- 1Department of Process Engineering, Faculty of Technology, University of El Oued, El-Oued, Algeria
- 2Laboratory of Biotechnology Biomaterial and Condensed Matter, Faculty of Technology, University of El Oued, El-Oued, Algeria
- 3Department of Chemistry, Faculty of Science, University of Tabuk, Tabuk, Saudi Arabia
- 4Department of Chemistry, Jerash University, Jerash, Jordan
- 5Department of Civil Engineering, Isra University, Amman, Jordan
- 6Center of Excellence for Advanced Materials Research (CEAMR) and Chemistry Department, Faculty of Science, King Abdulaziz University, Jeddah, Saudi Arabia
- 7Department of Physics, College of Science, Jazan University, Jazan, Saudi Arabia
- 8Department of Biomedical and Environmental Engineering (BEE), Fluorotronics, Inc.-California Innovations Corporation, San Diego, CA, United States
Introduction: In this paper, MgO@AgO/Ag2O nanoparticles were greenly synthesized, the current idea is to replace the harmful chemical technique with an ecofriendly synthesis of metal oxide nanoparticles (NPs) utilizing biogenic sources.
Methods: The current investigation was conducted to create silver oxide NPs decorated by MgO NPs (namely, MgO@AgO/Ag2O nanocom-posite) using the leaves extract of Purslane (Portulaca Oleracea) as the reducing and capping agent. The nanopowder was investigated by means of X-ray diffraction, scanning electron mi-croscope, BET surface area, Fourier transform infrared, and UV-vis spectrophotom-eter studies. XRD studies reveal the monophasic nature of these highly crystalline silver nano-particles. SEM studies the shape and morphology of the synthesis AgO/Ag2O and MgO@AgO/Ag2O NPs. The presence of magnesium and oxygen was further confirmed by EDS profile.
Results and discussion: The surface area was found to be 9.1787 m2/g and 7.7166 m2/g, respectively. FTIR analysis showed the presence of specific functional groups. UV-vis spectrophotometer studies show the absorption band at 450 nm due to surface plasmon resonance. The results have also indicated the high performance of the greenly synthesized AgO/Ag2O NPs and MgO@AgO/Ag2O NPs for photocatalytic activity dye degradation (methylene blue and toluidine blue).
1 Introduction
With widespread applications in the domains of biology, health, energy, and material science, among others, nanotechnology has emerged as a promising interdisciplinary field of the twenty-first century. Nanomaterials are produced often by a variety of physical and chemical processes that call for high temperatures, vacuum conditions, specialized equipment, and chemical additives (Cai et al., 2022). Due to the use of harmful compounds that remain attached to the synthesized nanoparticles, current improvements in chemical processes for the production of nanomaterials have raised biological dangers to the environment (Yu et al., 2022). Consequently, scientists are presently concentrating on the production of nanomaterials utilizing biogenic sources such as bacteria, algae, and plants. A new age for secure nanobiotechnology has begun because of recent advancements in the green synthesis of nanomaterials, which are rapid, inexpensive, and ecofriendly. Due to their unique physical and chemical features in the domains of biosensors, diagnostic tools, catalysts, anticancer, and antimicrobial agents, metal oxide nanoparticles have recently attracted a lot of attention (Zhang et al., 2020; Ahmad et al., 2021; Wijesinghe et al., 2021a; Wijesinghe et al., 2021b; Gherbi et al., 2022).
In part because of their non-biodegradable and persistent character, the considerable water pollution caused by inorganic (such as heavy metal ions) and organic compounds—such as synthetic organic colors, persistent organic pollutants, antibiotics, etc.—often present significant obstacles. Additionally, these emerging pollutants may have several detrimental effects on human bodies and aquatic microbes (Sharma and Bhattacharya, 2017; Al-Essa and Al-Essa, 2021). The world’s use of synthetic dyes now exceeds several million tons, with numerous industrial sectors including the printing and textile industries contributing to this (Mashkoor and Nasar, 2020). Therefore, solutions for wastewater pretreatment are required to reduce these contamination issues. Scientifically, the adsorption process is the most effective and practical method for purifying water is adsorption (Al-Essa, 2018; Bulgariu et al., 2019). Adsorbents should be created from free, sustainable, and locally accessible sources to achieve competitive efficacy (typically, plant resources) (Bulgariu et al., 2019).
Due to their high conductivity, silver oxide nanoparticles (AgONPs) stand out among the competition as a desirable material for metallic-based conductive filler (Stewart et al., 2017), capacity to encourage cell proliferation and osteogenic differentiation facilitate bone repair (Zhang et al., 2015; Xu et al., 2020), in addition to their capacity to prevent the development of microbial biofilm (Hileuskaya et al., 2020) and maybe defeat microorganisms that are resistant to many drugs (Rai et al., 2012). AgONPs were highly sought-after for application in biosensors due to these special characteristics (Zeng et al., 2014; Al Essa et al., 2021), prostheses (Gallo et al., 2016), and dental materials (Ohashi et al., 2004). The resultant AgONPs, however, are inappropriate for biomedicine use due to the common synthesis technique, which frequently employs toxic chemical reducing agents that are dangerous for human (such as sodium borohydride and hydrazine) (Kolarova et al., 2015; Rónavári et al., 2021). Magnesium oxide nanoparticles (MgONPs) are harmless and reasonably simple to synthesize among the many distinct inorganic metal oxides. MgONPs have received approval from the United States Food and Drug Administration as safe materials (21CFR184.1431) (Martinez-Boubeta et al., 2010). MgONPs, for instance, can reduce indigestion, start the post-activation of bone-repair scaffolds, and function as hyperthermia agents in cancer treatment. Both MgO and AgO NPs can be now produced using “green synthesis” techniques, which offer straightforward, affordable, quick, and non-toxic approaches to address these problems. The use of diverse biological entities, such as plant extract, in green synthesis techniques replaces the use of chemical reducing agents. (Ahmadi et al., 2018; Hamouda et al., 2019; Okaiyeto et al., 2019), and bacteria (Hamouda et al., 2019) produce a high output of somewhat uniform-sized metallic nanoparticles by acting as both reducing and capping agents. Due to their accessibility, safety, and simplicity of synthesis, plant-based extracts have drawn more attention. They don’t require the preservation of microbial cultures (Roy and Das, 2015). The most often reported use so far has been of various plant fruit and leaf extracts. Purslane is a fascinating possibility for this use among many different plants (Bouafia et al., 2020; Bouafia et al., 2021; Bouafia and Laouini, 2021).
The decor is another method for enhancing photocatalytic activity (Wang et al., 2011; Hunge et al., 2021; Yadav et al., 2021). Surface plasmon resonance (SPR), where Ag, Au, and Pt are typically utilized, is favored by decoration with noble metals (Xing et al., 2017), it has many positive benefits since it promotes the absorption of visible light and the activation of energetic charge carriers, many (Sohrabnezhad and Seifi, 2016), achieves a greater separation of charge, this leads to an increase in redox reactions and a higher formation rate of reactive oxygen species (Ong et al., 2013; Kuriakose et al., 2014; Sohrabnezhad and Seifi, 2016). Previous studies have confirmed the photocatalytic activity of AgO and Mg NPs (Balakrishnan et al., 2020). Moreover, prior studies demonstrated that the decoration of AgONPs with other metals NPs results in a significant improvement in the photocatalytic activity comparing to the nondecorated AgONPs (Laouini et al., 2021).
In this study, the degradation of the methylene blue (MB) and toluidine blue (TB) dyes under sunlight was used to examine the photocatalytic capabilities of AgO/Ag2O nanoparticles (NPs) coated with MgO. To the best of our knowledge AgO/Ag2O and MgO@AgO/Ag2O NPs photocatalytic activity was never tested against MB and TB dyes.
2 Materials and methods
2.1 Reagents
Silver nitrate (AgNO3, 98%), Magnesium chloride hydrates (MgCl2, 6H2O, 98%), MB (C16H18ClN3S, 98%), and TB (C15H16ClN3S, 98%) were purchased from Sigma-Aldrich,Germany. The leaves of purslane were collected from local fields in El Oued, Southeast of Algeria. Distilled water was used in all the experiments.
2.2 Preparation of the leaf extract
Fresh and healthy purslane (Portulaca oleracea) leaves were collected from nearby farms in the El Oued area (Southeast of Algeria). After properly cleaning them with flowing tap water to get rid of any dirts or other polluted organic materials, they were repeatedly rinsed in de-mineralized water. The fresh leaves were crushed. The leaves components were extracted by mixing 250.0 g of leaves with 900 ml of distilled water in a 1,000 ml glass beaker. The mixture was stirred for 15 min at 70°C. The extract was kept cool and then filtered using the decantation method and stored at 4°C for further use.
2.3 Synthesis of AgO/Ag2O and MgO@AgO/Ag2O NPs
The AgO/Ag2O and MgO@AgO/Ag2O NPs were synthesized by a green method using plant extract, following modified protocols from previous studies (Abdullah et al., 2020; Bouafia and Laouini, 2020; Bouafia et al., 2021a; Bouafia et al., 2021b; Belaiche et al., 2021; Laid et al., 2021; Laouini et al., 2021; Ben Amor et al., 2022; Daoudi et al., 2022; Djamila et al., 2022; Meneceur et al., 2022; Tedjani et al., 2022). For the synthesis of AgO/Ag2O NPs filtered extract was taken and diluted to 1,000 ml with deionized water, 1.7 g of AgNO3 was added to 1,000 ml of the extract with continuous stirring, 5 ml diluted hydroxide (0.1 M) was added dropwise to the mixture solution. With controlled and continuous stirring (600 rpm) at 70°C for 4 h, the precursor became brown. The precipitate was then centrifuged and washed several times with deionized water, and dried at 100°C in an oven for 48 h. Finally, the AgO/Ag2O NPs were manually grinded into a fine powder.
Nanostructured MgO loaded AgO/Ag2O were simply synthesized. Firstly, nanoparticle solution (AgO/Ag2O) was prepared by dissolving 0.7 g of (AgO/Ag2O) in de-ionized water, the solution maintained in continuous stirring at 70°C for 2 h. Then 70 ml (0.01 M) metal ion (MgCl2) solution was added to the nanoparticle solution, mixed thoroughly at 80°C for 2 h, and then dried at 100°C for 48 h.
After drying, the mixture was grinded to obtain a homogeneous powder.
2.4 Material characterizations
The employment of the following methods was necessary for the characterization of generated nanoparticles: X-ray diffractometer (XRD), a scanning electron microscope (SEM), EDS, BET surface area analyzer, Fourier transform infrared (FTIR), and UV-vis spectrophotometer.
Using XRD (Rigaka Miniflex 600) and Cu-K radiation with a wavelength of 0.15406 nm in the 2 range 10–80, the crystalline structure of the produced NPs was determined. The morphology of the prepared AgO/Ag2O and MgO@AgO/Ag2O NPs were verified using SEM (TESCAN VEGA 3) with an accelerating voltage of 10 kV. The BET surface area analyzer (micromeritics ASAP 2020 Plus Version 2.00) was used to capture the N2 adsorption isotherm, associated surface area, and pore parameter. A Nicolet iS5 (Thermo Fisher Scientific) was used to perform FTIR measurements on leaves extract, green synthesis AgO/Ag2O, and MgO@AgO/Ag2O NPs to determine the functional groups operating in the region of 4,000 to 400 cm−1. A UV-vis spectrophotometer was used to examine the optical properties of the samples (Shimadzu-1800). The measurement was made at the temperature of 28°C in the 300–900 nm wavelength range. UV-vis spectrometric measurements were performed using a quartz cell and distilled water as a blank solution to determine the stability of AgO/Ag2O NPs. The optical gap band energy (Egap) of these materials was calculated using the Tauc Equation considering these findings.
2.5 Photocatalytic degradation of MB dye
Based on the samples’ capacity to break down the MB dye in the presence of sunlight, the catalytic activity of the samples was evaluated. The experiment was conducted in a lab using sunlight (the samples were not exposed to direct sunlight). MB stock solution (2.5 × 10−5 M) was made. Four samples of 5 ml of dye solution and 5.0 mg of AgO/Ag2O NPs were combined and exposed to sunlight in. About 2 ml of the suspensions were taken from the reaction mixture every 15, 30, 60, and 120 min while it was exposed to sunlight, and the suspended particles were then extracted using ultracentrifugation. The same test was conducted using MgO@AgO/Ag2O. The absorption obtained at 664 nm in a UV-vis spectrophotometer was used to calculate the rate of dye degradation. Based on the formula, the dye degradation % was evaluated using the Eq. 1:
where
2.6 Photocatalytic degradation of TB dye
The photodegradation of TB dye in aqueous solution was investigated under sunlight in the presence of AgO/Ag2ONPs and MgO@AgO/Ag2O as a photocatalyst, A5.0 mg of the catalyst is added to 50 ml of TB dye solution. This solution is prepared in distilled water at a concentration of (4 × 10−3 M). It was then exposed to sunlight at different intervals (10, 20, 30, 40, and 60 min) at a temperature of 28°C and a neutral pH. The catalyst is separated by ultracentrifugation. A spectrophotometer is used for reading the absorbance values at 631 nm.
The equilibrium amount of adsorption is calculated by the Eq. 2:
where, QE (mg/g) is the adsorption capacity at equilibrium,
3 Results and discussion
3.1 Crystal structure and composition
Portulaca oleracea’s medicinal potency is attributed to its complex chemical makeup composition; it is high in primary and secondary metabolites, as well as minerals, vitamins, and other micronutrients.
Purslane is the common name for Portulaca oleracea L., a member of the Portulaceae family. A variety of substances have been identified from Portulaca oleracea, including flavonoids, alkaloids, polysaccharides, fatty acids, terpenoids, sterols, proteins, vitamins, and minerals. There is a wide range of pharmacological qualities that Portulaca oleracea exhibits, including neuroprotective, antibacterial, antidiabetic, antioxidant, anti-inflammatory, antiulcerogenic, and anticancer actions. (Zhou et al., 2015)
To investigate the size and make-up of the crystalline phases, XRD analysis was done.
Figure 1 shows the diffraction patterns of AgO/Ag2O and MgO@AgO/Ag2O. Similar results have already been described in the literature (Deekshitha and Shetty, 2021), regarding the peaks of Ag2O. Moreover, It is intriguing to see that there are two distinct peaks that can be attributed to MgO NPs. According to the Debye-Scherrer equation, the crystallite size was determined to be 22.16 nm for AgO/Ag2O and 21.60 nm for MgO@AgO/Ag2O NPs, respectively. The results are shown below in Table 1.
3.2 Morphological investigation
SEM was used to study the formation of the prepared AgO/Ag2O and MgO@AgO/Ag2O NPs (Figures 2A,C, respectively), and their morphological size (40–50 nm in average) (Figures 2B, D, respectively). The MgO@AgO/Ag2O NPs were oval and spherical. Most of the MgO@AgO/Ag2O NPs were placed as aggregated which may explain the slight increase in size of these NPs compared to AgO/Ag2O NPs; also, a few individual particles were also observed (Gosens et al., 2010).
Further analysis by EDS of the prepared AgO/Ag2O and MgO@AgO/Ag2O NPs (Figures 3A,B, respectively). The data associated to MgO@AgO/Ag2O NPs, confirm the presence of silver, oxygen, and magnesium, with a weight percentage of approximately 87.47% Ag, 12.18% O and 0.35% Mg. It is concluded that all nanoparticles were mixed together. In this way, the aimed ternary oxides nanoparticle system containing MgO, AgO, and Ag2O NPs was obtained.
The MgO@AgO/Ag2O NPs sample’s EDAX analysis revealed only clear peaks for the elements Ag, Mg, and O; no additional peaks could be observed, proving that the powder was produced without any impurities.
3.3 N2 adsorption–desorption isotherm
Figure 4 shows the N2 adsorption-desorption isotherm of 1) AgO/Ag2O and 2) MgO@AgO/Ag2O NPs. The specific BET surface area was found to be 9.1787 m2/g and 7.7166 m2/g (Table 2). The average pore diameter is 3.1 nm, which demonstrates the existence of mesopores. The pore size between 2.89018 and 2.83265 nm shows that the AgO/Ag2O and MgO@AgO/Ag2O NPs, belong to mesoporous materials (2–50 nm), this result can be easily verified by SEM images (Figure 4). The noticeable improvement, in the specific surface area value, proves the enormous photocatalytic activity of the MgO@AgO/Ag2O NPs.
The figure below presented (Figure 4) depicts the nitrogen adsorption isotherms on the surface of the AgO/Ag2O and MgO@AgO/Ag2O NPs. The figures clearly show that:
- All compounds of these nanoparticles belong to mesoporous materials.
- The compound MgO@AgO/Ag2O NPs presents the best catalytic behavior with concerning the adsorption of N2.
3.4 FTIR study
As a result of the aliphatic C-H stretching vibration of hydrocarbon chains and N-H bending vibration, the spectral peaks at 2,924, 2,851, and 1,454 cm1- (Ananthi et al., 2018; Hamouda et al., 2019) are shown in Figure 5.
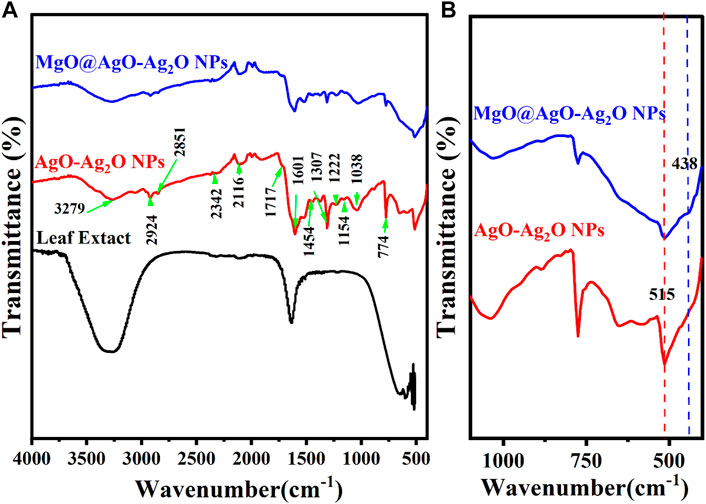
FIGURE 5. (A) FTIR spectra of Purslane (Portulaca Oleracea) leaf extract, AgO/Ag2O, and MgO@AgO/Ag2O; (B) zoomed view range (400 to 1,100 cm−1).
Peaks centered at 1,340, 1,224, 1,142, and 1,024, and cm−1 in the range 1,300–1,000 cm−1 are caused by stretching vibrations of C-O groups in anhydrides, esters, ethers, alcohols, and phenols, C-O-H groups in alcohols and phenols, and C-N groups in amines. The vibration of the C=C group of alkenes, the N-H groups of amides, & the amine salts is represented because of the stretch that was seen at 1,603 cm−1. It is possible that the broad stretch in the 3,400–2,400 cm−1 range, which is centered at 3,283 cm−1, is caused by the stretching vibrations of amines, amine salts, sulfonamides, alkenes and alkanes, amines’ N-H and C-H, and carboxylic acids’ C=O. Nitriles, aromatic rings, and aldehydes may all be appeared as other weak bands on the graph Thus, the results of the FTIR investigation show that the main contributing factors to the reduction of Ag+ ions to Ag0 nanoparticles in the floral extract were the -C=O (carboxyl), -OH (hydroxyl), and N-H (amine) groups (Chekuri et al., 2015). The interaction of these functional groups with the AgONPs may be responsible for the shifting of these peaks (Hamouda et al., 2019).
The FTIR spectra show that the Mg-O stretching frequency of MgO corresponds to the IR peak at 436 cm−1 (Sahoo et al., 2020).
Additionally, the disappearance of the phenolic compound-associated absorbance bands 3,278, 2,924, 2,851, and 1,454 cm−1 after the synthesis of MgO@AgO/Ag2O NPs leads us to conclude that the Portulaca oleracea L. leaf extract contains phytochemicals like alcohols, aldehydes, alkanes, epoxy groups, and ether groups that may be responsible for the nucleation process to reduce precursor from M+ to M0.
3.5 Optical properties
The formation of AgONPs was achieved within 90 min after adding 1.7 g of AgNO3 to the stirred and heated extract. The process did not require the addition of external additives. In addition, bioactive molecules in leaf extracts interact with silver ions (Ag+) and fuse neighboring small particles into nanoparticles, leading to nucleation of (Ag◦) atoms and promoting the nanoparticle growth (Sangar et al., 2019). The formation of AgONPs was confirmed visually by the color change of the reaction mixture from pale green to dark brown. UV-vis spectral scans are commonly implemented to confirm the formation of metal nanoparticles in aqueprobleous solution. Similarly, the product obtained exhibited a surface plasmon resonance (SPR) peak at 240 nm, as a characteristic of AgONPs (Aisida et al., 2019) (Figure 6A). Purslane extract exhibited a peak at approximately 240 nm attributed to the properties of bioactive reducing agent molecules.
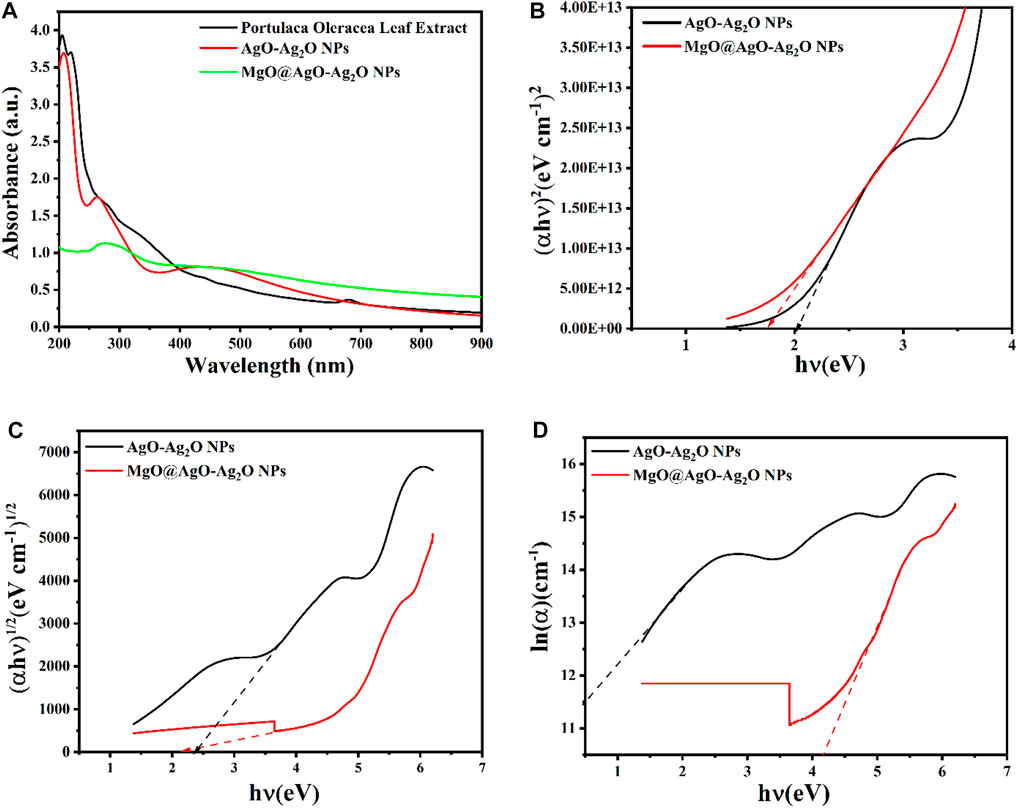
FIGURE 6. UV-vis spectra of (A) Extract, AgO/Ag2O, and MgO@AgO/Ag2O (B) Optical energy gap for direct, (C) Indirect transitions, and (D) Urbach energy.
To further improve the properties, we synthesized hybrid nanostructures MgO@AgO/Ag2O containing 10 mM MgCl2. Magnesium incorporation into the AgO/Ag2O NPs solution was confirmed by UV-vis spectral analysis. However, the reduced SPR peak intensity is related to confirmation of MgO capping on the AgNP surface (Sathiyaseelan et al., 2020).
The optical band gaps of the biosynthesized NPs were estimated using the absorption spectra of both direct and indirect transitions. The Tauc’s equation (Eq. 3) was used to obtain the optical bandgap (Eg) of AgO/Ag2O and MgO@AgO/Ag2O NPs (Soltan et al., 2017; Lassoued et al., 2018).
According to Lambert–Beer–Bouguer Law, the absorption coefficient (Eq. 4) is given as (Kilic et al., 2019):
here, a represents the absorbance and t is the of quartz cuvette width (10 mm).
For the direct transition Tauc plots were plotted using
The indirect optical bandgap values of the biosynthesized AgO/Ag2O and MgO@AgO/Ag2O NPs varied from 2.35 to 2.17 eV (Figure 6C), while the direct bandgap values varied from 2.02 to 1.75 eV (Figure 6B). The direct optical bandgap of the synthesized sample increased with decoration, while the indirect optical bandgap decreased. Higher bandgap values may indicate the presence of confinement effects in the manufactured product. Such high bandgap values of prepared nanostructures make them suitable for electro-optical devices (Shakir et al., 2009; Shkir et al., 2018).
The Urbach energies (Eq. 5) can be estimated exploiting the slope of the absorption edge in the semi-logarithmic plot (Figure 6D).
here,
During our investigation, we observed changes in the Urbach energy of the biosynthesized NPs. These changes occurred due to the influence of MgO. Where an increase in the Urbach energy (from 0.379 to 0.666 eV) was observed upon MgO incorporation, indicating a distortion of the structural order. The Urbach energy characterizes the homogeneity and stability of NPs. The lower the Urbach energy, the more uniform and stable the nanoparticles, and vice versa. The increasing AgO addition to the glass structure explains that the structure has become disordered and unstable. This result is consistent with the literature (Table 3) (Işsever et al., 2019).
3.6 Photocatalytic activity of AgO/Ag2O and MgO@AgO/Ag2O for azo dye degradation
The organic MB dye was used to measure the catalytic activity of AgO/Ag2O and MgO@AgO/Ag2O NPS by measuring the decomposition rate of MB. Selected at 664 nm.
The percentage of MB degradation by AgO/Ag2O and MgO@AgO/Ag2O NPs in the presence of sunlight is shown in (Figures 7B, D). The results showed that the percentage MB decomposition values of synthesized AgO/Ag2O and MgO@AgO/Ag2O NPs changed from 67.71363% to 76.86329% in the presence of sunlight.
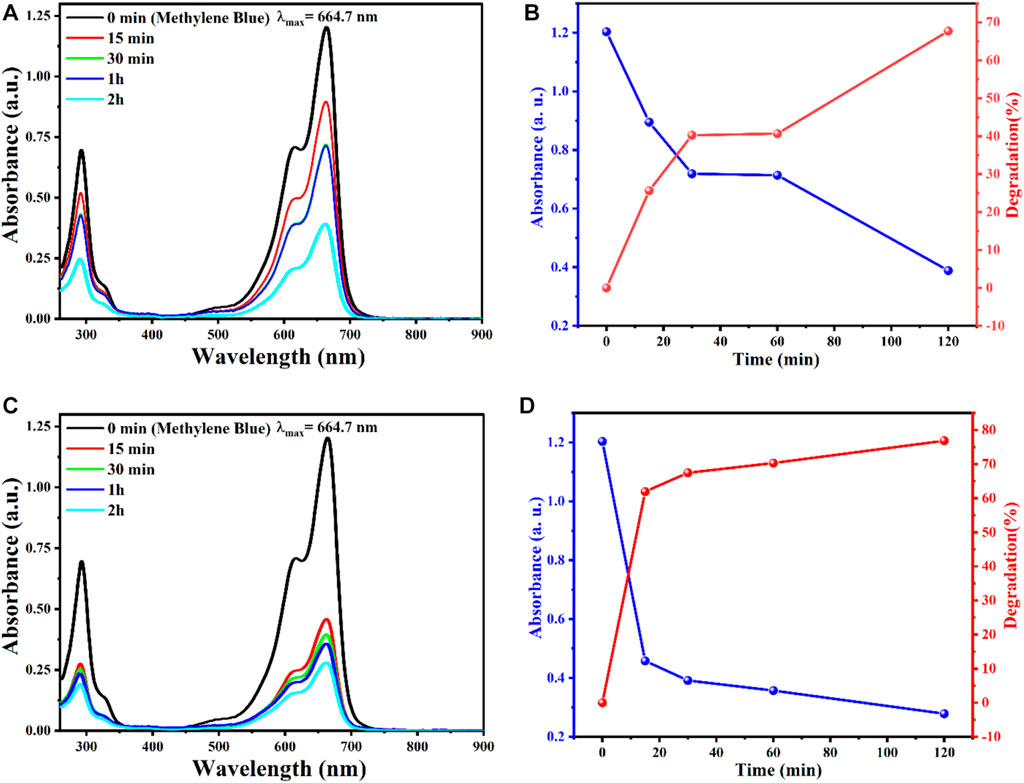
FIGURE 7. The effect reaction time on the degradation of MB, obtained by (A) AgO/Ag2O, Absorbance and degradation versus time: (B) AgO/Ag2O, (C) MgO@AgO/Ag2O; (D) MgO@AgO/Ag2O NPs catalyzed degradation of MB dye.
According to (Figures 7A, C), magnesium decoration had a significant effect on the photocatalytic efficiency of AgO/Ag2O powders. The photocatalytic efficiency of AgO/Ag2O powder was increased by magnesium decoration. As it can been seen, the photocatalytic efficiency was directly proportional to the exposure duration (time).
This result is caused by surface plasmon resonance (SPR), resulting in increased redox reactions and a higher rate of reactive oxygen species generation. Additionally, heterojunctions structured between AgO/Ag2O and MgO@AgO/Ag2O NPs act to increase charge carrier separation, thereby providing highly reactive species that function in photocatalysis (Wang et al., 2011; Ong et al., 2013; Paula et al., 2019).
The effect of photocatalyst decomposition is shown in the Figure 8. Irradiation of NPs affects the degradation of pollutant TB. This effect may be due to the availability of sufficient energy to excite electrons from the valence band to the conduction band. The band gap energy values for AgO/Ag2O and MgO@AgO/Ag2O NPs (2.02 eV to 1.75 eV) justify the reduction of the band gap energy. The reaction mechanism is shown in Figure 9.
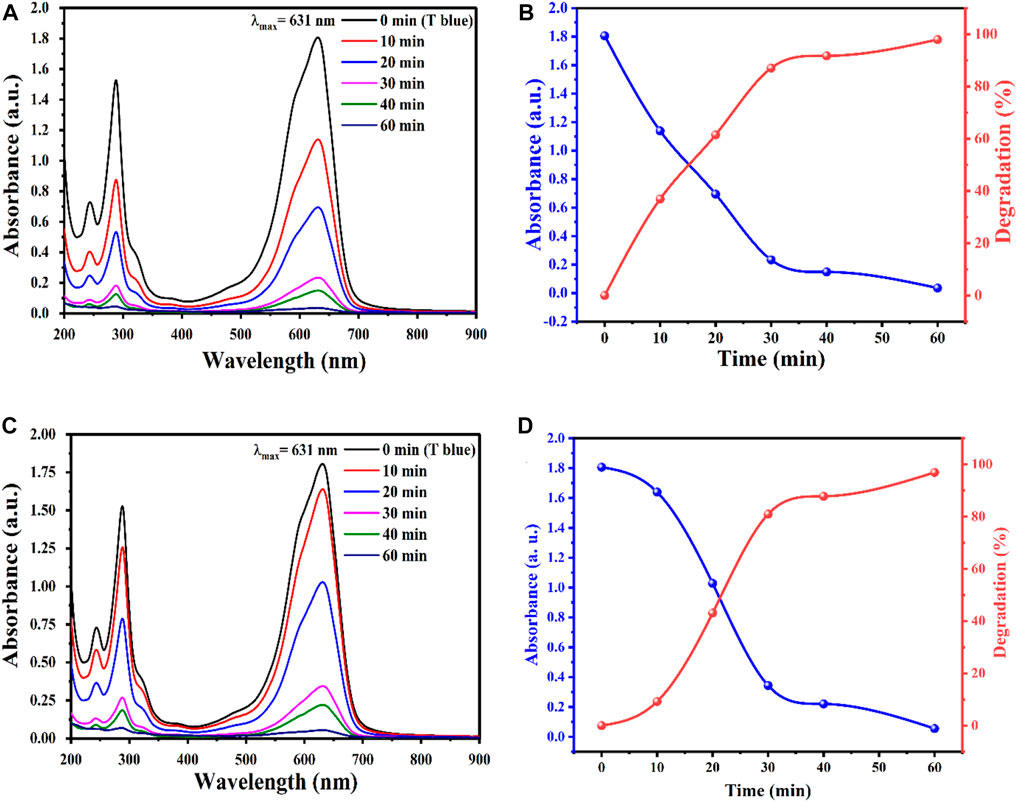
FIGURE 8. The effect of reaction time on degradation of TB, obtained by (A) AgO/Ag2O, Absorbance and degradation versus time: (B) AgO/Ag2O, (C) MgO@AgO/Ag2O; (D) MgO@AgO/Ag2O NPs catalyzed degradation of TB dye.
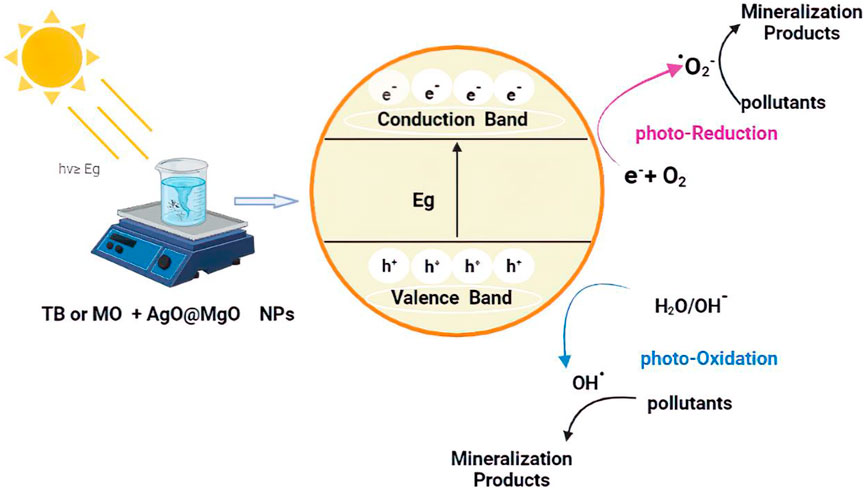
FIGURE 9. MB and TB photocatalytic mineralization mechanism on the surface of AgO/Ag2O and MgO@AgO/Ag2O.
Wastewater purification remains a major environmental issue due to the increasing and widespread spread of non-degradable pollutants in water, Therefore, this study presents the optimization of photocatalytic degradation of TB dye in an aqueous medium by photocatalyst, under sun irradiation. The obtained results revealed that most of the TB were removed within 60 min at a rate of 98%.
Photolysis is a chemical reaction in which a chemical substance is broken down by photons, whereas photocatalysis is defined as the acceleration of a photoreaction in the presence of a catalyst. Surface chemistry peculiar to this family of chemicals influences how electron donors and acceptors interact with metal oxide semiconductors. Light is absorbed by an absorption substrate during catalysed photolysis. For electrons to be promoted from the valence band (VB) to the conduction band (CB), forming electron-hole (e−/h+) pairs, photocatalyst on semiconducting oxides must absorb photons with energy equal to or greater than the oxide’s band gap (Yao et al., 2008; Mittal et al., 2009), as shown in the Eq. 6.
The following steps sum up the mechanism of oxidation for the photodegradation process of the pollutant MB and TB:
Step 1: The radical anion is formed Eq. 6:
Step 2: The OH radical group appears by the hole (7–11);
Step 3: The organic contaminants are oxidized either through direct contact with the holes or by the main radicals and OH· (Eq. 11);
4 Conclusion
The catalytic activity of AgO/Ag2O and MgO@AgO/Ag2O NPs was evaluated based on the rate of degradation of organic dye pollutant. Experimental data illustrated that the percentage of MB degradation values of the synthesized AgO/Ag2O and MgO@AgO/Ag2O NPs changed from 67.71 to 76.86%, and 98% of TB degradation. Furthermore, under environmental circumstances, the synthesized AgO/Ag2O and MgO@AgO/Ag2O NPs display strong photocatalytic activity for dye degradation of MB and TB stains. AgO/Ag2O and MgO@AgO/Ag2O NPs have been found to be useful in the treatment of wastewater (dye degradation). It has proven its ability to purify water from all suspended impurities and remove chemical dyes, and there is a possibility to apply photocatalytic methods to purify water contaminated with hydrocarbons.
Data availability statement
The original contributions presented in the study are included in the article/Supplementary Material, further inquiries can be directed to the corresponding authors.
Author contributions
Conceptualization, writing—original draft preparation: YZ, SL, AB, SM, MT, and FM; methodology, validation, data curation: YZ, SL, AB, SM, MT, SA, HA, KE, EMA-E, MR, OM, and FM; investigation: AB, MT, SM and FM; resources: YZ, AB, AA, KE, EMA-E, MR, and FM; writing—review and editing: AB, SM, MT, SA, HA, KE, EMA-E, MR, and FM; supervision: SL, SM, and FM; Submission: FM. The authors have read and agreed to the published version of the manuscript.
Conflict of interest
Author FM was employed by the company Fluorotronics, Inc.-California Innovations Corporation.
The remaining authors declare that the research was conducted in the absence of any commercial or financial relationships that could be construed as a potential conflict of interest.
Publisher’s note
All claims expressed in this article are solely those of the authors and do not necessarily represent those of their affiliated organizations, or those of the publisher, the editors and the reviewers. Any product that may be evaluated in this article, or claim that may be made by its manufacturer, is not guaranteed or endorsed by the publisher.
Abbreviations
NPs, Nanoparticles; MB, Methylene blue; TB, toluidine blue.
References
Ahmad, M., Rehman, W., Khan, M. M., Qureshi, M. T., Gul, A., Haq, S., et al. (2021). Phytogenic fabrication of ZnO and gold decorated ZnO nanoparticles for photocatalytic degradation of Rhodamine B. J. Environ. Chem. Eng. 9, 104725. doi:10.1016/j.jece.2020.104725
Abdullah, J. A. A., Salah Eddine, L., Abderrhmane, B., Alonso-González, M., Guerrero, A., and Romero, A. (2020). Green synthesis and characterization of iron oxide nanoparticles by pheonix dactylifera leaf extract and evaluation of their antioxidant activity. Sustain. Chem. Pharm. 17, 100280. doi:10.1016/j.scp.2020.100280
Ahmadi, O., Jafarizadeh-Malmiri, H., Jodeiri, N. J. G. P., and Synthesis, (2018). Eco-friendly microwave-enhanced green synthesis of silver nanoparticles using Aloe vera leaf extract and their physico-chemical and antibacterial studies. Green Process. Synthesis 7, 231–240. doi:10.1515/gps-2017-0039
Aisida, S. O., Ugwu, K., Akpa, P. A., Nwanya, A. C., Nwankwo, U., Botha, S. S., et al. (2019). Biosynthesis of silver nanoparticles using bitter leave (Veronica amygdalina) for antibacterial activities. Surfaces Interfaces 17, 100359. doi:10.1016/j.surfin.2019.100359
Al Essa, K., Radha, A., and Navrotsky, A. (2021). “Drop solution calorimetric studies of interface enthalpy of cubic silver (I) oxide (Ag2O) nanocrystals,” in Proceedings of the key engineering materials, 73.
Al-Essa, K. (2018). Activation of Jordanian bentonite by hydrochloric acid and its potential for olive mill wastewater enhanced treatment. J. Chem. 2018, 1–10. doi:10.1155/2018/8385692
Al-Essa, K., and Al-Essa, E. M. (2021). Effective approach of activated Jordanian bentonite by sodium ions for total phenolic compounds removal from olive mill wastewater. J. Chem. 2021, 1–16. doi:10.1155/2021/7405238
Ananthi, V., Prakash, G. S., Rasu, K. M., Gangadevi, K., Boobalan, T., Raja, R., et al. (2018). Comparison of integrated sustainable biodiesel and antibacterial nano silver production by microalgal and yeast isolates. J. Photochem. Photobiol. B Biol. 186, 232–242. doi:10.1016/j.jphotobiol.2018.07.021
Balakrishnan, G., Velavan, R., Mujasam Batoo, K., and Raslan, E. H. (2020). Microstructure, optical and photocatalytic properties of MgO nanoparticles. Results Phys. 16, 103013. doi:10.1016/j.rinp.2020.103013
Belaiche, Y., Khelef, A., Laouini, S. E., Bouafia, A., Tedjani, M. L., and Barhoum, A. (2021). Green synthesis and characterization of silver/silver oxide nanoparticles using aqueous leaves extract of Artemisia herba-alba as reducing and capping agents. Rev. Romana Mater. 51, 342
Ben Amor, M. L., Zeghdi, S., Laouini, S. E., Bouafia, A., and Meneceur, S. (2022). pH reaction effect on biosynthesis of CuO/Cu2O nanoparticles by Moringa oleifera leaves extracts for antioxidant activities. Inorg. Nano-Metal Chem., 1–11. doi:10.1080/24701556.2022.2077376
Bouafia, A., and Laouini, E. S. (2021). Plant-Mediated synthesis of iron oxide nanoparticles and evaluation of the antimicrobial activity: A review. Mini-Reviews Org. Chem. 18, 725–734. doi:10.2174/1570193X17999200908091139
Bouafia, A., Laouini, S. E., and Ouahrani, M. R. (2020). A review on green synthesis of CuO nanoparticles using plant extract and evaluation of antimicrobial activity. Asia. Jour. Rese. Chem. 13, 65–70. doi:10.5958/0974-4150.2020.00014.0
Bouafia, A., Laouini, S. E., Ahmed, A. S. A., Soldatov, A. V., Algarni, H., Feng Chong, K., et al. (2021). The recent progress on silver nanoparticles: Synthesis and electronic applications. Nanomaterials 11, 2318. doi:10.3390/nano11092318
Bouafia, A., and Laouini, S. E. (2020). Green synthesis of iron oxide nanoparticles by aqueous leaves extract of Mentha Pulegium L.: Effect of ferric chloride concentration on the type of product. Mater. Lett. 265, 127364. doi:10.1016/j.matlet.2020.127364
Bouafia, A., Laouini, S. E., Khelef, A., Tedjani, M. L., and Guemari, F. (2021). Effect of ferric chloride concentration on the type of magnetite (Fe3O4) nanoparticles biosynthesized by aqueous leaves extract of artemisia and assessment of their antioxidant activities. J. Clust. Sci. 32, 1033–1041. doi:10.1007/s10876-020-01868-7
Bouafia, A., Laouini, S. E., Tedjani, M. L., Ali, G. A. M., and Barhoum, A. (2021). Green biosynthesis and physicochemical characterization of Fe3O4 nanoparticles using Punica granatum L. fruit peel extract for optoelectronic applications. Text. Res. J. 92, 2685–2696. doi:10.1177/00405175211006671
Bulgariu, L., Escudero, L. B., Bello, O. S., Iqbal, M., Nisar, J., Adegoke, K. A., et al. (2019). The utilization of leaf-based adsorbents for dyes removal: A review. J. Mol. Liq. 276, 728–747. doi:10.1016/j.molliq.2018.12.001
Cai, J., Li, R., Cao, J., Liu, J., Han, J., and Huang, M. (2022). Plasmonic Au-decorated hierarchical p-NiO/n-ZnO heterostructure arrays for enhanced photoelectrochemical water splitting. Phys. E Low-dimensional Syst. Nanostructures 135, 114974. doi:10.1016/j.physe.2021.114974
Chekuri, M., Gangarudraiah, S., Roopavatharam, L. B., Kaphle, A., and Chalimeswamy, A. J. E. C. B. (2015). Green synthesis of stable silver nanoparticles using flower extract of rosa damascena: Characterization, antimicrobial and anti-oxidant activity study, 4, 454
Daoudi, H., Bouafia, A., Meneceur, S., Laouini, S. E., Belkhalfa, H., Lebbihi, R., et al. (2022). Secondary metabolite from nigella sativa seeds mediated synthesis of silver oxide nanoparticles for efficient antioxidant and antibacterial activity. J. Inorg. Organomet. Polym. 32, 4223–4236. doi:10.1007/s10904-022-02393-y
Deekshitha, D., and Shetty, K. V. (2021). Solar light active biogenic titanium dioxide embedded silver oxide (AgO/Ag2O@TiO2) nanocomposite structures for dye degradation by photocatalysis. Mater. Sci. Semicond. Process. 132, 105923. doi:10.1016/j.mssp.2021.105923
Djamila, B., Eddine, L. S., Abderrhmane, B., Nassiba, A., and Barhoum, A. (2022). In vitro antioxidant activities of copper mixed oxide (CuO/Cu2O) nanoparticles produced from the leaves of Phoenix dactylifera L. Biomass Conv. Bioref.. doi:10.1007/s13399-022-02743-3
Freund, F. (1974). “Ceramics and thermal transformations of minerals,” in The Infrared Spectra of Minerals. Editor V. C. Farmer (London: Mineralogical Society of Great Britain and Ireland), 462–485. Vol. 4, 0. Available at: https://www.yumpu.com/fr/document/read/16938251/paleontologie-taphonomie-et-prehistoire-227-p/214.
Gallo, J., Panacek, A., Prucek, R., Kriegova, E., Hradilova, S., Hobza, M., et al. (2016). Silver nanocoating technology in the prevention of prosthetic joint infection. Materials 9, 337. doi:10.3390/ma9050337
Gherbi, B., Laouini, S. E., Meneceur, S., Bouafia, A., Hemmami, H., Tedjani, M. L., et al. (2022). Effect of pH value on the bandgap energy and particles size for biosynthesis of ZnO nanoparticles: Efficiency for photocatalytic adsorption of methyl orange. Sustainability 14, 11300. doi:10.3390/su141811300
Gosens, I., Post, J. A., de la Fonteyne, L. J., Jansen, E. H., Geus, J. W., Cassee, F. R., et al. (2010). Impact of agglomeration state of nano-and submicron sized gold particles on pulmonary inflammation. Part. Fibre Toxicol. 7, 37–11. doi:10.1186/1743-8977-7-37
Hamouda, R. A., Hussein, M. H., Abo-Elmagd, R. A., and Bawazir, S. S. J. S. R. (2019). Synthesis and biological characterization of silver nanoparticles derived from the cyanobacterium Oscillatoria limnetica. Sci. Rep. 9, 13071–13117. doi:10.1038/s41598-019-49444-y
Hileuskaya, K., Ladutska, A., Kulikouskaya, V., Kraskouski, A., Novik, G., Kozerozhets, I., et al. (2020). ‘Green’approach for obtaining stable pectin-capped silver nanoparticles: Physico-chemical characterization and antibacterial activity. Colloids Surfaces A Physicochem. Eng. Aspects 585, 124141. doi:10.1016/j.colsurfa.2019.124141
Hunge, Y. M., Uchida, A., Tominaga, Y., Fujii, Y., Yadav, A. A., Kang, S.-W., et al. (2021). Visible light-assisted photocatalysis using spherical-shaped BiVO4 photocatalyst. Catalysts 11, 460. doi:10.3390/catal11040460
Işsever, U. G., Kilic, G., Peker, M., Ünaldi, T., and Aybek, A. Ş. J. J. o. M. S. M. i. E. (2019). Effect of low ratio V5+ doping on structural and optical properties of borotellurite semiconducting oxide glasses. J. Mat. Sci. Mat. Electron. 30, 15156–15167. doi:10.1007/s10854-019-01889-7
Kilic, G., Issever, U. G., and Ilik, E. J. J. O. M. S. M. I. E. (2019). Characterization of Er3+ doped ZnTeTa semiconducting oxide glass. J. Mat. Sci. Mat. Electron. 30, 8920–8930. doi:10.1007/s10854-019-01220-4
Kolarova, K., Vosmanska, V., Rimpelova, S., Ulbrich, P., and Svorcik, V. J. J. O. N. (2015). Silver nanoparticles stabilized using chitosan films: Preparation, properties and antibacterial activity. J. Nanosci. Nanotechnol. 15, 10120–10126. doi:10.1166/jnn.2015.11697
Kuriakose, S., Choudhary, V., Satpati, B., and Mohapatra, S. J. B. (2014). Enhanced photocatalytic activity of Ag–ZnO hybrid plasmonic nanostructures prepared by a facile wet chemical method. Beilstein J. Nanotechnol. 5, 639–650. doi:10.3762/bjnano.5.75
Laid, T. M., Abdelhamid, K., Eddine, L. S., and Abderrhmane, B. (2021). Optimizing the biosynthesis parameters of iron oxide nanoparticles using central composite design. J. Mol. Struct. 1229, 129497. doi:10.1016/j.molstruc.2020.129497
Laouini, S. E., Bouafia, A., Soldatov, A. V., Algarni, H., Tedjani, M. L., Ali, G. A. M., et al. (2021). Green synthesized of Ag/Ag2O nanoparticles using aqueous leaves extracts of phoenix dactylifera L. And their azo dye photodegradation. Membranes 11, 468. doi:10.3390/membranes11070468
Lassoued, A., Lassoued, M. S., Dkhil, B., Ammar, S., and Gadri, A. J. P. (2018). Synthesis, structural, morphological, optical and magnetic characterization of iron oxide (α-Fe 2 O 3 ) nanoparticles by precipitation method: Effect of varying the nature of precursor. Phys. E Low-dimensional Syst. Nanostructures 97, 328–334. doi:10.1016/j.physe.2017.12.004
Martinez-Boubeta, C., Balcells, L., Cristòfol, R., Sanfeliu, C., Rodríguez, E., Weissleder, R., et al. (2010). Self-assembled multifunctional Fe/MgO nanospheres for magnetic resonance imaging and hyperthermia. Nanomedicine Nanotechnol. Biol. Med. 6, 362–370. doi:10.1016/j.nano.2009.09.003
Mashkoor, F., and Nasar, A. J. J. O. M. (2020). Magsorbents: Potential candidates in wastewater treatment technology – a review on the removal of methylene blue dye. J. Magnetism Magnetic Mater. 500, 166408. doi:10.1016/j.jmmm.2020.166408
Meneceur, S., Hemmami, H., Bouafia, A., Laouini, S. E., Tedjani, M. L., Berra, D., et al. (2022). Photocatalytic activity of iron oxide nanoparticles synthesized by different plant extracts for the degradation of diazo dyes Evans blue and Congo red. Biomass Conv. bioref. doi:10.1007/s13399-022-02734-4
Mittal, N., Shah, A., Punjabi, P. B., and Sharma, V. K. (2009). Photodegradation of rose bengal using MnO2 (manganese dioxide). Rasayan J. Chem. 2, 516–520.
Ohashi, S., Saku, S., and Yamamoto, K. J. J. O. R. (2004). Antibacterial activity of silver inorganic agent YDA filler. J. Oral Rehabil. 31, 364–367. doi:10.1111/j.1365-2842.2004.01200.x
Okaiyeto, K., Ojemaye, M. O., Hoppe, H., Mabinya, L. V., and Okoh, A. I. J. M. (2019). Phytofabrication of silver/silver chloride nanoparticles using aqueous leaf extract of Oedera genistifolia: Characterization and antibacterial potential. Molecules 24, 4382. doi:10.3390/molecules24234382
Ong, W. L., Natarajan, S., Kloostra, B., and Ho, G. W. J. N. (2013). Metal nanoparticle-loaded hierarchically assembled ZnO nanoflakes for enhanced photocatalytic performance. Nanoscale 5, 5568–5575. doi:10.1039/c3nr00043e
Paula, C., Andrade Neto, N., Garcia, L., Nascimento, R., Paskocimas, C., Bomio, M., et al. (2019). Increased degradation capacity of methylene blue dye using Mg-doped ZnO nanoparticles decorated by Ag0 nanoparticles. J. Elec. Materi. 48, 3017–3025. doi:10.1007/s11664-019-07059-z
Rai, M. K., Deshmukh, S., Ingle, A., and Gade, A. J. J. O. A. M. (2012). Silver nanoparticles: The powerful nanoweapon against multidrug-resistant bacteria. J. Appl. Microbiol. 112, 841–852. doi:10.1111/j.1365-2672.2012.05253.x
Rónavári, A., Igaz, N., Adamecz, D. I., Szerencsés, B., Molnar, C., Kónya, Z., et al. (2021). Green silver and gold nanoparticles: Biological synthesis approaches and potentials for biomedical applications. Molecules 26, 844. doi:10.3390/molecules26040844
Roy, S., and Das, T. K. J. I (2015). Plant mediated green synthesis of silver nanoparticles, 3, 1044
Sahoo, S. K., Dhal, J. P., and Panigrahi, G. K. J. C. C. (2020). Magnesium oxide nanoparticles decorated iron oxide nanorods: Synthesis, characterization and remediation of Congo red dye from aqueous media. Compos. Commun. 22, 100496. doi:10.1016/j.coco.2020.100496
Sangar, S., Sharma, S., Vats, V. K., Mehta, S., and Singh, K. J. J. O. C. P. (2019). Biosynthesis of silver nanocrystals, their kinetic profile from nucleation to growth and optical sensing of mercuric ions. J. Clean. Prod. 228, 294–302. doi:10.1016/j.jclepro.2019.04.238
Sathiyaseelan, A., Saravanakumar, K., Mariadoss, A. V. A., and Wang, M.-H. J.(2020). Biocompatible fungal chitosan encapsulated phytogenic silver nanoparticles enhanced antidiabetic, antioxidant and antibacterial activity. Int. J. Biol. Macromol. 153, 63–71. doi:10.1016/j.ijbiomac.2020.02.291
Shakir, M., Kushwaha, S., Maurya, K., Bhagavannarayana, G., and Wahab, M. J. S. S. C. (2009). Characterization of ZnSe nanoparticles synthesized by microwave heating process. Solid State Commun. 149, 2047–2049. doi:10.1016/j.ssc.2009.08.021
Sharma, S., and Bhattacharya, A. J. A. W. S. (2017). Drinking water contamination and treatment techniques. Appl. Water Sci. 7, 1043–1067. doi:10.1007/s13201-016-0455-7
Shkir, M., Yahia, I., Ganesh, V., Bitla, Y., Ashraf, I., Kaushik, A., et al. (2018). A facile synthesis of Au-nanoparticles decorated PbI2 single crystalline nanosheets for optoelectronic device applications. Sci. Rep. 8, 13806–13810. doi:10.1038/s41598-018-32038-5
Sohrabnezhad, S., and Seifi, A. J. A. S. S. (2016). The green synthesis of Ag/ZnO in montmorillonite with enhanced photocatalytic activity. Appl. Surf. Sci. 386, 33–40. doi:10.1016/j.apsusc.2016.05.102
Soltan, W. B., Nasri, S., Lassoued, M. S., and Ammar, S. J. (2017). Structural, optical properties, impedance spectroscopy studies and electrical conductivity of SnO2 nanoparticles prepared by polyol method. J. Mat. Sci. Mat. Electron. 28, 6649–6656. doi:10.1007/s10854-017-6356-1
Stehlik, B., Weidenthaler, P., and Vlach, J. (1959). Kristallstruktur von Silber(II)-oxyd. Collect. Czech. Chem. Commun. 24, 1416–1419. doi:10.1135/cccc19591416
Stewart, I. E., Kim, M. J., and Wiley, B. J. J. A. A. M. (2017). Interfaces Effect of morphology on the electrical resistivity of silver nanostructure films. ACS Appl. Mat. Interfaces 9, 1870–1876. doi:10.1021/acsami.6b12289
Tedjani, M. L., Khelef, A., Laouini, S. E., Bouafia, A., and Albalawi, N. (2022). Optimizing the antibacterial activity of iron oxide nanoparticles using central composite design. J. Inorg. Organomet. Polym. 32, 3564–3584. doi:10.1007/s10904-022-02367-0
Tulsky, E. G., and Long, J. R. (2001). Dimensional reduction: A practical formalism for manipulating solid structures. Chem. Mat. 13, 1149–1166. doi:10.1021/cm0007858
Wang, Y., Shi, R., Lin, J., Zhu, Y. J. E., and Science, E. (2011). Enhancement of photocurrent and photocatalytic activity of ZnO hybridized with graphite-like C 3 N 4. Energy Environ. Sci. 4, 2922–2929. doi:10.1039/c0ee00825g
Wijesinghe, U., Thiripuranathar, G., Iqbal, H., and Menaa, F. (2021). Biomimetic synthesis, characterization, and evaluation of fluorescence resonance energy transfer, photoluminescence, and photocatalytic activity of zinc oxide nanoparticles. Sustainability 13, 2004. doi:10.3390/su13042004
Wijesinghe, U., Thiripuranathar, G., Menaa, F., Iqbal, H., Razzaq, A., and Almukhlifi, H. (2021). Green synthesis, structural characterization and photocatalytic applications of ZnO nanoconjugates using Heliotropium indicum. Catalysts 11, 831. doi:10.3390/catal11070831
Xing, Y.-Z., Zhang, H., Liu, X.-B., and Zheng, Y.-M. J. N. P. A. (2017). Pau li-blocking effect in two-body collisions dominates the in-medium effects in heavy-ion reactions near Fermi energy. Nucl. Phys. A 957, 135–143. doi:10.1016/j.nuclphysa.2016.08.006
Xu, L., Wang, Y.-Y., Huang, J., Chen, C.-Y., Wang, Z.-X., and Xie, H. J. T. (2020). Silver nanoparticles: Synthesis, medical applications and biosafety. Theranostics 10, 8996–9031. doi:10.7150/thno.45413
Yadav, A. A., Kang, S.-W., and Hunge, Y. M. (2021). Photocatalytic degradation of Rhodamine B using graphitic carbon nitride photocatalyst. J. Mat. Sci. Mat. Electron. 32, 15577–15585. doi:10.1007/s10854-021-06106-y
Yao, Y., Li, G., Ciston, S., Lueptow, R. M., and Gray, K. A. (2008). Photoreactive TiO2/carbon nanotube composites: Synthesis and reactivity. Environ. Sci. Technol. 42, 4952–4957. doi:10.1021/es800191n
Yu, X., Yang, H., and Wang, H. (2022). A cleaner biocementation method of soil via microbially induced struvite precipitation: A experimental and numerical analysis. J. Environ. Manag. 316, 115280. doi:10.1016/j.jenvman.2022.115280
Zeng, S., Baillargeat, D., Ho, H.-P., and Yong, K.-T. J. C. S. R. (2014). Nanomaterials enhanced surface plasmon resonance for biological and chemical sensing applications. Chem. Soc. Rev. 43, 3426–3452. doi:10.1039/c3cs60479a
Zhang, J., Ding, E., Xu, S., Li, Z., Fakhri, A., and Gupta, V. K. (2020). Production of metal oxides nanoparticles based on poly-alanine/chitosan/reduced graphene oxide for photocatalysis degradation, anti-pathogenic bacterial and antioxidant studies. Int. J. Biol. Macromol. 164, 1584–1591. doi:10.1016/j.ijbiomac.2020.07.291
Zhang, R., Lee, P., Lui, V. C., Chen, Y., Liu, X., Lok, C. N., et al. (2015). Biology; Medicine. Silver nanoparticles promote osteogenesis of mesenchymal stem cells and improve bone fracture healing in osteogenesis mechanism mouse model. Nanomedicine Nanotechnol. Biol. Med. 11, 1949–1959. doi:10.1016/j.nano.2015.07.016
Keywords: MgO@AgO/Ag2O NPs, green synthesis, Portulaca oleracea, photocatalysis, methylene blue, toluidine blue
Citation: Zidane Y, Laouini SE, Bouafia A, Meneceur S, Tedjani ML, Alshareef SA, Almukhlifi HA, Al-Essa K, Al-Essa EM, Rahman MM, Madkhali O and Menaa F (2022) Green synthesis of multifunctional MgO@AgO/Ag2O nanocomposite for photocatalytic degradation of methylene blue and toluidine blue. Front. Chem. 10:1083596. doi: 10.3389/fchem.2022.1083596
Received: 29 October 2022; Accepted: 28 November 2022;
Published: 15 December 2022.
Edited by:
Barkat. A. Khan, Gomal University, PakistanReviewed by:
J. Uddin, Coppin State University, United StatesM. Hasnat, Shahjalal University of Science and Technology, Bangladesh
Copyright © 2022 Zidane, Laouini, Bouafia, Meneceur, Tedjani, Alshareef, Almukhlifi, Al-Essa, Al-Essa, Rahman, Madkhali and Menaa. This is an open-access article distributed under the terms of the Creative Commons Attribution License (CC BY). The use, distribution or reproduction in other forums is permitted, provided the original author(s) and the copyright owner(s) are credited and that the original publication in this journal is cited, in accordance with accepted academic practice. No use, distribution or reproduction is permitted which does not comply with these terms.
*Correspondence: Mohammed L. Tedjani, medlaidtedjani@gmail.com; Farid Menaa, menaateam@gmail.com