- 1Central Nervous System Drug Key Laboratory of Sichuan Province, Department of Medicinal Chemistry, School of Pharmacy, Southwest Medical University, Luzhou, China
- 2Department of Chemistry, School of Basic Medical Sciences, Southwest Medical University, Luzhou, China
- 3School of Public Health, Southwest Medical University, Luzhou, China
A metal- and aldehyde-free visible-light-driven photoredox-neutral alkene acylarylation with readily available cyanoarenes is described. A variety of 3-(arylmethyl)chroman-4-ones (i.e., homoisoflavonoids) and analogs are efficiently synthesized with good functional group tolerance. This mild protocol relies on a phosphoranyl radical-mediated acyl radical-initiated cyclization and selective radical-radical coupling sequence, and is also further highlighted by subsequent derivatization to chromone and 2H-chromene as well as its application in the three-component alkene acylarylation.
Introduction
Chroman-4-one scaffolds, a class of important oxygen-containing structural motifs, are ubiquitous in a plethora of natural products, drug candidates, and biologically active molecules (Albrecht et al., 2005; Nibbs and Scheidt, 2011; Friden-Saxin et al., 2012; Lee et al., 2014; Seifert et al., 2014; Emami and Ghanbarimasir, 2015; Kumar et al., 2017; Mayuri et al., 2017). In the past years, the radical-initiated cascade cyclization strategy has attracted great attention for the construction of chroman-4-one scaffold and other (hetero)cyclic frameworks (Zhao et al., 2016; Yang et al., 2017; Hu et al., 2018a; Hu et al., 2018b; Liu et al., 2019; Sheng et al., 2019; Xiao et al., 2019; Zhou et al., 2019a; Das et al., 2020; Han et al., 2020; Huang et al., 2020; Liu et al., 2020; Mei et al., 2020; Xiong et al., 2020; Diana et al., 2021). Particularly, the photocatalytic radical-initiated cascade cyclization, including two mechanistically distinctive pathways, has emerged as an elegant, green, and powerful strategy for the synthesis of such scaffold and its derivatives. The first photocatalytic approach to diversely functionalized chroman-4-ones via various external radical-initiated cascade cyclization of o-(allyloxy) arylaldehydes is well-developed by the groups of Zhu (Lu et al., 2017; Zhou et al., 2019b), Yu (Zhu et al., 2021), Xuan (He et al., 2019), and others (Zhou et al., 2019a; Huang et al., 2020; Mei et al., 2020; Liu et al., 2022) (Figure 1A). In contrast, there are only a few examples of photocatalytic internal acyl radical-initiated cascade cyclization (Jung et al., 2017; Norman et al., 2018; Stache et al., 2018; Zhou et al., 2021) (Figures 1B–D), which limits their application for the rapid assembly of structurally diverse chroman-4-ones. Recently, Hong group (Jung et al., 2017) and Wan group (Zhou et al., 2021) independently developed a visible-light-driven radical cyclization/epoxidation of o-(allyloxy)arylaldehydes toward spiroepoxy chroman4-one scaffolds using Ru (bpy)3Cl2 or organoselenium as photocatalyst and tert-butyl hydroperoxide (TBHP) as oxidant (Figure 1B). In 2018, McErlean group (Norman et al., 2018) disclosed a photoredox-catalyzed indirect acyl radical generation from relatively stable Crich-type thioesters generated in a single step with carboxylic acid starting materials, followed by intramolecular alkene addition/cyclization to give various cyclic ketones including chroman-4-one scaffold (Figure 1C). However, these existing strategies are solely based on the elaboration of uneasily available o-(allyloxy)arylaldehydes (almost all) or carboxylic acid thioesters (only one) and also suffer from one or more drawbacks such as excess amounts of oxidants, limited structural diversity, and lack of functionality tolerance. Therefore, the development of alternative and efficient approaches to access diversely functionalized chroman-4-one and related cyclic ketone analogs via photocatalytic internal acyl radical-initiated cascade cyclization using accessible starting materials should be highly desirable.
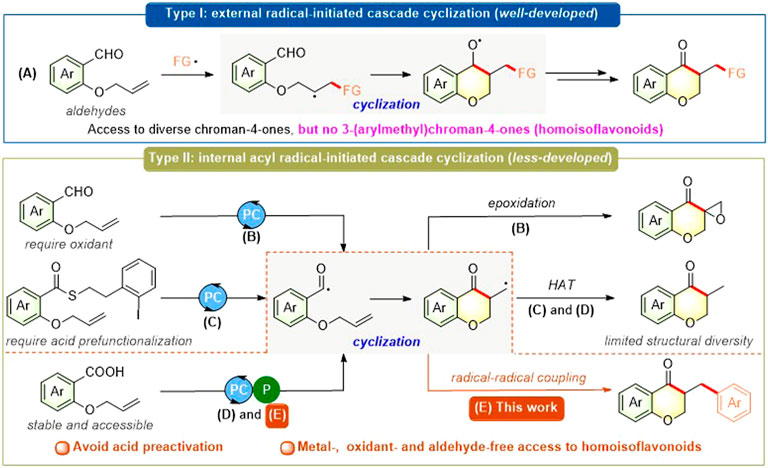
FIGURE 1. Photocatalytic radical-initiated cascade cyclization toward functionalized chroman-4-ones.
Carboxylic acids as starting materials are not only abundant, generally stable, and readily accessible in great structural diversity, and have also drawn much attention for their application as versatile radical precursors such as alkyl, aryl, carboxylic, and particularly acyl radicals (Mandal et al., 2018; Wang et al., 2019; Hu et al., 2020b; Chan et al., 2022; Kitcatt et al., 2022; Yan et al., 2022). Recently, an elegant strategy that combines photoredox catalysis and phosphoranyl radical-mediated deoxygenation makes it possible to form acyl radicals from carboxylic acids, providing streamlined access to structurally diverse ketones (Zhang et al., 2017; Stache et al., 2018). However, to the best of our knowledge, there are not only a few reports on the application of this powerful strategy to intermolecular and intramolecular alkene acylations including ipso-acylation (Li et al., 2022b), defluorinative acylation (Guo et al., 2020), and hydro-acylation (one example of hydro-acylation: one compound chroman-4-one using expensive iridium-based photocatalyst, Figure 1D) (Stache et al., 2018; Zhang et al., 2018; Martinez Alvarado et al., 2019; Merkens et al., 2021), but also no report on alkene difuntionalizations (especially carbon-acylation) with this strategy to date. Inspired by these work and seminal pioneering reports on the photoredox-catalyzed radical-type ipso-functionalizations of electron-deficient cyanoarene derivatives (Betori and Scheidt, 2019; Vorob’ev, 2019; Zhong et al., 2020; Zhou et al., 2020; Shen et al., 2021; Tong et al., 2021; Georgiou et al., 2022), we envisaged whether the radical relay strategy of the phosphoranyl radical-mediated acyl radical-initiated cascade cyclization from alkene-tethered carboxylic acids and subsequent radical-radical coupling process could enable the rapid construction of 3-(arylmethyl)chroman-4-ones, which are one of the core frameworks in a variety of homoisoflavonoids with various biological activities (Eggler et al., 1991; Desideri et al., 1997; Eggler et al., 1997; Tait et al., 2006; Basavarajappa et al., 2015). Herein, we report an efficient and practical approach for the metal-, oxidant-, and aldehyde-free synthesis of 3-(arylmethyl)chroman-4-ones and other cyclic ketone analogs via visible light-driven photoredox-neutral alkene acylarylation (being a class of alkene carbon-acylation, Figure 1E).
Results and discussion
To corroborate this hypothesis, we initially selected a model reaction of alkenoic acid 1a and 4-cyanopyridine 2a to explore the reaction conditions under 30 W blue LED irradiation at room temperature (Table 1). To our delight, the desired 3-(pyridylmethyl)chroman-4-one 3aa could be obtained in 75% yield by using 3DPAFIPN as a metal-free photocatalyst (entry 1). In light of the fact that the excited state *3DPAFIPN [E1/2 (PC*/PC•−) = +1.09 V vs. SCE] is a strong oxidant (Speckmeier et al., 2018), single electron transfer (SET) could occur from P (p-tol)3 (E1/2ox = +1.03 V vs. SCE, Supplementary Figure S3) to *3DPAFIPN. Additionally, the presence of alkenoic acid 1a shifted the reductive potential of 2a from −1.81 V vs. SCE to −1.33 V vs. SCE (Supplementary Figure S4), thus enabling SET between the reduced 3DPAFIPN•− [E1/2 (PC/PCred) = −1.59 V vs. SCE] and 2a to complete the photocatalytic cycle without any aid of external reductant and oxidant. Next, decreasing the loading of the photosensitizer from 2 mol% to 1 mol% or 0.5 mol% obtained a slightly decreasing yield (entry 1). Other organic photosensitizers such as 3DPA2FBN with suitable oxidative-reductive potential (Speckmeier et al., 2018) could also afford the desired chroman-4-one in good yields, while using 4CzIPN (Speckmeier et al., 2018) led to no desired product (entries 2–3). Furthermore, other electron-rich trivalent phosphorus compounds could also be used as the phosphorus source in this deoxygenative transformation (entries 4–8), whereas using relatively electron-deficient ones instead of P (p-tol)3 led to poor efficiency (entries 9–11). Then, the screening of solvents demonstrated that these photocatalytic reactions performed in CH2Cl2 or DCE also resulted in synthetically useful yields, while other solvents such as DMF, DMSO, and THF gave no desired product (entries 12–14). Further control experiments performed in the absence of light, photocatalyst, or phosphine failed to give the desired chroman-4-one, thus emphasizing their crucial role in this photocatalytic acylarylation (entry 15).
With the optimized reaction conditions in hand, we investigated the scope and limitations of this reaction using a variety of alkenoic acids (Figure 2). It was worth mentioning that this photocatalytic reaction could be run on a 1.0 mmol scale to provide the target product 3aa in 67% yield. Firstly, we examined the effect of the aromatic moiety of the substrate alkenoic acid. It was found that the electron-donating group (Me, OMe) and electron-withdrawing groups (F, Cl, Br, CF3) at the para- and mata-position with respect to the carboxylic acid were well compatible with this transformation and the corresponding chroman-4-ones were obtained with satisfactory yields (3ba–3ia). The structure of 3ga was confirmed by X-ray diffraction analysis (CCDC 2192065). Moreover, the ortho-F substituted alkenoic acid was also employed in this transformation, providing the desired chroman-4-one 3ja albeit in a relatively low yield. Then, we investigated the scope of the alkene moiety of the substrate alkenoic acid. 1,2-Disubstituted nonterminal alkenoic acid with a phenyl group at the terminal carbon participated well in such acylarylation to give the expected product 3ka, while one with an alkyl group was transformed into the compound 3la with a low yield. And 1,1-disubstituted or mono-substituted terminal alkenoic acid could also be subjected to this transformation, affording the corresponding chroman-4-ones (3ma–3oa) albeit with diminished yields. Interestingly, replacing the oxygen atom at the ortho-position with respect to the carboxylic acid by an atom of sulphur, nitrogen, or carbon favored the photocatalytic acylarylation, leading to the corresponding chroman-4-one analogs such as thiochroman-4-one 3pa, dihydroquinolin-4(1H)-one 3qa, and dihydronaphthalen-1(2H)-one 3ra. Additionally, N-(homo)allylindole-2-carboxylic acids were proved to be suitable heteroaromatic substrates for this photocatalytic process and gave the architecturally intriguing and valuable tricyclic ketone framework including 1H-pyrrolo [1,2-a]indol-1-one (3sa) and pyrido [1,2-a]indol-9(6H)-one (3ta) in comparable yields. These experimental outcomes fully highlighted the synthetic potential to construct structurally complex ketone-containing (hetero)cycles. However, pyridyl-substituted alkenoic acid 1u and acyclic aliphatic alkenoic acid 1v could be not suitable for this alkene acylarylation.
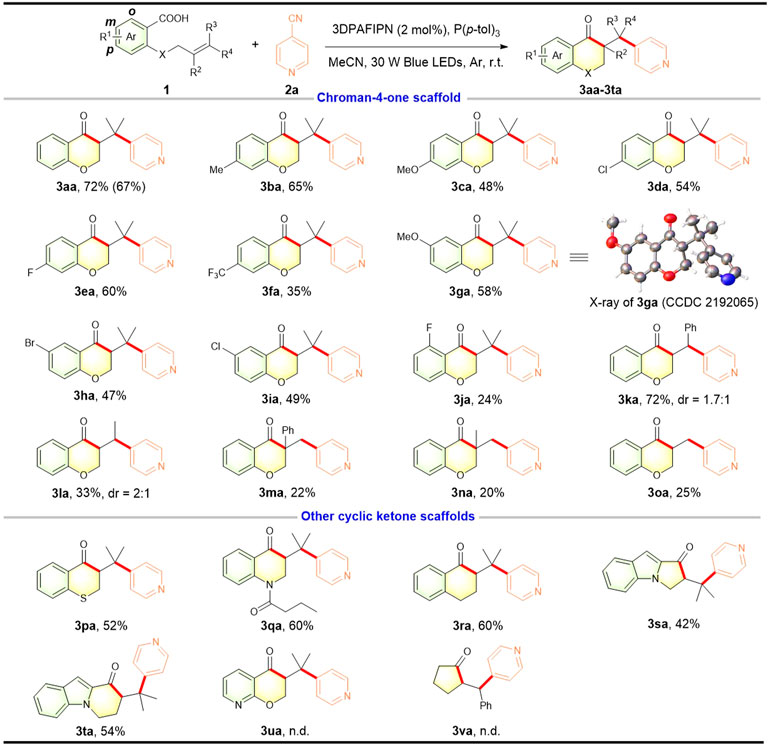
FIGURE 2. Scope of alkenoic acids. Reaction conditions: 1 (0.3 mmol), 2a (0.45 mmol), 3DPAFIPN (2 mol%), P (p-tol)3 (0.6 mmol), MeCN (6 ml), 30 W blue LEDs, argon atmosphere, r.t., 24 h. The isolated yield is based on 1. Isolated yield in parentheses is obtained on a 1.0 mmol scale.
To further explore the synthetic potential of our methodology, we then investigated differently substituted cyanoarene partners in this photocatalytic acylarylation (Figure 3). Firstly, the substituted phenyl and alkyl group at the 2-position of cyanopyridine were well tolerated, providing the corresponding 3-(pyridylmethyl)chroman-4-ones (3ab–3af) in moderate to good yields. Cyanopyridines bearing halogen substituents at 2- or 3-position afforded the desired products albeit in decreased yields (3ag–3ai), offering opportunities for further derivatization. The structure of 3ah was confirmed by X-ray diffraction analysis (CCDC 2192094). Notably, using 2, 4-dicyanopyridine as a coupling partner underwent selective coupling at the most electron-poor 4-position to produce the corresponding chroman-4-one 3aj in a synthetically useful yield along with C2-coupled chroman-4-one 3aj′. Additionally, non-pyridine cyanoarenes including quinoline and isoquinoline scaffolds were also successful with the standard conditions, leading to the formation of the corresponding chroman-4-ones 3ak and 3al with 49% and 48% yields, respectively. To our delight, other electron-withdrawing cyanoarene 1, 4-dicyanobenzene was also compatible with our protocol to give a satisfactory yield of 3-benzylchroman-4-one 3cm (belonging to classical homoisoflavonoid skeleton), while 1,2-dicyanobenzene 2n and ethyl 4-cyanobenzoate 2o were not suitable.
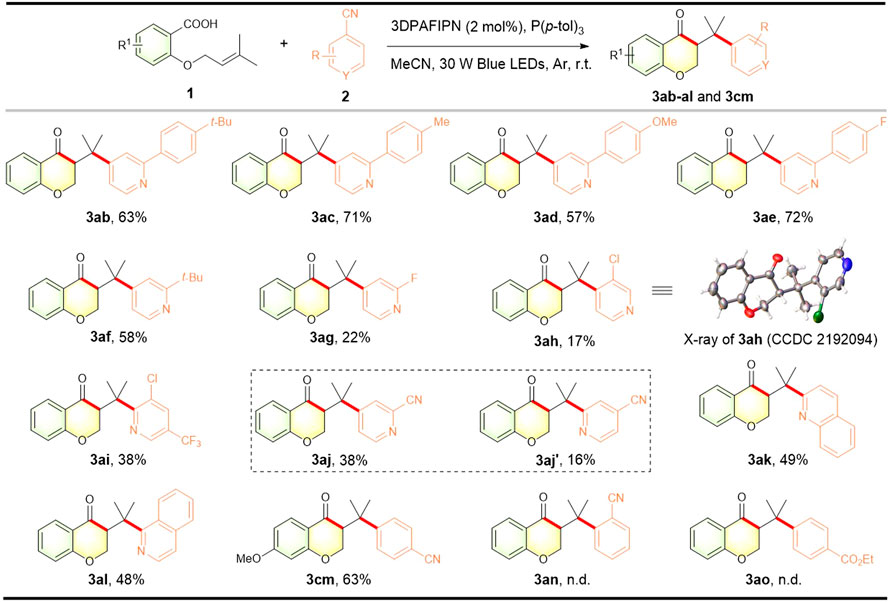
FIGURE 3. Scope of cyano (hetero)arenes. Reaction conditions: 1 (0.3 mmol), 2 (0.45 mmol), 3DPAFIPN (2 mol%), P (p-tol)3 (0.6 mmol), MeCN (6 ml), 30 W blue LEDs, argon atmosphere, r.t., 24 h. The isolated yield is based on 1.
To investigate the practical utility of this photocatalytic acylarylation process, several illustrative examples of simple derivatization of 3-(arylmethyl)chroman-4-ones were provided (Figure 4). For example, I2-mediated dehydrogenation of the resulting 3-(arylmethyl)chroman-4-ones (3aa and 3cm) proceeded well to provide the extensively studied and medicinally important chromones [4 (Zheng et al., 2015; Gobbi et al., 2016) and 5 (Kirkiacharian et al., 1989; Cavalli et al., 2005; Kirkiacharian and Gomis, 2005; Rao et al., 2008; Kupcewicz et al., 2013; Noshita et al., 2021)] with 86% and 75% yields, respectively. 3-Benzylchroman-4-one 3cm was treated with H2O2 in the presence of K2CO3 to obtain the corresponding amide 6 in 92% yield. Moreover, elaborated alkenyl triflate derived from 3cm could undergo Pd-mediated Suzuki coupling to afford biologically intriguing 3-benzyl 2H-chromene (Srikanth et al., 1997; Conti and Desideri, 2009) 7 in 80% yield. To our delight, the first example for the more challenging three-component alkene acylarylation using simple and easily accessible feedstocks could be realized to afford β-pyridylated ketone 8 albeit in a relatively low yield (Figure 4B), which is complementary to the previously reported two-component synthesis of pyridyl-containing ketones (Zhu et al., 2020; Yang et al., 2021; Li et al., 2022a).
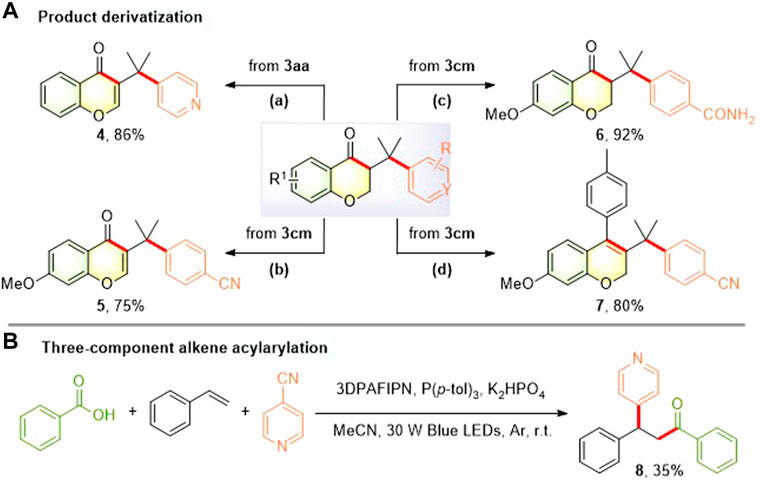
FIGURE 4. Product derivatization (A) and three-component alkene acylarylation (B). Reaction conditions: (a) I2, DMSO, reflux 2 h (b) I2, DMSO, reflux 2 h (c) 30% H2O2, K2CO3, DMSO, 0°C to r.t., 24 h (d) 2,6-di-tert-butylpyridine, Tf2O, CH2Cl2, 0°C to r.t., 5 h; Pd(PPh3)4, p-tolylboronic acid, DIPEA, NMP, 170°C, 10 min.
To elucidate the mechanism of this photocatalytic acylarylation, several control experiments using substrates 1a and 2a were carried out as shown in Figure 5A. When three equivalents of the radical scavenger TEMPO or the electron-transfer scavenger p-dinitrobenzene (DNB) were added under standard conditions, no product 3aa was observed and the corresponding TEMPO-adduct (TEMPO-1a) was detected by ESI-HRMS analysis. Additionally, when the model reaction was performed with an external radical-trapping reagent 1,1-diphenylethylene (DPE), the formation of the desired chroman-4-one 3aa was significantly inhibited and the corresponding radical-trapping product 3aa′ was also detected by ESI-HRMS analysis. Taken together, these results indicate that a radical/SET-based pathway might be involved in our photocatalytic acylarylation.
Based on the above experimental results and previous reports (Jiang et al., 2019; Hu et al., 2020a; Clarke et al., 2020; Nicastri et al., 2020; Pan et al., 2020; Rossi-Ashton et al., 2020; Shao et al., 2020; Zhou et al., 2020; Tong et al., 2021), a plausible mechanistic pathway for this photocatalytic acylarylation is proposed as described in Figure 5B. Under the blue LED irradiation, the photocatalyst 3DPAFIPN was initially raised to the excited state *3DPAFIPN, which was reductively quenched by P (p-tol)3 to form the strongly reducing 3DPAFIPN•− and phosphine radical cation. Subsequently, the phosphine radical cation recombined with the carboxylate anion of 1a to produce the phosphoranyl radical intermediate A, which underwent a facile β-scission to form acyl radical B and tri-p-tolylphosphine oxide. Then, the resulting radical B proceeded via intramolecular 6-exo-trig cyclization with the alkene moiety to provide alkyl radical C. Meanwhile, SET between the reduced 3DPAFIPN•− and 2a⋅H+ gave a persistent arene radical D and regenerated 3DPAFIPN. Finally, the alkyl radical C underwent intermolecular radical-radical coupling with radical D and sequential rearomatization via the elimination of both cyano anion and proton to achieve the corresponding chroman-4-one 3aa.
Conclusion
In summary, we have developed a novel visible-light-driven photoredox-neutral alkene acylarylation with cyanoarenes, enabling metal-, oxidant-, and aldehyde-free access to structurally diverse 3-(arylmethyl)chroman-4-ones (i.e., homoisoflavonoids) as well as other cyclic ketone analogs such as thiochroman-4-one, dihydroquinolin-4(1H)-one, dihydronaphthalen-1(2H)-one, pyrrolo [1,2-a]indol-1-one, and pyrido [1,2-a]indol-9(6H)-one. Furthermore, the resulting chroman-4-ones can be scale-up synthesized and also readily parlayed into skeletally diverse and valuable compounds such as chromone and 2H-chromene. In addition, the developed powerful protocol involves phosphoranyl radical-mediated acyl radical-initiated cascade cyclization followed by radical-radical coupling with the persistent aryl radical, enabling the concomitant introduction of ketone and aromatic fragments to organic molecules.
Data availability statement
The datasets presented in this study can be found in online repositories. The names of the repository/repositories and accession number(s) can be found below: https://www.ccdc.cam.ac.uk/structures/- CCDC 2192065.
Author contributions
QW, DY, and JW conceived and designed the study, and wrote the paper. YD, SY, MR, JL, QF, ZZ, and JB performed experiments and mechanistic studies. NH, LY, and SW analyzed the experiments. All authors contributed to the analysis and interpretation of the data.
Funding
This work was supported by the National Natural Science Foundation of China (22101237, 22171233), the Scientific Fund of Sichuan Province (2022NSFSC1219, 21YYJC0697), the research fund of Southwest Medical University (2021ZKMS042, 2021ZKQN107, 2017-ZRQN-159), and the Opening Project of Key Laboratory of Drug-Targeting and Drug Delivery System of the Education Ministry.
Conflict of interest
The authors declare that the research was conducted in the absence of any commercial or financial relationships that could be construed as a potential conflict of interest.
Publisher’s note
All claims expressed in this article are solely those of the authors and do not necessarily represent those of their affiliated organizations, or those of the publisher, the editors and the reviewers. Any product that may be evaluated in this article, or claim that may be made by its manufacturer, is not guaranteed or endorsed by the publisher.
Supplementary material
The Supplementary Material for this article can be found online at: https://www.frontiersin.org/articles/10.3389/fchem.2022.1059792/full#supplementary-material
References
Albrecht, U., Lalk, M., and Langer, P. (2005). Synthesis and structure-activity relationships of 2-vinylchroman-4-ones as potent antibiotic agents. Bioorg. Med. Chem. 13, 1531–1536. doi:10.1016/j.bmc.2004.12.031
Basavarajappa, H. D., Lee, B., Lee, H., Sulaiman, R. S., An, H., Magana, C., et al. (2015). Synthesis and biological evaluation of novel homoisoflavonoids for retinal neovascularization. J. Med. Chem. 58, 5015–5027. doi:10.1021/acs.jmedchem.5b00449
Betori, R. C., and Scheidt, K. A. (2019). Reductive arylation of arylidene malonates using photoredox catalysis. ACS Catal. 9, 10350–10357. doi:10.1021/acscatal.9b03608
Cavalli, A., Bisi, A., Bertucci, C., Rosini, C., Paluszcak, A., Gobbi, S., et al. (2005). Enantioselective nonsteroidal aromatase inhibitors identified through a multidisciplinary medicinal chemistry approach. J. Med. Chem. 48, 7282–7289. doi:10.1021/jm058042r
Chan, A. Y., Perry, I. B., Bissonnette, N. B., Buksh, B. F., Edwards, G. A., Frye, L. I., et al. (2022). Metallaphotoredox: The merger of photoredox and transition metal catalysis. Chem. Rev. 122, 1485–1542. doi:10.1021/acs.chemrev.1c00383
Clarke, A. K., Parkin, A., Taylor, R. J. K., Unsworth, W. P., and Rossi-Ashton, J. A. (2020). Photocatalytic deoxygenation of sulfoxides using visible light: Mechanistic investigations and synthetic applications. ACS Catal. 10, 5814–5820. doi:10.1021/acscatal.0c00690
Conti, C., and Desideri, N. (2009). Synthesis and antirhinovirus activity of new 3-benzyl chromene and chroman derivatives. Bioorg. Med. Chem. 17, 3720–3727. doi:10.1016/j.bmc.2009.03.051
Das, S., Parida, S. K., Mandal, T., Sing, L., De Sarkar, S., and Murarka, S. (2020). Organophotoredox-catalyzed cascade radical annulation of 2-(allyloxy)arylaldehydes with N-(acyloxy)phthalimides: Towards alkylated chroman-4-one derivatives. Chem. Asian J. 15, 568–572. doi:10.1002/asia.201901735
Desideri, N., Olivieri, S., Stein, M., Sgro, R., Orsi, N., and Conti, C. (1997). Synthesis and anti-picornavirus activity of homo-isoflavonoids. Antivir. Chem. Chemother. 8, 545–555. doi:10.1177/095632029700800609
Diana, E. J., Kanchana, U. S., and Mathew, T. V. (2021). Current developments in the synthesis of 4-chromanone-derived compounds. Org. Biomol. Chem. 19, 7995–8008. doi:10.1039/d1ob01352a
Eggler, J. F., Marfat, A., and Melvin, L. S. (1991). Substituted tetralins, chromans and related compounds in the treatment of asthma, arthritis and related diseases. Washington, DC, U.S: Patent and Trademark Office. U.S. Patent NO 5,059,609.
Eggler, J. F., Marfat, A., and Melvin, L. S. (1997). Tetralin and chroman derivatives useful in the treatment of asthma, arthritis and related diseases. Washington, DC, U.S: Patent and Trademark Office. U.S. Patent NO 5,698,550.
Emami, S., and Ghanbarimasir, Z. (2015). Recent advances of chroman-4-one derivatives: Synthetic approaches and bioactivities. Eur. J. Med. Chem. 93, 539–563. doi:10.1016/j.ejmech.2015.02.048
Friden-Saxin, M., Seifert, T., Landergren, M. R., Suuronen, T., Lahtela-Kakkonen, M., Jarho, E. M., et al. (2012). Synthesis and evaluation of substituted chroman-4-one and chromone derivatives as sirtuin 2-selective inhibitors. J. Med. Chem. 55, 7104–7113. doi:10.1021/jm3005288
Georgiou, E., Spinnato, D., Chen, K., Melchiorre, P., and Muniz, K. (2022). Switchable photocatalysis for the chemodivergent benzylation of 4-cyanopyridines. Chem. Sci. 13, 8060–8064. doi:10.1039/d2sc02698h
Gobbi, S., Hu, Q., Zimmer, C., Engel, M., Belluti, F., Rampa, A., et al. (2016). Exploiting the chromone scaffold for the development of inhibitors of corticosteroid biosynthesis. J. Med. Chem. 59, 2468–2477. doi:10.1021/acs.jmedchem.5b01609
Guo, Y. Q., Wang, R., Song, H., Liu, Y., and Wang, Q. (2020). Visible-light-Induced deoxygenation/defluorination protocol for synthesis of γ, γ-difluoroallylic ketones. Org. Lett. 22, 709–713. doi:10.1021/acs.orglett.9b04504
Han, Q.-Q., Li, G.-H., Sun, Y.-Y., Chen, D.-M., Wang, Z.-L., Yu, X.-Y., et al. (2020). Silver-catalyzed cascade radical cyclization of sodium sulfinates and o-(allyloxy)arylaldehydes towards functionalized chroman-4-ones. Tetrahedron Lett. 61, 151704–151708. doi:10.1016/j.tetlet.2020.151704
He, X. K., Cai, B. G., Yang, Q. Q., Wang, L., and Xuan, J. (2019). Visible-light-promoted cascade radical cyclization: Synthesis of 1, 4-diketones containing chroman-4-one skeletons. Chem. Asian J. 14, 3269–3273. doi:10.1002/asia.201901078
Hu, H., Chen, X., Sun, K., Wang, J., Liu, Y., Liu, H., et al. (2018b). Silver-catalyzed decarboxylative cascade radical cyclization of tert-carboxylic acids and o-(allyloxy)arylaldehydes towards chroman-4-one derivatives. Org. Chem. Front. 5, 2925–2929. doi:10.1039/c8qo00882e
Hu, H., Chen, X., Sun, K., Wang, J., Liu, Y., Liu, H., et al. (2018a). Silver-catalyzed radical cascade cyclization toward 1, 5-/1, 3-dicarbonyl heterocycles: An atom-/step-economical strategy leading to chromenopyridines and isoxazole-/pyrazole-containing chroman-4-ones. Org. Lett. 20, 6157–6160. doi:10.1021/acs.orglett.8b02627
Hu, X.-Q., Hou, Y.-X., Liu, Z.-K., and Gao, Y. (2020a). Recent advances in phosphoranyl radical-mediated deoxygenative functionalisation. Org. Chem. Front. 7, 2319–2324. doi:10.1039/d0qo00643b
Hu, X. Q., Liu, Z. K., Hou, Y. X., and Gao, Y. (2020b). Single electron activation of aryl carboxylic acids. iScience 23, 101266–101291. doi:10.1016/j.isci.2020.101266
Huang, H. L., Du, J. Y., Li, Q. L., Gao, F., and Ma, C. L. (2020). Visible-light-promoted cascade radical cyclization: Synthesis of chroman-4-ones and dihydroquinolin-4-ones. J. Org. Chem. 85, 3963–3972. doi:10.1021/acs.joc.9b03253
Jiang, H., Mao, G., Wu, H., An, Q., Zuo, M., Guo, W., et al. (2019). Synthesis of dibenzocycloketones by acyl radical cyclization from aromatic carboxylic acids using methylene blue as a photocatalyst. Green Chem. 21, 5368–5373. doi:10.1039/c9gc02380a
Jung, S., Kim, J., and Hong, S. (2017). Visible light-promoted synthesis of spiroepoxy chromanone derivatives via a tandem oxidation/radical cyclization/epoxidation process. Adv. Synth. Catal. 359, 3945–3949. doi:10.1002/adsc.201701072
Kirkiacharian, B. S., and Gomis, M. (2005). New convenient synthesis of homoisoflavanones and (±)-di-o-methyldihydroeucomin. Synth. Commun. 35, 563–569. doi:10.1081/scc-200049789
Kirkiacharian, S., Tongo, H. G., Bastide, J., Bastide, P., and Grenie, M. M. (1989). Synthe`se et activite´s angioprotectrice, anti-allergique et antihistaminique de benzyl-3 chromones (homo-isoflavones). Eur. J. Med. Chem. 24, 541–546. doi:10.1016/0223-5234(89)90060-3
Kitcatt, D. M., Nicolle, S., and Lee, A. L. (2022). Direct decarboxylative giese reactions. Chem. Soc. Rev. 51, 1415–1453. doi:10.1039/d1cs01168e
Kumar, D., Sharma, P., Singh, H., Nepali, K., Gupta, G. K., Jain, S. K., et al. (2017). The value of pyrans as anticancer scaffolds in medicinal chemistry. RSC Adv. 7, 36977–36999. doi:10.1039/c7ra05441f
Kupcewicz, B., Balcerowska-Czerniak, G., Malecka, M., Paneth, P., Krajewska, U., and Rozalski, M. (2013). Structure-cytotoxic activity relationship of 3-arylideneflavanone and chromanone (e, z isomers) and 3-arylflavones. Bioorg. Med. Chem. Lett. 23, 4102–4106. doi:10.1016/j.bmcl.2013.05.044
Lee, B., Basavarajappa, H. D., Sulaiman, R. S., Fei, X., Seo, S. Y., and Corson, T. W. (2014). The first synthesis of the antiangiogenic homoisoflavanone, cremastranone. Org. Biomol. Chem. 12, 7673–7677. doi:10.1039/c4ob01604a
Li, Y., Han, C., Wang, Y., Huang, X., Zhao, X., Qiao, B., et al. (2022a). Catalytic asymmetric reductive azaarylation of olefins via enantioselective radical coupling. J. Am. Chem. Soc. 144, 7805–7814. doi:10.1021/jacs.2c01458
Li, Y., Shao, Q., He, H., Zhu, C., Xue, X. S., and Xie, J. (2022b). Highly selective synthesis of all-carbon tetrasubstituted alkenes by deoxygenative alkenylation of carboxylic acids. Nat. Commun. 13, 10–17. doi:10.1038/s41467-021-27507-x
Liu, Q., Lu, W., Xie, G., and Wang, X. (2020). Metal-free synthesis of phosphinoylchroman-4-ones via a radical phosphinoylation-cyclization cascade mediated by K2S2O8. Beilstein J. Org. Chem. 16, 1974–1982. doi:10.3762/bjoc.16.164
Liu, Q., Xie, G., Wang, Q., Mo, Z., Li, C., Ding, S., et al. (2019). Synthesis of chroman-4-one and indanone derivatives via silver catalyzed radical ring opening/coupling/cyclization cascade. Tetrahedron 75, 130490–130498. doi:10.1016/j.tet.2019.130490
Liu, Y. C., Chen, P., Li, X. J., Xiong, B. Q., Liu, Y., Tang, K. W., et al. (2022). Visible-light-induced dual acylation of alkenes for the construction of 3-substituted chroman-4-ones. J. Org. Chem. 87, 4263–4272. doi:10.1021/acs.joc.1c03100
Lu, D., Wan, Y., Kong, L., and Zhu, G. (2017). Visible-light-induced tandem radical addition-cyclization of alkenyl aldehydes leading to indanones and related compounds. Org. Lett. 19, 2929–2932. doi:10.1021/acs.orglett.7b01162
Mandal, S., Bera, T., Dubey, G., Saha, J., and Laha, J. K. (2018). Uses of K2S2O8 in metal-catalyzed and metal-free oxidative transformations. ACS Catal. 8, 5085–5144. doi:10.1021/acscatal.8b00743
Martinez Alvarado, J. I., Ertel, A. B., Stegner, A., Stache, E. E., and Doyle, A. G. (2019). Direct use of carboxylic acids in the photocatalytic hydroacylation of styrenes to generate dialkyl ketones. Org. Lett. 21, 9940–9944. doi:10.1021/acs.orglett.9b03871
Mayuri, B., Kavitha, P., Basavoju, S., Bhargavi, G., and Reddy, K. L. (2017). Synthesis, structural characterisation and biological evolution of chromanones. J. Mol. Struct. 1145, 1–9. doi:10.1016/j.molstruc.2017.05.013
Mei, Y., Zhao, L., Liu, Q., Ruan, S., Wang, L., and Li, P. (2020). Synthesis of sulfone-functionalized chroman-4-ones and chromans through visible-light-induced cascade radical cyclization under transition-metal-free conditions. Green Chem. 22, 2270–2278. doi:10.1039/d0gc00009d
Merkens, K., Aguilar Troyano, F. J., Anwar, K., and Gomez-Suarez, A. (2021). Synthesis of gamma-oxo-alpha-amino acids via radical acylation with carboxylic acids. J. Org. Chem. 86, 8448–8456. doi:10.1021/acs.joc.0c02951
Nibbs, A. E., and Scheidt, K. A. (2011). Asymmetric methods for the synthesis of flavanones, chromanones, and azaflavanones. Eur. J. Org. Chem. 2012, 449–462. doi:10.1002/ejoc.201101228
Nicastri, M. C., Lehnherr, D., Lam, Y. H., DiRocco, D. A., and Rovis, T. (2020). Synthesis of sterically hindered primary amines by concurrent tandem photoredox catalysis. J. Am. Chem. Soc. 142, 987–998. doi:10.1021/jacs.9b10871
Norman, A. R., Yousif, M. N., and McErlean, C. S. P. (2018). Photoredox-catalyzed indirect acyl radical generation from thioesters. Org. Chem. Front. 5, 3267–3298. doi:10.1039/c8qo00867a
Noshita, T., Fujita, K., Koga, T., Ouchi, H., and Tai, A. (2021). Synthesis and biological activity of (±)-7, 3′, 4′-trihydroxyhomoisoflavan and its analogs. Bioorg. Med. Chem. Lett. 31, 127674–127677. doi:10.1016/j.bmcl.2020.127674
Pan, D., Nie, G., Jiang, S., Li, T., and Jin, Z. (2020). Radical reactions promoted by trivalent tertiary phosphines. Org. Chem. Front. 7, 2349–2371. doi:10.1039/d0qo00473a
Rao, V. M., Damu, G. L. V., Sudhakar, D., Siddaiah, V., and Rao, C. V. (2008). New efficient synthesis and bioactivity of homoisoflavonoids. ARKIVOC 2008, 285–294. doi:10.3998/ark.5550190.0009.b28
Rossi-Ashton, J. A., Clarke, A. K., Unsworth, W. P., and Taylor, R. J. K. (2020). Phosphoranyl radical fragmentation reactions driven by photoredox catalysis. ACS Catal. 10, 7250–7261. doi:10.1021/acscatal.0c01923
Seifert, T., Malo, M., Kokkola, T., Engen, K., Friden-Saxin, M., Wallen, E. A., et al. (2014). Chroman-4-one- and chromone-based sirtuin 2 inhibitors with antiproliferative properties in cancer cells. J. Med. Chem. 57, 9870–9888. doi:10.1021/jm500930h
Shao, X., Zheng, Y., Ramadoss, V., Tian, L., and Wang, Y. (2020). Recent advances in P(III)-assisted deoxygenative reactions under photochemical or electrochemical conditions. Org. Biomol. Chem. 18, 5994–6005. doi:10.1039/d0ob01083a
Shen, J., Zhang, Y., Yu, Y., and Wang, M. (2021). Metal-free visible-light-induced photoredox-catalyzed intermolecular pyridylation/phosphinoylation of alkenes. Org. Chem. Front. 8, 901–907. doi:10.1039/d0qo01218a
Sheng, J., Liu, J., Chen, L., Zhang, L., Zheng, L., and Wei, X. (2019). Silver-catalyzed cascade radical cyclization of 2-(allyloxy)arylaldehydes with cyclopropanols: Access to chroman-4-one derivatives. Org. Chem. Front. 6, 1471–1475. doi:10.1039/c9qo00292h
Speckmeier, E., Fischer, T. G., and Zeitler, K. (2018). A toolbox approach to construct broadly applicable metal-free catalysts for photoredox chemistry: Deliberate tuning of redox potentials and importance of halogens in donor-acceptor cyanoarenes. J. Am. Chem. Soc. 140, 15353–15365. doi:10.1021/jacs.8b08933
Srikanth, N., Ng, S.-C., Sim, K.-Y., and Kon, O.-L. (1997). Synthesis of 3-(p-Halobenzyl)-4-aryl-2H-chromenes as selective ligands for antiestrogen-binding sites. J. Chem. Res. (S) 1997, 202–203. doi:10.1039/A700565B
Stache, E. E., Ertel, A. B., Tomislav, R., and Doyle, A. G. (2018). Generation of phosphoranyl radicals via photoredox catalysis enables voltage-independent activation of strong C-O bonds. ACS Catal. 8, 11134–11139. doi:10.1021/acscatal.8b03592
Tait, S., Salvati, A. L., Desideri, N., and Fiore, L. (2006). Antiviral activity of substituted homoisoflavonoids on enteroviruses. Antivir. Res. 72, 252–255. doi:10.1016/j.antiviral.2006.07.003
Tong, S., Li, K., Ouyang, X., Song, R., and Li, J. (2021). Recent advances in the radical-mediated decyanative alkylation of cyano(hetero)arene. Green Synth. Catal. 2, 145–155. doi:10.1016/j.gresc.2021.04.003
Vorob’ev, A. Y. (2019). Photocatalytic reaction of 4-cyanopyridine with tertiary amines. Chem. Heterocycl. Compd. (N. Y). 55, 90–92. doi:10.1007/s10593-019-02423-7
Wang, X., Han, Y. F., Ouyang, X. H., Song, R. J., and Li, J. H. (2019). The photoredox alkylarylation of styrenes with alkyl N-hydroxyphthalimide esters and arenes involving C-H functionalization. Chem. Commun. (Camb.) 55, 14637–14640. doi:10.1039/c9cc07494e
Xiao, Y.-M., Liu, Y., Mai, W.-P., Mao, P., Yuan, J.-W., and Yang, L.-R. (2019). A novel and facile synthesis of chroman-4-one derivatives via cascade radical cyclization under metal-free condition. ChemistrySelect 4, 1939–1942. doi:10.1002/slct.201900147
Xiong, L., Hu, H., Wei, C.-W., and Yu, B. (2020). Radical reactions for the synthesis of 3-substituted chroman-4-ones. Eur. J. Org. Chem. 2020, 1588–1597. doi:10.1002/ejoc.201901581
Yan, Z., Sun, B., Huang, P., Zhao, H., Ding, H., Su, W., et al. (2022). Visible-light-promoted radical alkylation/cyclization of allylic amide with N-hydroxyphthalimide ester: Synthesis of oxazolines. Chin. Chem. Lett. 33, 1997–2000. doi:10.1016/j.cclet.2021.09.067
Yang, J., Ma, J., Yan, K., Tian, L., Li, B., and Wen, J. (2021). Electrochemical ammonium cation-assisted hydropyridylation of ketone-activated alkenes: Experimental and computational mechanistic studies. Adv. Synth. Catal. 364, 845–854. doi:10.1002/adsc.202101361
Yang, W.-C., Dai, P., Luo, K., Ji, Y.-G., and Wu, L. (2017). Aldehydes as carbon radical acceptors: Silver nitrate catalyzed cascade decarboxylation and oxidative cyclization toward dihydroflavonoid derivatives. Adv. Synth. Catal. 359, 2390–2395. doi:10.1002/adsc.201601407
Zhang, M., Ruzi, R., Xi, J., Li, N., Wu, Z., Li, W., et al. (2017). Photoredox-catalyzed hydroacylation of olefins employing carboxylic acids and hydrosilanes. Org. Lett. 19, 3430–3433. doi:10.1021/acs.orglett.7b01391
Zhang, M., Xie, J., and Zhu, C. (2018). A general deoxygenation approach for synthesis of ketones from aromatic carboxylic acids and alkenes. Nat. Commun. 9, 3517–3526. doi:10.1038/s41467-018-06019-1
Zhao, J., Li, P., Li, X., Xia, C., and Li, F. (2016). Straightforward synthesis of functionalized chroman-4-ones through cascade radical cyclization-coupling of 2-(allyloxy)arylaldehydes. Chem. Commun. (Camb.) 52, 3661–3664. doi:10.1039/c5cc09730d
Zheng, G., Zhang, Z., Kang, B., Yu, R., Cao, Y., and Zhang, S. (2015). Synthesis and vasodilatation of homoisoflavones. Chin. J. Org. Chem. 35, 1112–1122. doi:10.6023/cjoc201411033
Zhong, L.-J., Wang, H.-Y., Ouyang, X.-H., Li, J.-H., and An, D.-L. (2020). Benzylic C–H heteroarylation of N-(benzyloxy)phthalimides with cyanopyridines enabled by photoredox 1, 2-hydrogen atom transfer. Chem. Commun. 56, 8671–8674. doi:10.1039/d0cc03619f
Zhou, C., Lei, T., Wei, X.-Z., Ye, C., Liu, Z., Chen, B., et al. (2020). Metal-free, redox-neutral, site-selective access to heteroarylamine via direct radical-radical cross-coupling powered by visible light photocatalysis. J. Am. Chem. Soc. 142, 16805–16813. doi:10.1021/jacs.0c07600
Zhou, J., Huang, M., Liang, Y., and Wan, Y. (2021). A synergetic organoselenium catalytic system for constructing 4-chromanone derivatives via a tandem process under visible light radiation. ChemistrySelect 6, 5610–5613. doi:10.1002/slct.202101638
Zhou, N., Wu, M., Zhang, M., and Zhou, X. (2019a). Visible-light-induced difluoroacetylation of o-(allyloxy)aryl-aldehydes: Access to difluoroacetylated chroman-4-ones. Asian J. Org. Chem. 8, 828–831. doi:10.1002/ajoc.201900121
Zhou, Y., Xiong, Z., Qiu, J., Kong, L., and Zhu, G. (2019b). Visible light photocatalytic acyldifluoroalkylation of unactivated alkenes for the direct synthesis of gem-difluorinated ketones. Org. Chem. Front. 6, 1022–1026. doi:10.1039/c9qo00136k
Zhu, H. L., Zeng, F. L., Chen, X. L., Sun, K., Li, H. C., Yuan, X. Y., et al. (2021). Acyl radicals from alpha-keto acids: Metal-free visible-light-promoted acylation of heterocycles. Org. Lett. 23, 2976–2980. doi:10.1021/acs.orglett.1c00655
Keywords: chroman-4-one, 3-(arylmethyl)chroman-4-ones, phosphoranyl radical, acyl radical, radical-radical coupling
Citation: Ding Y, Yu S, Ren M, Lu J, Fu Q, Zhang Z, Wang Q, Bai J, Hao N, Yang L, Wei S, Yi D and Wei J (2022) Redox-neutral and metal-free synthesis of 3-(arylmethyl)chroman-4-ones via visible-light-driven alkene acylarylation. Front. Chem. 10:1059792. doi: 10.3389/fchem.2022.1059792
Received: 02 October 2022; Accepted: 18 October 2022;
Published: 31 October 2022.
Edited by:
Gu Zhan, Chengdu University of Traditional Chinese Medicine, ChinaReviewed by:
Xiaoming Chen, Lanzhou University, ChinaXuan-Hui Ouyang, Nanchang Hangkong University, China
Guang-Xun Li, Chengdu Institute of Biology, Chinese Academy of Sciences (CAS), China
Copyright © 2022 Ding, Yu, Ren, Lu, Fu, Zhang, Wang, Bai, Hao, Yang, Wei, Yi and Wei. This is an open-access article distributed under the terms of the Creative Commons Attribution License (CC BY). The use, distribution or reproduction in other forums is permitted, provided the original author(s) and the copyright owner(s) are credited and that the original publication in this journal is cited, in accordance with accepted academic practice. No use, distribution or reproduction is permitted which does not comply with these terms.
*Correspondence: Dong Yi, eWlkb25nQHN3bXUuZWR1LmNu; Jun Wei, d2VpanVuQHN3bXUuZWR1LmNu; Qin Wang, d3FfcmluZ0Bob3RtYWlsLmNvbQ==
†These authors have contributed equally to this work