- 1Central Laboratory, Tianjin Stomatological Hospital, School of Medicine, Nankai University, Tianjin, China
- 2Tianjin Key Laboratory of Oral and Maxillofacial Function Reconstruction, Tianjin, China
Chemiluminescence (CL), a distinct luminescent process by taking advantage of chemical reactions rather than external light source, has recently attracted considerable research interests due to its high sensitivity and low background signal. The sensitivity and specificity of chemiluminescent signals in complex tumor microenvironment provide a sound basis for accurate detection of tumors. Various chemiluminescent nanoprobes with superior performance have been obtained by structural modification of chemiluminescent units or introduction of fluorescent dyes. In this review, we focused on the recent progress of chemiluminescent polymeric systems based on various chromophore substrates, including luminol, peroxyoxalates, 1, 2-dioxetanes and their derivatives for tumor detecting. And we also emphasized the design strategies, mechanisms and diagnostic applications of representative chemiluminescent polymeric nanoprobes. Finally, the critical challenges and perspectives of chemiluminescent systems usage in tumor diagnosis were also discussed.
Introduction
Optical imaging plays a vital role in early diagnosis and treatment of diseases. It not only visualizes the detection of lesion sites, but also improves the accuracy of disease treatment (Thomas, 2015). However, due to tissue self-illumination, low tissue penetration depth and less noise interference, conventional optical imaging limits its application in living organisms (Li and Pu, 2019). Chemiluminescence refers to the process in which luminescence is generated through chemical reactions without external light source or other energy. In brief, chemical substances are oxidized into unstable high-energy intermediates, which subsequently disintegrate to emit light or transfer energy to surrounding fluorophores (Vacher et al., 2018). Chemiluminescence possesses the advantages of high sensitivity, deep tissue penetration depth, without external light source required, and high signal-to-background ratio, which provides new methods and ideas for the further development of optical imaging technology (Gnaim et al., 2018).
Chemiluminescence can be classified into two types, namely direct chemiluminescence and indirect chemiluminescence according to the energy conversion principle of luminescence (Yan et al., 2019). Direct chemiluminescence refers to the oxidation of a chemiluminescent substrate to form an excited-state intermediate with high energy, which then returns to the ground state to release photons, followed by the emission of light. The most representative is the luminol chemiluminescent system (Li et al., 2022). Indirect chemiluminescence generally involes chemiluminescence resonance energy transfer (CRET) process. 1, 2-dioxetane derivatives or peroxyoxalates are widely used as typical indirect CL systems for biomedical assays (Su et al., 2019; Tzani et al., 2021).
Despite the rapid development of chemiluminescence, the application of chemiluminescent substrate in biomedical fields is still limited compared to fluorescence due to its hydrophobicity, weak signal, and fast decay (Miao and Pu, 2018). To overcome these shortcomings, chemiluminescence systems combined with nanotechnology or different modifications have emerged and aroused the wide interest (Tiwari and Dhoble, 2018). In recent years, polymeric nanoparticles have attracted extensive attention due to their high brightness and high quantum yield, as well as their low toxicity, good biocompatibility and various synthesis methods (Kaeser and Schenning, 2010). This review aims to sum up recent advances in chemiluminescent polymeric nanoprobes based on three chromophore substrates, including luminol, peroxyoxalates, and 1, 2-dioxetanes. Moreover, the design strategies and luminescence mechanisms of different chemiluminescent polymeric nanoprobes are discussed, and their applications in tumor diagnosis are further elaborated.
Luminol-based chemiluminescent polymeric nanoprobes
Luminol (5-amino-2, 3-dihydrophthalazine-1, 4-dione) and its derivatives are currently the most classical chemiluminescent reagents. In 1928, Albrecht (1928) discovered that luminol could emit CL when reacting with oxidizing agents such as hydrogen peroxide (H2O2) in alkaline media. Despite its low detection limit and good selectivity, the luminol chemiluminescent probe still suffers from a short emission wavelength (∼425 nm) and limited tissue penetration depth, hindering the application of this system in bioimaging in vivo (Zhang et al., 2013). The chemiluminescent properties of luminol itself are susceptible to external factors, therefore, nanomaterials with different catalytic properties are often used to modulate the performance of this chemiluminescent system. Graphene oxide was utilized to amplify the CL signal of luminol (Yang et al., 2014). Huang et al. (2006) demonstrated effective CRET between luminol and CdTe quantum dots and the red shift of the luminescence wavelength to the quantum dots in the luminol/H2O2 CL system. In addition, the researchers obtained luminol-based chemiluminescenct polymeric systems with improved properties by modifying luminol or linking it with different fluorescent dyes.
Reactive oxygen species are critical for cancer development, progression, and metastasis, thus early detection of ROS within cancer cells is crucial for the monitoring and management of cancers. Abnormal superabundant generation of H2O2, a major ROS, has been closely associated with cancer development (Trachootham et al., 2009). An et al. (2020) utilized luminol and poly (ethylene glycol) to simultaneously couple chlorin e6 to form an amphiphilic conjugate (defined as CLP), followed by the self-assembly of CLP to form luminescent nanoparticles. These nanoparticles with core-shell nanostructures could be activated by H2O2, leading to CL imaging and in situ photodynamic therapy (PDT) of tumors with high expression of H2O2, shown in Figures 1A, B. Nan et al. (2019) designed a polycarbonate copolymer (PMPC-ONA) micelle decorated with benzyl alcohol and then luminol, fluorophore and heme were encapsulated into the micelle to form the L/H/S@PMPC-ONA nanoprobe for H2O2 imaging in vivo. Once H2O2 encountered the heme in the nanoparticles, ROS were generated, and then luminol would be excited to trigger chemiluminescence, while the stability of the nanoparticles decreases, thus releasing the fluorescent indicator to detect H2O2. Chemiluminescence exhibits the potential for ROS detection since no excitation light source needs to be involved and the relationship between light emission and analyte concentration is explicit. Lee et al. (2016) designed hydrogen peroxide responsive hybrid nanoparticles (HNPs) consisting of the PEGylated QDs and a luminol derivative (L012) as the CL agent. The energy transduction owning to the reaction between L012 and H2O2 enabled HNPs to achieve light production. Furthermore, as a promising diagnostic agent for cancer, HNPs displayed non-invasive near-infrared (NIR) imaging of H2O2 in vivo without background interference. Endogenous photoactivation is also of great importance for the diagnosis and treatment of tumors. Mao et al. (2021) constructed two gold nanoparticles (tAuNP and makuNP) by modifying 2, 5-diphenyltetrazole and methacrylic acid on the surface of gold nanoparticles for tumor imaging and therapy. The mAuNPs were absorbed with luminol to form self-illuminating mAuNP/Lu nanoparticles. Owning to the tetrazole/alkene cycloaddition, H2O2-initiated mAuNP/Lu nanoparticles could specifically crosslink with tAuNP nanoparticles to generate large particle aggregates. Subsequently, these aggregates contributed to strong CL effect catalyzed by H2O2 in tumor microenvironment, leading to strengthened uptake and retention of AuNPs.
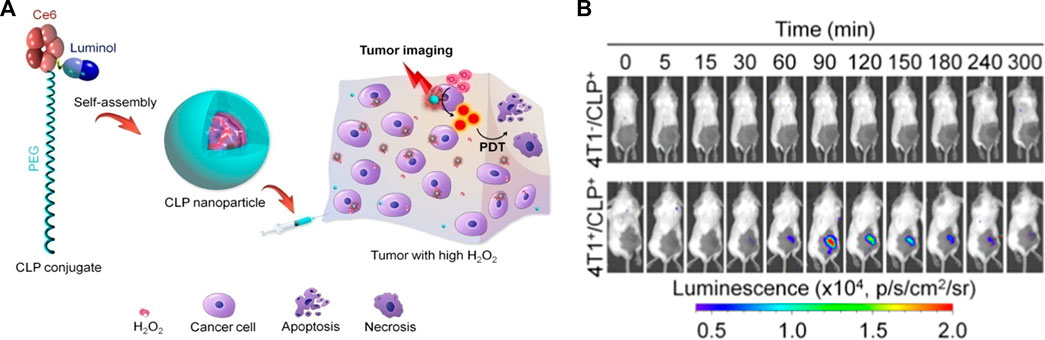
FIGURE 1. (A) Schematic illustration of CL imaging of tumors with high H2O2 and H2O2-mediated photodynamic therapy using CLP nanoparticles. (B) Time-dependent in vivo CL imaging of healthy mice and 4T1 tumor-bearing mice after local injection of CLP nanoparticles (An et al., 2020).
The specific identification of tumor markers in the blood and tissues of cancer patients is significant for early cancer diagnosis (Freedland, 2011). Yan et al. (2022) constructed a dual-carrier CL sensor that recognized tumor markers through an enzyme digestion CL signal amplification strategy. This sensor underwent aggregation and identified target markers, and then strong CL signals were collected under luminol catalysis reaction. Zhang et al. (2016) designed a novel signal enhancement strategy in which CRET occurred between reduced graphene oxide (rGO) as an energy acceptor and luminol as a donor. And the probe provided a more sensitive signal amplification strategy. Additionally, Ag-C3N4 nanosheet loaded with luminol capped AuNPs nanocomposite was developed as the electrochemiluminescence (ECL) signal nanoprobe for early tumor diagnosis (Liu et al., 2021). The CL signal could be obviously enhanced by cells in luminol-H2O2 system, and the signal intensity and the cell concentration were positively correlated. Based on the above phenomena, Ding et al. (2020) designed a new cell-assisted enhanced CL strategy to identify tumor cells rapidly. The above probes provided highly accurate and quantitative analysis of tumor markers in complex biological samples, showing great potential for clinical application.
Peroxyoxalate-based chemiluminescent polymeric nanoprobes
The reaction of anthracene, 9, 10-diphenylanthracene and N-methylacridine with hydrogen peroxide and oxalyl chloride can produce a “blue-white light,” which is known as peroxyoxalate chemiluminescence (PO-CL), firstly discovered by Chandross (1963). PO-CL modulates the emission wavelength by adding different fluorophores to the reaction system, rather than modifying the CL molecule. PO-CL systems belong to indirect chemiluminescence, generally consists of oxalate, oxidant (usually hydrogen peroxide) and suitable dye. The formation of high-energy 1, 2-dioxadione intermediates is a key step leading to chemiluminescence emission (Delafresnaye et al., 2020). The three most commonly used oxalate eaters are TCPO (bis (2, 4, 6-trichlorophenyl) oxalate), CPPO (bis (2, 4, 5-trichlorophenyl-6-carbopentoxyphenyl) oxalate) and DNPO (bis (2, 4-dinitrophenyl) oxalate) (Lee et al., 2012). The detection of H2O2 in water, microorganisms in food, and blood samples in crime scenes all rely on the PO-CL reaction (García and Baeyens, 2000). Thanks to its high sensitivity, high quantum yield and long luminescence lifetime, peroxyoxalate-based chemiluminescence systems have been widely used in bioimaging and tumor therapy (Yang and Zhang, 2021).
PO-CL systems are usually designed as nanoparticles for in vivo bioimaging because of their susceptibility to decomposition when exposed to water. Shuhendler et al. (2014) developed a nanoparticle based on a dual-channel imaging function for imaging liver injury in mice, i.e., CRET-based peroxyoxalate chemiluminescence for H2O2 detection and fluorescence resonance energy transfer (FRET)-based fluorescence for ONOO− and hypochlorite detection. The probe allowed for rapid and real-time direct assessment of acute hepatotoxicity. Zhen et al. (2016) constructed a H2O2-responsive chemiluminescent semiconducting polymer nanoparticle (SPN) using TCPO and applied it to the detection of endogenous H2O2 in peritonitis and neuroinflammation. With high brightness and proper near-infrared window, the SPN achieved ultrasensitive detection of H2O2. A PO-CL system based on CPPO with cascaded CRET and FRET processes was designed to permit near-infrared region II (NIR-II) chemiluminescence imaging in arthritic mice (Yang et al., 2020). The strategy avoided the loss of energy transfer as much as possible through the rational design of the probe.
Chemiluminescent polymeric nanoprobes that react with H2O2 within the tumor microenvironment may achieve accurate imaging of tumors. Yu et al. (2022) combined pluronic F-127 and polymer containing oxalate ester (POE) to form nanoparticles by means of hydrophilicity and hydrophobicity. The nanoparticles loaded anti-tumor drug could realize the tumor tracking by H2O2-related chemiluminescence and the tumor therapy by drug releasing, which possessed great potential for precise localization and efficient treatment of tumor. In addition, since tumor cells consume more glucose than normal tissues, the glucose level of tumor tissues ware also utilized to enable tumor detection and imaging (Li et al., 2019).
Apart from disease diagnosis related to hydrogen peroxide overproduction, the CL platform can be used to perform PDT, avoiding the limitations of external light sources and light penetration depth (Yuan et al., 2012). Excessive hydrogen peroxide is an intrinsic feature of tumor cells. Andrey et al. took advantage of this feature to designed and synthesised a dispersion composed of polyoxalate and tetramethylhematoporphyrin (TMHP). In the presence of TMHP, singlet oxygen (1O2) formation by reaction with endogenous H2O2 through PO-CL leaded to tumor cell elimination (Romanyuk et al., 2017). Wu et al. (2019) came up with a self-luminescing nanoreactor by coencapsulating CPPO, poly [(9,9′-dioctyl-2,7-divinylene-fluorenylene)-alt-2-methoxy-5-(2-ethyl-hexyloxy)-1,4-phenylene] (PFPV), and the photosensitizer tetraphenylporphyrin with polyethylene glycol-polycaprolactone (PEG-PCL) and folate-PEG-cholesterol. This novel system could achieve self-luminescence emission and singlet oxygen (1O2) production, which had important implications for imaging and treatment of cancer. Most conventional photosensitizers usually suffer from aggregation-caused quenching (ACQ) effects, resulting in reduced luminescence intensity and ROS generation (Li et al., 2021). The emergence of aggregation-induced emission luminogens (AIEgens) exhibiting enhanced fluorescence intensity and ROS production largely solves the above problems. Mao et al. developed a novel nanoparticle (C-TBD NPs) with chemiexcited far-red/NIR emission and 1O2 production capability using pluronic F127 and soybean oil co-loaded with CPPO and TBD, a photosensitizer with AIE properties. C-TBD NPs could emit chemiluminescence in response to hydrogen peroxide at the tumor site, thus enabling precise tumor tracking in vivo. In addition, C-TBD NPs also produced effective 1O2 to induce apoptosis of tumor cells (Mao et al., 2017).
Chemodynamic therapy (CDT), a new class of oncology therapeutic techniques based on the iron-based Fenton reaction, offers an alternative opportunity for cancer treatment (Yang et al., 2019). In order to enhance therapeutic efficiency and minimize side effects, the real-time monitoring of ROS production during CDT is extremely essential. However, CDT reagents that can emit ROS-associated signals are rare. Wang et al. (2019) constructed a semiconducting polymer nanoplatform containing CPPO, hemin and glucose oxidase (GOD). This ROS-dependent chemiluminescence of SPN allowed optical monitoring of intra-tumor ROS production during CDT. This nanoplatform represented the first intelligent strategy to enable chemiluminescence imaging for monitoring CDT, demonstrating great potential in assessing treatment responsiveness and predicting early treatment outcomes.
1, 2-dioxetane-based chemiluminescent polymeric nanoprobes
Chemiluminescent systems based on luminol and peroxyoxalates require the involvement of oxidants, making them often used for the detection of active species, which limits their scope of application. 1, 2-dioxetanes do not require the involvement of additional oxidants (hydrogen peroxide, oxygen, potassium permanganate, etc.), which simplifies the analytical process, improves detection sensitivity, and expands the field of chemiluminescence applications (Kopecky and Mumford, 1969; Schaap et al., 1989). Schaap’s group found that the deprotonation of the phenolic hydroxyl substituent could transform 1, 2-dioxetane into a superior luminescent intermediate in 1982 (Schaap and Gagnon, 1982). Subsequently, Schaap’s group further improved the thermal stability of 1, 2-dioxetane derivatives by introducing adamantane substituents (Schaap et al., 1987). However, previous 1, 2-dioxetane CL systems had a great tendency to be quenched by water, resulting in short emission wavelength and low luminescence intensity, which made it difficult to be applied in vivo.
To enhance the chemiluminescence intensity, the researchers have made numerous attempts, i.e., simple or complex modifications of the dioxetane scaffold: 1) the processing of dioxetane units into polymer monomers (Gnaim and Shabat, 2017). 2) the covalent binding of fluorescent dyes with higher fluorescence efficiency to Schaap’s 1, 2 dioxetanes (Matsumoto, 2004; Hananya et al., 2016). 3) the introduction of electron-withdrawing groups in the neighboring positions of phenoxy 1, 2-dioxetane (Eilon et al., 2018). Based on the above basic research, the researchers designed and constructed various chemiluminescent polymeric systems based on 1, 2-dioxetanes, which showed excellent performance in cancer detection.
Afterglow luminescence is a process of persistent luminescence after the cessation of light excitation, and afterglow imaging holds great promise in the biomedical fields. Ni et al. constructed a NIR afterglow luminescent nanoparticle (AGL AIE dots) with AIE characteristics by encapsulating the NIR emissive AIE molecule (TPE-Ph-DCM) and the enol ether precursor of Schaap’s 1, 2-dioxetane with Lipid-PEG2000 through nanoprecipitation. The AGL AIE dots finally emitted NIR afterglow luminescence through the generation of 1O2 by TPE-Ph-DCM, the formation and decomposition of dioxetane, the release of chemical energy, and the energy transfer to TPE-Ph-DCM, which lasted for more than 10 days after single light excitation through self-cycling luminescence mechanism. The afterglow luminescent signal of AGL AIE dots was extremely quenched in the liver, therefore, AGL AIE dots had great prospects in the application of accurate image-guided tumor surgery, shown in Figure 2A (Ni et al., 2018). Immunotherapy, an emerging cancer treatment in recent years, offers new treatment options for cancer patients, yet the response rate of patients in clinical applications has not been significant (Sambi et al., 2019). In order to improve the efficacy of immunotherapy, monitoring the immune responses of patients is inevitable. Cui et al. (2020) constructed semiconducting polymeric nanoreporters (SPNRs) by means of combining the semiconducting polymer and the dioxetane derivative. As the first reporter that could release chemiluminescent signals activated by superoxide anions, SPNRs could sensitively distinguish immune cells containing high O2-levels from other cells including cancer cells and normal cells, enabling real-time imaging of immune activation during cancer immunotherapy in vivo. The aforementioned PDT is a promising strategy for cancer therapy. Fan et al. (2021) designed nanoparticles that specifically respond to alkaline phosphatase overexpressed on hepatocellular carcinoma cells, ultimately producing 1O2 and NIR fluorescence for tumor diagnosis and treatment.
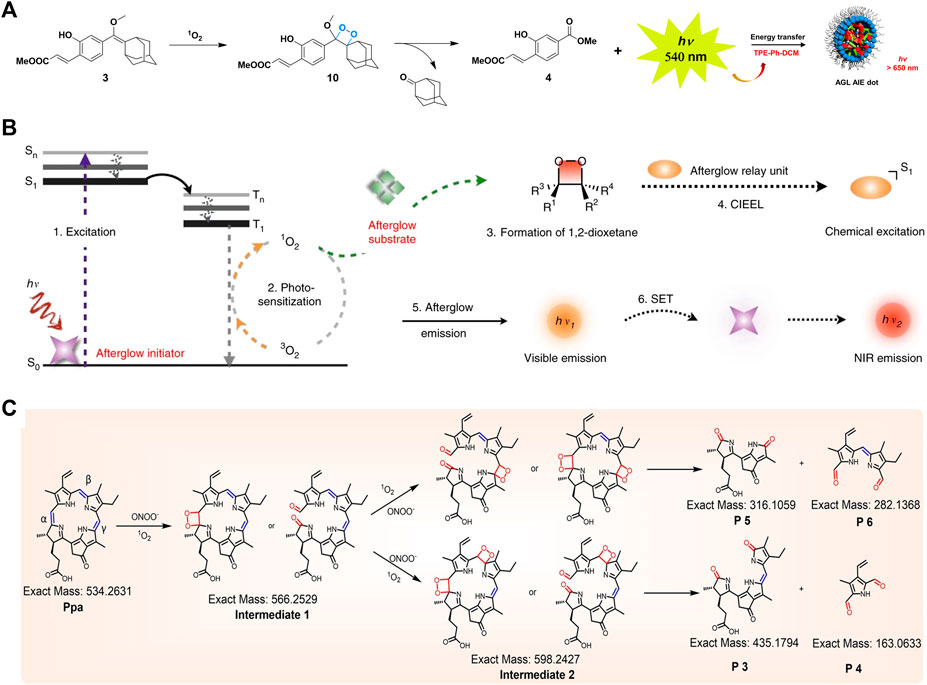
FIGURE 2. (A) Schematic diagram of the mechanism for NIR afterglow of the AGL AIE Dot (Ni et al., 2018). (B) Schematic diagram of the mechanism for intraparticle afterglow luminescence of ALNPs (Jiang et al., 2019). (C) Proposed mechanism for persistent luminescence of pheophorbide A (Duan et al., 2022).
In addition to the direct addition of dioxetane units, some chemiluminescence systems can also emit chemiluminescence by forming unstable dioxetane intermediates in response to the tumor microenvironment. Miao et al. (2017) applied PEG-b-PPG-b-PEG coated poly (2methoxy-5-(2-ethylhexyloxy)-1, 4-phenylenevinylene) (MEHPPV) to prepare SPNs to amplify and redshift the afterglow effectively. Under the oxidation of PPV, SPNs generated unstable dioxolane intermediates, which slowly degraded into PPV aldehyde and emitted afterglow. This strategy could be applied to the detection of lymph nodes and tumors in living mice. Jiang et al. (2019) demonstrated an excellent method for converting ordinary fluorescent agents into afterglow luminescent nanoparticles (ALNPs) by forming unstable 1, 2-dioxetanes intermediates in order to detect tumors in vivo rapidly. This method consisted of the following steps: the photosensitizer absorbed light and converted it into 1O2, then the reactive molecule reacted with 1O2 to generate unstable 1, 2-dioxetane, and event ually the luminescence emitted by receiving the energy from 1, 2-dioxetane through semiconducting polymer, shown in Figure 2B. Lu et al. (2020) developed a novel chemiluminescent polymeric system (ultrathin MnOx-SPNs) with both CDT and pH responsiveness. The 1O2 produced by MnOx in response to the acidic tumor microenvironment reacted with SP, followed by forming thiophene-dioxetane intermediates and transferring energy to SPNs, leading to real-time monitoring of 1O2 generation and ratiometric CL/FL imaging for guiding cancer therapy. Duan et al. (2022) demonstrated persistent luminescence was detected from porphyrins after stopping the excitation light or reacting with peroxynitrite, verifying the successive oxidation of vinylene bonds may form unstable dioxetane intermediates. Such supramolecular probes could realize the light-triggered function conversion from photoacoustic imaging to persistent luminescence imaging, resulting in the successful implementation of image-guided tumor surgery, shown in Figure 2C. The mechanisms, advantages and disadvantages of the three chemiluminescent substrates were shown in Table 1.
Discussion
This article briefly reviewed recent developments of chemiluminescent polymeric nanosystems based on the chemiluminescence substrates including luminol, peroxyoxalates and 1, 2-dioxetanes. Moreover, luminescence mechanisms, design strategies and applications in biosensing and tumor diagnosis of chemiluminescent polymeric nanosystems were described in detail. An increasing number of studies have been performed to modify these three chemiluminescent substrates by direct or indirect means to improve luminescence efficiency and intensity for highly sensitive and precise quantitative analysis of solid tumors and even tumor markers. However, despite the remarkable progress in a wide range of fields, there still exist some problems that need to be solved and broken through: 1) Chemiluminescent systems based on 1, 2-dioxetanes have limited their biological applications due to their complex synthetic routes and short emission wavelengths. 2) Similar to water-soluble and lipid-soluble fluorescent dyes, the released dye tends to diffuse from the reaction site when a chemiluminescent probe is activated, making it more difficult to provide in situ information for imaging. The introduction of a second near-infrared (NIR-II) window (1,000–1,700 nm) into chemiluminescence imaging can achieve deeper penetration depths and higher signal-to-noise ratios, which is certainly helpful to solve the above issues. In addition, finding more stable fluorophores with higher antioxidant capacity and increasing the fluorescence quantum yield of fluorophores can maintain good efficiency of chemiluminescence, and the choice of enzymes-initiated chemiluminescence systems to avoid ROS/RNS oxidation is also an effective means. Over the past few years, chemiluminescent polymeric nanoprobes have developed significantly as imaging analytical tools for tumor diagnosis. In addition to tumor tissue imaging, the chemical flexibility of chemiluminescent polymeric nanoprobes holds great promise for their application in multifunctional therapeutic platforms.
Author contributions
XZ designed and wrote the manuscript. CL, WC, and GW searched articles relating to the subject. HZ and HL supervised the whole work. All authors contributed to the article and approved the submitted version.
Funding
The work was supported by the National Nature Science Foundation of China (32201178), Science and Technology Project of Tianjin Health Commission (TJWJ2021QN068) and Tianjin Key Medical Discipline (Specialty) Construction Project (TJYXZDXK-048A and TJYXZDXK-078D).
Conflict of interest
The authors declare that the research was conducted in the absence of any commercial or financial relationships that could be construed as a potential conflict of interest.
Publisher’s note
All claims expressed in this article are solely those of the authors and do not necessarily represent those of their affiliated organizations, or those of the publisher, the editors and the reviewers. Any product that may be evaluated in this article, or claim that may be made by its manufacturer, is not guaranteed or endorsed by the publisher.
References
Albrecht, H. O. (1928). Über die Chemiluminescenz des Aminophthalsäurehydrazids. Z. für Phys. Chem. 136 (1), 321–330. doi:10.1515/zpch-1928-13625
An, H., Guo, C., Li, D., Liu, R., Xu, X., Guo, J., et al. (2020). Hydrogen peroxide-activatable nanoparticles for luminescence imaging and in-situ triggerable photodynamic therapy of cancer. ACS Appl. Mater. Interfaces 12 (15), 17230–17243. doi:10.1021/acsami.0c01413
Chandross, E. A. (1963). A new chemiluminescent system. Tetrahedron Lett. 4 (12), 761–765. doi:10.1016/b978-1-4831-9886-6.50153-4
Cui, D., Li, J., Zhao, X., Pu, K., and Zhang, R. (2020). Semiconducting polymer nanoreporters for near-infrared chemiluminescence imaging of immunoactivation. Adv. Mater. 32 (6), 1906314. doi:10.1002/adma.201906314
Delafresnaye, L., Bloesser, F. R., Kockler, K. B., Schmitt, C. W., Irshadeen, I. M., and Barner-Kowollik, C. (2020). All eyes on visible-light peroxyoxalate chemiluminescence read-out systems. Chemistry-A Eur. J. 26 (1), 114–127. doi:10.1002/chem.201904054
Ding, L., Wu, Y., Duan, Y., Yu, S., Yu, F., Wang, J., et al. (2020). A novel cell-assisted enhanced chemiluminescence strategy for rapid and label-free detection of tumor cells in whole blood. ACS Sensors 5 (2), 440–446. doi:10.1021/acssensors.9b02140
Duan, X., Zhang, G. Q., Ji, S., Zhang, Y., Li, J., Ou, H., et al. (2022). Activatable persistent luminescence from porphyrin derivatives and supramolecular probes with imaging-modality transformable characteristics for improved biological applications. Angew. Chem. Int. Ed. 61 (24), e202116174. doi:10.1002/anie.202116174
Eilon, S. T., Roth, K. M., Eldar, B. A., Satchi, F. R., and Shabat, D. (2018). Ortho-chlorination of phenoxy 1, 2-dioxetane yields superior chemiluminescent probes for in vitro and in vivo imaging. Org. Biomol. Chem. 16 (10), 1708–1712. doi:10.1039/c8ob00087e
Fan, N., Li, P., Wu, C., Wang, X., Zhou, Y., and Tang, B. (2021). ALP-activated chemiluminescence PDT nano-platform for liver cancer-specific theranostics. ACS Appl. Bio Mater. 4 (2), 1740–1748. doi:10.1021/acsabm.0c01504
Freedland, S. J. (2011). Screening, risk assessment, and the approach to therapy in patients with prostate cancer. Cancer 117 (6), 1123–1135. doi:10.1002/cncr.25477
García, C. A., and Baeyens, W. (2000). Principles and recent analytical applications of chemiluminescence. Analusis 28 (8), 686–698. doi:10.1051/analusis:2000280686
Gnaim, S., Green, O., and Shabat, D. (2018). The emergence of aqueous chemiluminescence: New promising class of phenoxy 1, 2-dioxetane luminophores. Chem. Commun. 54 (17), 2073–2085. doi:10.1039/c8cc00428e
Gnaim, S., and Shabat, D. (2017). Self-immolative chemiluminescence polymers: Innate assimilation of chemiexcitation in a domino-like depolymerization. J. Am. Chem. Soc. 139 (29), 10002–10008. doi:10.1021/jacs.7b04804
Hananya, N., Eldar, B. A., Bauer, C. R., Satchi, F. R., and Shabat, D. (2016). Remarkable enhancement of chemiluminescent signal by dioxetane-fluorophore conjugates: Turn-on chemiluminescence probes with color modulation for sensing and imaging. J. Am. Chem. Soc. 138 (40), 13438–13446. doi:10.1021/jacs.6b09173
Huang, X., Li, L., Qian, H., Dong, C., and Ren, J. (2006). A resonance energy transfer between chemiluminescent donors and luminescent quantum-dots as acceptors (CRET). Angew. Chem. Int. Ed. 45 (31), 5140–5143. doi:10.1002/anie.200601196
Jiang, Y., Huang, J., Zhen, X., Zeng, Z., Li, J., Xie, C., et al. (2019). A generic approach towards afterglow luminescent nanoparticles for ultrasensitive in vivo imaging. Nat. Commun. 10 (1), 2064–2110. doi:10.1038/s41467-019-10119-x
Kaeser, A., and Schenning, A. P. (2010). Fluorescent nanoparticles based on self-assembled π-conjugated systems. Adv. Mater. 22 (28), 2985–2997. doi:10.1002/adma.201000427
Kopecky, K. R., and Mumford, C. (1969). Luminescence in the thermal decomposition of 3, 3, 4-trimethyl-1, 2-dioxetane. Can. J. Chem. 47 (4), 709–711. doi:10.1139/v69-114
Lee, E. S., Deepagan, V., You, D. G., Jeon, J., Yi, G. R., Lee, J. Y., et al. (2016). Nanoparticles based on quantum dots and a luminol derivative: Implications for in vivo imaging of hydrogen peroxide by chemiluminescence resonance energy transfer. Chem. Commun. 52 (22), 4132–4135. doi:10.1039/c5cc09850e
Lee, Y. D., Lim, C. K., Singh, A., Koh, J., Kim, J., Kwon, I. C., et al. (2012). Dye/peroxalate aggregated nanoparticles with enhanced and tunable chemiluminescence for biomedical imaging of hydrogen peroxide. ACS Nano 6 (8), 6759–6766. doi:10.1021/nn3014905
Li, H., Lu, Y., Chung, J., Han, J., Kim, H., Yao, Q., et al. (2021). Activation of apoptosis by rationally constructing NIR amphiphilic AIEgens: Surmounting the shackle of mitochondrial membrane potential for amplified tumor ablation. Chem. Sci. 12 (31), 10522–10531. doi:10.1039/d1sc02227j
Li, J., and Pu, K. (2019). Development of organic semiconducting materials for deep-tissue optical imaging, phototherapy and photoactivation. Chem. Soc. Rev. 48 (1), 38–71. doi:10.1039/c8cs00001h
Li, Z., Lin, H., Wang, L., Cao, L., Sui, J., and Wang, K. (2022). Optical sensing techniques for rapid detection of agrochemicals: Strategies, challenges, and perspectives. Sci. Total Environ. 838, 156515. doi:10.1016/j.scitotenv.2022.156515
Li, Z., Zhu, B., Duan, X., and Tang, W. (2019). The imaging of local glucose levels in tumor periphery via peroxyoxalate chemiluminescent nanoparticle-glucose oxidase-doped alginate hydrogel. Anal. Methods 11 (21), 2763–2768. doi:10.1039/c9ay00625g
Liu, X., Wang, Q., Chen, J., Chen, X., and Yang, W. (2021). Ultrasensitive electrochemiluminescence biosensor for the detection of tumor exosomes based on peptide recognition and luminol-AuNPs@g-C3N4 nanoprobe signal amplification. Talanta 221, 121379. doi:10.1016/j.talanta.2020.121379
Lu, C., Zhang, C., Wang, P., Zhao, Y., Yang, Y., Wang, Y., et al. (2020). Light-free generation of singlet oxygen through manganese-thiophene nanosystems for pH-responsive chemiluminescence imaging and tumor therapy. Chem 6 (9), 2314–2334. doi:10.1016/j.chempr.2020.06.024
Mao, D., Wu, W., Ji, S., Chen, C., Hu, F., Kong, D., et al. (2017). Chemiluminescence-guided cancer therapy using a chemiexcited photosensitizer. Chem 3 (6), 991–1007. doi:10.1016/j.chempr.2017.10.002
Mao, Q., Fang, J., Wang, A., Zhang, Y., Cui, C., Ye, S., et al. (2021). Aggregation of gold nanoparticles triggered by hydrogen peroxide-initiated chemiluminescence for activated tumor theranostics. Angew. Chem. 133 (44), 23998–24004. doi:10.1002/ange.202109863
Matsumoto, M. (2004). Advanced Chemistry of dioxetane-based chemiluminescent substrates originating from bioluminescence. J. Photochem. Photobiol. C Photochem. Rev. 5 (1), 27–53. doi:10.1016/j.jphotochemrev.2004.02.001
Miao, Q., and Pu, K. (2018). Organic semiconducting agents for deep-tissue molecular imaging: Second near-infrared fluorescence, self-luminescence, and photoacoustics. Adv. Mater. 30 (49), 1801778. doi:10.1002/adma.201801778
Miao, Q., Xie, C., Zhen, X., Lyu, Y., Duan, H., Liu, X., et al. (2017). Molecular afterglow imaging with bright, biodegradable polymer nanoparticles. Nat. Biotechnol. 35 (11), 1102–1110. doi:10.1038/nbt.3987
Nan, Y., Zhao, W., Li, N., Liang, Z., and Xu, X. (2019). Chemiluminescence-triggered fluorophore release: Approach for in vivo fluorescence imaging of hydrogen peroxide. Sensors Actuators B Chem. 281, 296–302. doi:10.1016/j.snb.2018.10.129
Ni, X., Zhang, X., Duan, X., Zheng, H. L., Xue, X. S., and Ding, D. (2018). Near-infrared afterglow luminescent aggregation-induced emission dots with ultrahigh tumor-to-liver signal ratio for promoted image-guided cancer surgery. Nano Lett. 19 (1), 318–330. doi:10.1021/acs.nanolett.8b03936
Romanyuk, A. V., Grozdova, I. D., Ezhov, A. A., and Melik-Nubarov, N. S. (2017). Peroxyoxalate chemiluminescent reaction as a tool for elimination of tumour cells under oxidative stress. Sci. Rep. 7 (1), 3410–3413. doi:10.1038/s41598-017-03527-w
Sambi, M., Bagheri, L., and Szewczuk, M. R. (2019). Current challenges in cancer immunotherapy: Multimodal approaches to improve efficacy and patient response rates. J. Oncol. 2019, 1–12. doi:10.1155/2019/4508794
Schaap, A., Akhavan, H., and Romano, L. (1989). Chemiluminescent substrates for alkaline phosphatase: Application to ultrasensitive enzyme-linked immunoassays and DNA probes. Clin. Chem. 35 (9), 1863–1864. doi:10.1093/clinchem/35.9.1863
Schaap, A. P., and Gagnon, S. D. (1982). Chemiluminescence from a phenoxide-substituted 1, 2-dioxetane: A model for firefly bioluminescence. J. Am. Chem. Soc. 104 (12), 3504–3506. doi:10.1021/ja00376a044
Schaap, A. P., Handley, R. S., and Giri, B. P. (1987). Chemical and enzymatic triggering of 1, 2-dioxetanes. 1: Aryl esterase-catalyzed chemiluminescence from a naphthyl acetate-substituted dioxetane. Tetrahedron Lett. 28 (9), 935–938. doi:10.1016/s0040-4039(00)95878-7
Shuhendler, A. J., Pu, K., Cui, L., Uetrecht, J. P., and Rao, J. (2014). Real-time imaging of oxidative and nitrosative stress in the liver of live animals for drug-toxicity testing. Nat. Biotechnol. 32 (4), 373–380. doi:10.1038/nbt.2838
Su, Y., Song, H., and Lv, Y. (2019). Recent advances in chemiluminescence for reactive oxygen species sensing and imaging analysis. Microchem. J. 146, 83–97. doi:10.1016/j.microc.2018.12.056
Thomas, J. A. (2015). Optical imaging probes for biomolecules: An introductory perspective. Chem. Soc. Rev. 44 (14), 4494–4500. doi:10.1039/c5cs00070j
Tiwari, A., and Dhoble, S. (2018). Recent advances and developments on integrating nanotechnology with chemiluminescence assays. Talanta 180, 1–11. doi:10.1016/j.talanta.2017.12.031
Trachootham, D., Alexandre, J., and Huang, P. (2009). Targeting cancer cells by ROS-mediated mechanisms: A radical therapeutic approach? Nat. Rev. Drug Discov. 8 (7), 579–591. doi:10.1038/nrd2803
Tzani, M. A., Gioftsidou, D. K., Kallitsakis, M. G., Pliatsios, N. V., Kalogiouri, N. P., Angaridis, P. A., et al. (2021). Direct and indirect chemiluminescence: Reactions, mechanisms and challenges. Molecules 26 (24), 7664. doi:10.3390/molecules26247664
Vacher, M., Fdez, G. I., Ding, B. W., Schramm, S., Berraud, P. R., Naumov, P., et al. (2018). Chemi-and bioluminescence of cyclic peroxides. Chem. Rev. 118 (15), 6927–6974. doi:10.1021/acs.chemrev.7b00649
Wang, Y., Shi, L., Ye, Z., Guan, K., Teng, L., Wu, J., et al. (2019). Reactive oxygen correlated chemiluminescent imaging of a semiconducting polymer nanoplatform for monitoring chemodynamic therapy. Nano Lett. 20 (1), 176–183. doi:10.1021/acs.nanolett.9b03556
Wu, M., Wu, L., Li, J., Zhang, D., Lan, S., Zhang, X., et al. (2019). Self-luminescing theranostic nanoreactors with intraparticle relayed energy transfer for tumor microenvironment activated imaging and photodynamic therapy. Theranostics 9 (1), 20–33. doi:10.7150/thno.28857
Yan, X., Zhao, K., Yang, Y., Qiu, A., Zhang, X., Liu, J., et al. (2022). Utilizing dual carriers assisted by enzyme digestion chemiluminescence signal enhancement strategy simultaneously detect tumor markers CEA and AFP. Anal. Sci. 38, 889–897. doi:10.1007/s44211-022-00109-3
Yan, Y., Shi, P., Song, W., and Bi, S. (2019). Chemiluminescence and bioluminescence imaging for biosensing and therapy: In vitro and in vivo perspectives. Theranostics 9 (14), 4047–4065. doi:10.7150/thno.33228
Yang, B., Chen, Y., and Shi, J. (2019). Reactive oxygen species (ROS)-Based nanomedicine. Chem. Rev. 119 (8), 4881–4985. doi:10.1021/acs.chemrev.8b00626
Yang, L., Zhang, R., Liu, B., Wang, J., Wang, S., Han, M. Y., et al. (2014). π-conjugated carbon radicals at graphene oxide to initiate ultrastrong chemiluminescence. Angew. Chem. 126 (38), 10273–10277. doi:10.1002/ange.201405295
Yang, Y., Wang, S., Lu, L., Zhang, Q., Yu, P., Fan, Y., et al. (2020). NIR-II chemiluminescence molecular sensor for in vivo high-contrast inflammation imaging. Angew. Chem. 132 (42), 18538–18543. doi:10.1002/ange.202007649
Yang, Y., and Zhang, F. (2021). Activatable chemiluminescent molecular probes for bioimaging and biosensing. Analysis Sens. 1 (2), 75–89. doi:10.1002/anse.202000033
Yu, Y., Xie, B. R., Liu, X. H., Ye, J. J., Cheng, H., Zhong, Z., et al. (2022). A H2O2-responsive theranostic platform for chemiluminescence detection and synergistic therapy of tumors. J. Mater. Chem. B 10 (10), 1634–1640. doi:10.1039/d2tb00015f
Yuan, H., Chong, H., Wang, B., Zhu, C., Liu, L., Yang, Q., et al. (2012). Chemical molecule-induced light-activated system for anticancer and antifungal activities. J. Am. Chem. Soc. 134 (32), 13184–13187. doi:10.1021/ja304986t
Zhang, N., Francis, K. P., Prakash, A., and Ansaldi, D. (2013). Enhanced detection of myeloperoxidase activity in deep tissues through luminescent excitation of near-infrared nanoparticles. Nat. Med. 19 (4), 500–505. doi:10.1038/nm.3110
Zhang, Y., Sun, G., Yang, H., Yu, J., Yan, M., and Song, X. (2016). Multifunctional reduced graphene oxide trigged chemiluminescence resonance energy transfer: Novel signal amplification strategy for photoelectrochemical immunoassay of squamous cell carcinoma antigen. Biosens. Bioelectron. 79, 55–62. doi:10.1016/j.bios.2015.12.008
Keywords: chemiluminescence, tumor diagnosis, luminol, peroxyoxalates, 1,2-dioxetanes
Citation: Zhang X, Li C, Chen W, Wang G, Zou H and Liu H (2023) Chemiluminescent polymeric nanoprobes for tumor diagnosis: A mini review. Front. Chem. 10:1106791. doi: 10.3389/fchem.2022.1106791
Received: 24 November 2022; Accepted: 28 December 2022;
Published: 09 January 2023.
Edited by:
Bo Shen, Northwestern University, United StatesReviewed by:
Yuerou Zhang, Brown University, United StatesJiahong Shen, Northwestern University, United States
Jing Cao, Hunan Institute of Science and Technology, China
Copyright © 2023 Zhang, Li, Chen, Wang, Zou and Liu. This is an open-access article distributed under the terms of the Creative Commons Attribution License (CC BY). The use, distribution or reproduction in other forums is permitted, provided the original author(s) and the copyright owner(s) are credited and that the original publication in this journal is cited, in accordance with accepted academic practice. No use, distribution or reproduction is permitted which does not comply with these terms.
*Correspondence: Xiaoyan Zhang, eGlhb3lhbnpoYW5nMTk5MkAxMjYuY29t; Hao Liu, a3FsaDIwMTNAMTYzLmNvbQ==