J = 8.0 Hz, 1H), 8.38 (d, J = 5.6 Hz, 1H), 8.30 (t, J = 7.2 Hz, 1H), 7.75–7.67 (m, 3H), 7.58–7.32 (m, 3H), 7.32–7.27 (m, 3H), 7.23–7.15 (m, 3H), 7.14–6.78 (m, 6H). 13C{1H} NMR (101 MHz, CDCl3) δ 161.4, 148.9, 142.6, 142.5, 136.0, 134.2, 129.7, 128.0, 127.3, 127.1, 125.4, 125.3, 125.2, 124.2, 124.1, 122.8, 77.4, 77.1, 76.7. HRMS (ESI) m/z calcd for C28H21BN2ONa+ (M + Na)+ 435.1639, found 435.1636. IR (cm−1): 3,047, 1,674, 1,398, 1,354, 774, 702.
1,1-bis(4-(benzyloxy)phenyl)-2-phenyl-1,2-dihydro-3H-1λ4,8λ4-[1,3,2]diazaborolo[1,5-a]pyridin-3-one (3w) The product was isolated by flash chromatography as a yellow-green solid (60.3 mg, 70%): mp: 83.7–85.6°C; 1H NMR (400 MHz, CDCl3) δ 8.37 (d, J = 7.2 Hz, 2H), 8.22 (t, J = 8.0 Hz, 1H), 7.66–7.54 (m, 3H), 7.44–7.35 (m, 8H), 7.35–7.28 (m, 6H), 7.19 (t, J = 7.6 Hz, 2H), 7.05 (t, J = 7.6 Hz, 1H), 6.89 (d, J = 8.4 Hz, 4H), 5.02 (s, 4H). 13C{1H} NMR (101 MHz, CDCl3) δ 161.2, 158.2, 148.8, 142.2, 141.5, 140.1, 137.3, 134.9, 128.6, 128.3, 127.9, 127.6, 127.0, 124.8, 124.6, 122.3, 114.2, 77.4, 77.2, 76.8, 69.8. HRMS (ESI) m/z calcd for C38H31BN2O3Na+ (M + Na)+ 597.2320, found 597.2322. IR (cm−1): 3,020, 1,674, 1,592, 1,170, 698.
Data Availability Statement
The original contributions presented in the study are included in the article/Supplementary Material, further inquiries can be directed to the corresponding authors. Additional 1H and 13C spectra and spectral data for all compounds are available free of charge at http://pubs.acs.org.
Author Contributions
GY performed the experiments. LX and YW wrote the manuscript.
Conflict of Interest
The authors declare that the research was conducted in the absence of any commercial or financial relationships that could be construed as a potential conflict of interest.
Publisher’s Note
All claims expressed in this article are solely those of the authors and do not necessarily represent those of their affiliated organizations, or those of the publisher, the editors and the reviewers. Any product that may be evaluated in this article, or claim that may be made by its manufacturer, is not guaranteed or endorsed by the publisher.
Acknowledgments
We thank the financial support by the National Natural Science Foundation of China (No. 21963010), Shihezi University (CXRC201601 and CXRC201602).
Supplementary Material
The Supplementary Material for this article can be found online at: https://www.frontiersin.org/articles/10.3389/fchem.2022.856832/full#supplementary-material
References
Adamo, C., and Jacquemin, D. (2013). The Calculations of Excited-State Properties with Time-Dependent Density Functional Theory. Chem. Soc. Rev. 42, 845–856. doi:10.1039/C2CS35394F
Chen, P.-Z., Niu, L.-Y., Chen, Y.-Z., and Yang, Q.-Z. (2017). Difluoroboron β-diketonate Dyes: Spectroscopic Properties and Applications. Coord. Chem. Rev. 350, 196–216. doi:10.1016/j.ccr.2017.06.026
Dou, C., Liu, J., and Wang, L. (2017). Conjugated Polymers Containing B←N Unit as Electron Acceptors for All-Polymer Solar Cells. Sci. China Chem. 60, 450–459. doi:10.1007/s11426-016-0503-x
Frisch, M. J., Trucks, G. W., Schlegel, H. B., Scuseria, G. E., Robb, M. A., Cheeseman, J. R., et al. (2009). Gaussian 09, Revision A. Wallingford, CT: Gaussian, Inc..
Gong, S., Liu, Q., Wang, X., Xia, B., Liu, Z., and He, W. (2015). AIE-active Organoboron Complexes with Highly Efficient Solid-State Luminescence and Their Application as Gas Sensitive Materials. Dalton Trans. 44, 14063–14070. doi:10.1039/C5DT01525A
Huang, Z., Wang, S., Dewhurst, R. D., Ignat'ev, N. V., Finze, M., and Braunschweig, H. (2020). Boron: Its Role in Energy‐Related Processes and Applications. Angew. Chem. Int. Ed. 59, 8800–8816. doi:10.1002/anie.201911108
Ito, S., Gon, M., Tanaka, K., and Chujo, Y. (2021). Molecular Design and Application of Luminescent Materials Composed of Group 13 Elements with an Aggregation-Induced Emission Property. Natl. Sci. Rev. 8, nwab049. doi:10.1093/nsr/nwab049
Kubota, Y., Tanaka, S., Funabiki, K., and Matsui, M. (2012). Synthesis and Fluorescence Properties of Thiazole-Boron Complexes Bearing a β-Ketoiminate Ligand. Org. Lett. 14, 4682–4685. doi:10.1021/ol302179r
Li, Q., Zhang, S.-Y., He, G., Ai, Z., Nack, W. A., and Chen, G. (2014). Copper-Catalyzed Carboxamide-Directed Ortho Amination of Anilines with Alkylamines at Room Temperature. Org. Lett. 16, 1764–1767. doi:10.1021/ol500464x
Liu, Z., Jiang, Z., Yan, M., and Wang, X. (2019). Recent Progress of BODIPY Dyes With Aggregation-Induced Emission. Front. Chem. 7, 712. doi:10.3389/fchem.2019.00712
Luo, J., Xie, Z., Lam, J. W. Y., Cheng, L., Tang, B. Z., Chen, H., et al. (2001). Aggregation-induced Emission of 1-Methyl-1,2,3,4,5-Pentaphenylsilole. Chem. Commun., 1740–1741. doi:10.1039/B105159H
Mei, J., Leung, N. L. C., Kwok, R. T. K., Lam, J. W. Y., and Tang, B. Z. (2015). Aggregation-Induced Emission: Together We Shine, United We Soar!. Chem. Rev. 115, 11718–11940. doi:10.1021/acs.chemrev.5b00263
Mellerup, S. K., and Wang, S. (2019). Boron-based Stimuli Responsive Materials. Chem. Soc. Rev. 48, 3537–3549. doi:10.1039/C9CS00153K
Min, X., Yi, F., Han, X.-L., Li, M., Gao, Q., Liang, X., et al. (2022). Targeted Photodynamic Therapy Using a Water-Soluble Aggregation-Induced Emission Photosensitizer Activated by an Acidic Tumor Microenvironment. Chem. Eng. J. 432, 134327. doi:10.1016/j.cej.2021.134327
Nitti, A., Botta, C., Forni, A., Cariati, E., Lucenti, E., and Pasini, D. (2020). Crystallization-induced Room-Temperature Phosphorescence in Fumaramides. CrystEngComm 22, 7782–7785. doi:10.1039/D0CE01253J
Qi, Y., Cao, X., Zou, Y., and Yang, C. (2021). Color-tunable Tetracoordinated Organoboron Complexes Exhibiting Aggregation-Induced Emission for the Efficient Turn-On Detection of Fluoride Ions. Mater. Chem. Front. 5, 2353–2360. doi:10.1039/D1QM00046B
Sawazaki, T., Shimizu, Y., Oisaki, K., Sohma, Y., and Kanai, M. (2018). Convergent and Functional-Group-Tolerant Synthesis of B-Organo BODIPYs. Org. Lett. 20, 7767–7770. doi:10.1021/acs.orglett.8b03138
Shiu, Y.-J., Cheng, Y.-C., Tsai, W.-L., Wu, C.-C., Chao, C.-T., Lu, C.-W., et al. (2016). Pyridyl Pyrrolide Boron Complexes: The Facile Generation of Thermally Activated Delayed Fluorescence and Preparation of Organic Light-Emitting Diodes. Angew. Chem. Int. Ed. 55, 3017–3021. doi:10.1002/anie.201509231
Virgili, T., Forni, A., Cariati, E., Pasini, D., and Botta, C. (2013). Direct Evidence of Torsional Motion in an Aggregation-Induced Emissive Chromophore. J. Phys. Chem. C 117, 27161–27166. doi:10.1021/jp4104504
Wang, X., Wu, Y., Liu, Q., Li, Z., Yan, H., Ji, C., et al. (2015). Aggregation-induced Emission (AIE) of Pyridyl-Enamido-Based Organoboron Luminophores. Chem. Commun. 51, 784–787. doi:10.1039/C4CC07451C
Wang, Z., Cheng, C., Kang, Z., Miao, W., Liu, Q., Wang, H., et al. (2019). Organotrifluoroborate Salts as Complexation Reagents for Synthesizing BODIPY Dyes Containing Both Fluoride and an Organo Substituent at the Boron Center. J. Org. Chem. 84, 2732–2740. doi:10.1021/acs.joc.8b03145
Wu, Y., Li, Z., Liu, Q., Wang, X., Yan, H., Gong, S., et al. (2015). High Solid-State Luminescence in Propeller-Shaped AIE-Active Pyridine-Ketoiminate-boron Complexes. Org. Biomol. Chem. 13, 5775–5782. doi:10.1039/C5OB00607D
Yan, N., Wang, F., Wei, J., Song, J., Yan, L., Luo, J., et al. (2019). Highly Emissive B←N Unit Containing Four-Coordinate C,N-Chelated Organoboron Compound for the Detection of Fluoride Ions. Dyes Pigm. 166, 410–415. doi:10.1016/j.dyepig.2019.03.057
Yan, W., Hong, C., Long, G., Yang, Y., Liu, Z., Bian, Z., et al. (2014). Synthesis, crystal Structures and Photophysical Properties of Novel boron-containing Derivatives of Phenalene with Bright Solid-State Luminescence. Dyes Pigm. 106, 197–204. doi:10.1016/j.dyepig.2014.03.017
Yang, K., Zhang, G., and Song, Q. (2018). Four-coordinate Triarylborane Synthesis via cascade B-Cl/C-B Cross-Metathesis and C-H Bond Borylation. Chem. Sci. 9, 7666–7672. doi:10.1039/C8SC02281J
Yang, T., Tang, N., Wan, Q., Yin, S.-F., and Qiu, R. (2021). Recent Progress on Synthesis of N,N′-Chelate Organoboron Derivatives. Molecules 26, 1401. doi:10.3390/molecules26051401
Yin, Y., Chen, Z., Li, R.-H., Yuan, C., Shao, T.-Y., Wang, K., et al. (2021). Ligand-Triggered Platinum(II) Metallacycle with Mechanochromic and Vapochromic Responses. Inorg. Chem. 60, 9387–9393. doi:10.1021/acs.inorgchem.1c00233
Zhao, Y., and Truhlar, D. G. (2008). The M06 Suite of Density Functionals for Main Group Thermochemistry, Thermochemical Kinetics, Noncovalent Interactions, Excited States, and Transition Elements: Two New Functionals and Systematic Testing of Four M06-Class Functionals and 12 Other Functionals. Theor. Chem. Account. 120, 215–241. doi:10.1007/s00214-007-0310-x
Keywords: tetracoordinated organoboron, AIE, picolinamide, potassium trifluoroborate, manganese
Citation: You G, Xu L and Wei Y (2022) The Synthesis of Picolinamide-Supported Tetracoordinated Organoboron Complexes with Aggregation-Induced Emission Property. Front. Chem. 10:856832. doi: 10.3389/fchem.2022.856832
Received: 17 January 2022; Accepted: 21 February 2022;
Published: 22 March 2022.
Edited by:
Jamal Rafique, Federal University of Mato Grosso do Sul, BrazilReviewed by:
Zhao Chen, Jiangxi Science and Technology Normal University, ChinaDario Pasini, University of Pavia, Italy
Copyright © 2022 You, Xu and Wei. This is an open-access article distributed under the terms of the Creative Commons Attribution License (CC BY). The use, distribution or reproduction in other forums is permitted, provided the original author(s) and the copyright owner(s) are credited and that the original publication in this journal is cited, in accordance with accepted academic practice. No use, distribution or reproduction is permitted which does not comply with these terms.
*Correspondence: Liang Xu, eHVsaWFuZzQ0MjNAc2h6dS5lZHUuY24=; Yu Wei, eXV3ZWljaGVtQHNoenUuZWR1LmNu