- 1Central Nervous System Drug Key Laboratory of Sichuan Province, Department of Medicinal Chemistry, School of Pharmacy, Southwest Medical University, Luzhou, China
- 2Natural Products Research Center, Chengdu Institution of Biology, Chinese Academy of Science, Chengdu, Sichuan, China
- 3Department of Chemistry, School of Basic Medical Science, Southwest Medical University, Luzhou, China
A chemodivergent photocatalytic approach to 1-pyrrolines and 1-tetralones from alkyl bromides and vinyl azides has been developed through chemoselectively controllable intermolecular [3 + 2] and [4 + 2] cyclization. This photoredox-neutral two-component protocol involves intermolecular radical addition and switchable distal C(sp3)–H functionalization enabled by iminyl radical-mediated 1,5-hydrogen atom transfer. Meanwhile, chemoselectivity between C(sp3)–N bond formation and C(sp3)–C(sp2) bond formation is precisely switched by photocatalysts (Ru(bpy)3(PF6)2 vs. fac-Ir(ppy)3) and additives (base vs. acid).
Introduction
1-Pyrroline motifs are core structures of a plethora of biologically active molecules such as natural products and drugs. Joined to this, they could also act as versatile synthetic intermediates of various valuable molecules including pyrrolidine alkaloids (Tyroller et al., 2002; Pluotno and Carmeli, 2005; Newcomb et al., 2016), ligands (Lasri et al., 2012), pharmaceuticals (Rosser and Faulkner, 1984; Schann et al., 2001; Tsukamoto et al., 2001; Aicher et al., 2011), etc (Miltyk and Pałka, 2000; Stapon et al., 2003). With the rapid development of visible-light-driven photoredox catalysis, photocatalytic iminyl radical-mediated cyclization reactions have emerged as greener and milder approaches for the straightforward synthesis of 1-pyrroline frameworks. One of the novel synthetic methods is photocatalytic iminyl radical-mediated intramolecular cyclization/functionalization of C–C double bonds for rapid synthesis of diversely functionalized 1-pyrroline derivatives developed by the groups of Leonori (Davies et al., 2015), Itoh (Usami et al., 2018), and Studer (Jiang and Studer, 2017), our group (Zhang et al., 2021), and others (Chen et al., 2020; Wang et al., 2020; Krylov et al., 2021) (Scheme 1A, left). On the other hand, photocatalytic iminyl radical-mediated intramolecular 1,5-hydrogen atom transfer (HAT)/cyclization cascade has been exploited by Nevado group and Yu group as a valuable tool for distal C(sp3)–H bond functionalization to access 1-pyrrolines and related scaffolds (Scheme 1A, right) (Shu and Nevado, 2017; Wu et al., 2021). Despite the marked advances achieved, these two iminyl radical-mediated approaches to 1-pyrroline skeletons are based on the self-elaboration of single puzzling oxime derivative. Thus, the development of novel, straightforward, and efficient approaches to construct 1-pyrroline architectures via photocatalytic iminyl radical-mediated intermolecular cyclization, especially with two or more simple and readily available starting materials, is highly desirable but challenging.
Meanwhile, vinyl azides, as another extremely attractive iminyl radical source, could provide iminyl radicals through the radical addition followed by the release of an N2 molecule to trigger further cyclization for the facile construction of various hetero- and carbocycles (Shu et al., 2017; Tang et al., 2017; Lei et al., 2018; Li et al., 2018; Tang et al., 2019; Liao et al., 2020; Lin et al., 2020; Jiao et al., 2021; Liang et al., 2021). In 2021, our group developed an unprecedented trifunctionalizing ipso-spirocyclization of vinyl azides with unactivated alkenes as 1,2,n-tri-radical precursors to afford novel spiroaminal skeletons through photocatalytic nitrogen radical-triggered cyclization-trapping-translocation-cyclization cascade (Qi et al., 2021). Prompted by this work and seminal pioneering reports on the unparalleled functional diversity of vinyl azides (Fu et al., 2017), we envisioned whether readily available alkyl bromides could be used as 1,2-diradical precursors and react with iminyl radical source vinyl azides to undergo the photocatalytic intermolecular radical addition, iminyl radical-mediated intramolecular 1,5-HAT (Dong et al., 2022), and sequential radical cyclization cascade, thus providing access to highly valuable 1-pyrroline architectures. Herein, we report an example of photocatalytic iminyl radical-mediated intermolecular [3 + 2] cyclization from alkyl bromides and vinyl azides to access structurally intriguing and highly functionalized five-membered heterocycle 1-pyrrolines (Scheme 1B). Interestingly, a chemodivergent approach to pharmaceutically important 1-tetralones (Taber et al., 2004; Odagi et al., 2017; Legoabe et al., 2018) (six-membered carbocycles) via photocatalytic iminyl radical-mediated intermolecular [4 + 2] cyclization from the same starting materials could also be established (Miller and Sarpong, 2011; Zhan et al., 2017; Najera et al., 2019).
Results and discussion
To validate our hypothesis, diethyl 2-benzyl-2-bromomalonate 1a and vinyl azide 2a were firstly selected as model substrates to optimize the reaction conditions (Table 1). It was pleasing to find that when using fac-Ir(ppy)3 as the photocatalyst, K2CO3 as the base, and anhydrous CH2Cl2 as the solvent, the expected [3 + 2] cyclization product 1-pyrroline 3aa could be obtained in 12% yield as well as the [4 + 2] cyclization product 1-tetralone 4aa in relatively higher yield (entry 1). Subsequently, screening of photocatalysts demonstrated that replacing fac-Ir(ppy)3 with Ru(bpy)3(PF6)2 remarkably enhanced the reaction efficiency of [3 + 2] cyclization, whereas only a trace amount of the [4 + 2] cyclization product 4aa was detected (entries 2 and 3). After selecting Ru(bpy)3(PF6)2 as an optimal photocatalyst, we further explored the effect of solvents and additives. However, when other solvents such as DMF, THF, and MeCN were tested, it led to the expected product 3aa in the decreased yields of 13%, 46%, and 55%, respectively (entries 4–6). Furthermore, other tested bases delivered no more significant improvement, and notably, the yield of the desired product 3aa dropped sharply to 12% without the addition of a base (entries 7–10), indicating that a base is critical to the success of this [3 + 2] cyclization. Interestingly, the yield of the desired product 3aa could be further improved to 82% yield by adjusting the loading of reaction substrates (entries 11 and 12). As anticipated, control experiments indicated that visible light and photocatalyst fac-Ir(ppy)3 were indispensable for such photocatalytic [3 + 2] cyclization (entries 13 and 14). Delightfully, using AcOH instead of the essential base in the predominant [3 + 2] cyclization could not only enable the preferential [4 + 2] cyclization, but the yield of 1-tetralone 4aa could also be improved from 60% to 76% by further increasing the amount of solvent CH2Cl2 (entries 15 and 16 vs. entry 1).
Having established the optimal reaction conditions (Table 1, entry 12), we next explored the substrate scope of alkyl bromides and vinyl azides to extend the synthetic potential and generality of this method as summarized in Scheme 2. Firstly, the generality of this photocatalytic [3 + 2] cyclization with regard to alkyl bromides was examined. Generally, a diverse array of alkyl bromides bearing different electronic groups at various positions of the phenyl moiety could be successfully transformed into the desired 1-pyrroline products (3aa–3qa). Notably, the molecular structure of the representative 1-pyrroline 3ba was confirmed by X-ray crystallography (CCDC 2173209). Gratifyingly, this photocatalytic cyclization tolerated well a broad range of diverse functionalities such as alkyl (3ca, 3da, 3na, and 3qa), alkoxyl (3ba and 3ma), cyano (3ha), and acetyl (3ia), as well as halogen (3ea–3ga, 3ja–3la, and 3oa–3pa) that could provide opportunities for further product functionalization. Interestingly, the [3 + 2] cyclization reaction between indole-derived bromide 1r and vinyl azide 2a also proceeded smoothly to produce the corresponding 1-pyrroline 3ra with a satisfactory yield. Additionally, homoallylic and homopropargyl-substituted bromides have also been proven to be suitable substrates for this [3 + 2] cyclization, providing the desired highly substituted 1-pyrrolines (3sa and 3ta) with yields of 71% and 15%, while saturated alkyl bromides (1u and 1v) failed to give the desired products. Subsequently, we examined the scope of vinyl azides with 2-benzyl-2-bromomalonate 1a as the coupling partner. Different substituents on the aromatic ring of the vinyl azides were found compatible with the [3 + 2] cyclization conditions to obtain the desired products in moderate to good yields (3ab–3am). It is worth noting that the estrone-derived vinyl azide 2p effectively participated in such [3 + 2] cyclization to give the structurally intriguing 1-pyrroline derivative 3ap, demonstrating the capacity of this protocol in late-stage modification of complex bioactive molecules. However, alkyl-substituted vinyl azide seemed to be not suitable for this cyclization and provided the unseparated mixture including a trace amount of the expected product 3aq.
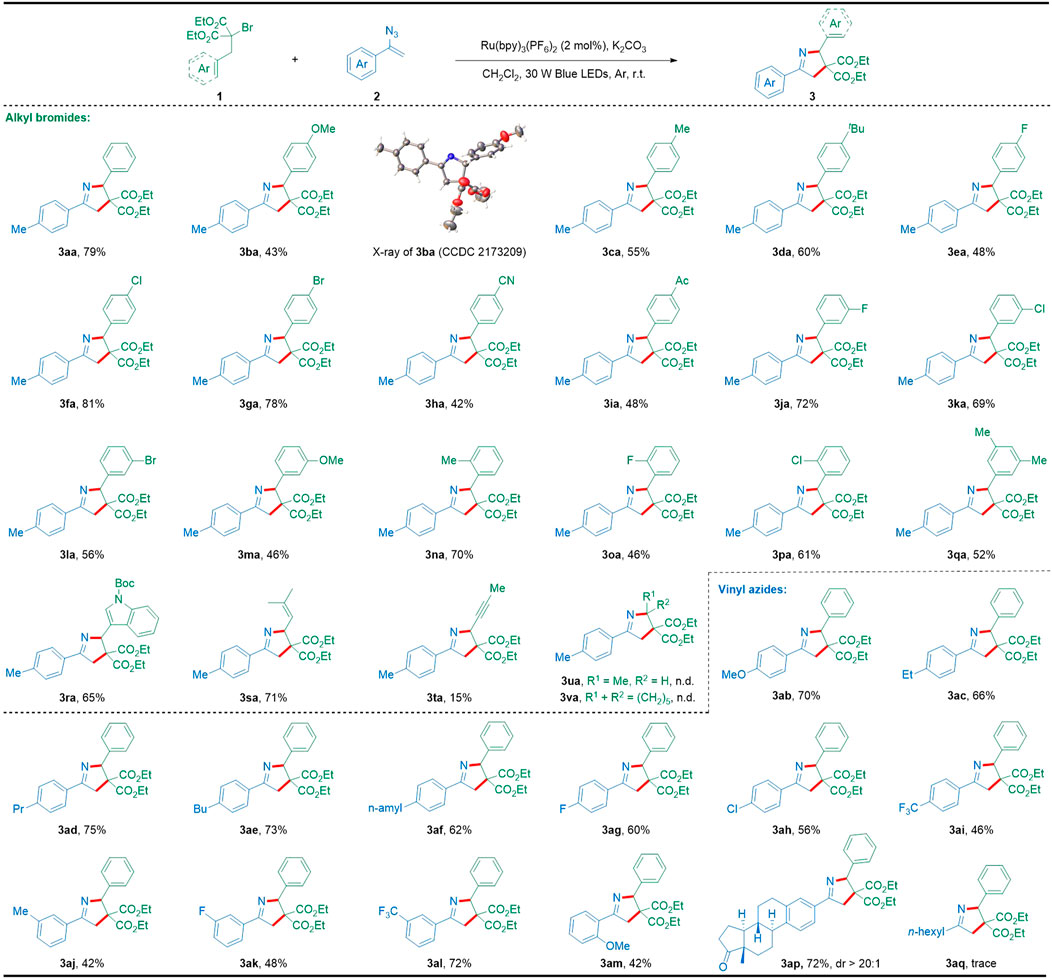
SCHEME 2. Substrate scope with respect to [3 + 2] cyclization. Reaction conditions: 1 (0.2 mmol), 2 (0.5 mmol), Ru(bpy)3(PF6)2 (2 mol%), K2CO3 (0.3 mmol), CH2Cl2 (2 ml), 30 W blue LEDs, argon atmosphere, r.t., 9 h, in a sealed tube; isolated yields based on 1 after the chromatographic purification.
After completing the [3 + 2] cyclization, we continued to expand the substrate scope to afford the [4 + 2] cyclization product 1-tetralones under the conditions of fac-Ir(ppy)3 as the photocatalyst and AcOH as the additive (Table 1, entry 16). As illustrated in Scheme 3, this [4 + 2] cyclization showed good tolerance of various substituents such as alkyl, halogen, cyano, acetyl, and alkoxyl, providing a great variety of highly functionalized 1-tetralones (4aa–4qa). In particular, the molecular structure of the representative 1-tetralone 4ga was also confirmed by X-ray crystallographic analysis (CCDC 2173208). On the other hand, a panel of vinyl azides with electron-donating or electron-withdrawing groups at the para-position of the aromatic ring could be successfully converted into the corresponding 1-tetralones (4ab–4ah) in satisfactory yields. However, vinyl azide with methyl at the meta-position of the aromatic ring furnished the corresponding 1-tetralones as mixtures of regio-isomer (4aj and 4aj′). In addition, the ortho-substituted vinyl azide reacted smoothly with 1a to give the corresponding 1-tetralone in an acceptable yield (4an). Furthermore, this photocatalytic [4 + 2] cyclization also displayed good tolerance of other important heteroaromatics including thiophene (4ao). However, during our preparation of this manuscript, Xu, Hu, and co-workers also reported an elegant radical cascade cyclization between vinyl azides and alkyl bromides toward 1-tetralone skeletons by using a metal-free photocatalyst (Jiao et al., 2021).
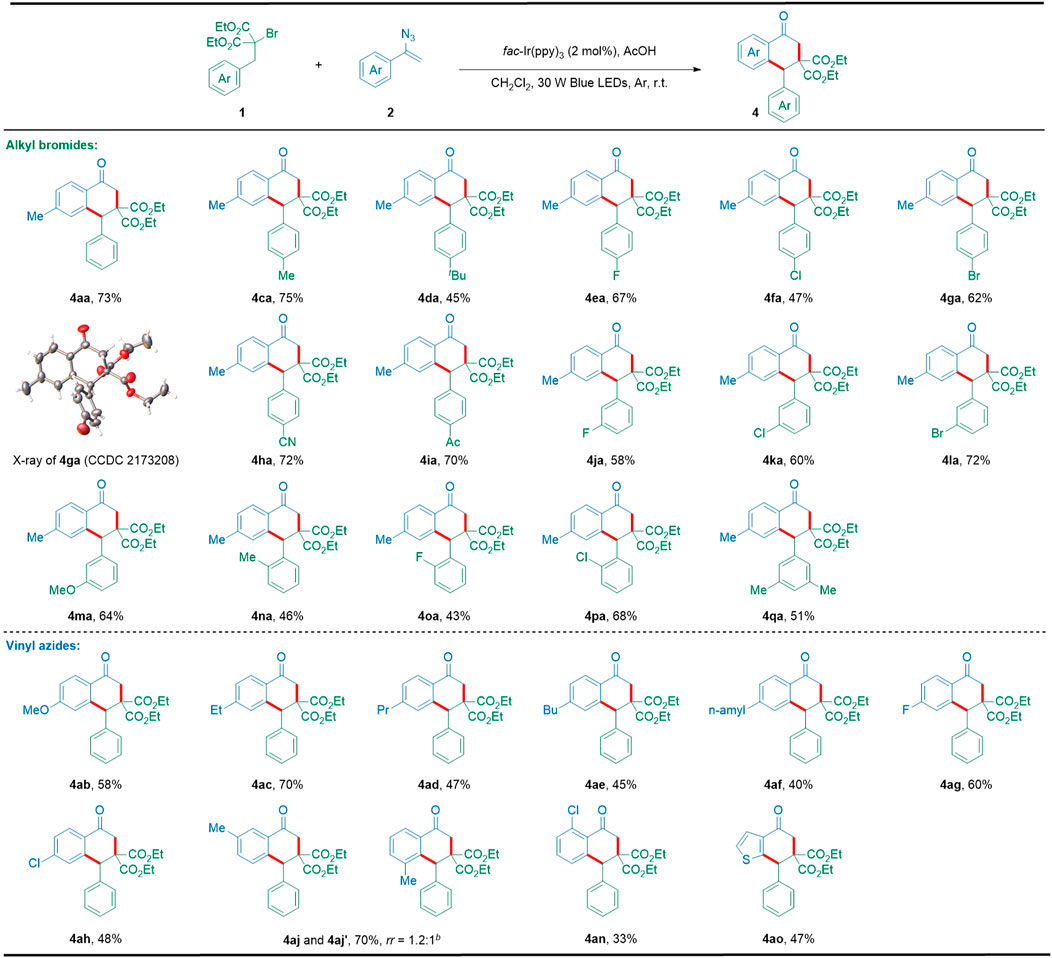
SCHEME 3. Substrate scope with respect to [4 + 2] cyclization. Reaction conditions: 1 (0.2 mmol), 2 (0.4 mmol), fac-Ir(ppy)3 (2 mol%), AcOH (0.2 mmol), CH2Cl2 (4 ml), 30 W blue LEDs, argon atmosphere, r.t., 9 h, in a sealed tube; isolated yields based on 1 after the chromatographic purification. rr: regioisomeric ratio.
To illustrate the potential application of this protocol, we performed a scale-up reaction containing 1.0 mmol of 1a under standard conditions to obtain the target product 3aa with a synthetically useful yield (Scheme 4A). To further showcase the synthetic utility of this protocol, we next performed a set of facile derivatization applications using the formed 1-pyrroline 3aa. In the presence of trifluoroacetic anhydride or acetyl chloride, 3aa could be readily transformed into the acylated 2-pyrroline derivatives (5 and 6) with 89% and 92% yields, respectively (Schemes 4B,C). Additionally, [3 + 2] cycloaddition of 3aa with N-hydroxybenzimidyl chloride successfully obtained biologically relevant pyrroline-fused 1,2,4-oxadiazoline 7 in an excellent yield of 90% (Scheme 4D). Upon treatment of 3aa with N-chlorosuccinimide (NCS) at 80 °C, the α,α-dichlorinated 1-pyrroline derivative 8 was isolated in very good yield (Scheme 4E). Interestingly, treatment of 3aa with LiCl in DMSO and trace H2O at 180°C resulted in the mono-decarboxylation, aromatization, and unclarified methylation process to afford tetra-substituted pyrrole 9 in moderate yield (Scheme 4F).
Subsequently, some control experiments were conducted to elucidate the reaction mechanism as shown in Scheme 5. When the electron-transfer scavenger p-dinitrobenzene (DNB) or radical scavenger including 2,6-di-tert-butyl-4-methylphenol (BHT), 2,2,6,6-tetramethyl-piperidinyloxyl (TEMPO), and 1,1-diphenylethylene (DPE) was added into the reaction system, the model reaction was suppressed to varying degrees (Scheme 5A). Notably, the existence of both 1a-derived adduct TEMPO-1a detected by LC-HRMS and 3aa′ isolated by the chromatographic purification indicated that the corresponding alkyl radical might be generated from 1a. Furthermore, a trace amount of other TEMPO-trapped adducts (TEMPO-C and TEMPO-C′) were also detected by LC-HRMS analysis, suggesting that the carbon-radical intermediates generated through the radical addition followed by iminyl radical-triggered 1,5-HAT might be involved in such transformation. Additionally, no desired product 3aa was observed when using 3-phenyl-2H-azidocyclopropane 2a′ generated under standard conditions instead of vinyl azide 2a, which seems to exclude the participation of 2H-aziridine intermediate in this transformation (Scheme 5B).
Based on the above-mentioned results and literature survey, the reaction mechanism of [3 + 2] cyclization is depicted in Scheme 6. MacMillan group (Nicewicz and MacMillan, 2008) and Stephenson group (Wallentin et al., 2012; Swift et al., 2016) disclosed that, under the irradiation of visible light, the weakly reducing excited-state *Ru2+ [E1/2red (Ru3+/*Ru2+) = −0.81 V vs. SCE] (Teegardin et al., 2016) can not be oxidatively quenched by the electron-deficient diethyl bromomalonate [E1/2red = −1.0 V vs. SCE (Wang et al., 2016); while our model substrate diethyl 2-benzyl-2-bromomalonate 1a (E1/2red = −1.27 V vs. SCE) see the Supplementary Material]. In contrast, single electron transfer from the photogenerated electron-rich Ru1+ (−1.33 V vs. SCE) to 1a could deliver an electrophilic alkyl radical A, although that the excited-state *Ru2+ [E1/2red (*Ru2+/Ru1+) = +0.77 V vs. SCE] might be reductively quenched by certain unclarified reductant to initiate the first photocatalytic cycle and provide Ru1+ has not been clarified in detail yet. Subsequently, the addition of radical A onto vinyl azide 2a leads to the release of an N2 molecule and the formation of an iminyl radical B, which undergoes 1,5-H atom transfer (1,5-HAT) to produce a more stabilized benzyl radical C. In path A, radical intermediate C proceeds via the 5-endo-trig radical cyclization with an imine moiety to give the electron-rich α-aminoalkyl radical D (–0.92 to –1.12 V vs. SCE) (Wayner et al., 1986; Du et al., 2020; Liang et al., 2020; Wu et al., 2020), which is readily oxidized by the excited-state *Ru2+ to access iminium cation E and finally yield the target 1-pyrroline 3aa with the aid of a base. For the [4 + 2] cyclization, the strongly reducing excited-state *Ir3+ [E1/2red (Ir4+/*Ir3+) = −1.73 V vs. SCE] (Teegardin et al., 2016) could be oxidatively quenched by diethyl 2-benzyl-2-bromomalonate 1a to give radical intermediate C through the same radical addition/1,5-HAT sequence. As depicted in path B, intermediate C undergoes the 6-endo-trig radical cyclization onto the phenyl ring of vinyl azide to form G, which is further oxidized by the oxidized ground-state Ir4+ (+0.77 V vs. SCE) and then undergoes the deprotonation/hydrolysis process to release 1-tetralone 4aa with the assistance of AcOH. In addition, another alternative path C, in which benzyl radical C could also undergo the single electron transfer with *Ru2+ or Ir4+ having a higher oxidation potential followed by 5- or 6-endo-trig ionic cyclization and further transformation to access 3aa or 4aa, could not be completely ruled out. In the [4 + 2] cyclization reaction, the additive acid might suppress the oxidation of α-aminoalkyl radical D in path A or 5-endo-trig ionic cyclization in path C, which resulted in the low efficiency of the [3 + 2] cyclization reaction. Notably, compound C⋅H, hydrolyzed C⋅H, compound J⋅Br, and hydrolyzed J⋅Br detected through LC-HRMS analysis of the ongoing reaction mixture validate the existence of radical C and cation J, providing further evidence for our proposed mechanism (for details see the Supplementary Material).
Conclusion
In conclusion, we have developed a controllable photoredox-neutral [3 + 2] and [4 + 2] cyclization of alkyl bromides and vinyl azides by simply manipulating the photocatalysts and additives, providing chemodivergent access to highly substituted 1-pyrrolines and 1-tetralones. This protocol relies on intermolecular radical addition triggered 1,5-hydrogen atom transfer/switchable C(sp3)–H bond functionalization tandem sequences. In addition, the mild reaction conditions, easy-to-handle feedstocks, good tolerance of functional groups, easy scalability, facile derivatization of products, and late-stage functionalization of bioactive molecule provide great potential for application in synthetic chemistry and pharmaceutical chemistry. Further mechanistic research and application of this protocol are currently undergoing in our laboratory.
Data availability statement
The datasets presented in this study can be found in online repositories. The names of the repository/repositories and accession number(s) can be found in the article/Supplementary Material.
Author contributions
SZ, XD, and DY conceived and designed the study, and wrote the paper. ST, ZQ, WL, ZZ, and LY performed the experiments and mechanistic studies. JW and SW analyzed the experiments. All authors contributed to the analysis and interpretation of the data.
Acknowledgments
The author gratefully acknowledges the financial support from the National Natural Science Foundation of China (22101237, 22171233), the Scientific Fund of Sichuan Province (2022NSFSC1219), the Open Project of Central Nervous System Drug Key Laboratory of Sichuan Province (200023-01SZ), and the research fund of Southwest Medical University (2021ZKMS042).
Conflict of interest
The authors declare that the research was conducted in the absence of any commercial or financial relationships that could be construed as a potential conflict of interest.
Publisher’s note
All claims expressed in this article are solely those of the authors and do not necessarily represent those of their affiliated organizations, or those of the publisher, the editors and the reviewers. Any product that may be evaluated in this article, or claim that may be made by its manufacturer, is not guaranteed or endorsed by the publisher.
Supplementary material
The Supplementary Material for this article can be found online at: https://www.frontiersin.org/articles/10.3389/fchem.2022.1058596/full#supplementary-material
References
Aicher, D., Grafe, S., Stark, C. B., and Wiehe, A. (2011). Synthesis of β-functionalized temoporfin derivatives for an application in photodynamic therapy. Bioorg. Med. Chem. Lett. 21, 5808–5811. doi:10.1016/j.bmcl.2011.07.113
Chen, C., Zhao, J., Shi, X., Liu, L., Zhu, Y.-P., Sun, W., et al. (2020). Recent advances in cyclization reactions of unsaturated oxime esters (ethers): Synthesis of versatile functionalized nitrogen-containing scaffolds. Org. Chem. Front. 7, 1948–1969. doi:10.1039/d0qo00397b
Davies, J., Booth, S. G., Essafi, S., Dryfe, R. A., and Leonori, D. (2015). Visible-light-mediated generation of nitrogen-centered radicals: Metal-free hydroimination and iminohydroxylation cyclization reactions. Angew. Chem. Int. Ed. 54, 14017–14021. doi:10.1002/anie.201507641
Dong, D.-Q., Song, J.-C., Yang, S.-H., Qin, Q.-X., Wang, Z.-L., Zhang, E.-X., et al. (2022). C(sp3)−H bond functionalization of oximes derivatives via 1, 5−hydrogen atom transfer induced by iminyl radical. Chin. Chem. Lett. 33, 1199–1206. doi:10.1016/j.cclet.2021.08.067
Du, F., Li, S. J., Jiang, K., Zeng, R., Pan, X. C., Lan, Y., et al. (2020). Iron-catalyzed radical relay enabling the modular synthesis of fused pyridines from alkyne-tethered oximes and alkenes. Angew. Chem. Int. Ed. 59, 23755–23762. doi:10.1002/anie.202010752
Fu, J., Zanoni, G., Anderson, E. A., and Bi, X. (2017). α-Substituted vinyl azides: An emerging functionalized alkene. Chem. Soc. Rev. 46, 7208–7228. doi:10.1039/c7cs00017k
Jiang, H., and Studer, A. (2017). Iminyl-radicals by oxidation of α-imino-oxy acids: Photoredox-neutral alkene carboimination for the synthesis of pyrrolines. Angew. Chem. Int. Ed. 56, 12273–12276. doi:10.1002/anie.201706270
Jiao, M. J., Hu, Q., Hu, X. Q., and Xu, P. F. (2021). Visible-light-promoted multistep tandem reaction of vinyl azides toward the formation of 1-tetralones. J. Org. Chem. 86, 17156–17163. doi:10.1021/acs.joc.1c02261
Krylov, I. B., Segida, O. O., Budnikov, A. S., and Terent'ev, A. O. (2021). Oxime-derived iminyl radicals in selective processes of hydrogen atom transfer and addition to carbon-carbon π-bonds. Adv. Synth. Catal. 363, 2502–2528. doi:10.1002/adsc.202100058
Lasri, J., Gupta, S., da Silva, M. F. C. G., and Pombeiro, A. J. L. (2012). Copper(II)-mediated in-situ hydrolyses of pyrroline N-oxide and benzonitrile leading to a mixed ligand complex. Inorg. Chem. Commun. 18, 69–72. doi:10.1016/j.inoche.2012.01.012
Legoabe, L. J., Van der Walt, M. M., and Terre'Blanche, G. (2018). Evaluation of 2-benzylidene-1-tetralone derivatives as antagonists of A1 and A2A adenosine receptors. Chem. Biol. Drug Des. 91, 234–244. doi:10.1111/cbdd.13074
Lei, W. L., Feng, K. W., Wang, T., Wu, L. Z., and Liu, Q. (2018). Eosin Y- and copper-catalyzed dark reaction to construct ene-gamma-lactams. Org. Lett. 20, 7220–7224. doi:10.1021/acs.orglett.8b03147
Li, Y., Zhu, Y., and Yang, S.-D. (2018). Visible-light-induced tandem phosphorylation cyclization of vinyl azides under mild conditions. Org. Chem. Front. 5, 822–826. doi:10.1039/c7qo01004d
Liang, Q., Lin, L., Li, G., Kong, X., and Xu, B. (2021). Synthesis of phenanthridine and quinoxaline derivatives via copper-catalyzed radical cyanoalkylation of cyclobutanone oxime esters and vinyl azides. Chin. J. Chem. 39, 1948–1952. doi:10.1002/cjoc.202100050
Liang, W., Jiang, K., Du, F., Yang, J., Shuai, L., Ouyang, Q., et al. (2020). Iron-catalyzed, iminyl radical-triggered cascade 1, 5-hydrogen atom transfer/(5+2) or (5+1) annulation: Oxime as a five-atom assembling unit. Angew. Chem. Int. Ed. 59, 19222–19228. doi:10.1002/anie.202007825
Liao, Y., Ran, Y., Liu, G., Liu, P., and Liu, X. (2020). Transition-metal-free radical relay cyclization of vinyl azides with 1, 4-dihydropyridines involving a 1, 5-hydrogen-atom transfer: Access to α-tetralone scaffolds. Org. Chem. Front. 7, 3638–3647. doi:10.1039/d0qo01042a
Lin, L., Liang, Q., Kong, X., Chen, Q., and Xu, B. (2020). Electrochemical tandem fluoroalkylation-cyclization of vinyl azides: Access to trifluoroethylated and difluoroethylated N-heterocycles. J. Org. Chem. 85, 15708–15716. doi:10.1021/acs.joc.0c02213
Miller, L. C., and Sarpong, R. (2011). Divergent reactions on racemic mixtures. Chem. Soc. Rev. 40, 4550–4562. doi:10.1039/c1cs15069c
Miltyk, W., and Pałka, J. A. (2000). Potential role of pyrroline 5-carboxylate in regulation of collagen biosynthesis in cultured human skin fibroblasts. Comp. Biochem. Physiology Part A Mol. Integr. Physiology 125, 265–271. doi:10.1016/s1095-6433(99)00181-6
Najera, C., Beletskaya, I. P., and Yus, M. (2019). Metal-catalyzed regiodivergent organic reactions. Chem. Soc. Rev. 48, 4515–4618. doi:10.1039/c8cs00872h
Newcomb, E. T., Knutson, P. C., Pedersen, B. A., and Ferreira, E. M. (2016). Total synthesis of gelsenicine via a catalyzed cycloisomerization strategy. J. Am. Chem. Soc. 138, 108–111. doi:10.1021/jacs.5b12263
Nicewicz, D. A., and MacMillan, D. W. (2008). Merging photoredox catalysis with organocatalysis: The direct asymmetric alkylation of aldehydes. Science 322, 77–80. doi:10.1126/science.1161976
Odagi, M., Furukori, K., Takayama, K., Noguchi, K., and Nagasawa, K. (2017). Total synthesis of rishirilide B by organocatalytic oxidative kinetic resolution: Revision of absolute configuration of (+)-rishirilide B. Angew. Chem. Int. Ed. Engl. 56, 6709–6712. doi:10.1002/ange.201701431
Pluotno, A., and Carmeli, S. (2005). Banyasin A and banyasides A and B, three novel modified peptides from a water bloom of the cyanobacterium Nostoc sp. Tetrahedron 61, 575–583. doi:10.1016/j.tet.2004.11.016
Qi, Z. Y., Zhang, Z. J., Yang, L., Zhang, D., Lu, J., Wei, J., et al. (2021). Nitrogen-radical-triggered trifunctionalizing ipso-spirocyclization of unactivated alkenes with vinyl azides: A modular access to spiroaminal frameworks. Adv. Synth. Catal. 363, 3762–3768. doi:10.1002/adsc.202100517
Rosser, R. M., and Faulkner, D. J. (1984). Two steroidal alkaloids from a marine sponge, Plakina sp. J. Org. Chem. 49, 5157–5160. doi:10.1021/jo00200a029
Schann, S., Bruban, V., Pompermayer, K., Feldman, J., Pfeiffer, B., Renard, P., et al. (2001). Synthesis and biological evaluation of pyrrolinic isosteres of rilmenidine. Discovery of cis-/trans-dicyclopropylmethyl-(4, 5-dimethyl-4, 5-dihydro-3H-pyrrol-2-yl)-amine (LNP 509), an I1 imidazoline receptor selective ligand with hypotensive activity. J. Med. Chem. 44, 1588–1593. doi:10.1021/jm001111b
Shu, W., Lorente, A., Gomez-Bengoa, E., and Nevado, C. (2017). Expeditious diastereoselective synthesis of elaborated ketones via remote Csp3-H functionalization. Nat. Commun. 8, 13832. doi:10.1038/ncomms13832
Shu, W., and Nevado, C. (2017). Visible-light-mediated remote aliphatic C-H functionalizations through a 1, 5-hydrogen transfer cascade. Angew. Chem. Int. Ed. 56, 1881–1884. doi:10.1002/anie.201609885
Stapon, A., Li, R., and Townsend, C. A. (2003). Carbapenem biosynthesis: Confirmation of stereochemical assignments and the role of CarC in the ring stereoinversion process from L-proline. J. Am. Chem. Soc. 125, 8486–8493. doi:10.1021/ja034248a
Swift, E., Williams, T., and Stephenson, C. (2016). Intermolecular photocatalytic C–H functionalization of electron-rich heterocycles with tertiary alkyl halides. Synlett 27, 754–758. doi:10.1055/s-0035-1561320
Taber, G. P., Pfisterer, D. M., and Colberg, J. C. (2004). A new and simplified process for preparing N-[4-(3, 4-dichlorophenyl)-3, 4-dihydro-1(2H)-naphthalenylidene]methanamine and a telescoped process for the synthesis of (1S-cis)-4-(3, 4-dichlorophenol)-1, 2, 3, 4-tetrahydro-N-methyl-1-naphthalenamine mandelate: Key intermediates in the synthesis of sertraline hydrochloride. Org. Process Res. Dev. 8, 385–388. doi:10.1021/op0341465
Tang, J., Sivaguru, P., Ning, Y., Zanoni, G., and Bi, X. (2017). Silver-catalyzed tandem C≡C bond hydroazidation/radical addition/cyclization of biphenyl acetylene: One-pot synthesis of 6-methyl sulfonylated phenanthridines. Org. Lett. 19, 4026–4029. doi:10.1021/acs.orglett.7b01771
Tang, Y. Q., Yang, J. C., Wang, L., Fan, M., and Guo, L. N. (2019). Ni-catalyzed redox-neutral ring-opening/radical addition/ring-closing cascade of cycloketone oxime esters and vinyl azides. Org. Lett. 21, 5178–5182. doi:10.1021/acs.orglett.9b01773
Teegardin, K., Day, J. I., Chan, J., and Weaver, J. (2016). Advances in photocatalysis: A microreview of visible light mediated ruthenium and iridium catalyzed organic transformations. Org. Process Res. Dev. 20, 1156–1163. doi:10.1021/acs.oprd.6b00101
Tsukamoto, D., Shibano, M., Okamoto, R., and Kusano, G. (2001). Studies on the constituents of Broussonetia species VIII. Four new pyrrolidine alkaloids, broussonetines R, S, T, and V and a new pyrroline alkaloid, broussonetine U, from Broussonetia kazinoki Sieb. Chem. Pharm. Bull. 49, 492–496. doi:10.1248/cpb.49.492
Tyroller, S., Zwickenpflug, W., and Richter, E. (2002). New sources of dietary myosmine uptake from cereals, fruits, vegetables, and milk. J. Agric. Food Chem. 50, 4909–4915. doi:10.1021/jf020281p
Usami, K., Yamaguchi, E., Tada, N., and Itoh, A. (2018). Visible-light-mediated iminyl radical generation from benzyl oxime ether: Synthesis of pyrroline via hydroimination cyclization. Org. Lett. 20, 5714–5717. doi:10.1021/acs.orglett.8b02429
Wallentin, C. J., Nguyen, J. D., Finkbeiner, P., and Stephenson, C. R. (2012). Visible light-mediated atom transfer radical addition via oxidative and reductive quenching of photocatalysts. J. Am. Chem. Soc. 134, 8875–8884. doi:10.1021/ja300798k
Wang, L., Huang, W., Li, R., Gehrig, D., Blom, P. W., Landfester, K., et al. (2016). Structural design principle of small-molecule organic semiconductors for metal-free, visible-light-promoted photocatalysis. Angew. Chem. Int. Ed. 55, 9783–9787. doi:10.1002/anie.201603789
Wang, P., Zhao, Q., Xiao, W., and Chen, J. (2020). Recent advances in visible-light photoredox-catalyzed nitrogen radical cyclization. Green Synthesis Catal. 1, 42–51. doi:10.1016/j.gresc.2020.05.003
Wayner, D. D. M., Dannenberg, J. J., and Griller, D. (1986). Oxidation potentials of α-aminoalkyl radicals: Bond dissociation energies for related radical cations. Chem. Phys. Lett. 131, 189–191. doi:10.1016/0009-2614(86)80542-5
Wu, C., Liu, T. X., Zhang, P., Zhu, X., and Zhang, G. (2020). Iron-catalyzed redox-neutral radical cascade reaction of [60]fullerene with γ, δ-unsaturated oxime esters: Preparation of free (N-H) pyrrolidino[2', 3':1, 2]fullerenes. Org. Lett. 22, 7327–7332. doi:10.1021/acs.orglett.0c02658
Wu, D., Cui, S. S., Bian, F., and Yu, W. (2021). Visible light driven and copper-catalyzed C(sp3)-H functionalization of O-pentafluorobenzoyl ketone oximes. Org. Lett. 23, 6057–6061. doi:10.1021/acs.orglett.1c02133
Zhan, G., Du, W., and Chen, Y. C. (2017). Switchable divergent asymmetric synthesis via organocatalysis. Chem. Soc. Rev. 46, 1675–1692. doi:10.1039/c6cs00247a
Keywords: 1-pyrrolines, hydrogen atom transfer, [3+2] cyclization, [4+2] cyclization, 1-tetralones
Citation: Tu S, Qi Z, Li W, Zhang S, Zhang Z, Wei J, Yang L, Wei S, Du X and Yi D (2022) Chemodivergent photocatalytic access to 1-pyrrolines and 1-tetralones involving switchable C(sp3)–H functionalization. Front. Chem. 10:1058596. doi: 10.3389/fchem.2022.1058596
Received: 30 September 2022; Accepted: 14 October 2022;
Published: 25 October 2022.
Edited by:
Xuefeng Cong, RIKEN, JapanReviewed by:
Shao-Jie Lou, Zhejiang University of Technology, ChinaXu-Ge Liu, Henan University, China
Copyright © 2022 Tu, Qi, Li, Zhang, Zhang, Wei, Yang, Wei, Du and Yi. This is an open-access article distributed under the terms of the Creative Commons Attribution License (CC BY). The use, distribution or reproduction in other forums is permitted, provided the original author(s) and the copyright owner(s) are credited and that the original publication in this journal is cited, in accordance with accepted academic practice. No use, distribution or reproduction is permitted which does not comply with these terms.
*Correspondence: Shiqi Zhang, emhhbmdzcUBjaWIuYWMuY24=; Xi Du, ZHguZEAxNjMuY29t; Dong Yi, eWlkb25nQHN3bXUuZWR1LmNu