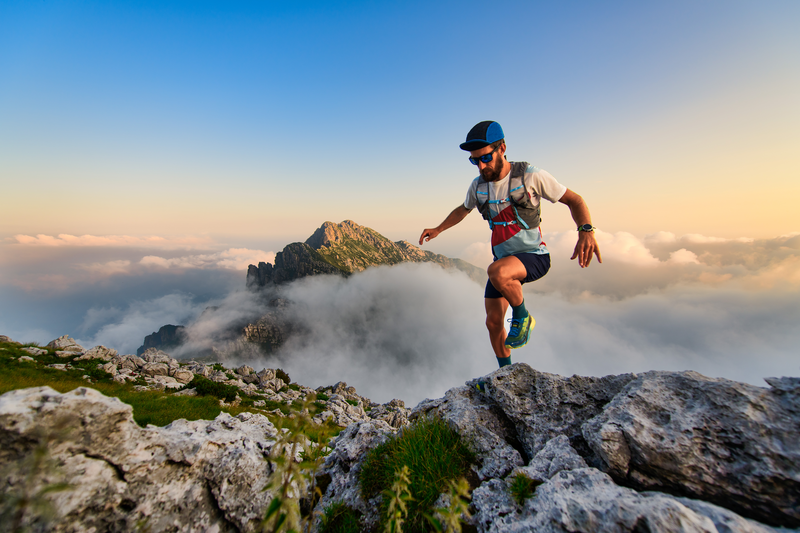
95% of researchers rate our articles as excellent or good
Learn more about the work of our research integrity team to safeguard the quality of each article we publish.
Find out more
ORIGINAL RESEARCH article
Front. Chem. , 22 February 2022
Sec. Medicinal and Pharmaceutical Chemistry
Volume 10 - 2022 | https://doi.org/10.3389/fchem.2022.854274
A series of 1,3,4-oxadiazole contained sesquiterpene derivatives were synthesized, and the activity of the target compounds against Xanthomonas oryzae pv. oryzae (Xoo), Xanthomonas axonopodis pv. citri (Xac), and tobacco mosaic virus (TMV) were evaluated. The biological activity results showed that the EC50 values of compounds H4, H8, H11, H12, H14, H16, and H19 for Xac inhibitory activity were 33.3, 42.7, 56.1, 74.5, 37.8, 43.8, and 38.4 μg/ml, respectively. Compounds H4, H8, H15, H19, H22, and H23 had inhibitory effects on Xoo, with EC50 values of 51.0, 43.3, 43.4, 50.5, 74.6, and 51.4 μg/ml, respectively. In particular, the curative and protective activities of compound H8 against Xoo in vivo were 51.9 and 49.3%, respectively. In addition, the EC50 values of the inactivation activity of compounds H4, H5, H9, H10, and H16 against TMV were 69.6, 58.9, 69.4, 43.9, and 60.5 μg/ml, respectively. The results of molecular docking indicated that compound H10 exhibited a strong affinity for TMV-coat protein, with a binding energy of −8.88 kcal/mol. It may inhibit the self-assembly and replication of TMV particles and have an anti-TMV effect, which supports its potential usefulness as an antiviral agent.
Most plant diseases are caused by biological agents such as bacteria, fungi, viruses, and nematodes, which have adverse impact on the growth and development of plants (Das et al., 2016). Rice bacterial blight caused by Xanthomonas oryzae pv. oryzae (Xoo) seriously threatens the growth and production of rice by affecting the tillering stage of rice (Wang et al., 2021). Citrus bacterial canker caused by Xanthomonas axonopodis pv. citri (Xac) reduces the quality and yield of fruits (Graham et al., 2004). Tobacco mosaic virus (TMV) can survive in dry plant debris for up to 100 years, and the associated plant diseases cause economic losses of more than USD 30 billion each year (Wang et al., 2018; Guo et al., 2021). At present, pesticides are the main means of controlling crop diseases and insect pests (Kemmitt et al., 2018). For plant disease, such as Xoo, Xac or TMV, although there are traditional medicines (such as Bismerthiazol, Thiodiazole copper, Ningnanmycin and Ribavirin), their effectiveness is limited various forms of disease and insect resistance (Buttimer et al., 2017; Liu et al., 2021). Natural products have special structural characteristics and unique biological activity mechanisms, and they are an important source for discovery of highly effective, safe, and environmentally compatible drugs (Zhang et al., 2018; Zheng and Hua, 2020; Li and Wang, 2021).
Sesquiterpenes are the most common type of terpenoids in terms of the number of compounds and the type of structural skeleton. They have thousands of representative structures and more than 300 different skeletons (Sacchettini and Poulter, 1997; Arroo, 2007). Sesquiterpenes are natural products of terpenoids found in plants, fungi, marine organisms, insects, and microorganisms. They are widely used in agriculture, medicine, perfume, cosmetics, and biofuels (Liu C.-L. et al., 2021; Liu T. et al., 2021; Mai et al., 2021). Sesquiterpenes have a variety of biological activities due to their complex three-dimensional structure, such as antiviral (Shang et al., 2016; Zhao et al., 2017), antibacterial (Duan et al., 2020; Wang et al., 2020), antifungal (Aricu et al., 2016), insecticidal and antifeedant activities (Inocente et al., 2019). In addition, at least some have excellent pharmacological activity, such as artemisinin for anti-malaria (Platon et al., 2021). There may also have anti-inflammatory (Gao et al., 2015), anti-HIV (Liu Y.-P. et al., 2021), and cytotoxic activity (Ryu et al., 2015). Collectively, sesquiterpenes offer a wide potential for research and commercial applications.
Heterocyclic compounds often combine good activity, high selectivity, and low dosage, thus features attractive to new pesticide research (Jin and Zhang_, 2010; Wu et al., 2011; Wu et al., 2013). The presence of nitrogen in the molecule is usually accompanied by the emergence of new compound activities or the enhancement of the original activity characteristics of natural terpenoids (Lungu., 2015). Among them, 1,3,4-oxadiazole is a kind of heterocyclic compound with a variety of biological activities, and its derivatives show antiviral (Gan et al., 2017; He et al., 2021), antibacterial (Vasantha et al., 2019; Yu et al., 2021; Wang S. et al., 2021), antifungal (Wen et al., 2019; Wang X. et al., 2021) and insecticidal activity (Yang et al., 2020) in agricultural applications. Some also proved to be attractive anti-cancer (Kumar et al., 2009), anti-depressant (Ergun et al., 2010), anti-HIV (Parizadeh et al., 2018), and anti-inflammatory (Naseer et al., 2019) medicines. Additionally, the presence of alkyl groups on the oxadiazole nucleus increases their ability to penetrate active sites and enhance their biological activity (Vasantha et al., 2019).
In view of the above findings, as one of the most active research fields in natural product chemistry, sesquiterpenes can be derived from their skeletons to obtain active different compounds. In this study, using the principle of active substructure splicing, sclareolide was used as the lead compound and the active fragment of oxadiazole was introduced (Figure 1). A series of 1,3,4-oxadiazole contained sesquiterpene derivatives were synthesized and their biological activities were evaluated.
The in vitro antibacterial activity of synthetic compounds H1–H23 against Xoo and Xac was tested by the turbidity method (Zhang et al., 2021). The preliminary biological activity results are shown in Table 1. The inhibitory activities of compounds H4, H8, H15, H19, and H22 on Xoo were 64.5, 70.2, 69.5, 65.7, and 60.1% at 100 μg/ml, respectively, which were higher than that of thiodiazole copper (56.7%). The inhibitory effects of compounds H4, H8, H14, and H19 on Xac at 100 μg/ml were 73.3, 65.1, 70.5, and 66.6%, respectively, which were better than that of bismerthiazol (64.6%).
TABLE 1. In vitro antibacterial activity of the target compounds against Xoo and Xaca.
The concentration values for 50% of maximal effect (EC50) of some compounds are shown in Table 2. The EC50 values of compounds H8 and H15 against Xoo were 43.3 and 43.4 μg/ml, respectively, which were close to bismerthiazol (41.8 μg/ml) and superior to that of thiodiazole copper (61.4 μg/ml). Compounds H4 and H14 had an inhibitory effect on Xac, with their EC50 values being 33.3 and 37.8 μg/ml, respectively, thus better than for bismerthiazol (38.2 μg/ml).
TABLE 2. Antibacterial activities of some target compounds against Xoo and Xac in Vitroa.
To further verify the control effect of the compound on rice bacterial leaf blight, the in vivo antibacterial activity of compound H8 was determined by the leaf-cutting method at 200 μg/ml (Zhang et al., 2021). The results are shown in Table 3, Table 4; Figure 2. The curative activity of compound H8 was 51.9%, which was better than that of bismerthiazol (47.1%) and thiodiazole copper (46.1%). Concomitantly, the compound H8 showed good protective activity of 49.3% compared to bismerthiazol (45.8%) and thiodiazole copper (43.7%).
TABLE 3. The curative activity of compound H8 against Xanthomonas oryzae pv. oryzae in Vivo at 200 μg/ml.
TABLE 4. The Protective activity of compound H8 against Xanthomonas oryzae pv. oryzae in Vivo at 200 μg/ml
FIGURE 2. Curative and protective activities of compound H8 against rice bacterial leaf blight under greenhouse conditions at 200 μg/ml, with BT and TC as the positive control agents.
According to the classic literature method (Ren et al., 2020), the activity of the target compound H1–H23 on TMV was tested. Preliminary bioactivity showed that most of the compounds exhibited a good inhibitory effect on TMV at 500 μg/ml. The results are shown in Table 5. Compared with ribavirin, most compounds had moderate to good activity. The curative activities of compounds H8, H12, H16, and H19 were 68.3, 63.5, 67.5, and 63.3%, respectively, which were significantly higher than ribavirin (45.4%). Notably, the curative activity of compound H9 was 77.5%, which was better than ningnanmycin (70.0%). The inactivation potency of compounds H3, H4, H5, H9, and H16 were 81.7, 82.0, 87.5, 82.0, and 87.3%, respectively, which were higher than that of ribavirin (72.3%). It was worth noting that the inactivation potency of compound H10 was 90.5%, which was slightly better than that of ningnanmycin (90.0%)
TABLE 5. Antiviral activities of target compounds against TMV in Vivo at 500 μg/mLa.
The EC50 values of some compounds were further tested, as shown in Table 6. The results indicated that the EC50 value of compound H10 was 43.9 μg/ml, which was better than ningnanmycin (44.8 μg/ml).
TMV coat protein (TMV-CP) plays an important role in the replication and assembly of plant viruses. Our goal was to investigate the interaction between active target compounds and TMV-CP. The binding method of ligand molecules (compound H10 and ningnanmycin) and TMV-CP (PDB 97 code: 1EI7) was explored through molecular docking, and the results are shown in Figures 3A,B. Compound H10 had a strong affinity for TMV-CP, with a binding energy of -8.88 kcal/mol, while that of ningnanmycin was 6.35 kcal/mol. The hydroxyl oxygen atom of compound H10 formed a strong hydrogen bond with ASN73 and ELU131 (the bond length is 3.1Å and 2.8Å, respectively), and the residue ELU131 can also be seen in ningnanmycin. Compound H10 had two hydrophobic interactions with amino acid residues TYR139 and THR136 in addition to interacted with VAL260 via hydrophobic bonds like ningnanmycin.
FIGURE 3. Molecule docking and MD simulation studies: (A) molecule docking of ningnanmycin, (B) molecule docking of compound H10, (C) MD simulation of ningnanmycin, (D) MD simulation of compound H10.
The stability and interaction mode of the ligand molecule and TMV-CP under the simulated conditions were further studied through molecular dynamics (MD) simulation, and the root-mean-square deviation (RMSD) of the atom and its initial position was measured (Figures 3C,D). Due to the significant interaction between the ligand and the binding site, the difference in energy characteristics results in a stable conformation and strong binding. Therefore, the biological activity can be influenced by optimizing the structure of the compound, and the properties of inhibiting TMV can be explored.
The preliminary structure-activity relationship showed that the different substituents R of sesquiterpene derivatives had a great influence on Xoo, Xac, and TMV. According to Table 1, when there are electron-withdrawing F, Cl or F, Br atoms on the benzene ring at the same time, the activity of the compound against Xoo is reduced: H4 (R = Ph) > H21 (R = 4-Br-2-F-Ph) > H1 (R = 2-Cl-5-F-Ph) > H9 (R = 2-Br-5-F-Ph) > H16 (R = 2-Br-4-F-Ph) > H17 (R = 3-Cl-2-F-Ph) > H6 (R = 2-Cl-4-F-Ph). The position of difluoro substitution on the aromatic ring also had an effect on the activity of Xac: H8 (R = 2,4-di-F-Ph) > H12 (R = 2,3-di-F-Ph) > H5 (R = 2,6-di-F-Ph) > H13 (R = 3,5-di-F-Ph) > H2 (R = 2,5-di-F-Ph). As shown in Table 5, introduction of different groups at the 4-position of the aromatic ring, altered the compounds’ curative activities against TMV, with the electron-donating group having improved activity over the electron-withdrawing group: H19 (R = 4-OCH3-Ph) > H23 (R = 4-NO2-Ph) > H3 (R = 4-CF3-Ph) > H22 (R = 4-OCF3-Ph) > H14 (R = 4-Cl-Ph) > H18 (R = 4-Br-2-F-Ph). The type and position of a single halogen atom on the benzene ring and heterocyclic ring may affect the inactivation potency of the compound: H10 (R = 3-Br-Ph) > H11 (R = 4-Cl-Py) > H14 (R = 4-Cl-Ph) > H15 (R = 2-Cl-Ph) > H18 (R = 4-Br-2-F-Ph) > H20 (R = 5-Cl-thiazol).
Melting points (uncorrected) of the synthetic compounds were determined using the XT-4 micro melting point instrument (Beijing Tech Instrument Co., China). All of the reactions were performed using a magnetic stir bar, followed by thin-layer chromatography (TLC) on silica gel GF254 and identified by UV. The 1H, 13C, and 19F nuclear magnetic resonance (NMR) spectra were obtained with AVANCE III HD 400 MHz or 500 MHz (Bruker Corporation, Switzerland) system in CDCl3, and used TMS as an internal standard at room temperature. High-resolution mass spectrometer (HRMS) data was conducted using an Orbitrap LC-MS instrument (Q-Exative, Thermo Scientific™, United States). All reagents and solvents were purchased from commercial suppliers and were not subjected to further purification and drying.
According to the synthetic route shown in Scheme 1, the target compounds H1–H23 were obtained. The natural product sclareolide was used as raw material to produce hydrazide intermediate 1 by hydrazinolysis reaction with hydrazine hydrate under weakly alkaline conditions. Intermediate 1 continues to form a closed loop with carbon disulfide under reflux to obtain oxadiazole intermediate 2. Then, under the alkaline condition in the presence of anhydrous potassium carbonate, intermediate 2 reacts with different substituted benzyl halides to synthesize the target compounds H1–H23.
As shown in Scheme 1, the previously published methods were used (Zhang et al., 2013; Mishra et al., 2017). The raw material sclareolide (500 mg, 1 mol) was dissolved in a round bottom flask with EtOH, and hydrazine hydrate (1 ml, 11 mol) was added and stirred at room temperature for 2 h. After the reaction was completed, an appropriate amount of water was added to the system, and the precipitate was collected by filtration to obtain Intermediate 1. Subsequently, Intermediate 1 (300 mg, 1 mol) was dissolved in DMF and stirred for 30 min, carbon disulfide (743 mg, 5 mol) was slowly added and refluxed for 6–8 h. The reaction mixture was diluted with water and extracted with ethyl acetate. The organic layer was dried over NaSO4 and concentrated under vacuum. The residue was purified by silica gel chromatography with petroleum ether/ethyl acetate (8:1) concentrated eluent to obtain Intermediate 2.
According to the published method (Wang et al., 2019), Intermediate 2 (200mg, 1 mol) and potassium carbonate (107mg, 1.2 mol) were dissolved in a round bottom flask with DMF and stirred for 30 min. Different substituted benzyl halides were added and reacted at room temperature for 6–7 h. An appropriate amount of water was added to the reaction mixture to filter the residue. The crude product was subjected to column chromatography with petroleum ether/ethyl acetate (5:1) to extract target compounds H1-H23.
The structures of synthesized compounds H1-H23 were confirmed by 1H NMR, 13C NMR, 19F NMR, and HRMS.
(1R,2R,8aS)-1-((5-((2-fluoro-5-(trifluoromethyl)benzyl)thio)-1,3,4-oxadiazol-2-yl)methyl)-2,5,5,8a-tetramethyldecahydronaphthalen-2-ol (H1). Yield 95%; White solid; m. p.75–76°C. 1H NMR (400 MHz, CDCl3) δ 7.81 (dd, J = 6.8, 2.1 Hz, 1H), 7.61–7.54 (m, 1H), 7.19 (t, J = 8.9 Hz, 1H), 4.47 (s, 2H), 2.90 (ddd, J = 76.1, 16.3, 5.7 Hz, 2H), 1.95–1.89 (m, 2H), 1.74–1.51 (m, 4H), 1.46 (d, J = 3.7 Hz, 1H), 1.40–1.30 (m, 4H), 1.21 (s, 3H), 1.00 (dd, J = 12.1, 2.2 Hz, 1H), 0.88 (s, 3H), 0.87 (s, 3H), 0.80 (s, 3H). 13C NMR (100 MHz, CDCl3) δ 170.5, 162.7 (d, J = 254.3 Hz), 162.3, 128.8 (d, J = 8.0 Hz), 127.4 (d, J = 3.7 Hz), 127.3 (d, J = 3.7 Hz), 124.6 (d, J = 15.6 Hz), 123.5 (d, J = 272.0 Hz), 116.2 (d, J = 22.7 Hz), 73.2, 59.0, 55.7, 44.5, 41.5, 39.3, 38.8, 33.3, 33.2, 29.5, 23.3, 21.4, 21.1, 20.4, 18.3, 15.1.19F NMR (376 MHz, CDCl3) δ -61.91, -110.96. HRMS (ESI+) m/z Calcd for C25H33F4SN2O2 [M + H]+ 501.21934; Found 501.21936.
(1R,2R,8aS)-1-((5-((2,5-difluorobenzyl)thio)-1,3,4-oxadiazol-2-yl)methyl)-2,5,5,8a-tetramethyldecahydronaphthalen-2-ol (H2). Yield 63%; White solid; m. p.84–86°C. 1H NMR (400 MHz, CDCl3) δ 7.26–7.22 (m, 1H), 7.04–7.00 (m, 1H), 6.98–6.95 (m, 1H), 4.40 (s, 2H), 2.91 (ddd, J = 76.7, 16.3, 5.6 Hz, 2H), 1.96–1.91 (m, 2H), 1.75–1.67 (m, 2H), 1.54–1.49 (m, 2H), 1.47–1.43 (m, 1H), 1.40–1.30 (m, 4H), 1.21 (s, 3H), 1.00 (dd, J = 12.1, 2.2 Hz, 1H), 0.88 (s, 3H), 0.87 (s, 3H), 0.80 (s, 3H). 13C NMR (100 MHz, CDCl3) δ 170.5, 162.6, 158.3 (d, J = 245.5 Hz), 156.8 (d, J = 246.6 Hz), 125.0 (dd, J = 17.1, 8.1 Hz), 117.7 (dd, J = 24.7, 3.6 Hz), 116.6 (dd, J = 21.2, 5.5 Hz), 116.3 (dd, J = 20.9, 5.4 Hz), 73.2, 59.0, 55.7, 44.5, 41.5, 39.3, 38.8, 33.4, 33.2, 29.7, 23.3, 21.4, 21.1, 20.4, 18.3, 15.1.19F NMR (376 MHz, CDCl3) δ -118.08, -122.78. HRMS (ESI+) m/z Calcd for C24H33F2SN2O2 [M + H]+ 451.22253; Found 451.22229.
(1R,2R,8aS)-2,5,5,8a-tetramethyl-1-((5-((4-(trifluoromethyl)benzyl)thio)-1,3,4-oxadiazol-2-yl)methyl)decahydronaphthalen-2-ol (H3). Yield 64%; White solid; m. p.81–83°C. 1H NMR (400 MHz, CDCl3) δ 7.58 (d, J = 8.5 Hz, 2H), 7.55 (d, J = 8.4 Hz, 2H), 4.44 (s, 2H), 2.89 (ddd, J = 75.7, 16.2, 5.7 Hz, 2H), 1.93–1.87 (m, 1H), 1.73–1.58 (m, 4H), 1.44 (ddt, J = 10.0, 6.8, 5.2 Hz, 4H), 1.33 (ddd, J = 13.5, 6.2, 1.3 Hz, 2H), 1.21 (s, 3H), 0.99 (dd, J = 12.1, 2.2 Hz, 1H), 0.88 (s, 3H), 0.86 (s, 3H), 0.80 (s, 3H). 13C NMR (100 MHz, CDCl3) δ 170.4, 162.5, 140.1 (d, J = 1.3 Hz), 130.1 (d, J = 32.6 Hz), 129.4, 129.2, 125.6 (d, J = 3.8 Hz), 125.6 (d, J = 11.2 Hz), 123.9 (d, J = 272.3 Hz), 73.2, 59.1, 55.8, 44.5, 41.5, 39.4, 38.8, 36.0, 33.3, 33.2, 23.3, 21.4, 21.1, 20.4, 18.3, 15.1. HRMS (ESI+) m/z Calcd for C25H34F3SN2O2 [M + H]+ 483.22876; Found 483.22870.
(1R,2R,8aS)-1-((5-(benzylthio)-1,3,4-oxadiazol-2-yl)methyl)-2,5,5,8a-tetramethyldecahydronaphthalen-2-ol (H4). Yield 91%; Pink solid; m. p.78–80°C. 1H NMR (400 MHz, CDCl3) δ 7.41 (dd, J = 8.0, 1.4 Hz, 2H), 7.35–7.30 (m, 3H), 4.42 (s, 2H), 2.90 (ddd, J = 77.6, 16.2, 5.6 Hz, 2H), 1.91 (ddd, J = 15.1, 9.0, 4.4 Hz, 2H), 1.73–1.64 (m, 1H), 1.59–1.47 (m, 2H), 1.43–1.34 (m, 4H), 1.32–1.23 (m, 2H), 1.20 (s, 3H), 0.99 (dd, J = 12.1, 2.2 Hz, 1H), 0.88 (s, 3H), 0.87 (s, 3H), 0.80 (s, 3H). 13C NMR (100 MHz, CDCl3) δ 170.1, 163.1, 135.7, 129.1, 128.7, 128.0, 73.2, 59.1, 55.7, 44.5, 41.5, 39.3, 38.8, 36.8, 33.3, 33.2, 23.3, 21.4, 21.1, 20.4, 18.3, 15.1. HRMS (ESI+) m/z Calcd for C24H35SN2O2 [M + H]+ 415.24138; Found 415.24130.
(1R,2R,8aS)-1-((5-((2,6-difluorobenzyl)thio)-1,3,4-oxadiazol-2-yl)methyl)-2,5,5,8a-tetramethyldecahydronaphthalen-2-ol (H5). Yield 84%; Pink solid; m. p.87–88°C. 1H NMR (400 MHz, CDCl3) δ 7.21–7.16 (m, 1H), 6.88–6.83 (m, 2H), 4.24 (s, 2H), 2.49–2.40 (m, 2H), 2.08–2.04 (m, 1H), 1.89–1.76 (m, 2H), 1.67–1.59 (m, 2H), 1.48–1.39 (m, 4H), 1.38–1.28 (m, 2H), 1.25 (s, 3H), 1.02 (d, J = 2.6 Hz, 1H), 0.88 (s, 3H), 0.87 (s, 3H), 0.82 (s, 3H). 13C NMR (100 MHz, CDCl3) δ 168.3, 161.5 (d, J = 250.1 Hz), 161.4 (d, J = 250.1 Hz), 154.5, 128.9 (d, J = 10.5 Hz), 113.9 (d, J = 19.4 Hz), 111.2 (d, J = 25.1 Hz), 111.2 (d, J = 12.8 Hz), 73.2, 59.1, 56.6, 42.1, 39.4, 38.6, 36.2, 33.3, 33.1, 26.3, 21.9, 20.9, 20.7, 18.1, 15.1.19F NMR (376 MHz, CDCl3) δ -113.32, -113.49. HRMS (ESI+) m/z Calcd for C24H33F2SN2O2 [M + H]+ 421.22253; Found 421.22253.
(1R,2R,8aS)-1-((5-((2-chloro-4-fluorobenzyl)thio)-1,3,4-oxadiazol-2-yl)methyl)-2,5,5,8a-tetramethyldecahydronaphthalen-2-ol (H6). Yield 84%; White solid; m. p.86–87°C. 1H NMR (400 MHz, CDCl3) δ 7.51 (dd, J = 8.3, 6.3 Hz, 1H), 7.09 (dd, J = 8.5, 2.6 Hz, 1H), 6.90 (td, J = 8.4, 2.6 Hz, 1H), 4.21 (s, 2H), 2.45 (t, J = 9.3 Hz, 2H), 2.08–2.04 (m, 1H), 1.83 (ddd, J = 30.2, 10.4, 5.2 Hz, 2H), 1.67–1.58 (m, 2H), 1.47–1.39 (m, 4H), 1.32 (m, 2H), 1.24 (s, 3H), 1.01 (d, J = 2.3 Hz, 2H), 0.88 (s, 3H), 0.87 (s, 3H). 13C NMR (100 MHz, CDCl3) δ 170.4, 162.9, 162.2 (d, J = 250.8 Hz), 135.0 (d, J = 10.4 Hz), 132.6 (d, J = 8.9 Hz), 129.9 (d, J = 3.7 Hz), 117.1 (d, J = 24.9 Hz), 114.3 (d, J = 21.1 Hz), 73.2, 59.0, 55.7, 44.4, 41.5, 39.3, 38.8, 33.8, 33.3, 33.2, 23.3, 21.4, 21.1, 20.4, 18.3, 15.1.19F NMR (376 MHz, CDCl3) δ -111.21. HRMS (ESI+) m/z Calcd for C24H33FClSN2O2 [M + H]+ 467.19298; Found 467.19293.
(1R,2R,8aS)-2,5,5,8a-tetramethyl-1-((5-((2-(trifluoromethyl)benzyl)thio)-1,3,4-oxadiazol-2-yl)methyl)decahydronaphthalen-2-ol (H7). Yield 98%; Pink solid; m. p.115–117°C. 1H NMR (400 MHz, CDCl3) δ 7.67 (d, J = 7.6 Hz, 1H), 7.60 (d, J = 7.7 Hz, 1H), 7.45 (dd, J = 14.3, 6.6 Hz, 1H), 7.37–7.29 (m, 1H), 4.35 (s, 2H), 2.49–2.40 (m, 2H), 2.08–2.03 (m, 1H), 1.95–1.84 (m, 2H), 1.67–1.61 (m, 2H), 1.47–1.39 (m, 4H), 1.37–1.29 (m, 2H), 1.25 (s, 3H), 1.00 (dd, J = 12.5, 2.7 Hz, 1H), 0.88 (s, 3H), 0.87 (s, 3H), 0.82 (s, 3H). 13C NMR (100 MHz, CDCl3) δ 168.6, 154.6, 137.4, 132.2 (d, J = 24.9 Hz), 131.9 (d, J = 5.0 Hz), 128.2, 127.1, 125.7 (d, J = 5.8 Hz), 124.3 (d, J = 274.0 Hz), 73.2, 59.1, 56.6, 44.5, 42.1, 39.4, 38.6, 36.2, 33.3, 33.1, 26.3, 21.9, 20.9, 20.7, 18.1, 15.1.19F NMR (376 MHz, CDCl3) δ -59.24. HRMS (ESI+) m/z Calcd for C25H34F3SN2O2 [M + H]+ 483.22876; Found 483.22855.
(1R,2R,8aS)-1-((5-((2,4-difluorobenzyl)thio)-1,3,4-oxadiazol-2-yl)methyl)-2,5,5,8a-tetramethyldecahydronaphthalen-2-ol (H8). Yield 72%; White solid; m. p.93–95°C. 1H NMR (400 MHz, CDCl3) δ 7.44 (dd, J = 15.4, 8.5 Hz, 1H), 6.83–6.78 (m, 1H), 6.77–6.72 (m, 1H), 4.12 (s, 2H), 2.50–2.40 (m, 4H), 2.10–1.73 (m, 1H), 1.49–1.40 (m, 4H), 1.38–1.28 (m, 2H), 1.25 (s, 3H), 1.02 (d, J = 2.4 Hz, 1H), 0.88 (s, 3H), 0.87 (s, 3H), 0.83 (s, 3H). 13C NMR (126 MHz, CDCl3) δ 170.5, 162.8 (d, J = 249.6 Hz), 162.8, 161.1 (d, J = 250.7 Hz), 132.3 (dd, J = 9.6, 4.8 Hz), 119.4 (dd, J = 14.5, 3.5 Hz), 111.5 (dd, J = 21.0, 3.5 Hz), 104.2 (d, J = 25.4 Hz), 73.3, 59.1, 55.8, 44.6, 41.6, 39.4, 38.8, 33.4, 33.3, 29.5, 23.3, 21.5, 21.2, 20.4, 18.4, 15.2.19F NMR (376 MHz, CDCl3) δ -109.30, -112.11. HRMS (ESI+) m/z Calcd for C24H32F2SN2O2Na [M + Na]+ 473.20448; Found 473.20499.
(1R,2R,8aS)-1-((5-((2-bromo-5-fluorobenzyl)thio)-1,3,4-oxadiazol-2-yl)methyl)-2,5,5,8a-tetramethyldecahydronaphthalen-2-ol (H9). Yield 84%; White solid; m. p.120–122°C. 1H NMR (400 MHz, CDCl3) δ 7.47 (dd, J = 8.8, 5.3 Hz, 1H), 7.30 (dd, J = 9.3, 3.0 Hz, 1H), 6.82 (td, J = 8.4, 3.1 Hz, 1H), 4.22 (s, 2H), 2.56–2.39 (m, 2H), 2.10–1.74 (m, 4H), 1.61 (dd, J = 11.6, 4.2 Hz, 1H), 1.44 (ddd, J = 20.8, 12.2, 5.6 Hz, 4H), 1.32 (ddd, J = 16.5, 10.4, 3.4 Hz, 2H), 1.25 (s, 3H), 1.02 (d, J = 2.5 Hz, 1H), 0.88 (s, 3H), 0.87 (s, 3H), 0.83 (s, 3H). 13C NMR (100 MHz, CDCl3) δ 168.3, 161.8 (d, J = 246.9 Hz), 154.7, 140.3 (d, J = 8.0 Hz), 133.6 (d, J = 8.1 Hz), 118.6 (d, J = 3.6 Hz), 118.2 (d, J = 23.4 Hz), 115.8 (d, J = 23.9 Hz), 74.1, 59.1, 56.6, 42.1, 39.4, 38.6, 36.2, 33.3, 33.1, 26.3, 21.9, 20.9, 20.7, 18.1, 15.1.19F NMR (376 MHz, CDCl3) δ -114.65. HRMS (ESI+) m/z Calcd for C24H32FBrSN2O2Na [M + Na]+ 533.12441; Found 533.12457.
(1R,2R,8aS)-1-((5-((3-bromobenzyl)thio)-1,3,4-oxadiazol-2-yl)methyl)-2,5,5,8a-tetramethyldecahydronaphthalen-2-ol (H10). Yield 83%; White solid; m. p.68–70°C.
1H NMR (400 MHz, CDCl3) δ 7.57 (t, J = 1.7 Hz, 1H), 7.44–7.40 (m, 1H), 7.36 (d, J = 7.8 Hz, 1H), 7.20 (t, J = 7.8 Hz, 1H), 4.37 (s, 2H), 3.05–2.72 (m, 2H), 1.96–1.79 (m, 4H), 1.73–1.66 (m, 1H), 1.56–1.42 (m, 4H), 1.28 (ddd, J = 13.4, 6.6, 3.5 Hz, 2H), 1.20 (s, 3H), 1.01 (d, J = 2.2 Hz, 1H), 0.88 (s, 3H), 0.86 (s, 3H), 0.80 (s, 3H). 13C NMR (100 MHz, CDCl3) δ 170.3, 162.6, 138.1, 132.0, 131.1, 130.3, 127.8, 122.6, 73.2, 59.0, 55.7, 44.5, 41.5, 39.3, 38.8, 36.0, 33.4, 33.2, 23.3, 21.4, 21.1, 20.4, 18.3, 15.1. HRMS (ESI+) m/z Calcd for C24H34BrSN2O2 [M + H]+ 493.15189; Found 493.15204.
(1R,2R,8aS)-1-((5-(((6-chloropyridin-3-yl)methyl)thio)-1,3,4-oxadiazol-2-yl)methyl)-2,5,5,8a-tetramethyldecahydronaphthalen-2-ol (H11). Yield 72%; White solid; m. p.102–104°C. 1H NMR (400 MHz, CDCl3) δ 8.44–8.36 (m, 2H), 7.69 (dd, J = 8.2, 2.3 Hz, 1H), 4.07 (s, 2H), 2.55–2.39 (m, 2H), 2.06 (dt, J = 11.6, 3.1 Hz, 1H), 1.88–1.74 (m, 2H), 1.68–1.60 (m, 2H), 1.44 (dt, J = 20.2, 5.8 Hz, 4H), 1.32 (ddd, J = 16.5, 11.1, 3.4 Hz, 2H), 1.26 (s, 3H), 1.02 (d, J = 2.7 Hz, 1H), 0.88 (s, 6H), 0.83 (s, 3H). 13C NMR (100 MHz, CDCl3) δ 168.0, 154.9, 149.9, 139.5, 133.8, 123.9, 89.0, 59.1, 56.6, 42.1, 39.4, 38.6, 36.2, 33.3, 33.0, 29.4, 26.4, 21.9, 20.9, 20.7, 18.1, 15.1. HRMS (ESI+) m/z Calcd for C23H31ClSN3O2 [M-H]- 448.18200; Found 448.18344.
(1R,2R,8aS)-1-((5-((2,3-difluorobenzyl)thio)-1,3,4-oxadiazol-2-yl)methyl)-2,5,5,8a-tetramethyldecahydronaphthalen-2-olH-12 (H12). Yield 67%; White solid; m. p.99–100°C. 1H NMR (400 MHz, CDCl3) δ 7.19 (ddd, J = 8.8, 5.8, 3.1 Hz, 1H), 6.95 (td, J = 9.0, 4.5 Hz, 1H), 6.92–6.84 (m, 1H), 4.12 (s, 2H), 2.53–2.40 (m, 2H), 2.06 (dt, J = 11.6, 3.2 Hz, 1H), 1.87 (ddd, J = 14.0, 6.8, 3.2 Hz, 2H), 1.78 (dd, J = 13.5, 7.2 Hz, 2H), 1.69–1.59 (m, 2H), 1.49–1.32 (m, 4H), 1.25 (s, 3H), 1.02 (d, J = 2.5 Hz, 1H), 0.88 (s, 3H), 0.88 (s, 3H), 0.83 (s, 3H). 13C NMR (100 MHz, CDCl3) δ 168.3, 159.6, 156.8 (d, J = 243.0 Hz), 155.9 (d, J = 255.4 Hz), δ 127.6 (dd, J = 17.6, 8.1 Hz), 117.5 (dd, J = 24.4, 3.4 Hz), 116.0 (dd, J = 24.6, 8.7 Hz), 115.0 (dd, J = 23.9, 8.5 Hz), 88.8, 59.1, 56.6, 42.1, 39.4, 38.6, 36.2, 33.3, 33.1, 26.3, 21.9, 20.9, 20.7, 18.1, 15.1.19F NMR (376 MHz, CDCl3) δ -119.09, -123.36. HRMS (ESI+) m/z Calcd for C24H33F2SN2O2 [M + H]+ 451.22253; Found 451.22275.
(1R,2R,8aS)-1-((5-((3,5-difluorobenzyl)thio)-1,3,4-oxadiazol-2-yl)methyl)-2,5,5,8a-tetramethyldecahydronaphthalen-2-ol (H13). Yield 60%; White solid; m. p.86–88°C. 1H NMR (400 MHz, CDCl3) δ 6.90 (dd, J = 8.2, 2.2 Hz, 2H), 6.65 (tt, J = 9.0, 2.3 Hz, 1H), 4.08 (s, 2H), 2.54–2.41 (m, 2H), 2.07 (dt, J = 11.8, 3.2 Hz, 1H), 1.91–1.79 (m, 2H), 1.65–1.58 (m, 2H), 1.49–1.38 (m, 4H), 1.39–1.27 (m, 2H), 1.26 (s, 3H), 1.02 (d, J = 2.7 Hz, 1H), 0.88 (s, 6H), 0.83 (s, 3H). 13C NMR (100 MHz, CDCl3) δ 168.1, 162.9 (d, J = 248.2 Hz), 162.7 (d, J = 248.1 Hz), 154.8, 142.6 (d, J = 9.0 Hz), 111.8 (d, J = 11.7 Hz), 111.8 (d, J = 25.4 Hz), 102.3 (d, J = 25.3 Hz), 88.9, 59.1, 56.6, 42.1, 39.4, 38.6, 36.2, 33.3, 33.1, 26.4, 21.9, 20.9, 20.7, 18.1, 15.1.19F NMR (376 MHz, CDCl3) δ -110.18, -110.18. HRMS (ESI+) m/z Calcd for C24H33F2SN2O2 [M + H]+ 451.22253; Found 451.22287.
(1R,2R,8aS)-1-((5-((4-chlorobenzyl)thio)-1,3,4-oxadiazol-2-yl)methyl)-2,5,5,8a-tetramethyldecahydronaphthalen-2-ol (H14). Yield 65%; White solid; m. p.95–96°C.
1H NMR (500 MHz, CDCl3) δ 7.38–7.34 (m, 2H), 7.32–7.28 (m, 2H), 4.37 (s, 2H), 2.89 (ddd, J = 21.7, 16.3, 5.7 Hz, 2H), 1.99–1.84 (m, 2H), 1.74–1.66 (m, 2H), 1.56–1.48 (m, 2H), 1.46–1.34 (m, 4H), 1.26 (dd, J = 13.3, 3.2 Hz, 1H), 1.20 (s, 3H), 0.99 (dd, J = 12.2, 2.1 Hz, 1H), 0.88 (s, 3H), 0.86 (s, 3H), 0.80 (s, 3H). 13C NMR (126 MHz, CDCl3) δ 170.4, 162.8, 134.5, 133.9, 130.5, 128.9, 73.3, 59.1, 55.8, 44.6, 41.6, 39.4, 38.8, 36.1, 33.4, 33.3, 23.4, 21.5, 21.2, 20.4, 18.4, 15.2. HRMS (ESI+) m/z Calcd for C24H34ClSN2O2 [M + H]+ 449.20240; Found 449.20154.
(1R,2R,8aS)-1-((5-((2-chlorobenzyl)thio)-1,3,4-oxadiazol-2-yl)methyl)-2,5,5,8a-tetramethyldecahydronaphthalen-2-ol (H15). Yield 74%; White solid; m. p.87–89°C.
1H NMR (500 MHz, CDCl3) δ 7.56 (dd, J = 7.3, 1.9 Hz, 1H), 7.39 (dd, J = 7.7, 1.3 Hz, 1H), 7.26–7.18 (m, 2H), 4.53 (s, 2H), 2.89 (ddd, J = 96.9, 16.3, 5.5 Hz, 2H), 1.92 (ddd, J = 14.8, 8.8, 4.3 Hz, 2H), 1.75–1.67 (m, 2H), 1.55–1.47 (m, 2H), 1.44–1.33 (m, 4H), 1.26 (dd, J = 13.5, 3.5 Hz, 1H), 1.20 (s, 3H), 0.99 (dd, J = 12.1, 2.0 Hz, 1H), 0.88 (s, 3H), 0.86 (s, 3H), 0.80 (s, 3H). 13C NMR (126 MHz, CDCl3) δ 170.4, 163.1, 134.3, 133.9, 131.5, 129.8, 129.6, 127.1, 73.3, 59.1, 55.8, 44.6, 41.6, 39.4, 38.9, 34.61, 33.4, 33.3, 23.3, 21.5, 21.2, 20.4, 18.4, 15.2. HRMS (ESI+) m/z Calcd for C24H34ClSN2O2 [M + H]+ 449.20240; Found 449.20117.
(1R,2R,8aS)-1-((5-((2-bromo-4-fluorobenzyl)thio)-1,3,4-oxadiazol-2-yl)methyl)-2,5,5,8a-tetramethyldecahydronaphthalen-2-ol (H16). Yield 70%; White solid; m. p.92–94°C. 1H NMR (500 MHz, CDCl3) δ 7.60 (dd, J = 8.6, 5.9 Hz, 1H), 7.32 (dd, J = 8.1, 2.6 Hz, 1H), 6.97 (td, J = 8.3, 2.7 Hz, 1H), 4.50 (s, 2H), 2.89 (ddd, J = 95.7, 16.2, 5.6 Hz, 2H), 1.95–1.87 (m, 2H), 1.68 (dd, J = 9.9, 6.5 Hz, 2H), 1.58–1.48 (m, 2H), 1.46–1.34 (m, 4H), 1.30–1.25 (m, 1H), 1.20 (s, 3H), 0.99 (dd, J = 12.2, 2.1 Hz, 1H), 0.87 (s, 3H), 0.86 (s, 3H), 0.79 (s, 3H). 13C NMR (126 MHz, CDCl3) δ 170.4, 162.9, 162.0 (d, J = 251.6 Hz), 132.6 (d, J = 8.5 Hz), 131.7 (d, J = 3.5 Hz), 124.8 (d, J = 9.7 Hz), 120.3 (d, J = 24.8 Hz), 114.9 (d, J = 21.1 Hz), 73.3, 59.1, 55.8, 44.6, 41.6, 39.4, 38.9, 36.4, 33.4, 33.3, 23.3, 21.5, 21.2, 20.4, 18.4, 15.2.19F NMR (376 MHz, CDCl3) δ -111.25. HRMS (ESI+) m/z Calcd for C24H33FBrSN2O2 [M + H]+ 511.14247; Found 511.14197.
(1R,2R,8aS)-1-((5-((3-chloro-2-fluorobenzyl)thio)-1,3,4-oxadiazol-2-yl)methyl)-2,5,5,8a-tetramethyldecahydronaphthalen-2-ol (H17). Yield 61%; White solid; m. p.79–81°C. 1H NMR (500 MHz, CDCl3) δ 7.44–7.39 (m, 1H), 7.36–7.32 (m, 1H), 7.03 (dt, J = 8.2, 4.2 Hz, 1H), 4.44 (s, 2H), 2.90 (ddd, J = 96.4, 16.3, 5.7 Hz, 2H), 1.92 (ddd, J = 14.8, 8.9, 4.4 Hz, 2H), 1.76–1.66 (m, 2H), 1.56–1.47 (m, 2H), 1.45–1.32 (m, 4H), 1.31–1.26 (m, 1H), 1.20 (s, 3H), 1.00 (dd, J = 12.2, 2.1 Hz, 1H), 0.88 (s, 3H), 0.86 (s, 3H), 0.80 (s, 3H). 13C NMR (126 MHz, CDCl3) δ 170.5, 162.6, 156.4 (d, J = 250.3 Hz), 130.5, 129.7, 125.3 (d, J = 14.4 Hz), 124.7 (d, J = 4.7 Hz), 121.3 (d, J = 17.8 Hz), 73.3, 59.1, 55.8, 44.6, 41.6, 39.4, 38.8, 33.4, 33.3, 30.0, 23.3, 21.5, 21.2, 20.4, 18.4, 15.2.19F NMR (376 MHz, CDCl3) δ -118.45. HRMS (ESI+) m/z Calcd for C24H33FClSN2O2 [M + H]+ 467.19298; Found 467.19138.
(1R,2R,8aS)-1-((5-((4-bromobenzyl)thio)-1,3,4-oxadiazol-2-yl)methyl)-2,5,5,8a-tetramethyldecahydronaphthalen-2-ol (H18). Yield 62%; White solid; m. p.99–101°C. 1H NMR (400 MHz, CDCl3) δ 7.46 (s, 1H), 7.44 (s, 1H), 7.31 (s, 1H), 7.29 (s, 1H), 4.36 (s, 2H), 2.89 (ddd, J = 77.1, 16.3, 5.7 Hz, 2H), 1.96–1.85 (m, 2H), 1.63–1.42 (m, 4H), 1.41–1.29 (m, 4H), 1.28–1.24 (m, 1H), 1.20 (s, 3H), 0.99 (dd, J = 12.1, 2.2 Hz, 1H), 0.88 (s, 3H), 0.86 (s, 3H), 0.80 (s, 3H). 13C NMR (100 MHz, CDCl3) δ 170.3, 162.7, 135.0, 131.8, 131.8, 130.8, 122.0, 122.0, 73.2, 59.0, 55.8, 44.5, 41.5, 39.4, 38.8, 36.1, 33.4, 33.2, 23.3, 21.4, 21.1, 20.4, 18.3, 15.1. HRMS (ESI+) m/z Calcd for C24H34BrSN2O2 [M + H]+ 493.15189; Found 493.15070.
(1R,2R,8aS)-1-((5-((4-methoxybenzyl)thio)-1,3,4-oxadiazol-2-yl)methyl)-2,5,5,8a-tetramethyldecahydronaphthalen-2-ol (H19). Yield 70%; White solid; m. p.86–88°C. 1H NMR (400 MHz, CDCl3) δ 7.34 (s, 1H), 7.32 (s, 1H), 6.86 (s, 1H), 6.84 (s, 1H), 4.39 (s, 2H), 3.79 (s, 3H), 2.90 (ddd, J = 78.2, 16.3, 5.6 Hz, 2H), 1.92 (ddd, J = 16.1, 8.8, 4.4 Hz, 2H), 1.77–1.65 (m, 2H), 1.60–1.49 (m, 2H), 1.46–1.33 (m, 4H), 1.29–1.23 (m, 1H), 1.20 (s, 3H), 1.00 (dd, J = 12.1, 2.2 Hz, 1H), 0.88 (s, 3H), 0.87 (s, 3H), 0.80 (s, 3H). 13C NMR (100 MHz, CDCl3) δ 170.1, 163.2, 159.3, 130.3, 127.5, 114.1, 73.2, 59.0, 55.7, 55.2, 44.4, 41.5, 39.3, 38.8, 36.4, 33.3, 33.2, 23.2, 21.4, 21.1, 20.3, 18.3, 15.1. HRMS (ESI+) m/z Calcd for C25H37SN2O3 [M + H]+ 445.25194; Found (H20). Yield 75%; White solid; m. p.98–100°C. 1H NMR (400 MHz, CDCl3) δ 7.52 (s, 1H), 4.55 (s, 2H), 2.92 (ddd, J = 75.5, 16.2, 5.7 Hz, 2H), 1.96–1.89 (m, 2H), 1.75–1.69 (m, 2H), 1.55–1.50 (m, 2H), 1.45–1.34 (m, 4H), 1.28–1.25 (m, 1H), 1.22 (s, 3H), 1.01 (dd, J = 12.2, 2.2 Hz, 1H), 0.88 (s, 3H), 0.87 (s, 3H), 0.80 (s, 3H). 13C NMR (100 MHz, CDCl3) δ 170.8, 161.9, 152.3, 140.9, 135.9, 73.2, 59.0, 55.7, 44.5, 41.5, 39.4, 38.7, 33.3, 33.2, 28.5, 23.3, 21.4, 21.1, 20.3, 18.3, 15.1. HRMS (ESI+) m/z Calcd for C21H30ClS2N3O2Na [M + Na]+ 478.13602; Found 478.13550.
(1R,2R,8aS)-1-((5-((4-bromo-2-fluorobenzyl)thio)-1,3,4-oxadiazol-2-yl)methyl)-2,5,5,8a-tetramethyldecahydronaphthalen-2-ol (H21). Yield 68%; White solid; m. p.120–122°C. 1H NMR (400 MHz, CDCl3) δ 7.40 (t, J = 8.3 Hz, 1H), 7.23 (dd, J = 7.1, 2.0 Hz, 2H), 4.38 (s, 2H), 2.89 (ddd, J = 77.6, 16.3, 5.6 Hz, 2H), 1.92 (ddd, J = 13.8, 8.7, 4.4 Hz, 2H), 1.63–1.42 (m, 4H), 1.41–1.30 (m, 4H), 1.28–1.24 (m, 1H), 1.20 (s, 3H), 1.00 (dd, J = 12.1, 2.2 Hz, 1H), 0.88 (s, 3H), 0.86 (s, 3H), 0.80 (s, 3H). 13C NMR (100 MHz, CDCl3) δ 170.4, 162.6, 160.6 (d, J = 253.0 Hz), 132.4 (d, J = 4.0 Hz), 127.6 (d, J = 3.8 Hz), 122.7 (d, J = 14.6 Hz), 122.3 (d, J = 9.5 Hz), 119.2 (d, J = 24.4 Hz), 73.2, 59.0, 55.7, 44.5, 41.5, 39.3, 38.7, 33.3, 33.2, 29.5, 23.3, 21.4, 21.1, 20.3, 18.3, 15.1.19F NMR (376 MHz, CDCl3) δ -113.84. HRMS (ESI+) m/z Calcd for C24H33BrFSN2O2 [M + H]+ 511.14247; Found 511.14252.
(1R,2R,8aS)-2,5,5,8a-tetramethyl-1-((5-((4-(trifluoromethoxy)benzyl)thio)-1,3,4-oxadiazol-2-yl)methyl)decahydronaphthalen-2-ol (H22). Yield 70%; White solid; m. p.83–85°C. 1H NMR (500 MHz, CDCl3) δ 7.45 (s, 1H), 7.43 (s, 1H), 7.16 (s, 1H), 7.14 (s, 1H), 4.39 (s, 2H), 2.88 (ddd, J = 21.7, 16.4, 5.8 Hz, 2H), 1.90 (ddd, J = 15.5, 7.8, 4.4 Hz, 2H), 1.60–1.39 (m, 4H), 1.38–1.22 (m, 4H), 1.19 (s, 3H), 1.14–1.07 (m, 1H), 0.98 (dd, J = 12.1, 2.2 Hz, 1H), 0.86 (s, 3H), 0.85 (s, 3H), 0.78 (s, 3H). 13C NMR (126 MHz, CDCl3) δ 170.4, 162.8, 148.9, 134.7, 130.7, 121.2, 120.4 (d, J = 257.8 Hz), 73.3, 59.1, 55.8, 44.6, 41.6, 39.4, 38.8, 35.9, 33.4, 33.3, 23.4, 21.5, 21.2, 20.4, 18.4, 15.2.19F NMR (376 MHz, CDCl3) δ -57.72. HRMS (ESI+) m/z Calcd for C25H33F3SN2O3 [M + H]+ 521.20562; Found 521.20575.
(1R,2R,8aS)-2,5,5,8a-tetramethyl-1-((5-((4-nitrobenzyl)thio)-1,3,4-oxadiazol-2-yl)methyl)decahydronaphthalen-2-ol (H23). Yield 53%; White solid; m. p.105–107°C. 1H NMR (500 MHz, CDCl3) δ 8.17 (s, 1H), 8.15 (s, 1H), 7.61 (s, 1H), 7.59 (s, 1H), 4.45 (s, 2H), 2.87 (ddd, J = 21.6, 16.3, 5.7 Hz, 2H), 1.94–1.83 (m, 2H), 1.60–1.39 (m, 4H), 1.38–1.29 (m, 4H), 1.18 (s, 3H), 1.13–1.06 (m, 1H), 0.96 (dd, J = 12.2, 2.1 Hz, 1H), 0.86 (s, 3H), 0.84 (s, 3H), 0.78 (s, 3H). 13C NMR (126 MHz, CDCl3) δ 170.7, 162.2, 147.5, 143.7, 130.1, 124.0, 73.3, 59.1, 55.8, 44.6, 41.6, 39.4, 38.8, 35.7, 33.4, 33.3, 23.4, 21.5, 21.2, 20.4, 18.4, 15.2. HRMS (ESI+) m/z Calcd for C24H34SN3O4 [M + H]+ 460.22645; Found 460.22681.
The in vitro antibacterial activities of target compounds H1-H23 against Xoo and Xac was evaluated by the turbidity method (Zhang et al., 2021). According to Schaad’s method (Zhang et al., 2021), the curative and protective activities of compound H8 against rice bacterial blight were determined in vivo. Based on the previous work (Wang et al., 2019; Luo et al., 2020), TMV was extracted and purified, and the interaction mode of active molecules with TMV-CP was explored by molecular docking. Detailed methods for bacterial bioactivity testing, as well as specific steps for TMV extraction and purification can be found in the Supplementary Datasheet S1.
In conclusion, a series of 1,3,4-oxadiazole contained sesquiterpene derivatives were synthesized, and the biological activity of title compounds was evaluated. The results exhibited that the synthetic compounds had good antibacterial activity against Xoo and Xac. The EC50 values of compounds H4, H8, H11, H12, H14, H16, and H19 for Xac inhibitory activity were 33.3, 42.7, 56.1, 74.5, 37.8, 43.8, and 38.4 μg/ml, respectively. Compounds H4, H8, H15, H19, H22, and H23 had inhibitory effects on Xoo, with EC50 values of 51.0, 43.3, 43.4, 50.5, 74.6, and 51.4 μg/ml, respectively. In particular, the curative and protective activities of compound H8 were 51.9 and 49.3%, respectively, showing good antibacterial activity against Xoo in vitro. In addition, the EC50 values of the inactivation activities of the compounds H4, H5, H9, H10, and H16 against TMV were 69.6, 58.9, 69.4, 43.9, and 60.5 μg/ml, respectively. It is worth noting that the molecular docking results indicated that compound H10 binds to the active site of TMV-CP through amino acid residues ASN73, VAL260, TYR139, ELU131, and THR136. And it existed a strong affinity for TMV-CP, with a binding energy of -8.88 kcal/mol. Thus, the process of self-assembly and replication of TMV particles is inhibited and the anti-TMV effect is played.
The original contributions presented in the study are included in the article/Supplementary Material, further inquiries can be directed to the corresponding author.
AD, JW conceived and designed the experiments. Synthesis and bio-assay were carried out by AD, LY, and ZZ; Computational chemistry and the analysis of docking was conducted by YH; AD, ZZ and JW analyzed the data; AD wrote the original draft; ZZ and JW reviewed and edited the manuscript.
We are grateful for the financial supports from NSFC (National Natural Science Foundation of China) (Nos. 32072445, 21762012), the Program of Introducing Talents to Chinese Universities (111 Program, D20023), and the S&T Planning ProJect of Guizhou Province (Nos. (2017) 1402, (2017) 5788).Natural Science research project of Guizhou Education Department (KY(2018)009).
The authors declare that the research was conducted in the absence of any commercial or financial relationships that could be construed as a potential conflict of interest.
All claims expressed in this article are solely those of the authors and do not necessarily represent those of their affiliated organizations, or those of the publisher, the editors and the reviewers. Any product that may be evaluated in this article, or claim that may be made by its manufacturer, is not guaranteed or endorsed by the publisher.
The Supplementary Material for this article can be found online at: https://www.frontiersin.org/articles/10.3389/fchem.2022.854274/full#supplementary-material
Aricu, A., Ciocarlan, A., Lungu, L., Barba, A., Shova, S., Zbancioc, G., et al. (2016). Synthesis, Antibacterial, and Antifungal Activities of New Drimane Sesquiterpenoids with Azaheterocyclic Units. Med. Chem. Res. 25, 2316–2323. doi:10.1007/s00044-016-1665-0
Arroo, R. (2007). Eberhard Breitmaier. Terpenes-Flavors, Fragrances, Pharmaca, Pheromones. Wiley-VCH, 2006, 214 Pp ISBN 3-527-31786-4. Appl. Organometal. Chem. 21, 377. doi:10.1002/aoc.1209
Buttimer, C., McAuliffe, O., Ross, R. P., Hill, C., O’Mahony, J., and Coffey, A. (2017). Bacteriophages and Bacterial Plant Diseases. Front. Microbiol. 8, 34. doi:10.3389/fmicb.2017.00034
Das, S. K., Patra, J. K., and Thatoi, H. (2016). Antioxidative Response to Abiotic and Biotic Stresses in Mangrove Plants: a Review. Internat. Rev. Hydrobiol. 101, 3–19. doi:10.1002/iroh.201401744
Duan, R.-T., Yang, R.-N., Li, H.-T., Tang, L.-H., Liu, T., Yang, Y.-B., et al. (2020). Peniterester, a Carotane-type Antibacterial Sesquiterpene from an Artificial Mutant Penicillium Sp. T2-M20. Fitoterapia 140, 104422. doi:10.1016/j.fitote.2019.104422
Ergün, Y., Orhan, Ö. F., Özer, U. G., and Gişi, G. (2010). Synergistic Effect of [1H-[1,2,4]Oxadiazole[4,3-A]quinoxalin-1-One] and Antidepressant Drugs in the Mouse Forced Swimming Test: Possible Involvement of Serotonergic Pathway. Eur. J. Pharmacol. 630, 74–78. doi:10.1016/j.ejphar.2009.12.021
Gan, X., Hu, D., Chen, Z., Wang, Y., and Song, B. (2017). Synthesis and Antiviral Evaluation of Novel 1,3,4-Oxadiazole/thiadiazole-Chalcone Conjugates. Bioorg. Med. Chem. Lett. 27, 4298–4301. doi:10.1016/j.bmcl.2017.08.038
Gao, C., Huang, X.-X., Bai, M., Wu, J., Li, J.-Y., Liu, Q.-B., et al. (2015). Anti-inflammatory Sesquiterpene Pyridine Alkaloids from Tripterygium Wilfordii. Fitoterapia 105, 49–54. doi:10.1016/j.fitote.2015.06.003
Graham, J. H., Gottwald, T. R., Cubero, J., and Achor, D. S. (2004). Xanthomonas axonopodispv.Citri: Factors Affecting Successful Eradication of Citrus Canker. Mol. Plant Pathol. 5, 1–15. doi:10.1046/j.1364-3703.2004.00197.x
Guo, S., Zhao, W., Wang, Y., Zhang, W., Chen, S., Wei, P., et al. (2021). Design, Synthesis, and Mechanism of Antiviral Acylurea Derivatives Containing a Trifluoromethylpyridine Moiety. J. Agric. Food Chem. 69, 12891–12899. doi:10.1021/acs.jafc.1c03586
He, F., Wei, P., Yu, G., Guo, S., Zheng, Z., Chen, S., et al. (2021). Synthesis of Trans- Methyl Ferulate Bearing an Oxadiazole Ether as Potential Activators for Controlling Plant Virus. Bioorg. Chem. 115, 105248. doi:10.1016/j.bioorg.2021.105248
Inocente, E. A., Nguyen, B., Manwill, P. K., Benatrehina, K., Kweka, E., Wu, S. J., et al. (2019). Insecticidal and Antifeedant Activities of Malagasy Medicinal Plant (Cinnamosma sp.) Extracts and Drimane-type Sesquiterpenes against aedes Aegypti Mosquitoes. Insects 10, 373. doi:10.3390/insects10110373
Jin, L. H., and Zhang, K. K. (2010). The Progress of Heterocyclic Herbicide Metabolism and Degradation, J. Guizhou Univ. (Nat. Sci. Ed. 27, 1–5. doi:10.15958/j.cnki.gdxbzrb.2010.03.014
Kumar, D., Sundaree, S., Shah, K., and Shah, K. (2009). An Efficient Synthesis and Biological Study of Novel Indolyl-1,3,4-Oxadiazoles as Potent Anticancer Agents. Bioorg. Med. Chem. Lett. 19, 4492–4494. doi:10.1016/j.bmcl.2009.03.172
Li, S. K., and Wang, X. (2021). Advance in the Synthesis and Biological Activities of the Natural Meroterpenoid Chromazonarol, J. Guizhou Univ. (Nat. Sci. Ed. 38, 1–10. doi:10.15958/j.cnki.gdxbzrb.2021.04.0110.1039/d0np00042f
Liu, C.-L., Xue, K., Yang, Y., Liu, X., Li, Y., Lee, T. S., et al. (2021). Metabolic Engineering Strategies for Sesquiterpene Production in Microorganism. Crit. Rev. Biotechnol. 42, 73–92. doi:10.1080/07388551.2021.1924112
Liu, T., Peng, F., Cao, X., Liu, F., Wang, Q., Liu, L., et al. (2021). Design, Synthesis, Antibacterial Activity, Antiviral Activity, and Mechanism of Myricetin Derivatives Containing a Quinazolinone Moiety. ACS Omega 6, 30826–30833. doi:10.1021/acsomega.1c05256
Liu, Y.-P., Yu, X.-M., Qiao, Z.-H., Jiang, B., Xie, L., Tang, H.-X., et al. (2021). Cadinane-type Sesquiterpenes with Potential Anti-inflammatory and Anti-HIV Activities from the Stems and Leaves of Mappianthus Iodoides. Nat. Product. Res. 1-7, 1–7. doi:10.1080/14786419.2021.1956921
Lungu, L. (2015). Synthesis of New Nitrogen-Containing Drimane and Homodrimane Sesquiterpenoids from Sclareolide. ChemJMold 10, 58–61. doi:10.19261/cjm.2015.10(2).07
Luo, D., Guo, S., He, F., Chen, S., Dai, A., Zhang, R., et al. (2020). Design, Synthesis, and Bioactivity of α-Ketoamide Derivatives Bearing a Vanillin Skeleton for Crop Diseases. J. Agric. Food Chem. 68, 7226–7234. doi:10.1021/acs.jafc.0c00724
Mai, J., Li, W., Ledesma-Amaro, R., and Ji, X.-J. (2021). Engineering Plant Sesquiterpene Synthesis into Yeasts: a Review. J. Agric. Food Chem. 69, 9498–9510. doi:10.1021/acs.jafc.1c03864
Mishra, C. B., Mongre, R. K., Kumari, S., Jeong, D. K., and Tiwari, M. (2017). Novel Triazole-Piperazine Hybrid Molecules Induce Apoptosis via Activation of the Mitochondrial Pathway and Exhibit Antitumor Efficacy in Osteosarcoma Xenograft Nude Mice Model. ACS Chem. Biol. 12, 753–768. doi:10.1021/acschembio.6b01007
Naseer, M. A., Ali, Z., and Husain, A. (2019). Design, Synthesis, Anti-inflammatory and Analgesic Studies of Novel 1,3,4- Oxadiazole-Thione Derivatives. Ajbpr 9, 49–60. doi:10.24214/ajbpr/9/1/4960
Padejjar Vasantha, S., Poojary, B., and Bistuvalli Chandrashekarappa, R. (2019). Novel Arylpyridine‐based 1,3,4‐oxadiazoles: Synthesis, Antibacterial, and Anti‐inflammatory Evaluation. J. Chin. Chem. Soc. 66, 638–650. doi:10.1002/jccs.201800248
Parizadeh, N., Alipour, E., Soleymani, S., Zabihollahi, R., Aghasadeghi, M. R., Hajimahdi, Z., et al. (2018). Synthesis of Novel 3-(5-(alkyl/arylthio)-1,3,4-Oxadiazol-2-Yl)-8-Phenylquinolin-4(1h)-One Derivatives as Anti-HIV Agents. Phosphorus, Sulfur, Silicon Relat. Elem. 193, 225–231. doi:10.1080/10426507.2017.1394302
Platon, L., Cao, J., and Ménard, D. (2021). Compensating P. Falciparum Artemisinin Resistance. Cell Host & Microbe 29, 1732–1734. doi:10.1016/j.chom.2021.11.007
Ren, X., Li, X., Yin, L., Jiang, D., and Hu, D. (2020). Design, Synthesis, Antiviral Bioactivity, and Mechanism of the Ferulic Acid Ester-Containing Sulfonamide Moiety. ACS Omega 5, 19721–19726. doi:10.1021/acsomega.0c02421
Ryu, B., Kim, H. M., Lee, J.-S., Cho, Y. J., Oh, M. S., Choi, J.-H., et al. (2015). Sesquiterpenes from Rhizomes of Cyperus Rotundus with Cytotoxic Activities on Human Cancer Cellsin Vitro. Hca 98, 1372–1380. doi:10.1002/hlca.201500117
Sacchettini, J. C., and Poulter, C. D. (1997). Creating Isoprenoid Diversity. Science 277, 1788–1789. doi:10.1126/science.277.5333.1788
Shang, S.-Z., Zhao, W., Tang, J.-G., Xu, X.-M., Sun, H.-D., Pu, J.-X., et al. (2016). Antiviral Sesquiterpenes from Leaves of Nicotiana Tabacum. Fitoterapia 108, 1–4. doi:10.1016/j.fitote.2015.11.004
Sparks, T. C., Hunter, J. E., Lorsbach, B. A., Hanger, G., Gast, R. E., Kemmitt, G., et al. (2018). Crop protection Discovery: Is Being the First Best. J. Agric. Food Chem. 66, 10337–10346. doi:10.1021/acs.jafc.8b03484
Wang, M., Zhao, L., Chen, K., Shang, Y., Wu, J., Guo, X., et al. (2020). Antibacterial Sesquiterpenes from the Stems and Roots of Thuja Sutchuenensis. Bioorg. Chem. 96, 103645. doi:10.1016/j.bioorg.2020.103645
Wang, S., Chen, J., Shi, J., Wang, Z., Hu, D., and Song, B. (2021). Novel Cinnamic Acid Derivatives Containing the 1,3,4-oxadiazole Moiety: Design, Synthesis, Antibacterial Activities, and Mechanisms. J. Agric. Food Chem. 69, 11804–11815. doi:10.1021/acs.jafc.1c03087
Wang, X., Chai, J., Kong, X., Jin, F., Chen, M., Yang, C., et al. (2021). Expedient Discovery for Novel Antifungal Leads: 1,3,4-oxadiazole Derivatives Bearing a Quinazolin-4(3h)-One Fragment. Bioorg. Med. Chem. 45, 116330. doi:10.1016/j.bmc.2021.116330
Wang, Y.-Y., Xu, F.-Z., Zhu, Y.-Y., Song, B., Luo, D., Yu, G., et al. (2018). Pyrazolo[3,4-d]pyrimidine Derivatives Containing a Schiff Base Moiety as Potential Antiviral Agents. Bioorg. Med. Chem. Lett. 28, 2979–2984. doi:10.1016/j.bmcl.2018.06.049
Wang, P.-Y., Wang, M.-W., Zeng, D., Xiang, M., Rao, J.-R., Liu, Q.-Q., et al. (2019). Rational Optimization and Action Mechanism of Novel Imidazole (Or Imidazolium)-Labeled 1,3,4-oxadiazole Thioethers as Promising Antibacterial Agents against Plant Bacterial Diseases. J. Agric. Food Chem. 67, 3535–3545. doi:10.1021/acs.jafc.8b06242
Wang, Y., Xu, F., Luo, D., Guo, S., He, F., Dai, A., et al. (2019). Synthesis of Anthranilic Diamide Derivatives Containing Moieties of Trifluoromethylpyridine and Hydrazone as Potential Anti-viral Agents for Plants. J. Agric. Food Chem. 67, 13344–13352. doi:10.1021/acs.jafc.9b05441
Wen, L., Jian, W., Shang, J., and He, D. (2019). Synthesis and Antifungal Activities of Novel Thiophene-Based Stilbene Derivatives Bearing an 1,3,4-oxadiazole Unit. Pest Manag. Sci. 75, 1123–1130. doi:10.1002/ps.5229
Wu, J., Hu, D. Y., and Yang, S. (2011). Research Progress in Herbicidal Activity of Three-Membered Heterocyclic Derivatives. J. Guizhou Univ. (Nat. Sci. Ed. 28, 1–5. doi:10.15958/j.cnki.gdxbzrb.2011.04.002
Wu, Z. B., Zhang, T. T., Wu, S. X., Kuang, J. Q., and He, X. F. (2013). Antivirus Activity of N-(1,4-substitued Pyrazole-Yl)-,2,3-Thiadiazole Carboxamide Derivatives. J. Guizhou Univ. (Nat. Sci. Ed. 30, 9–12. doi:10.15958/j.cnki.gdxbzrb.2013.04.025
Yang, Z., Li, P., He, Y., Luo, J., Zhou, J., Wu, Y., et al. (2020). Design, Synthesis, and Biological Evaluation of Novel Pyrethrin Derivatives Containing 1,3,4-oxadiazole and Thioether Moieties as Active Insecticidal Agents. Chem. Pap. 74, 1621–1632. doi:10.1007/s11696-019-01012-4
Yu, G., Chen, S., Guo, S., Xu, B., and Wu, J. (2021). Trifluoromethylpyridine 1,3,4-oxadiazole Derivatives: Emerging Scaffolds as Bacterial Agents. ACS Omega 6, 31093–31098. doi:10.1021/acsomega.1c04472
Zhang, M.-Z., Mulholland, N., Beattie, D., Irwin, D., Gu, Y.-C., Chen, Q., et al. (2013). Synthesis and Antifungal Activity of 3-(1,3,4-Oxadiazol-5-Yl)-Indoles and 3-(1,3,4-Oxadiazol-5-Yl)methyl-Indoles. Eur. J. Med. Chem. 63, 22–32. doi:10.1016/j.ejmech.2013.01.038
Zhang, R., Deng, P., Dai, A., Guo, S., Wang, Y., Wei, P., et al. (2021b). Design, Synthesis, and Biological Activity of Novel Ferulic Amide Ac5c Derivatives. ACS Omega 6, 27561–27567. doi:10.1021/acsomega.1c04644
Zhang, R., Guo, S., Deng, P., Wang, Y., Dai, A., and Wu, J. (2021a). Novel Ferulic Amide Ac6c Derivatives: Design, Synthesis, and Their Antipest Activity. J. Agric. Food Chem. 69, 10082–10092. doi:10.1021/acs.jafc.1c03892
Zhang, S., Jia, Q., Gao, Q., Fan, X., Weng, Y., and Su, Z. (2018). Dual-specificity Phosphatase CDC25B Was Inhibited by Natural Product HB-21 through Covalently Binding to the Active Site. Front. Chem. 6, 531. doi:10.3389/fchem.2018.00531
Zhao, L., Dong, J., Hu, Z., Li, S., Su, X., Zhang, J., et al. (2017). Anti-TMV Activity and Functional Mechanisms of Two Sesquiterpenoids Isolated from Tithonia Diversifolia. Pestic. Biochem. Physiol. 140, 24–29. doi:10.1016/j.pestbp.2017.05.009
Keywords: sesquiterpene derivatives, 1,3,4-oxadiazole, synthesis, rice bacterial blight, tobacco mosaic virus, biological activity
Citation: Dai A, Zheng Z, Yu L, Huang Y and Wu J (2022) 1,3,4-Oxadiazole Contained Sesquiterpene Derivatives: Synthesis and Microbiocidal Activity for Plant Disease. Front. Chem. 10:854274. doi: 10.3389/fchem.2022.854274
Received: 13 January 2022; Accepted: 24 January 2022;
Published: 22 February 2022.
Edited by:
Yaqiong Su, Xi’an Jiaotong University, ChinaReviewed by:
Song Bai, Guizhou Institute of Technology, ChinaCopyright © 2022 Dai, Zheng, Yu, Huang and Wu. This is an open-access article distributed under the terms of the Creative Commons Attribution License (CC BY). The use, distribution or reproduction in other forums is permitted, provided the original author(s) and the copyright owner(s) are credited and that the original publication in this journal is cited, in accordance with accepted academic practice. No use, distribution or reproduction is permitted which does not comply with these terms.
*Correspondence: Jian Wu, d3VqaWFuMjY5MUAxMjYuY29t
Disclaimer: All claims expressed in this article are solely those of the authors and do not necessarily represent those of their affiliated organizations, or those of the publisher, the editors and the reviewers. Any product that may be evaluated in this article or claim that may be made by its manufacturer is not guaranteed or endorsed by the publisher.
Research integrity at Frontiers
Learn more about the work of our research integrity team to safeguard the quality of each article we publish.