- 1Chemistry Department, College of Sciences and Humanities, Prince Sattam Bin Abdulaziz University, Al-Kharij, Saudi Arabia
- 2Chemistry Department, Faculty of Science, Minia University, El-Minia, Egypt
- 3Pharmaceutical Organic Chemistry Department, Faculty of Pharmacy, Assiut University, Asyut, Egypt
- 4Institute of Organic Chemistry, Karlsruher Institut fur Technologie, Karlsruhe, Germany
- 5Institute of Biological and Chemical Systems (IBCS-FMS), Karlsruhe Institute of Technology, Karlsruhe, Germany
- 6Chemistry Department, Florida Institute of Technology, Melbourne, FL, United States
- 7Computational Chemistry Laboratory, Chemistry Department, Faculty of Science, Minia University, Minia, Egypt
Thiourea derivatives of uracil were efficiently synthesized via the reaction of 5-aminouracil with isothiocyanates. Then, we prepared uracil-containing thiazoles via condensation of thioureas with diethyl/dimethyl acetylenedicarboxylates. The structures of the products were confirmed by a combination of spectral techniques including infra-red (IR), nuclear magnetic resonance (NMR), mass spectrometry (MS) and elemental analyses. A rationale for the formation of the products is presented. The newly synthesized compounds were evaluated for their in vitro antiproliferative activity against four cancer cell lines. The compounds tested showed promising antiproliferative activity, with GI50 values ranging from 1.10 µM to 10.00 µM. Compounds 3c, 5b, 5c, 5h, 5i, and 5j were the most potent derivatives, with GI50 values ranging from 1.10 µM to 1.80 µM. Compound 5b showed potent inhibitory activity against EGFR and BRAFV600E with IC50 of 91 ± 07 and 93 ± 08 nM, respectively, indicating that this compound could serve as a dual inhibitor of EGFR and BRAFV600E with promising antiproliferative properties. Docking computations revealed the great potency of compounds 5b and 5j towards EGFR and BRAFV600E with docking scores of −8.3 and −9.7 kcal/mol and −8.2 and −9.3 kcal/mol, respectively.
1 Introduction
Uracil compounds are promising structures in the field of drug discovery (Bouhadir et al., 2016; Putz and Dudas, 2013). Uracils substituted in position five stand out in bioactivity (Gimadieva et al., 2015), with various biological activities including antiviral properties (Palasz and Ciez, 2015), anticancer, cytotoxic (Tanase et al., 2015; Krutikov and Erkin, 2009), antimycobacterial (Isobe et al., 2003; Baraldi et al., 2002), and antitumor (Seferoglu and Ertan, 2008), to antibacterial (Lee et al., 1997). According to Rana and Ganesh (2000), 5-aminouracil (5-AU) (compound I, Figure 1) binds to receptors with high affinity by forming hydrogen-bonded triplexes via amino and carbonyl groups and a ring nitrogen. Interestingly, 5-aminouracil has antitumor, antibacterial, and antiviral properties (Zielenkiewicz et al., 2000). Furthermore, 5-AU is widely used as a cell cycle inhibitor (Oliev, 1994), as it inhibits the mitotic cycle and the incorporation of guanosine into nucleic acids (Roth and Cheng, 1982). Many thiourea derivatives demonstrate antibacterial, antifungal (Abbas et al., 2013a; El-Sharief et al., 2013), and antiviral (Abbas et al., 2013b) activities. Their ability to inhibit enzymes such as protein tyrosine kinases (PTKs) (Li et al., 2010), topoisomerase II (Huang et al., 2010), human sirtuin type proteins (Napper et al., 2005), and DNA repair synthesis (Ziegler-Skylakakis et al., 1985) may explain their anticancer activity. Some diaryl-thiourea derivatives have been reported to be EGFR inhibitors (Xiong et al., 2008). The thiourea derivative DC27 (compound II, Figure 1) was tested for antitumor activity in a panel of human lung carcinoma cell lines (Xiong et al., 2008). The outcomes demonstrated dose-dependent inhibition of cell proliferation, with an IC50 of 2.5–12.9 µM, comparable to gefitinib (1.1–15.6 µM). In contrast to gefitinib (IC50 = 0.018 ± 007 µM), DC27 showed potent inhibition of EGFR with a value of 0.020 ± 005 µM. Additionally, a flow cytometry study of DC27 (Xiong et al., 2008) induced apoptosis and G0/G1 cell cycle arrest.
The thiazolidin-4-one moiety has been proposed as a scaffold for constructing new molecules in medicinal chemistry. Positions 2, 3, and 5 of the thiazolidin-4-one ring, are amenable to modification. When modified with other substituents, thiazolidin-4-one exhibits a wide range of biological activities, including anticancer (Asati et al., 2014; Aly et al., 2020; Sharma et al., 2020). Thiazolidin-4-one hybrids were developed (Aziz et al., 2021), and their anticancer properties were tested on breast cancer (MCF-7) and lung cancer (A549) cell lines. The most effective derivative against the lung cancer (A549) cell line was compound III (Figure 1), with an IC50 value of 0.72 µM and promising EGFR inhibitory activity at a concentration of 65 nM.
In response to the previous, continuing our efforts to discover novel hybrids as inhibitors of cancer cell growth with dual targeting inhibitory action (Al-Wahaibi et al., 2020; Gomaa et al., 2022), we have now prepared two new series of hybrids (Figure 2): the dihydropyrimidine-2,4-dione/thioureas 3a-f (Scaffold A) and the dihydropyrimidine-2,4-dione/thiazolidin-4-ones 5a-l (Scaffold B). Our goal was to obtain a new antiproliferative agent that can target EGFR and/or BRAFV600E. Using an MTT assay, the compounds were tested on a panel of four different cancer cell lines. The EGFR and BRAF enzymatic assays were used to investigate the hybrids’ potential antiproliferative mechanism. A molecular docking study was conducted on the most active compounds within the target active sites of the enzymes.
2 Results and discussion
2.1 Chemistry
The syntheses of the target compounds are depicted in Schemes 1 and 2. The new thioureas 3a-f (Scaffold A) were synthesized by the reaction of 5-aminouracil (1) with isothiocyanate derivatives 2a-f in boiling methanol for 10–12 h. The structures of the target compounds 3a-f were confirmed by elemental analyses, IR, NMR (1H, 13C, 2D NMR, 15N), and mass spectroscopy. The 1H NMR spectrum of 3d showed six singlet signals at δH 11.33, 10.79, 8.69, 8.45, 7.97 and 4.69 ppm, assigned as NH-3, NH-1, NH-5a, NH-5c, CH-6 and CH2-benzyl protons, respectively. The aromatic protons were appeared between δH 7.25–7.30 ppm. The 13C NMR spectrum showed two carbonyl carbon (C=O) signals at δC 161.6 and 150.4 ppm, assigned as C-4 and C-2; there were also signals at δC 181.8, 134.8, and 112.7 ppm, assigned as C=S, C-6, and C-5 respectively. A non-protonated carbon at δC 138.9 ppm gave HMBC correlation with H-o, H-m, and H-5d; this carbon is assigned as C-i. The four nitrogens all gave HSQC correlation with their attached protons (Table 1; Figure 3).
A new series of pyrimidine-bearing thiazolidinones (Scaffold B, 5a-l) was synthesized by refluxing thioureas 3a-f with acetylenedicarboxylate derivatives 4a,b in methanol for 4–6 h in 76–85% yields. The spectral and elemental data revealed that all 5a-l derivatives underwent the reaction smoothly to give the respective 2,4-dioxo-1,2,3,4-tetrahydro-pyrimidin-5-yl)imino)-4-oxo-3-substituted thiazolidin-5-ylidene)-acetates.
The 1H NMR spectra showed the disappearance of NH-5a signal of the thiourea in molecule 3. For example, 5d formed by the reaction of compound 3d with diethyl acetylenedicarboxylate (4a). The signals of the ethoxy group were distinctive at δH 4.23 (H-5c′) and 1.25 (H-5d′), δC 61.5 (C-5c′) and 13.9 ppm (C-5d′): H-5c′ gave HMBC correlation with a carbon at δC 165.3 ppm, assigned as C-5b’. C-5b′ also gave HMBC correlation with a proton at δH 6.77 ppm, assigned as H-5a’; its attached carbon appeared at δC 115.2 ppm. H-5a′ also gave HMBC correlation with carbons at δC 140.5 and 164.1 ppm; these carbons are assigned as C-5′ and C-4′, respectively on chemical-shift grounds, confirmed by HMBC correlation between C-4′ and the singlet of CH2-benzyl at δH 5.02, assigned as H-3a’. H-3a′ gave HSQC correlation with its attached carbon at δC 45.5; H-3a′ also gave HMBC correlation with a nitrogen at δN 160.4, assigned as N-3′, and with a carbon at δC 153.0, assigned as C-2’. H-6 gave HMBC correlation with an sp2 nitrogen at δN 244.8 ppm, assigned as N-5a, and with carbon at δC 159.6. The IR spectrum of 5d showed strong absorption bands between ν = 3150 (NH), 2975 (Ar-CH), 1687 (CO), 1647 (C=N) and 1510 cm−1 (C=C). The mass spectrum and elemental analyses of 5d agreed with the assigned structure (Table 2; Figure 4).
On reacting compounds 3a-f with dimethyl acetylene dicarboxylate (4b), methyl (Z)-2-((Z)-2-((2,4-dioxo-1,2,3,4-tetrahydropyrimidin-5-yl)imino)-3-yl-4-oxothiazolidin-5-ylidene) acetates 5g-l were formed. The structure assignments of 5g–I were delineated from their spectroscopic properties and elemental analyses. The molecular structure of 5j, for example, was supported as follows: the molecular formula of 5j (C17H14N4O5S) corresponded to one molecule of 3d and one molecule of dimethyl acetylenedicarboxylate (4b) less one molecule of methanol, giving rise to the ion m/z = 386. The NMR spectra of 5j closely resembled those of 5d, with a methyl ester replacing the ethyl ester (Table 3).
We recently reacted thioureas bearing a [2.2]paracyclophane moiety with diethyl acetylene-dicarboxylate (4a) to form thiazolidinones (Alshammari et al., 2022). An X-ray crystal structure showed the C=N and C=C double bonds to both have (Z) stereochemistry (Figure 5); in the current series 5a-l, we assign the C=N and C=C bonds as both (Z) by analogy with our earlier work. Further evidence was based upon the suggestion that there is a resonance stabilized by the hydrogen bond formed via the oxygen of the carbonyl group and the exo-cyclic hydrogen as shown in Figure 6.
The rationale for forming 5a-l begins with conjugate attack by the sulfur lone-pair of the thione group in 3a-f on the triple bond of 4a,b by the nitrogen lone pairs to generate the zwitterions 6a-l. Subsequently, proton migration would give intermediates 7a-l (Scheme 3). Finally, the lone pair of the nitrogen atom in the intermediate 7a-l would attack the carbonyl group of the same compound, which is accompanied by the elimination of an alcohol molecule to give the final product 5a-l (Scheme 3).
2.2 Biology
2.2.1 Cell viability assay
To test the viability of new compounds, the human mammary gland epithelial (MCF-10A) cell line was used (Youssif et al., 2019; Mahmoud et al., 2022). MCF-10A cells were incubated with compounds 3a-f and 5a-l for 4 days before being tested for viability using the MTT assay. Table 4 shows that none of the compounds tested exhibited cytotoxic effects, and cell viability was greater than 86% for the compounds tested at 50 µM.
2.2.2 Antiproliferative assay
Using the MTT assay (Abdelrahman et al., 2017; El-Sherief et al., 2018) and doxorubicin as the reference drug, 3a-f and 5a-l were tested for antiproliferative activity against four human cancer cell lines: Panc-1 (pancreas cancer cell line), MCF-7 (breast cancer cell line), HT-29 (colon cancer cell line), and A-549 (epithelial cancer cell line). The median inhibitory concentrations (IC50) are shown in Table 4.
The 18 newly synthesized compounds have two main backbones: thiourea-based derivatives, Scaffold A (3a-f), and thiazolidin-4-ones, Scaffold B (5a-l). The compounds tested showed promising antiproliferative activity, with GI50 values ranging from 1.10 µM to 10.00 µM. Compounds 3c, 5b, 5c, 5h, 5i, and 5j were the most potent derivatives of both backbones, with GI50 values ranging from 1.10 µM to 1.80 µM. Compound 5j (R = benzyl, R1 = Me; thiazolidin-4-one backbone) demonstrated the most potent activity, with a GI50 value of 1.10 µM, comparable to the reference doxorubicin (GI50 = 1.10 µM) and even more potent than doxorubicin against A-549 and Panc-1 cancer cell lines, as shown in Table 4. Compound 5d (R = benzyl, R1 = Et; thiazolidin-4-one backbone) showed a GI50 of 5.15 µM, which is approximately 5-fold less potent than compound 5j, indicating the importance of methyl ester for antiproliferative action, which is more tolerated than ethyl ester. Furthermore, compound 3d (R = benzyl; thiourea-based backbone) showed moderate antiproliferative activity with a GI50 value of 4.15 µM, four times less active than compound 5j of thiazolidin-4-one backbone, indicating that thiazolidin-4-one backbone is more tolerated for antiproliferative action than thiourea one.
Compound 5b (R = p-CH3-Ph, R1 = Et; thiazolidin-4-one backbone) ranks second in activity, with a GI50 of 1.30 µM, which is 1.2-fold less potent than compounds 5j and doxorubicin. Compound 5b had the same potency as doxorubicin against both A-549 and Panc-1 cancer cell lines, with IC50 values of 1.20 µM and 1.40 µM, respectively. 5b was found to be more potent than its methyl ester derivative, compound 5h (R = p-CH3-Ph, R1 = Me; thiazolidin-4-one backbone), which had a GI50 value of 1.65 µM (Table 1).
Once again, the methyl ester derivative compound 5i (R = m-OCH3-Ph, R1 = Me; thiazolidin-4-one backbone) outperformed the ethyl ester derivative 5c (R = m-OCH3-Ph, R1 = Et; thiazolidin-4-one backbone) with GI50 values of 1.35 µM and 1.70 µM against the four cancer cell lines, respectively. Moreover, the antiproliferative activity of compounds 5i and 5c (thiazolidin-4-one-based derivatives; R = m-OMe-Ph) was comparable to that of compound 5b (thiazolidin-4-one-based derivatives; R = p-Me-Ph), indicating that both m-OMe-Ph and p-Me-Ph groups are well tolerated. Compound 3c (R = m-OCH3-Ph; thiourea-based backbone) was the only thiourea-based derivative with a GI50 less than 2 µM (GI50 = 1.80 µM), confirming that thiazolidin-4-one based derivatives have higher inhibitory activity against the tested cancer cell line than thiourea-based derivatives.
2.2.3 EGFR inhibitory assay
The six most potent antiproliferative derivatives (3c, 5b, 5c, 5h, 5i, and 5j) were further tested for their inhibitory action against EGFR (Mohamed et al., 2021) as a potential target for their antiproliferative activity. Table 5; Figure 7 shows the results as IC50 values.
The findings of this test are consistent with the findings of the antiproliferative assay, in which compound 5j (R = benzyl, R1 = Me; thiazolidin-4-one backbone), the most potent antiproliferative, demonstrated the highest inhibitory activity against EGFR with an IC50 value of 87 ± 05 nM, which is very close to that of the reference erlotinib (IC50 = 80 ± 05 nM). Compounds 5b (R = p-CH3-Ph, R1 = Et; thiazolidin-4-one backbone) and 5i (R = m-OCH3-Ph, R1 = Me; thiazolidin-4-one backbone) rank second and third in activity with IC50 values of 91 ± 07 nM and 97 ± 07 nM, respectively. Compound 3c (R = m-OCH3-Ph; thiourea-based backbone) was the least potent of the six compounds tested, with an IC50 value of 125 ± 11 nM, making it 1.6-fold less potent than erlotinib. Based on the results of this assay, compounds 5b, 5i, and 5j showed promising antiproliferative activity and have the potential to act as EGFR inhibitors.
2.2.4 BRAFV600E inhibitory assay
Compounds 3c, 5b, 5c, 5h, 5i, and 5j were further tested for their inhibitory action against mutant BRAF (Mohassab, A. M., et al., 2021), and results were cited in Table 5 and Figure 7 as IC50 values. The tested compounds showed moderate inhibitory activity against the tested mutant BRAF, with IC50 values ranging from 93 nM to 148 nM and were all less potent than the reference erlotinib (IC50 = 60 ± 05 nM). Compound 5b (R = p-CH3-Ph, R1 = Et; thiazolidin-4-one backbone) was the most potent derivative as BRAFV600E inhibitor with IC50 of 93 ± 08 nM indicating that this compound could serve as a dual inhibitor of EGFR and BRAFV600E with promising antiproliferative properties.
2.3 Molecular docking
AutoDock4.2.6 software was utilized to carry out all docking computations (Morris et al., 2009). The crystal structures of EGFR and BRAFV600E with PDB accession codes: 1M17 (Stamos et al., 2002) and 3OG7 (Bollag et al., 2010), respectively, were obtained and utilized as templates for all docking computations. The pdbqt file of both EGFR and BRAFV600E was prepared as described by Ibrahim et al. (2022a) and Ibrahim et al. (2022b). The Lamarckian genetic algorithm (LGA) opted for inhibitor conformational searching and docking parameters involving 25,000,000 energy evaluations and 250 genetic algorithm runs. The rest parameters were kept as default. A grid box with 50 Å × 50 Å × 50 Å in the x, y, and z directions were utilized to include the binding pocket of EGFR and BRAFV600E. The grid maps with a spacing of 0.375 Å were generated utilizing the AutoGrid program (Goodford, 1985). The grid was positioned at the center of the active sites of EGFR and BRAFV600E. The molecular interactions were depicted using the BIOVIA Discovery Studio Visualizer 2020 (Dassault Systèmes, 2019).
To reveal the binding modes of the synthesized compounds with the active site of the EGFR and BRAFV600E, docking computations were performed. Validation of the AutoDock4.2.6 software with the employed parameters was initially executed according to the accessible experimental data. The co-crystallized inhibitors–namely 4-anilinoquinazoline and PLX4032—with the EGFR and BRAFV600E were redocked and compared to the native structures (PDB ID; 1M17 and 3OG7, respectively) (Figure 8). As shown in Figure 8, the predicted docking poses were approximately identical to the co-crystallized structures, having 0.20 and 0.18 Å RMSD compared to the co-crystallized conformations of 4-anilinoquinazoline and PLX4032, respectively (Figure 8). In essence, the utilized docking protocol could be applied to foretell the correct binding mode of ligands with the targeted receptors.
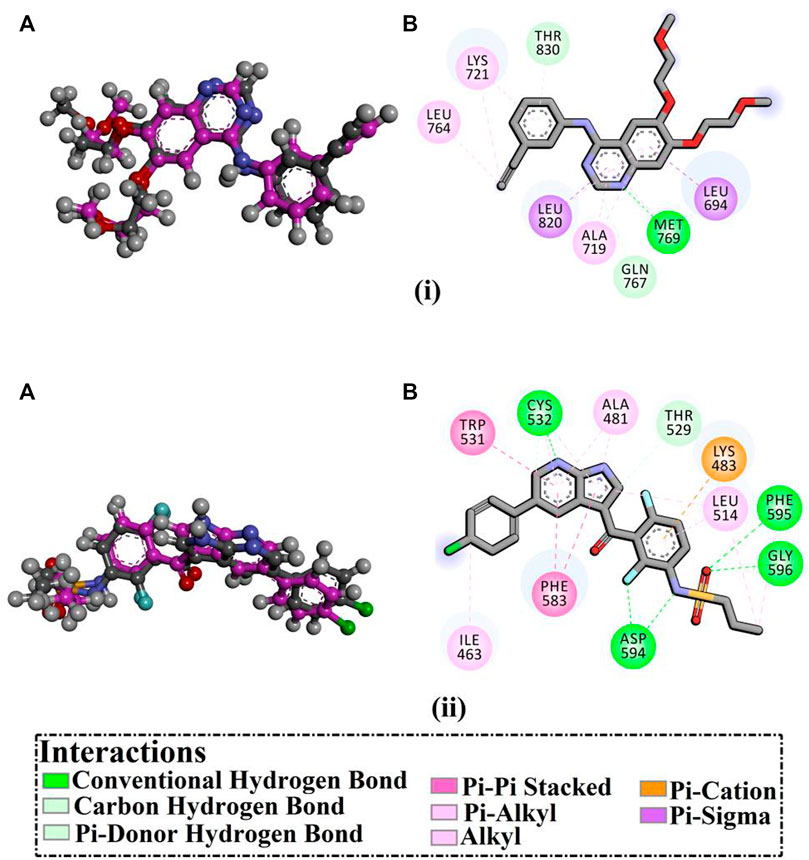
FIGURE 8. (A) 3D molecular interactions of the predicted binding modes (in purple) and the native structures (in gray) and (B) 2D representations of the portended binding modes of (i) 4-anilinoquinazoline with EGFR and (ii) PLX4032 with BRAFV600E.
Based on the validated performance of AutoDock4.2.6 software, it was utilized to predict the docking scores and binding features of the synthesized compounds against EGFR and BRAFV600E. The anticipated binding features and docking scores are compiled in Supplementary Table S1. Based on the data enrolled in Supplementary Table S1, all investigated molecules demonstrated superior docking scores against EGFR and BRAFV600E, with values ranging from −8.0 to −8.3 and from −9.1 to −9.7 kcal/mol, respectively. The special binding affinities against EGFR and BRAFV600E may be attributed to their capability of exhibiting a diversity of H-bonds, π-based, hydrophobic, and vdW interactions with the most important amino acids inside the binding pockets of EGFR and BRAFV600E. Comparing the docking results demonstrated that compounds 5b and 5j unveiled promising docking scores against EGFR and BRAFV600E with values of −8.3 and −9.7 kcal/mol and −8.2 and −9.3 kcal/mol, respectively. More exactly, compound 5b exhibited four and five hydrogen bonds with LYS721 (2.14 Å), MET769 (2.09, 2.43 Å), and GLY772 (2.83 Å) and THR529 (2.24, 3.03 Å), CYS532 (2.13 Å), ASN580 (2.65 Å), and ASN581 (2.12 Å) within the binding pockets of EGFR and BRAFV600E, respectively (Supplementary Table S1; Figure 9).
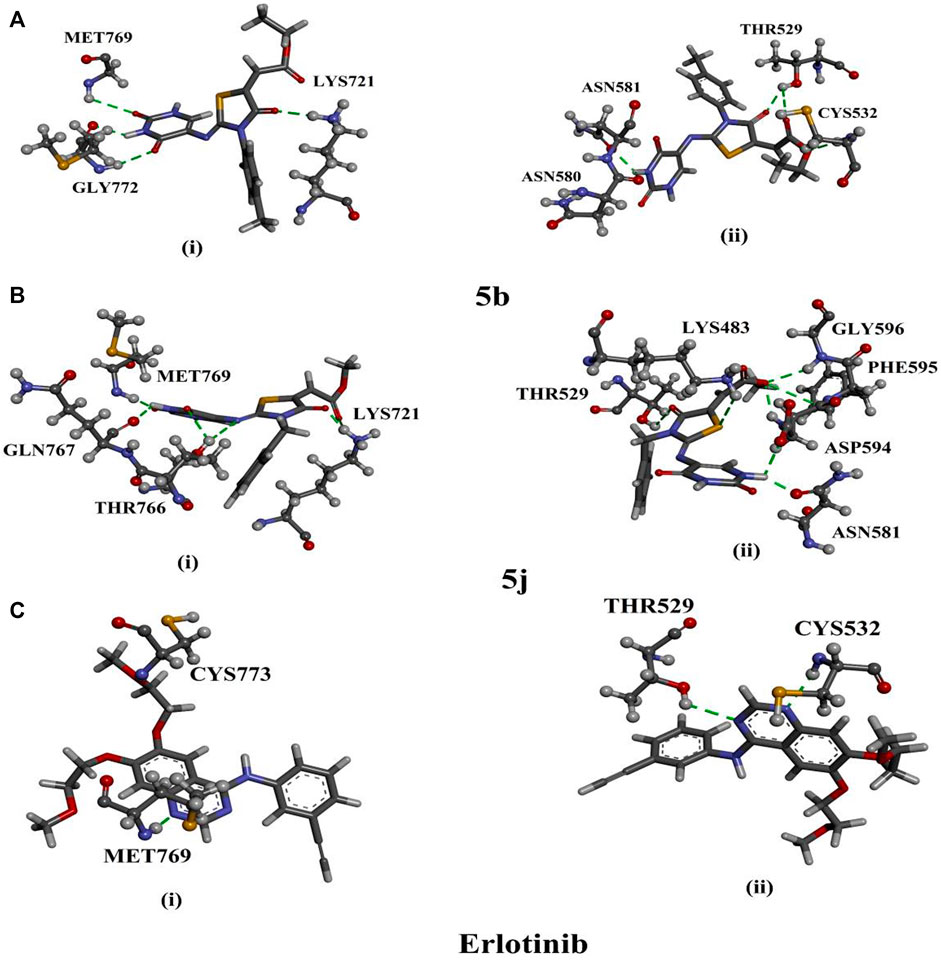
FIGURE 9. 3D representations of interactions of compounds (A) 5b, (B) 5j, and (C) erlotinib with key residues of (i) EGFR and (ii) BRAFV600E.
Compound 5j formed six and seven hydrogen bonds with LYS721 (1.63, 2.96 Å), THR766 (2.44, 2.84 Å), GLN767 (2.29 Å), and MET769 (1.82 Å) and LYS483 (1.86 Å), THR529 (2.52 Å), ASN581 (1.97 Å), ASP594 (2.08, 2.20 Å), PHE595 (2.95 Å), and GLY596 (2.39 Å) inside the binding sites of EGFR and BRAFV600E, respectively (Supplementary Table S1; Figure 9).
Erlotinib, a positive control, manifested good docking scores towards EGFR and BRAFV600E with values of −8.6 and −8.4 kcal/mol, respectively (Supplementary Table S1). As illuminated in Figure 9, erlotinib demonstrated two hydrogen bonds with MET769 (1.62 Å) and CYS773 (1.91 Å) within the active site of EGFR and two hydrogen bonds with THR529 (2.07 Å) and CYS532 (2.02 Å) within the active site of BRAFV600E (Supplementary Table S1). A docking comparison of erlotinib with compounds 5b and 5j exposed competing docking scores proposing the in-silico perspective of the three molecules as EGFR and BRAFV600E inhibitors.
3 Experimental
Instrumentation: See Supplementary Appendix SA.
3.1 General procedure for the synthesis of compounds 3a-f
Compounds 3a-f were synthesized by refluxing of 5-aminouracil (1, 10 mmol) and different isothiocyanates 2a-f (1.2 mmol) in methanol (50 ml) and the presence of a few drops of triethylamine (0.5 ml) as a catalyst for 10–12 h. The resulting solid was filtered and recrystallized from DMF.
3.1.1 1-(2,4-Dioxo-1,2,3,4-tetrahydropyrimidin-5-yl)-3-phenylthiourea (3a)
Yield: 84%; mp 340–342°C, IR (KBr): υmax/cm−1 = 3165 (NH), 2991 (Ar-CH), 1735 (CO), 1669 (CO), 1574 (C=C), 1330, 1208 (C=S). 1H NMR (DMSO-d6): δH = 7.15 (t, 1H, J = 7.4 Hz, Ar-H), 7.34 (dd, 2H, J = 8.1, 7.6 Hz, Ar-H), 7.48 (d, 2H, J = 7.8 Hz, Ar-H), 11.41 (bs, 1H, 3NH), 8.16 (bs, 1H, CH-6), 8.87 (s, 1H, 5aNH), 9.98 (bs, 1H, 5cNH), 10.82 ppm (bs, 1H, 1NH), 13C NMR (DMSO-d6): δC = 113.2 (C-5), 123.9, 124.7, 128.4 (CH-Ar), 134.9 (C-6), 139.2 (C-Ar), 150.1 (2C = O), 161.5 (4C = O), 179.7 ppm (C=S). 15N NMR (DMSO-d6): δN = 126.9 (N-1), 131.8 (N-5c), 157.0 ppm (N-3). MS: m/z = 262 (M+, 40), 228 (100), 185 (12), 169 (15), 157 (15), 103 (50), 77 (15). Anal. Calcd for C11H10N4O2S (262.29):C, 50.37; H, 3.84; N, 21.36; S, 12.23. Found: C, 50.46; H, 3.87; N, 21.48; S, 12.31.
3.1.2 1-(2,4-Dioxo-1,2,3,4-tetrahydropyrimidin-5-yl)-3-(p-tolyl)thiourea (3b)
Yield: 82%; mp 338–340°C, IR (KBr): υmax/cm−1 = 3165 (NH), 2994 (Ar-CH), 1743 (CO), 1668 (CO), 1575 (C=C), 1330, 1210 (C=S). 1H NMR (DMSO-d6): δH = 2.29 (s, 3H, CH3), 7.14 (d, 2H, J = 8.2 Hz, Ar-H), 8.15 (s, 1H, CH-6), 8.78 (s, 1H, 5aNH), 9.88 (bs, 1H, 5cNH), 10.80 (bs, 1H, 1NH), 11.39 ppm (bs,1H, 3NH). 13C NMR (DMSO-d6): δC = 20.5 (CH3), 113.3 (C-5), 124.1, 128.8 (CH-Ar), 134.0 (C-6), 136.5 (C-Ar), 150.1 (2C = O), 161.5 (4C = O), 179.7 ppm (C=S). 15N NMR (DMSO-d6): δN = 111.9 (N-5a, 5c), 126.8 (N-1), 156.9 ppm (N-3). MS: m/z = 276 (M+, 10), 242 (100), 169 (16), 149 (25), 127 (27), 117 (52), 91 (28). Anal. Calcd for C12H12N4O2S (276.31): C, 52.16; H, 4.38; N, 20.28; S, 11.60. Found: C, 52.27; H, 4.41; N, 20.36; S, 11.71.
3.1.3 1-(2,4-Dioxo-1,2,3,4-tetrahydropyrimidin-5-yl)-3-(3-methoxyphenyl)thiourea (3c)
Yield: 84%; mp = 350–352°C, IR (KBr) υmax/cm−1 = 3164 (NH), 2996 (Ar-CH), 1741 (CO), 1668 (CO), 1574 (C=C), 1330, 1208 (C=S). 1H NMR (DMSO-d6): δH = 3.75 (s, 3H, OCH3), 6.73 (dd, 1H, J = 8.1, 2.0 Hz, Ar-H), 7.02 (bd, 1H, J = 7.9 Hz, Ar-H), 7.22 (bs, 1H, Ar-H), 7.24 (t, 1H, J = 8.2 Hz, Ar-H), 8.16 (b, 1H, H-6), 8.87 (bs, 1H,5cNH), 10.00 (b, 1H, 5aNH), 10.81 (bs, 1H, 1NH), 11.40 ppm (bs, 1H, 3NH). 13C NMR (DMSO-d6): δC = 55.1 (OCH3), 109.3, 110.2 (CH-Ar), 113.3 (C-5), 115.7, 129.2 (CH-Ar), 140.3 (C-Ar), 134.9 (C-6), 150.1 (2C = O), 161.5 (4C = O), 179.4 ppm (C=S). MS: m/z = 292 (M+, 28), 264 (100), 233 (30), 157 (28), 143 (14), 84 (44), 77 (35). 15N NMR (DMSO-d6): δN = 113.5 (N-5c), 126.7 (N-1), 156.9 ppm (N-3). Anal. Calcd for C12H12N4O3S (292.31): C, 49.31; H, 4.14; N, 19.17; S, 10.97. Found: C, 49.45; H, 4.17; N, 19.32; S, 10.86.
3.1.4 1-Benzyl-3-(2,4-dioxo-1,2,3,4-tetrahydropyrimidin-5-yl)thiourea (3d)
Yield: 83%; mp = 350–352 (decomp) °C, IR (KBr) υmax/cm−1 = 3162 (NH), 2998 (Ar-CH), 1743 (CO), 1668 (CO), 1575 (C=C), 1330, 1210 (C=S). NMR (DMSO-d6): See Table 1; Figure 3. MS: m/z = 276 (M+, 24), 244 (100), 171 (20), 157 (35), 143 (20), 98 (41), 91 (48). Anal. Calcd for C12H12N4O2S (276.31): C, 52.16; H, 4.38; N, 20.28; S, 11.60. Found: C, 52.27; H, 4.41; N, 20.40; S, 11.72.
3.1.5 1-(2,4-Dioxo-1,2,3,4-tetrahydropyrimidin-5-yl)-3-methylthiourea (3e)
Yield: 85%; mp = 332–334°C, IR (KBr) υmax/cm−1 = 3127 (NH), 3086 (Ar-CH), 1689 (CO), 1654 (CO), 1553 (C=C), 1335, 1207 (C=S). 1H NMR (DMSO-d6): δH = 2.85 (d, 3H, J = 4.2 Hz, CH3), 7.89 (bs, 2H,5aNH, CH-6), 8.53 (bs, 1H, 5cNH), 10.77 ppm (bs, 1H, 1NH), 11.29 ppm (bs, 1H, 3NH). 13C NMR (DMSO-d6): δC = 31.1 (CH3), 112.7 (C-5), 136.3 (C-6), 150.4 (2C = O), 161.7 (4C = O), 182.1 ppm (C=S). 15N NMR (DMSO-d6): δN = 104.6 (N-5a), 106.1 (N-5c), 127.3 (N-1), 157.0 ppm (N-3). MS: m/z = 200 (M+, 24), 127 (100), 56 (52). Anal. Calcd for C6H8N4O2S (200.22): C, 35.99; H, 4.03; N, 27.98; S, 16.02. Found: C, 35.89; H, 4.07; N, 28.05; S, 16.12.
3.1.6 1-Allyl-3-(2,4-dioxo-1,2,3,4-tetrahydropyrimidin-5-yl)thiourea (3f)
Yield: 83%; mp = 348–350 °C (decomp), IR (KBr) υmax/cm−1 = 3209 (NH), 3011 (Ar-CH), 1738 (CO), 1667 (CO), 1574 (C=C), 1328, 1205 (C=S). 1H NMR (DMSO-d6): δH = 4.08 (bs, 2H, N-CH2), 5.07 (d, 1H, J = 10.2, H-5f), 5.17 (dd, 1H, J = 17.2, 1.1 Hz, H-5f), 5.83 (ddt, 1H, Jd = 17.2, 10.4 Hz, Jt = 5.2 Hz, H-5e), 7.98 (b, 1H, H-6), 8.14 (b, 1H; NH-5a), 8.62 (bs, 1H, NH-5c), 10.76 (b, 1H, NH-1), 11.31 ppm (bs, 1H, NH-3). 13C NMR (DMSO-d6): δC = 46.2 (N-CH2), 112.9 (C-5), 115.5 (C-5f), 134.7 (C-5e, C-6), 150.3 (2C = O), 161.6 (4C = O), 182.4 ppm (C=S). MS: m/z = 226 (M+, 100), 127 (100), 98 (55), 84 (15), 56 (44). 15N NMR (DMSO-d6): δN = 107.0 (N-5a), 114.9 (N-5c), 157.2 ppm (N-3), Anal. Calcd for C8H10N4O2S (226.26): C, 42.47; H, 4.45; N, 24.76; S, 14.17. Found: C, 42.56; H, 4.48; N, 24.88; S, 14.25.
3.2 General procedure for the synthesis of compounds 5a-l
A solution of 3a-f (1 mmol) in methanol (20 ml) was added to a 100 ml round bottom flask containing 4a or 4b (1.2 mmol) in methanol (10 ml), with refluxing for 4–6 h. After cooling, the yellow precipitate was filtered off, washed with methanol, and recrystallized from a suitable solvent to give pure crystals of 5a-l.
3.2.1 (Z)-Ethyl-2-((Z)-2-((2,4-dioxo-1,2,3,4-tetrahydropyrimidin-5-yl)imino)-4-oxo-3-phenyl-thiazolidin-5-ylidene)acetate (5a)
Yield: 76%; mp 320–322°C, IR (KBr) υmax/cm−1 = 3147 (NH), 2979 (Ar-CH), 1686 (CO), 1643 (C=N), 1510 (C=C). 1H NMR (DMSO-d6): δH = 1.24 (t, 3H, J = 6.9 Hz, CH3), 4.20 (q, 2H, J = 7.0 Hz, CH2), 6.86 (s, 1H, CH-5a′), 6.92–6.94 (d, 2H, J = 7.8 Hz, Ar-H),7.20–7.23 (t, 2H, J = 7.8 Hz, Ar-H), 7.45–7.47 (d, 2H, J = 7.8 Hz, Ar-H), 8.00 (s, 1H, CH-6), 11.45 (bs, 1H, 1NH), 11.68 ppm (bs, 1H, 3NH). 13C NMR (DMSO-d6): δC = 13.9 (CH3), 61.6 (OCH2), 116.5 (C-5′a), 120.7 (C-5), 125.2, 128.2, 129.0, 129.5 (CH-Ar), 134.2 (C-Ar), 140.1 (C-6), 143.7 (C-5′), 147.0 (2C = O), 150.6 (C-2′), 159.8 (C-4), 163.5 (C-4′), 165.2 ppm (C-5′b). MS: m/z = 388 (M+2, 24), 387 (M+1, 100), 386 (M+, 32), 373 (10), 289 (7), 229 (15), 172 (80), 136 (65). Anal. Calcd for C18H20N8O7S2 (524.53): C, 41.22; H, 3.84; N, 21.36; S, 12.23. Found: C, 41.37; H, 3.87; N, 21.43; S, 12.30.
3.2.2 (Z)-Ethyl-2-((Z)-2-((2,4-dioxo-1,2,3,4-tetrahydropyrimidin-5-yl)imino)-4-oxo-3-(p-tolyl)thiazolidin-5-ylidene)acetate (5b)
Yield: 78%; mp 348–350°C, IR (KBr) υmax/cm−1 = 3148 (NH), 2979 (Ar-CH), 1686 (CO), 1644 (C=N), 1505 (C=C). 1H NMR (DMSO-d6): δH = 1.24 (t, 3H, J = 7.1 Hz, CH3), 2.32 (s, 3H, CH3), 3.28 (s, 3H, NCH3), 4.22 (q, 2H, J = 7.1 Hz, CH2), 6.58 (d, 1H, J = 5.2, 0.6 Hz, H-6), 6.83 (d, 2H, J = 8.3 Hz, Ar-H),6.85 (s, 1H, H-5′a), 7.22 (d, 2H, J = 8.00 Hz, Ar-H), 11.05 (bs, 1H, 1NH), 11.42 ppm (bs, 1H, 3NH). 13C NMR (DMSO-d6): δC = 13.9 (C-5d′), 20.5 (CH3), 61.6 (C-5c′), 108.0 (C-5), 116.4 (C-5a′), 116.4 (C-6), 120.6 (C-o), 129.9 (C-m), 134.4 (C-p), 143.8 (C-i), 144.5 (C-2′), 150.6 (C-2), 163.5 (C-4), 161.6 (C-4′), 165.2 ppm (C-5b′). 15N NMR (DMSO-d6): δN = 119.2 (N-1), 155.8 ppm (N-3). MS: m/z = 402 (M+2, 20), 401 (M+1, 85), 400 (M+, 30), 373 (18), 301 (10), 243 (10), 154 (25), 149 (48), 136 (22), 107 (14), 91 (14). Anal. Calcd for C18H16N4O5S (400.41): C, 53.99; H, 4.03; N, 13.99; S, 8.01. Found: C, 53.90; H, 4.06; N, 14.07; S, 8.11.
3.2.3 (Z)-Ethyl-2-((Z)-2-((2,4-dioxo-1,2,3,4-tetrahydropyrimidin-5-yl)imino)-3-(3-methoxyphenyl)-4-oxo-thiazolidin-5-ylidene)acetate (5c)
Yield: 75%; mp 330–332°C, IR (KBr) υmax/cm−1 = 3142 (NH), 2950 (Ar-CH), 1691 (CO), 1648 (C=N), 1598 (C=C). 1H NMR (DMSO-d6): δH = 1.24 (t, 3H, J = 7.1 Hz, H-5d′), 3.76 (s, 3H, OCH3), 4.22 (q, 2H, J = 7.1 Hz, H-5c′), 6.46 (d, 1H, J = 1.9 Hz, H-2″), 6.51 (d, 1H, J = 8.6 Hz, H-6″), 6.79 (dd, 1H, J = 8.2 Hz, 1.7 Hz, H-4″), 6.86 (s, 1H, H-5a′), 7.32 (dd, 1H, J = 8.1, 8.0 Hz, H-5″), 7.99 (s, 1H, H-5), 11.45 (b, 1H, NH-1), 11.67 ppm (bs, 1H, NH-3). 13C NMR (DMSO-d6): δC = 13.9 (C-5d′), 55.2 (C-3a''), 61.6 (C-5c′), 106.3 (C-2″), 108.0 (C-5), 111.0 (C-4″), 112.7 (C-6″), 116.5 (C-5a''), 130.3 (C-5″), 140.2 (C-5′), 143.7 (C-6), 148.3 (C-1″), 150.6 (C-2′), 150.9 (C-1), 159.8 (C-3″), 160.1 (3″), 163.5 (C-4′), 165.2 ppm (C-5b′). 15N NMR (DMSO-d6): δN = 133.1 (N-1), 158.3 ppm (N-3). MS: m/z = 418 (M+2, 20), 417 (M+1, 84), 416 (M+, 32), 372 (10), 289 (15), 259 (14), 195 (10), 154 (100), 137 (66), 107 (22). Anal. Calcd for C18H16N4O6S (416.41): C, 51.92; H, 3.87; N, 13.45; S, 7.70. Found: C, 51.98; H, 3.85; N, 13.56; S, 7.78.
3.2.4 (Z)-Ethyl-2-((Z)-3-benzyl-2-((2,4-dioxo-1,2,3,4-tetrahydropyrimidin-5-yl)imino)-4-oxo-thiazolidin-5-ylidene)acetate (5d)
Yield: 85%; mp 314–316°C, IR (KBr) υmax/cm−1 = 3150 (NH), 2975 (Ar-CH), 1687 (CO), 1647 (C=N), 1510 (C=C). NMR (DMSO-d6): See Table 2; Figure 4. MS: m/z = 402 (M+2, 25), 401 (M+1, 100), 400 (M+, 25), 341 (8), 313 (9), 289 (10), 91 (30). Anal. Calcd for C18H16N4O5S (400.41): C, 53.99; H, 4.03; N, 13.99; S, 8.01. Found: C, 53.90; H, 4.06; N, 14.07; S, 8.11.
3.2.5 (Z)-Ethyl-2-((Z)-2-((2,4-dioxo-1,2,3,4-tetrahydropyrimidin-5-yl)imino)-3-methyl-4-oxo-thiazolidin-5-ylidene)acetate (5e)
Yield: 84%; mp 302–304°C, IR (KBr) υmax/cm−1 = 3149 (NH), 3062 (Ar-CH), 1716 (CO), 1674 (CO), 1647 (C=N), 1520 (C=C). 1H NMR (DMSO-d6): δH = 1.25 (t, 3H, J = 6.9 Hz, CH3), 3.28 (s, 3H, NCH3), 4.23 (q, 2H, J = 7.0 Hz, CH2), 6.77 (s, 1H, CH-5′a), 7.25 (s, 1H, CH-6), 10.92 (bs, 1H, 1NH), 11.41 ppm (bs, 1H, 3NH). 13C NMR (DMSO-d6): δC = 13.9 (CH3), 29.2 (NCH3), 61.5 (OCH2), 115.2 (C-5′a), 121.1 (C-5), 131.0 (C-6), 141.0 (C-5′), 150.4 (2C = O), 153.9 (C-2′), 159.6 (C-4), 164.4 (C-4′), 165.3 ppm (C-5b′). 15N NMR (DMSO-d6): δN = 127.0 (N-1), 149.0 (N-3′), 158.2 (N-3), 244.8 ppm (N-5a). MS: m/z = 326 (M+2, 5), 325 (M+1, 30), 324 (M+, 20), 289 (10), 279 (5), 242 (5), 195 (10). Anal. Calcd for C12H12N4O5S (324.31): C, 44.44; H, 3.73; N, 17.28; S, 9.89. Found: C, 44.56; H, 3.80; N, 17.40; S, 9.98.
3.2.6 (Z)-Ethyl-2-((Z)-3-allyl-2-((2,4-dioxo-1,2,3,4-tetrahydropyrimidin-5-yl)imino)-4-oxo-thiazolidin-5-ylidene)acetate (5f)
Yield: 76%; mp 310–312°C, IR (KBr) υmax/cm−1 = 3148 (NH), 2989 (Ar-CH), 1714 (CO), 1675 (CO), 1643 (C=N), 1510 (C=C). 1H NMR (DMSO-d6): δH = 1.25 (t, 3H, J = 7.1 Hz, CH3), 4.23 (q, 2H, J = 7.1 Hz, OCH2), 4.44 (d, 2H, J = 5.2 Hz, NCH2), 5.19 (dd, 1H, J = 10.3 Hz, 1.0 Hz, 3c′CH), 5.21 (dd, 1H, J = 17.2 Hz, 1.1 Hz, CH-3c′), 5.89 (ddt, 1H, Jd = 17.2, 10.4 Hz, Jt = 5.2 Hz, CH-3b′), 6.78 (s, 1H, CH-5a′), 7.27 (d, 1H, J = 6.6 Hz, CH-6), 10.94 (bd, 1H, J = 4.7 Hz, 1NH), 11.40 ppm (bs, 1H, 3NH). 13C NMR (DMSO-d6): δC = 13.9 (CH3), 44.4 (NCH2), 61.5 (OCH2), 115.5 (C-5a′), 117.7 (C-3c′), 120.8 (C-5), 130.9 (C-3b′), 131.3 (C-6), 140.7 (C-5′), 150.4 (C-2), 152.8 (C-2′), 159.5 (C-4), 163.9 (C-4′), 165.2 ppm (C-5b′). 15N NMR (DMSO-d6): δN = 127.3 (N-1), 157.2 (N-3′), 158.1 (N-3), 245.0 ppm (N-4a). MS: m/z = 352 (M+2, 20), 351 (M+1, 100), 350 (M+, 30), 335 (5), 289 (10), 273 (5), 107 (20). Anal. Calcd for C14H14N4O5S (350.35): C, 47.99; H, 4.03; N, 15.99; S, 9.15. Found: C, 47.87; H, 4.07; N, 15.89; S, 9.21.
3.2.7 (Z)-Methyl-2-((Z)-2-((2,4-dioxo-1,2,3,4-tetrahydropyrimidin-5-yl)imino)-4-oxo-3-phenyl-thiazolidin-5-ylidene)acetate (5g)
Yield: 83%; mp 302–304°C, IR (KBr) υmax/cm−1 = 3307, 2975, 1740, 1647. 1H NMR (DMSO-d6): δH = 3.76 (s, 3H, OCH3), 6.89 (s, 1H, H-5a′), 6.93 (d, 2H, J = 7.4 Hz, Ar-H), 7.22 (t, 1H, J = 7.4 Hz, Ar-H), 7.42 (dd, 2H, J = 8.0, 7.7 Hz, Ar-H), 8.00 (s, 1H, H-6), 11.45 (b, 1H, 1NH), 11.67 ppm (b, 1H, 3NH). 13C NMR (DMSO-d6): δC = 52.6 (CH3), 108.0 (C-5), 116.3 (C-5a′), 120.7 (C-o), 125.2 (C-p), 129.5 (C-m), 140.2 (C-5′), 143.8 (C-6), 147.0 (C-i), 150.6, 150.7 (C-2, 2′), 159.8 (C-4), 163.5 (C-4′), 165.6 ppm (C-5b′). 15N NMR (DMSO-d6): δN = 133.5 (N-1), 151.4 ppm (N-3). Anal. Calcd for C16H12N4O5S (372.36): C, 51.61; H, 3.25; N, 15.05; S, 8.61. Found: C, 51.72; H, 3.28; N, 15.16; S, 8.73.
3.2.8 (Z)-Methyl-2-((Z)-2-((2,4-dioxo-1,2,3,4-tetrahydropyrimidin-5-yl)imino)-4-oxo-3-(p-tolyl)-thiazolidin-5-ylidene)acetate (5h)
Yield: 78%; mp 308–310°C, IR (KBr) υmax/cm−1 = 3215 (NH), 3092 (Ar-CH), 1710 (CO), 1679 (CO), 1641 (C=N), 1512 (C=C). 1H NMR (DMSO-d6): δH = 2.32 (s, 3H, CH3), 3.67 (s, 3H, OCH3), 6.87 (s, 1H, H-5a′), 7.13–7.15 (m, 4H, Ar-H), 8.00 (s, 1H; H-6), 11.44 (s, 1H, 1NH), 11.66 ppm (b, 1H, 3NH). 13C NMR (DMSO-d6): δC = 20.45 (CH3), 52.7 (OCH3), 107.9 (C-5), 116.2 (C-5a′), 120.6, 125.1, 129.9 (CH-Ar), 139.7 (C-5′), 143.8 (C-6), 144.2, 147.0 (C-Ar), 150.5 (C-2), 151.1 (C-2′), 160.0 (C-4), 163.5 (C-4′), 165.6 ppm (C-5b′). MS: m/z = 388 (M+2, 35), 387 (M+1, 100), 386 (M+, 25), 341 (10), 289 (15), 273 (10), 242 (10), 217 (20), 195 (35), 107 (20). Anal. Calcd for C17H14N4O5S (386.38): C, 52.84; H, 3.65; N, 14.50; S, 8.30. Found: C, 52.93; H, 3.69; N, 14.61; S, 8.43.
3.2.9 (Z)-Methyl-2-((Z)-2-((2,4-dioxo-1,2,3,4-tetrahydropyrimidin-5-yl)imino)-3-(3-methoxyphenyl)-4-oxothiazolidin-5-ylidene)acetate (5i)
Yield: 80%; mp 322–324°C, IR (KBr) υmax/cm−1 = 3198 (NH), 2998 (Ar-CH), 1711 (CO), 1674 (CO), 1641 (C=N), 1510 (C=C). 1H NMR (DMSO-d6): δH = 3.77 (s, 6H, 2OCH3), 6.46 (bs, 1H, Ar-H), 6.51 (d, J = 7.8 Hz, 1H, Ar-H), 6.79 (d, 1H, J = 7.4 Hz, Ar-H), 6.89 (s, 1H, H-5a′), 7.32 (dd, 1H, J = 8.0, 8.0 Hz, Ar-H), 8.00 (bd, 1H, H-6), 11.45 (b, 1H, 1NH), 11.67 ppm (bs, 1H, 3NH). 13C NMR (DMSO-d6): δC = 52.6 (OCH3), 55.2 (OCH3), 106.3 (CH-Ar), 108.0 (C-5), 111.0 (CH-Ar), 112.7 (CH-Ar), 116.3 (C-5a′), 130.4 (CH-Ar), 140.2 (C-5′), 143.7 (C-6), 148.3 (Ar-C), 150.8 (C-1), 150.6 (C-2′), 160.1 (C-4), 159.8 (Ar-C), 163.5 (C-4′), 165.6 ppm (C-5b′). 15N NMR (DMSO-d6): δN = 133.1 (N-1), 158.1 (N-3), 145.5 ppm (N-3′). MS: m/z = 404 (M+2, 25), 403 (M+1, 100), 402 (M+, 38), 391 (5), 273 (10), 274 (15), 258 (30), 167 (32). Anal. Calcd for C17H14N4O6S (402.38): C, 50.74; H, 3.51; N, 13.92; S, 7.97. Found: C, 50.86; H, 3.55; N, 13.85; S, 7.85.
3.2.10 (Z)-Methyl-2-((Z)-3-benzyl-2-((2,4-dioxo-1,2,3,4-tetrahydropyrimidin-5-yl)imino)-4-oxothiazolidin-5-ylidene)acetate (5j)
Yield: 85%; mp 306–308°C, IR (KBr) υmax/cm−1 = 3307, 2975, 1740, 1647. NMR (DMSO-d6): See Table 3. MS: m/z = 404 (M+2, 25), 403 (M+1, 100), 402 (M+, 38), 391 (5), 273 (10), 274 (15), 258 (30), 167 (32). Anal. Calcd for C17H14N4O5S (386.38): C, 52.84; H, 3.65; N, 14.50; S, 8.30. Found: C, 52.94; H, 3.68; N, 14.62; S, 8.44.
3.2.11 (Z)-Methyl-2-((Z)-2-((2,4-dioxo-1,2,3,4-tetrahydropyrimidin-5-yl)imino)-3-methyl-4-oxothiazolidin-5-ylidene)acetate (5k)
Yield: 85%; mp 300–302°C, IR (KBr) υmax/cm−1 = 3307, 2975, 1740, 1647. 1H NMR (DMSO-d6): δH = 3.27 (s, 3H, NCH3), 3.77 (s, 3H, OCH3), 6.80 (s, 1H, H-5a′), 7.24 (d, 1H, J = 5.1 Hz, H-6), 10.92 (bs, 1H, 1NH), 11.40 ppm (bs, 1H, 3NH). 13C NMR (DMSO-d6): δC = 29.3 (NCH3), 52.5 (OCH3), 115.0 (C-5a′), 121.0 (C-5), 131.0 (C-6), 141.0 (C-5’), 150.4 (C-2), 153.8 (C-2′), 159.6 (C-4), 164.4 (C-4′), 165.7 ppm (C-5b′). 15N NMR (DMSO-d6): δN = 127.2 (N-1), 158.0 (N-3), 160.9 ppm (N-3′). Anal. Calcd for C11H10N4O5S (310.29): C, 42.58; H, 3.25; N, 18.06; S, 10.33. Found: C, 42.70; H, 3.28; N, 18.15; S, 10.44.
3.2.12 (Z)-Methyl-2-((Z)-3-allyl-2-((2,4-dioxo-1,2,3,4-tetrahydropyrimidin-5-yl)imino)-4-oxothiazolidin-5-ylidene)acetate (5l)
Yield: 78%; mp 314–316°C, IR (KBr) υmax/cm−1 = 3147 (NH), 2999 (Ar-CH), 1710 (CO), 1675 (CO), 1644 (C=N), 1511 (C=C). 1H NMR (DMSO-d6): δH = 3.77 (s, 3H, OCH3), 4.21 (d, 2H, J = 5.2 Hz, NCH2), 5.19 (dd, 1H, J = 10.3 Hz, 1.0 Hz, 3c′CH), 5.22 (dd, 1H, J = 17.2 Hz, 1.1 Hz, CH-3c′), 5.80 (ddt, 1H, Jd = 17.2, 10.4 Hz, Jt = 5.2 Hz, CH-3b′), 6.75 (s, 1H, CH-5a′), 7.24 (d, 1H, J = 6.6 Hz, CH-6), 10.89 (bs, 1H, 1NH), 11.42 ppm (bs, 1H, 3NH). 13C NMR (DMSO-d6): δC = 42.6 (NCH2), 53.6 (OCH3), 116.1 (C-5a′), 116.5 (C-3c′), 120.6 (C-5), 130.0 (C-3b′), 134.4 (C-6), 140.3 (C-5′), 150.3 (C-2), 150.6 (C-2′), 159.8 (C-4), 163.5 (C-4′), 165.6 ppm (C-5b′). MS: m/z = 336 (M+, 35), 321 (20), 305 (10), 273 (5), 277 (14), 250 (25), 125 (100). Anal. Calcd for C13H12N4O5S (336.32): C, 46.43; H, 3.60; N, 16.66; S, 9.53. Found: C, 46.56; H, 3.64; N, 16.75; S, 9.66.
4 Biology
Supplementary Appendix SA contains information on all biological assay tests.
5 Conclusion
Due to the importance of thiazolidinone-pyrimidine derivatives, we direct for the synthesis of 2,4-dioxo-1,2,3,4-tetrahydropyrimidin-5-yl)imino)-4-oxo-3-yl-thiazolidin-5-ylidene)acetates 5a-l through the reaction of thioureas 3a-f with diethyl/dimethyl acetylenedicarboxylates (4a,b). The structure of compounds was examined by 1H, 13C-NMR, 2D-NMR, and 15N-NMR spectroscopy and elemental analyses. Compounds 5b and 5j were the most potent EGFR and BRAFV600E inhibitors and could be used as dual EGFR and BRAFV600E inhibitors with promising antiproliferative properties. Moreover, the synthesized molecules were in-silico inspected towards EGFR and BRAFV600E as anticarcinoma drug candidates using AutoDock4.2.6 software. Based on docking scores, compounds 5b and 5j disclosed auspicious docking scores towards EGFR and BRAFV600E. These findings shed new light on the importance of compounds 5b and 5j as appropriate therapeutic treatments for cancer disease.
Data availability statement
The original contributions presented in the study are included in the article/Supplementary Material, further inquiries can be directed to the corresponding authors.
Author contributions
CRediT authorship contribution statement. MA: editing and revision. ASA: Conceptualization, writing, and editing. BY: Biology, methodology, writing, and editing, AB: NMR, editing. AKA: editing. SB: Editing and revision; AM: Conceptualization, writing, methodology, editing and writing the draft.
Acknowledgments
The authors thank the Deanship of Scientific Research at Prince Sattambin Abdulaziz University under research project No 2021/01/18104. We also acknowledge support from the KIT-Publication Fund of the Karlsruhe Institute of Technology.
Conflict of interest
The authors declare that the research was conducted in the absence of any commercial or financial relationships that could be construed as a potential conflict of interest.
Publisher’s note
All claims expressed in this article are solely those of the authors and do not necessarily represent those of their affiliated organizations, or those of the publisher, the editors and the reviewers. Any product that may be evaluated in this article, or claim that may be made by its manufacturer, is not guaranteed or endorsed by the publisher.
Supplementary material
The Supplementary Material for this article can be found online at: https://www.frontiersin.org/articles/10.3389/fchem.2022.1076383/full#supplementary-material
References
Abbas, S. Y., Farag, A. A., Ammar, Y. A., Atrees, A. A., Mohamed, A. F., and El-Henawy, A. A. (2013a). Synthesis, characterization, and antiviral activity of novel fluorinated isatin derivatives. Monatsh. Chem. 144, 1725–1733. doi:10.1007/s00706-013-1034-3
Abbas, S. Y., El-Sharief, M. A. M. S., Basyouni, W. M., Fakhr, I. M. I., and El-Gammal, E. W. (2013b). Thiourea derivatives incorporating a hippuric acid moiety: Synthesis and evaluation of antibacterial and antifungal activities. Eur. J. Med. Chem. 64, 111–120. doi:10.1016/j.ejmech.2013.04.002
Abdelrahman, M. H., Aboraia, H. S., Youssif, B. G. M., and Elsadek, B. E. M. (2017). Design, synthesis and pharmacophoric model building of new 3-alkoxy-methyl/3-phenyl indole-2-carboxamides with potential antiproliferative activity. Chem. Biol. Drug Des. 90, 64–82. doi:10.1111/cbdd.12928
Alshammari, M. B., Aly, A. A., Bräse, S., Nieger, M., and Abd El-Haleem, L. E. (2022). Efficient synthesis of various substituted (thio)ureas, semicarbazides, thiosemicarbazides, thiazolidones, and oxadiazole derived from [2.2]paracyclophane. ACS Omega 7, 12879–12890. doi:10.1021/acsomega.2c00141
Al-Wahaibi, L. H., Gouda, A. M., Abou-Ghadir, O. F., Salem, O. I. A., Ali, A. T., Farghaly, H. S., et al. (2020). Design and synthesis of novel 2, 3-dihydropyrazino[1, 2-a]indole-1, 4-dione derivatives as antiproliferative EGFR and BRAFV600E dual inhibitors. Bioorg. Chem. 104, 104260. doi:10.1016/j.bioorg.2020.104260
Aly, A. A., Bräse, S., Hassan, A. A., Mohamed, N. K., Abd El-Haleem, L. E., Nieger, M., et al. (2020). New paracyclophanyl-thiazoles of anti-leukemia activity; design, synthesis, molecular docking, and mechanistic study. Molecules 25, 3089. doi:10.3390/molecules25133089
Asati, V., Mahapatra, D. K., and Bharti, S. K. (2014). Thiazolidine-2, 4-diones as multi-targeted scaffold in medicinal chemistry: Potential anticancer agents. Eur. J. Med. Chem. 87, 814–833. doi:10.1016/j.ejmech.2014.10.025
Aziz, M. W., Kamal, A. M., Mohamed, K. O., and Elgendy, A. A. (2021). Design, synthesis, and assessment of new series of quinazolinone derivatives as EGFR inhibitors along with their cytotoxic evaluation against MCF7 and A549 cancer cell lines. Bioorg. Med. Chem. Lett. 41, 127987. doi:10.1016/j.bmcl.2021.127987
Baraldi, P. G., Romagnoli, R., Guadix, A. E., Pineda de las Infantas, M. J., Gallo, M. A., Espinosa, A., et al. (2002). Design, synthesis, and biological activity of hybrid compounds between uramustine and DNA minor groove binder distamycin A. J. Med. Chem. 45, 3630–3638. doi:10.1021/jm011113b
Bollag, G., Hirth, P., Tsai, J., Zhang, J., Ibrahim, P. N., Cho, H., et al. (2010). Clinical efficacy of a RAF inhibitor needs broad target blockade in BRAF-mutant melanoma. Nature 467, 596–599. doi:10.1038/nature09454
Bouhadir, K. H., Koubeissi, A., Mohsen, F. A., El-Harakeh, M. D., Cheaib, R., Younes, J., et al. (2016). Novel carbocyclic nucleoside analogs suppress glomerular mesangial cells proliferation and matrix protein accumulation through ROS-dependent mechanism in the diabetic milieu. II. Acylhydrazone-functionalized pyrimidines. Bioorg. Med. Chem. Lett. 26, 1020–1024. doi:10.1016/j.bmcl.2015.12.042
Dassault Systèmes (2019). BIOVIA, B.D.S.V.; Version 2019. San Diego, CA, USA: Dassault Systèmes BIOVIA.
El-Sharief, M. A. M. S., Abbas, S. Y., El-Bayouki, K. A. M., and El-Gammal, E. W. (2013). Synthesis of thiosemicarbazones derived from N-(4-hippuric acid)-thiosemicarbazide and different carbonyl compounds as antimicrobial agents. EurJ. Med. Chem. 67, 263–268. doi:10.1016/j.ejmech.2013.06.031
El-Sharief, H. A. M., Youssif, B. G. M., Bukhari, S. N. A., Abdel-Aziz, M., and Abdel-Rahman, H. M. (2018). Novel 1, 2, 4-triazole derivatives as potential anticancer agents: Design, synthesis, molecular docking, and mechanistic studies. Bioorg. Chem. 76, 314–325. doi:10.1016/j.bioorg.2017.12.013
Gimadieva, A. R., Khazimullina, Yu.Z., Belaya, E. A., Zimin, Yu.S., Abdrakhmanov, L. B., and Mustafin, A. G. (2015). Express evaluation of antioxidant activity of uracil derivatives. Biomed. Khim. 61, 765–769. doi:10.18097/pbmc20156106765
Gomaa, H. A. M., Shaker, M. E., Alzarea, S. I., Hendawy, O. M., Mohamed, F. A. M., Gouda, A. M., et al. (2022). Optimization and SAR investigation of novel 2, 3-dihydro-pyrazino[1, 2-a]indole-1, 4-dione derivatives as EGFR and BRAFV600E dual inhibitors with potent antiproliferative and antioxidant activities. Bioorg. Chem. 120, 105616. doi:10.1016/j.bioorg.2022.105616
Goodford, P. J. (1985). A computational procedure for determining energetically favorable binding sites on biologically important macromolecules. J. Med. Chem. 28, 849–857. doi:10.1021/jm00145a002
Huang, H., Chen, Q., Xin, K., Meng, L., Lin, L., Wang, X., et al. (2010). A series of alpha-heterocyclic carboxaldehyde thiosemicarbazones inhibit topoisomerase IIα catalytic activity. J. Med. Chem. 53, 3048–3064. doi:10.1021/jm9014394
Ibrahim, M. A. A., Abdeljawaad, K. A. A., Abdelrahman, A. H. M., Jaragh-Alhadad, L. A., Oraby, H. F., Elkaeed, E. B., et al. (2022a). Exploring natural product activity and species source candidates for hunting ABCB1 transporter inhibitors: An in silico drug discovery study. Molecules 27, 3104. doi:10.3390/molecules27103104
Ibrahim, M. A. A., Badr, E. A. A., Abdelrahman, A. H. M., Almansour, N. M., Mekhemer, G. A. H., Shawky, A. M., et al. (2022b). In silico targeting human multidrug transporter ABCG2 in breast cancer: Database screening, molecular docking, and molecular dynamics study. Mol. Inf. 41, 2060039. doi:10.1002/minf.202060039
Isobe, Y., Tobe, M., Inoue, Y., Isobe, M., Tsuchiya, M., and Hayashi, H. (2003). Structure and activity relationships of novel uracil derivatives as topical anti-inflammatory agents. Bioorg. Med. Chem. 11, 4933–4940. doi:10.1016/j.bmc.2003.09.012
Krutikov, V. I., and Erkin, A. V. (2009). 5-Arylideneaminouracils: II. Synthesis of sodium and ammonium salts. Russ. J. Gen. Chem. 79, 991–995. doi:10.1134/s1070363209050211
Lee, B.-H., Shin, J.-H., Lim, M.-K., Jang, T.-S., Park, J.-S., Kim, K.-H., et al. (1997). Partition property of 5-nitrothiopyrimidine nucleoside. Bull. Kor. Chem. Soc. 18, 734–736. doi:10.5012/bkcs.1997.18.7.734
Li, H.-Q., Yan, T., Yang, Y., Shi, L., Zhou, C.-F., and Zhu, H.-L. (2010). Synthesis and structure–activity relationships of N-benzyl-N-(X-2-hydroxybenzyl)-N′-phenylureas and thioureas as antitumor agents. Bioorg. Med. Chem. 18, 305–313. doi:10.1016/j.bmc.2009.10.054
Mahmoud, M. A., Mohammed, A. F., Salem, O. I. A., Gomaa, H. A. M., and Youssif, B. G. M. (2022). New 1, 3, 4-oxadiazoles linked with the 1, 2, 3-triazole moiety as antiproliferative agents targeting the EGFR tyrosine kinase. Arch. Pharm. 355, 2200009. doi:10.1002/ardp.202200009
Mohamed, F. A. M., Gomaa, H. A. M., Hendawy, O. M., Ali, A. T., Farghaly, H. S., Gouda, A. M., et al. (2021). Design, synthesis, and biological evaluation of novel EGFR inhibitors containing 5-chloro-3-hydroxymethyl-indole-2-carboxamide scaffold with apoptotic antiproliferative activity. Bioorg. Chem. 112, 104960. doi:10.1016/j.bioorg.2021.104960
Mohassab, A. M., Hassan, H. A., Abdelhamid, D., Gouda, A. M., Youssif, B. G. M., Tateishi, H., et al. (2021). Design and synthesis of novel quinoline/chalcone/1, 2, 4-triazole hybrids as potent antiproliferative agent targeting EGFR and BRAFV600E kinases. Bioorg. Chem. 106, 104510. doi:10.1016/j.bioorg.2020.104510
Morris, G. M., Huey, R., Lindstrom, W., Sanner, M. F., Belew, R. K., Goodsell, D. S., et al. (2009). AutoDock4 and AutoDockTools4: Automated docking with selective receptor flexibility. J. Comput. Chem. 30, 2785–2791. doi:10.1002/jcc.21256
Napper, A. D., Hixon, J., McDonagh, T., Keavey, K., Pons, J.-F., Barker, J., et al. (2005). Discovery of indoles as potent and selective inhibitors of the deacetylase SIRT1. J. Med. Chem. 48, 8045–8054. doi:10.1021/jm050522v
Oliev, R. (1994). Response to auxin by cells of Riella helicophylla during reversible arrest in different cell-cycle phases. Planta 194, 510–515. doi:10.1007/bf00714464
Palasz, A., and Ciez, D. (2015). In search of uracil derivatives as bioactive agents. Uracils and fused uracils: Synthesis, biological activity and applications. Eur. J. Med. Chem. 97, 582–611. doi:10.1016/j.ejmech.2014.10.008
Putz, M. V., and Dudas, N. A. (2013). Variational principles for mechanistic quantitative structure–activity relationship (QSAR) studies: Application on uracil derivatives’ anti-HIV action. Struct. Chem. 24, 1873–1893. doi:10.1007/s11224-013-0249-6
Rana, V. S., and Ganesh, K. N. (2000). Recognition of 5-aminouracil (U#) in the central strand of a DNA triplex: Orientation selective binding of different third strand bases. Nucleic Acids Res. 28, 1162–1169. doi:10.1093/nar/28.5.1162
Roth, B., and Cheng, C. C. (1982). Recent progress in the medicinal chemistry of 2, 4-diaminopyrimidines. Prog. Med. Chem. 19, 269–331. doi:10.1016/s0079-6468(08)70332-1
Seferoglu, Z., and Ertan, N. (2008). Synthesis, characterization and spectroscopic properties of some new phenylazo-6-aminouracil. Open Chem. 6, 81–88. doi:10.2478/s11532-007-0062-4
Sharma, P. C., Bansal, K. K., Sharma, A., Sharma, D., and Deep, A. (2020). Thiazole-containing compounds as therapeutic targets for cancer therapy. Eur. J. Med. Chem. 188, 112016. doi:10.1016/j.ejmech.2019.112016
Stamos, J., Sliwkowski, M. X., and Eigenbrot, C. (2002). Structure of the epidermal growth factor receptor kinase domain alone and in complex with a 4-anilinoquinazoline inhibitor. J. Biol. Chem. 277, 46265–46272. doi:10.1074/jbc.m207135200
Tanase, C. I., Draghici, C., Cojocaru, A., Galochkina, A. V., Orshanskaya, J. R., Zarubaev, V. V., et al. (2015). New carbocyclic N6-substituted adenine and pyrimidine nucleoside analogues with a bicyclo[2.2.1]heptane fragment as sugar moiety; synthesis, antiviral, anticancer activity and X-ray crystallography. Bioorg. Med. Chem. 23, 6346–6354. doi:10.1016/j.bmc.2015.08.033
Xiong, X., Liu, H., Fu, L., Li, L., Li, J., Luo, X., et al. (2008). Antitumor activity of a new N-substituted thiourea derivative, an EGFR signaling-targeted inhibitor against a panel of human lung cancer cell lines. Chemotherapy 54, 463–474. doi:10.1159/000159272
Youssif, B. G. M., Mohamed, A. M., Osman, E. E. A., Abou-Ghadir, O. F., Elnaggar, D. H., Abdelrahman, M. H., et al. (2019). 5-Chlorobenzo-furan-2-carboxamides: From allosteric CB1 modulators to potential apoptotic antitumor agents. Eur. J. Med. Chem. 177, 1–11. doi:10.1016/j.ejmech.2019.05.040
Ziegler-Skylakakis, K., Rossberger, S., and Andrae, U. (1985). Thiourea induces DNA repair synthesis in primary rat hepatocyte cultures and gene mutations in V79 Chinese hamster cells. Arch. Toxicol. 58, 5–9. doi:10.1007/bf00292608
Keywords: 5-AU, thiourea, thiazolidinone, EGFR, B-RAF, viability, molecular modeling
Citation: Alshammari MB, Aly AA, Youssif BGM, Bräse S, Ahmad A, Brown AB, Ibrahim MAA and Mohamed AH (2022) Design and synthesis of new thiazolidinone/uracil derivatives as antiproliferative agents targeting EGFR and/or BRAFV600E. Front. Chem. 10:1076383. doi: 10.3389/fchem.2022.1076383
Received: 21 October 2022; Accepted: 18 November 2022;
Published: 12 December 2022.
Edited by:
Pezhman Shiri, Shiraz University of Medical Sciences, IranReviewed by:
Ibrahim F. Nassar, Ain Shams University, EgyptAtefeh Roosta, Tianjin Institute of Industrial Biotechnology (CAS), China
Azim Ziyaei Halimehjani, Kharazmi University, Iran
Copyright © 2022 Alshammari, Aly, Youssif, Bräse, Ahmad, Brown, Ibrahim and Mohamed. This is an open-access article distributed under the terms of the Creative Commons Attribution License (CC BY). The use, distribution or reproduction in other forums is permitted, provided the original author(s) and the copyright owner(s) are credited and that the original publication in this journal is cited, in accordance with accepted academic practice. No use, distribution or reproduction is permitted which does not comply with these terms.
*Correspondence: Ashraf A. Aly, YXNocmFmYWx5NjNAeWFob28uY29t, YXNocmFmLnNoZWhhdGFAbXUuZWR1LmVn; Bahaa G. M. Youssif, Ymd5b3Vzc2lmQGp1LmVkdS5zYQ==; Stefan Bräse, c3RlZmFuLmJyYWVzZUBraXQuZWR1