- 1Luoyang Key Laboratory of Organic Functional Molecules, College of Food and Drug, Luoyang Normal University, Luoyang, Henan, China
- 2Hubei Key Laboratory of Natural Products Research and Development, College of Biological and Pharmaceutical Sciences, China Three Gorges University, Yichang, China
Prostate cancer (PCa) is a malignant tumor with a higher mortality rate in the male reproductive system. In this study, the hydroxyazine derivatives were synthesized with different structure from traditional anti-prostate cancer drugs. In the evaluation of in vitro cytotoxicity and antagonistic activity of PC-3, LNCaP, DU145 and androgen receptor, it was found that the mono-substituted derivatives on the phenyl group (4, 6, 7, and 9) displayed strong cytotoxic activities, and compounds 11–16 showed relatively strong antagonistic potency against AR (Inhibition% >55). Docking analysis showed that compounds 11 and 12 mainly bind to AR receptor through hydrogen bonds and hydrophobic bonds, and the structure-activity relationship was discussed based on activity data. These results suggested that these compounds may have instructive implications for drug structural modification in prostate cancer.
1 Introduction
Targeted anti-tumor drugs are the focus of modern anti-cancer research, because of their special targeting, they can greatly reduce the toxicity to normal cells (Falciani et al., 2010). In addition, cells in rapid division were more sensitive to the most drugs due to the differences in cell dynamics (Tannock, 1978). Therefore, targeted antitumor drugs can simultaneously inhibit the proliferation and differentiation of tumor cells and greatly accelerate the death of tumor cells (Vinaya et al., 2011). Prostate cancer (PCa) is a malignant tumor with a higher mortality rate in the male reproductive system (Greenlee et al., 2001). Prostate cancer is driven by the androgen receptor (Yap et al., 2016; Dai et al., 2017), and is directly associated with nuclear steroidal AR (Bentel and Tilley, 1996; Culig et al., 2002; Gelmann, 2002). Androgens bind to the AR and form a hormone-receptor complex, which can bind to the DNA and induce downstream biological effects (Dehm and Tindall, 2007). This complex also induces the proliferation of prostate cells, and ultimately causes tumorigenesis (Heinlein and Chang, 2004). Although some current treatments (hormonotherapy, radical prostatectomy, chemotherapy, or local radiotherapy) can treat androgen-dependent prostate cancer (Frydenberg et al., 1997). However, drug resistance problems hinder its therapeutic efficacy. Therefore, early detection and elimination of both types of prostate cancer cells are very important for decreasing prostate cancer-related death (Feldman and Feldman, 2001).
Piperazine moieties play an important role in many drugs (Chaudhary et al., 2006), and piperazine derivatives also have exhibited biological importance, such as receptor high-affinity properties (Leopoldo et al., 2007; Romeiro et al., 2011; Chen et al., 2012; Ananthan et al., 2014; Baran et al., 2014) and anti-proliferative properties (Berardi et al., 2008; Lee et al., 2010; Abate et al., 2011; Cao et al., 2013; Liu et al., 2013; Arnatt et al., 2014; Guo et al., 2015). Hydroxyzine (Figure 1) has antihistamine effect and can be quickly absorbed and distributed by oral or muscle injection. The arylpiperazine derivatives were reported to exhibit significant antagonism against AR with an IC50 of 0.11 μM, whereas the IC50 of bicaluramide is 50 μM. Results of animal experiments have shown that the mass of prostate in rats is significantly reduced, and the concentration of serum testosterone is not significantly changed (Kinoyama et al., 2004; Kinoyama et al., 2005; Gupta et al., 2016). However, there have been few studies on hydroxyzine derivatives. Based on the results of our group’s previous anti-prostate cancer study (Chen et al., 2016; Chen et al., 2017; Chen et al., 2018a; Chen et al., 2019a; Chen et al., 2019b; Qi et al., 2022), we tried to design and synthesize a series of novel hydroxyzine derivatives (Scheme 1) with 2-p-tolylethanol group instead of 2-ethoxyethanol group in hydroxyzine. Unexpectedly, some derivatives exhibited strong anti-cancer activities and antagonistic activities. These results can provide valuable information for further designing hydroxyzine derivatives as potential prostate cancer therapeutics.
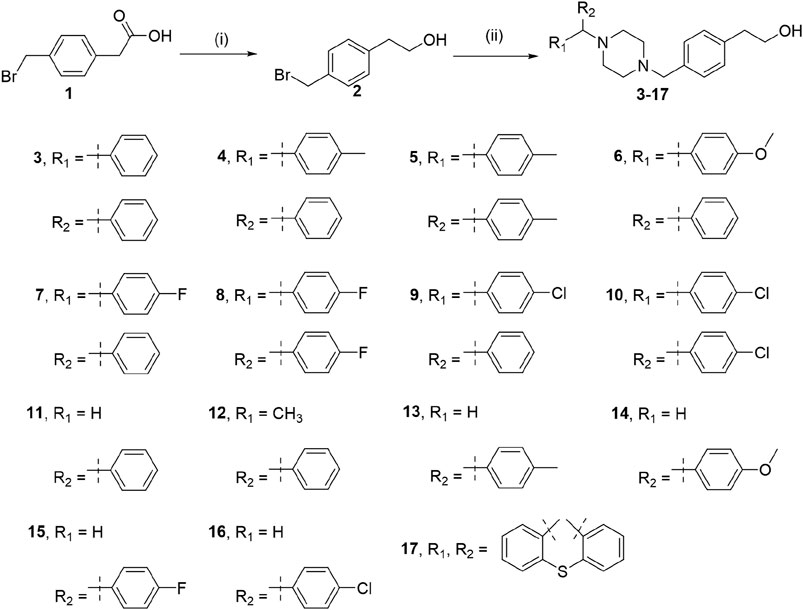
SCHEME 1. Reagents and conditions: (i) BH3.S(CH3)2, THF, 0°C for 1 h, and room temperature for 10 h; (ii) Piperazines, K2CO3, CH3CN, reflux, 16 h.
2 Materials and methods
2.1 Materials and instruments
Reagents and solvents were of analytical purity and dried and using standard procedures. The melting point was measured using the Shanghai electrical optical SGW X-4 micromelting point instrument. The HRMS mass spectrometry was measured using the LCQ DECA XP LC-MS. The NMR spectra were measured using the Bruker AV-400 NB, with TMS as the internal standard, and DMSO-d6 or CDCl3 as the solvent. Column chromatography silica gel was the 300–400 mesh silicone of Qingdao Marine Chemical Plant.
2.2 Synthesis of 2-[4-(bromomethyl)phenyl]ethanol (2)
The borane–dimethyl sulfide (20.0 ml, 0.038 mol, 2 M in THF) was added to the tetrahydrofuran (THF, 100 ml) solution, supplemented with carboxylic acid 1 (5 g, 0.021 mol), and stirred at 0°C for 1 h. Then stirred at r. t. for 10 h. Extracted with ethyl acetate (100 ml) and water (20 ml). Concentrated organic phase, the resulting residue was directly used without further purification.
2.3 Preparation of derivatives 3–17
Piperazines (1.3 equiv), potassium carbonate (5.5 equiv), acetonitrile (CH3CN, 10 ml), and 2 (50 mg, 0.11 mmol) were successively added to the flask, stirred with reflux for 10 h. The reaction solution was filtered and concentrated, and purified by silica gel column chromatography (ethyl acetate/petroleum ether = 1/5).
2.3.1 2-(4-((4-benzhydrylpiperazin-1-yl)methyl)phenyl)ethan-1-ol (3)
White solid (ethyl acetate), yield: 85% (from compound 1); M.p. 122°C–123°C; 1H NMR (400 MHz, DMSO-d6) δ in ppm: 7.75–7.10 (m, 14H), 4.26 (br s, 2H), 3.64 (br s, 10H), 3.56 (t, J = 6.9 Hz, 2H), 2.70 (t, J = 6.9 Hz, 2H); MS (ESI, m/z): 387.1 [M+1]+; HRMS (EI) calcd for C26H29ClN2O, 386.2358; found, 386.2354.
2.3.2 2-(4-((4-(phenyl(p-tolyl)methyl)piperazin-1-yl)methyl)phenyl)ethan-1-ol (4)
White solid (ethyl acetate), yield: 82% (from compound 1); M.p. 125°C–126°C; 1H NMR (400 MHz, DMSO-d6) δ in ppm: 7.80–7.11 (m, 13H), 4.27 (br s, 2H), 3.66 (br s, 10H), 3.57 (t, J = 6.9 Hz, 2H), 2.72 (t, J = 6.9 Hz, 2H); 2.34 (s, 3H), MS (ESI, m/z): 401.2 [M+1]+; HRMS (EI) calcd for C27H32N2O, 400.2515; found, 400.2510.
2.3.3 2-(4-((4-(di-p-tolylmethyl)piperazin-1-yl)methyl)phenyl)ethan-1-ol (5)
White solid (ethyl acetate), yield: 82% (from compound 1); M.p. 128°C–129°C; 1H NMR (400 MHz, DMSO-d6) δ in ppm: 7.75 (br s, 4H), 7.45 (d, J = 8.0 Hz, 2H), 7.23 (d, J = 8.0 Hz, 2H), 7.16 (t, J = 8.1 Hz, 4H), 4.25 (br s, 2H), 3.64 (br s, 10H), 3.56 (t, J = 6.9 Hz, 2H), 2.71 (t, J = 6.9 Hz, 2H); 2.36 (s, 6H), MS (ESI, m/z): 415.1 [M+1]+; HRMS (EI) calcd for C28H34N2O, 414.2671; found, 414.2668.
2.3.4 2-(4-((4-((4-methoxyphenyl)(phenyl)methyl)piperazin-1-yl)methyl)phenyl)ethan-1-ol (6)
White solid (ethyl acetate), yield: 87% (from compound 1); M.p. 116°C–117°C; 1H NMR (400 MHz, DMSO-d6) δ in ppm: 7.76–7.13 (m, 13H), 4.25 (br s, 2H), 3.77 (s, 3H), 3.67 (br s, 10H), 3.54 (t, J = 6.9 Hz, 2H), 2.74 (t, J = 6.9 Hz, 2H), MS (ESI, m/z): 417.1 [M+1]+; HRMS (EI) calcd for C27H32N2O2, 416.2464; found, 416.2462.
2.3.5 2-(4-((4-((4-fluorophenyl)(phenyl)methyl)piperazin-1-yl)methyl)phenyl)ethan-1-ol (7)
White solid (ethyl acetate), yield: 87% (from compound 1); M.p. 116°C–117°C; 1H NMR (400 MHz, DMSO-d6) δ in ppm: 7.70–7.11 (m, 13H), 4.27 (br s, 2H), 3.64 (br s, 10H), 3.57 (t, J = 6.9 Hz, 2H), 2.72 (t, J = 6.9 Hz, 2H), MS (ESI, m/z): 405.1 [M+1]+; HRMS (EI) calcd for C26H29FN2O, 404.2264; found, 404.2260.
2.3.6 2-(4-((4-(bis(4-fluorophenyl)methyl)piperazin-1-yl)methyl)phenyl)ethanol (8)
White solid (ethyl acetate), yield: 70% (from compound 1); M.p. 133°C–134°C; 1H NMR (400 MHz, DMSO-d6) δ in ppm: 1H NMR (400 MHz, DMSO-d6) δ 7.70 (br s, 4H), 7.46 (d, J = 8.0 Hz, 2H), 7.22 (d, J = 8.0 Hz, 2H), 7.18 (t, J = 8.2 Hz, 4H), 4.27 (br s, 2H), 3.66 (br s, 10H), 3.55 (t, J = 6.9 Hz, 2H), 2.68 (t, J = 6.9 Hz, 2H); MS (ESI, m/z): 423.2 [M+1]+; HRMS (EI) calcd for C26H28F2N2O, 422.2170; found, 422.2168.
2.3.7 2-(4-((4-((4-chlorophenyl)(phenyl)methyl)piperazin-1-yl)methyl)phenyl)ethanol (9)
White solid (ethyl acetate), yield: 75% (from compound 1); M.p. 130°C–131°C; 1H NMR (400 MHz, DMSO-d6) δ in ppm: 7.89–7.13 (m, 13H), 4.29 (br s, 2H), 3.66 (br s, 10H), 3.57 (t, J = 6.9 Hz, 2H), 2.71 (t, J = 6.9 Hz, 2H); MS (ESI, m/z): 421.1 [M+1]+; HRMS (EI) calcd for C26H29ClN2O, 420.1968; found, 420.1962.
2.3.8 2-(4-((4-(bis(4-chlorophenyl)methyl)piperazin-1-yl)methyl)phenyl)ethan-1-ol (10)
White solid (ethyl acetate), yield: 72% (from compound 1); M.p. 127°C–128°C; 1H NMR (400 MHz, DMSO-d6) δ in ppm: 7.67 (br s, 4H), 7.42 (d, J = 8.0 Hz, 2H), 7.23 (d, J = 8.0 Hz, 2H), 7.16 (t, J = 8.0 Hz, 4H), 4.23 (br s, 2H), 3.68 (br s, 10H), 3.57 (t, J = 6.9 Hz, 2H), 2.66 (t, J = 6.9 Hz, 2H); MS (ESI, m/z): 455.2 [M+1]+; HRMS (EI) calcd for C26H28Cl2N2O, 454.1579; found, 454.1575.
2.3.9 2-(4-((4-benzylpiperazin-1-yl)methyl)phenyl)ethan-1-ol (11)
White solid (ethyl acetate), yield: 82% (from compound 1); M.p. 119°C–120°C; 1H NMR (400 MHz, CDCl3) δ in ppm: 7.43 (d, J = 8.0 Hz, 2H), 7.32 (t, J = 8.0 Hz, 2H), 7.24 (d, J = 8.0 Hz, 2H), 7.05 (d, J = 7.8 Hz, 2H), 6.98 (t, J = 7.6 Hz, 1H), 4.25 (s, 2H), 3.76 (t, J = 6.8 Hz, 2H), 3.62 (s, 2H), 3.54 (t, J = 5.0 Hz, 4H), 2.72 (t, J = 6.8 Hz, 2H), 2.68 (t, J = 5.0 Hz, 4H); MS (ESI, m/z): 311.1 [M+1]+; HRMS (EI) calcd for C20H26N2O, 310.2045; found, 310.2040.
2.3.10 2-(4-((4-(1-phenylethyl)piperazin-1-yl)methyl)phenyl)ethan-1-ol (12)
White solid (ethyl acetate), yield: 78% (from compound 1); M.p. 114°C–115°C; 1H NMR (400 MHz, CDCl3) δ in ppm: 7.58–7.09 (m, 9H), 4.23 (q, J = 6.6 Hz, 1H), 3.64 (br s, 8H), 3.62 (t, J = 6.8 Hz, 2H), 3.55 (s, 2H), 2.68 (t, J = 6.8 Hz, 2H), 1.12 (d, J = 6.6 Hz, 3H); MS (ESI, m/z): 325.2 [M+1]+; HRMS (EI) calcd for C21H28N2O, 324.2202; found, 324.2200.
2.3.11 2-(4-((4-(4-methylbenzyl)piperazin-1-yl)methyl)phenyl)ethan-1-ol (13)
White solid (ethyl acetate), yield: 75% (from compound 1); M.p. 112°C–113°C; 1H NMR (400 MHz, CDCl3) δ in ppm: 7.41 (d, J = 8.0 Hz, 2H), 7.30 (t, J = 8.0 Hz, 2H), 7.22 (d, J = 8.0 Hz, 2H), 7.02 (d, J = 8.0 Hz, 2H), 4.27 (s, 2H), 3.74 (t, J = 6.8 Hz, 2H), 3.66 (s, 2H), 3.56 (t, J = 5.0 Hz, 4H), 2.70 (t, J = 6.8 Hz, 2H), 2.64 (t, J = 5.0 Hz, 4H); MS (ESI, m/z): 325.1 [M+1]+; HRMS (EI) calcd for C21H28N2O, 324.2202; found, 324.2200.
2.3.12 2-(4-((4-(4-methoxybenzyl)piperazin-1-yl)methyl)phenyl)ethan-1-ol (14)
White solid (ethyl acetate), yield: 82% (from compound 1); M.p. 117°C–118°C; 1H NMR (400 MHz, CDCl3) δ in ppm: 7.43 (d, J = 8.0 Hz, 2H), 7.31 (t, J = 8.0 Hz, 2H), 7.23 (d, J = 8.0 Hz, 2H), 7.00 (d, J = 8.0 Hz, 2H), 4.25 (s, 2H), 3.81 (s, 3H), 3.72 (t, J = 6.8 Hz, 2H), 3.67 (s, 2H), 3.54 (t, J = 5.0 Hz, 4H), 2.72 (t, J = 6.8 Hz, 2H), 2.62 (t, J = 5.0 Hz, 4H); MS (ESI, m/z): 341.0 [M+1]+; HRMS (EI) calcd for C21H28N2O2, 340.2151; found, 340.2149.
2.3.13 2-(4-((4-(4-fluorobenzyl)piperazin-1-yl)methyl)phenyl)ethan-1-ol (15)
White solid (ethyl acetate), yield: 70% (from compound 1); M.p. 114°C–115°C; 1H NMR (400 MHz, CDCl3) δ in ppm: 7.42 (d, J = 8.0 Hz, 2H), 7.32 (t, J = 8.0 Hz, 2H), 7.21 (d, J = 8.0 Hz, 2H), 7.03 (d, J = 8.0 Hz, 2H), 4.26 (s, 2H), 3.70 (t, J = 6.8 Hz, 2H), 3.64 (s, 2H), 3.55 (t, J = 5.0 Hz, 4H), 2.70 (t, J = 6.8 Hz, 2H), 2.63 (t, J = 5.0 Hz, 4H); MS (ESI, m/z): 329.1 [M+1]+; HRMS (EI) calcd for C20H25FN2O, 328.1951; found, 328.1948.
2.3.14 2-(4-((4-(4-chlorobenzyl)piperazin-1-yl)methyl)phenyl)ethan-1-ol (16)
White solid (ethyl acetate), yield: 72% (from compound 1); M.p. 109°C–110°C; 1H NMR (400 MHz, CDCl3) δ in ppm: 7.43 (d, J = 8.0 Hz, 2H), 7.33 (t, J = 8.0 Hz, 2H), 7.20 (d, J = 8.0 Hz, 2H), 7.01 (d, J = 8.0 Hz, 2H), 4.24 (s, 2H), 3.72 (t, J = 6.8 Hz, 2H), 3.67 (s, 2H), 3.56 (t, J = 5.0 Hz, 4H), 2.72 (t, J = 6.8 Hz, 2H), 2.66 (t, J = 5.0 Hz, 4H); MS (ESI, m/z): 345.1 [M+1]+; HRMS (EI) calcd for C20H25ClN2O, 344.1655; found, 344.1653.
2.3.15 2-(4-((4-(9H-thioxanthen-9-yl)piperazin-1-yl)methyl)phenyl)ethan-1-ol (17)
White solid (ethyl acetate), yield: 78% (from compound 1), M.p. 121°C–122°C; 1H NMR (400 MHz, DMSO-d6) δ in ppm: 7.73–7.08 (m, 12H), 4.24 (br s, 2H), 3.67 (br s, 10H), 3.57 (t, J = 6.9 Hz, 2H), 2.74 (t, J = 6.9 Hz, 2H); MS (ESI, m/z): 417.1 [M+1]+; HRMS (EI) calcd for C26H28N2OS, 416.1922; found, 416.1920.
2.4 Biological evaluation
2.4.1 Cell culture
LNCaP, PC-3 and WPMY-1 cells were cultured in Ham’s F-12K (PM150910) supplemented with 10% FBS (164210-50) and 1% P/S (PB180120). DU145 cells were cultured in MEM (PM150410) supplemented with 10% FBS (164210–50) and 1% P/S (PB180120). The cells were incubated at 37°C with 5% CO2 (Qi et al., 2022).
2.4.2 Assessment of antitumor activity by CCK-8 assay
Cell proliferation was measured using the CCK-8 assay kit (Kaspers et al., 1997; Kaspers et al., 1998; Ding et al., 2010). Cells were seeded into 96-well plates (>5*104) with approximately 100 ul of cell suspension per well and incubated in 37°C incubator for 4 h. Various concentrations of the compounds were then added and incubated for a further 24 h in a 37°C incubator. Finally, 10 ul CCK8 was added and incubated for 0.5–4 h, absorbance at 450 nm was determined. (Guo et al., 2021; He et al., 2022a; Hu et al., 2022).
2.5 AR reporter gene assay
Firefly and Renilla luciferase activities, were determined using the Dual-Glo™luciferase assay kit. RLUs were determined using the GloMax®96-Microplate Luminometer. IC50 was calculated using the GraphPad Prism 5.0 (He et al., 2022b; Qi et al., 2022).
2.6 Molecular docking simulation
Binding mechanism experiments performed docking analysis of the three active pockets (LBP, AF2 and BF3) (Axerio-Cilies et al., 2011; Lack et al., 2011) in AR receptors using AutoDock software (Trott and Olson, 2010). Its PDB protein data (2OZ7, 2YHD and 2YLO) was downloaded from the protein data bank (PDB) (Rose et al., 2011), and the proteins were optimized by the addition of hydrogen atoms and the removal of foreign ligands before docking. A docking space of 40 Å × 40 Å × 30 Å was constructed centered on the ligand of the AR active pocket, with compounds 11 and 12 as template molecules, docked into the optimized cavity and repeated 10 times to find a conformation of the one with the lowest binding free energy.
3 Results and discussions
3.1 Chemistry
Compounds 3–17 were synthesized by the following two-step method as depicted in Scheme 1. The compound 1 was reduced to compound 2 with BH3.S(CH3)2. Then, the compound 2 was heated at reflux with various piperines in an alkaline environment for 16 h.
3.2 Cytotoxic activity and AR antagonist activity
The in vitro cytotoxic activity results of the synthesized derivatives 3–17 against human prostate cancer lines (PC-3, LNCaP, and DU145) and the human prostate epithelial cell line (WPMY-1) were evaluated, as shown in Table 1.
The compounds 3–10 and 12–17 showed strong cytotoxic activity against PC-3 cells and were more potent than finasteride; the compounds 3–12 and 17 showed strong cytotoxic activity against LNCaP cells; the compounds 3–11 and 17 showed strong cytotoxic activity against DU145 cells. In addition, mono-substituted derivatives on the phenyl group (4, 6, 7, and 9) displayed strong cytotoxic activities against all the tested cancer cells. And these compounds exhibited low cytotoxicity to normal human prostate epithelial WPMY-1 cells.
Structure-activity relationship investigation was focused on the effects of changes in different substituents on the phenyl group. For instance, compared to compound 3, the compounds with mono-substituted group on the phenyl group (4, 6, 7, and 9) exhibited potent anticancer activity against LNCaP, DU145, and PC-3 cells. However, Dimethyl-substituted derivative 5 displayed moderate activity against PC-3 and DU145 cells, compounds 8 and 10 also had the similar properties. Moreover, compound 6 with electron-donating group also demonstrated strong cytotoxic activities against all the tested cancer cells. In order to compare the cytotoxic activity of compounds 3–10, the compounds 11–16 were synthesized, and the substitution of R1 and R2 groups with two phenyl groups showed high cytotoxic activity against the tested cancer cells. In summary, the introduction of this piperazine moiety contributes to its activity. Both PC-3 and DU145 cells are androgen-insensitive cell lines, but the compounds have different inhibitory activities against PC-3 and DU145 cells. The p53 is one of the most commonly mutated genes in human cancer, and the expression of p53 gene may be a key determinant of derivatives sensitivity in prostate cancer DU145 cells (Liu et al., 2013b). The literatures have reported that the p53 in DU145 cells were significantly activated by drugs, but in PC-3 cells the expression of the p53 gene was undetectable (Isaacs et al., 1991; Mashimo et al., 2000). So the compounds have different inhibitory activities against PC-3 and DU145 cells, and PC-3 cells are insensitive to derivatives.
The antagonistic activity of these derivatives against AR was assessed using luciferase assays (Xu et al., 2014; Xu et al., 2015; Zuo et al., 2017; Xu et al., 2018). As shown in Table 2, the compounds 3–10 exhibited weak antagonistic potency against AR. However, compounds 11–16 demonstrated relatively potent antagonistic potency (>55% inhibition).The above results were be contrary to the tested cancer cells antiproliferation activity. The results indicated that a small group introduction to the piperazine ring may be helpful for antagonistic activity against AR.
3.3 Docking study
In order to better understand the binding site of derivatives targets, the docking simulation into the three binding sites of AR (LBP, AF2, and BF3) of compounds 11 and 12 were performed using AutoDock Vina software, as shown in Table 3.
As displayed in Table 3, the binding free energies of the compounds 11 and 12 to all three sites of the AR were calculated, both of the LBP sites had the lowest binding free energy, as measured at −8.1 and −8.5 kcal/mol, respectively. As shown in Figure 2, both compounds 11 and 12 could form hydrophobic interactions with over a dozen amino acid residues, such as Gln711, Met745, and Ala877. More importantly, they were all able to form hydrogen bonds with the amino acid residue Phe697, at a distance of between 3.5 Å.
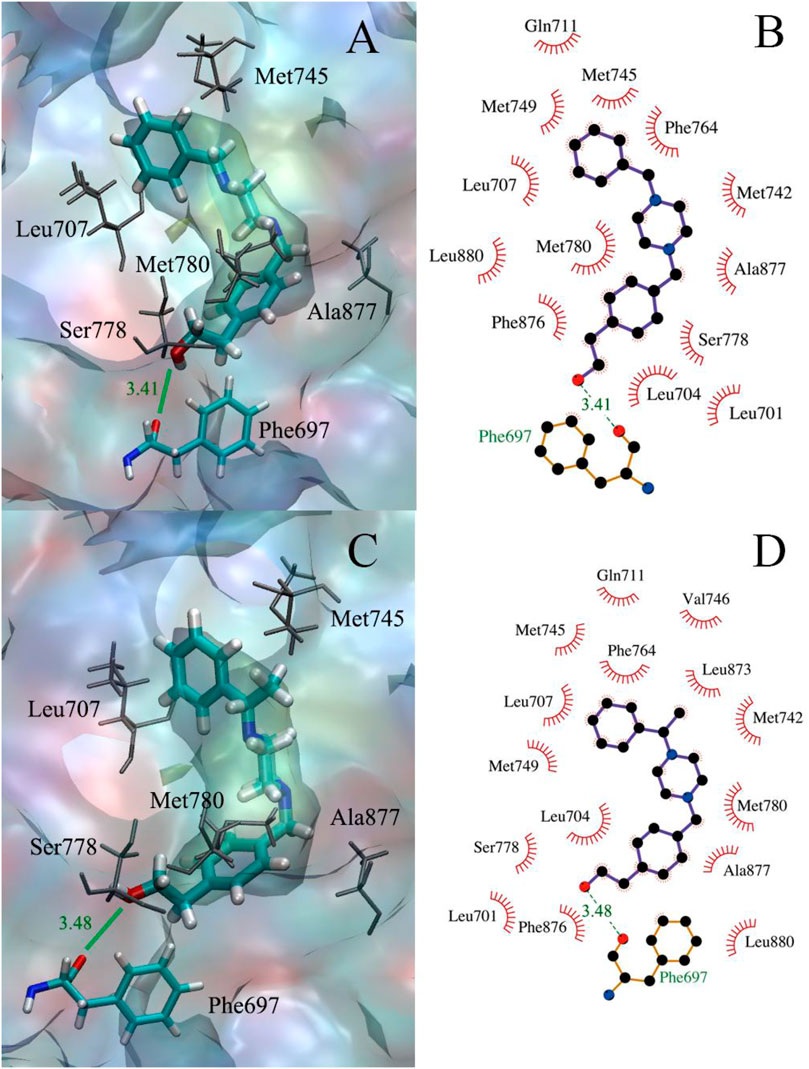
FIGURE 2. (A,B) The docking of compound 11 to the AR receptor; (C,D) The docking of compound 12 to the AR receptor.
4 Conclusion
In summary, in this study a series of novel hydroxyazine derivatives were synthesized and evaluated for antagonistic activity against AR and cytotoxic activity against human prostate cancer cells. The results showed that the derivative 4, 6, 7, and 9 displayed strong cytotoxic activities against the LNCaP, DU145 and PC-3 cells, and compounds 11–16 showed strong AR antagonism (Inhibition% >55). The SAR results suggested that mono-substituted derivatives on the phenyl group contributed to improve the cytotoxic activity against human prostate cancer cells. These hydroxyzazine piperazine derivatives may be instructive for structural modification of novel anti-prostate cancer drugs.
Data availability statement
The original contributions presented in the study are included in the article/Supplementary Material, further inquiries can be directed to the corresponding authors.
Author contributions
YQ, BX, and SC performed synthesis experiments. YQ, BX, and WW conducted the biological evaluation and molecular docking. YQ, HZ, and HC designed experiments. YQ and HC interpreted the data and wrote the paper. All authors contributed to the article and approved the submitted version.
Funding
The work was supported by the Henan Province Science and Technology Attack Plan Foundation (Nos 212102110241 and 222102310356), the Key Scientific Research Project of Higher Education of Henan Province (Nos 21A350006 and 22B350004), the National Natural Science Foundation of China (No. 22278244), and 111 Project (No. D20015).
Conflict of interest
The authors declare that the research was conducted in the absence of any commercial or financial relationships that could be construed as a potential conflict of interest.
Publisher’s note
All claims expressed in this article are solely those of the authors and do not necessarily represent those of their affiliated organizations, or those of the publisher, the editors and the reviewers. Any product that may be evaluated in this article, or claim that may be made by its manufacturer, is not guaranteed or endorsed by the publisher.
Supplementary material
The Supplementary Material for this article can be found online at: https://www.frontiersin.org/articles/10.3389/fchem.2022.1053675/full#supplementary-material
References
Abate, C., Niso, M., Contino, M., Colabufo, N. A., Ferorelli, S., Perrone, R., et al. (2011). 1-Cyclohexyl-4-(4-arylcyclohexyl) piperazines: Mixed σ and human Δ(8)-Δ(7) sterol isomerase ligands with antiproliferative and P-glycoprotein inhibitory activity. ChemMedChem 6, 73–80. doi:10.1002/cmdc.201000371
Ananthan, S., Saini, S. K., Zhou, G., Hobrath, J. V., Padmalayam, I., Zhai, L., et al. (2014). Design, synthesis, and structure-activity relationship studies of a series of [4-(4-carboxamidobutyl)]-1-arylpiperazines: Insights into structural features contributing to dopamine D3 versus D2 receptor subtype selectivity. J. Med. Chem. 57, 7042–7060. doi:10.1021/jm500801r
Arnatt, C. K., Adams, J. L., Zhang, Z., Haney, K. M., Li, G., and Zhang, Y. (2014). Design, syntheses, and characterization of piperazine based chemokine receptor CCR5 antagonists as anti-prostate cancer agents. Bioorg. Med. Chem. Lett. 24, 2319–2323. doi:10.1016/j.bmcl.2014.03.073
Axerio-Cilies, P., Lack, N. A., Nayana, M. R., Chan, K. H., Yeung, A., Leblanc, E., et al. (2011). Inhibitors of androgen receptor activation function-2 (AF2) site identified through virtual screening. J. Med. Chem. 54, 6197–6205. doi:10.1021/jm200532b
Baran, M., Kepczynska, E., Zylewski, M., Siwek, A., Bednarski, M., and Cegla, M. T. (2014). Studies on novel pyridine and 2-pyridone derivatives of N-arylpiperazine as α-adrenoceptor ligands. Med. Chem. Los. Angeles. 10, 144–153. doi:10.2174/0929867320999131122114922
Bentel, J. M., and Tilley, W. D. (1996). Androgen receptors in prostate cancer. J. Endocrinol. 151, 1–11. doi:10.1677/joe.0.1510001
Berardi, F., Abate, C., Ferorelli, S., De Robertis, A. F., Leopoldo, M., Colabufo, N. A., et al. (2008). Novel 4-(4-Aryl)cyclohexyl-1-(2-pyridyl)piperazines as Δ8−Δ7 sterol isomerase (emopamil binding protein) selective ligands with antiproliferative activity. J. Med. Chem. 51, 7523–7531. doi:10.1021/jm800965b
Cao, S. L., Han, Y., Yuan, C. Z., Wang, Y., Xiahou, Z. K., Liao, J., et al. (2013). Synthesis and antiproliferative activity of 4-substituted-piperazine-1-carbodithioate derivatives of 2, 4-diaminoquinazoline. Eur. J. Med. Chem. 64, 401–409. doi:10.1016/j.ejmech.2013.04.017
Chaudhary, P., Kumar, R., Verma, A. K., Singh, D., Yadav, V., Chhillar, A. K., et al. (2006). Synthesis and antimicrobial activity of N-alkyl and N-aryl piperazine derivatives. Bioorg. Med. Chem. 14, 1819–1826. doi:10.1016/j.bmc.2005.10.032
Chen, H., Liang, X., Sun, T., Qiao, X. G., Zhou, Z., Li, Z. Y., et al. (2018a). Synthesis and biological evaluation of estrone 3-O-ether derivatives containing the piperazine moiety. Steroids 134, 101–109. doi:10.1016/j.steroids.2018.02.002
Chen, H., Wang, C. L., Sun, T., Zhou, Z., Niu, J. X., Tian, X. M., et al. (2018b). Synthesis, biological evaluation and SAR of naftopidil-based arylpiperazine derivatives. Bioorg. Med. Chem. Lett. 28, 1534–1539. doi:10.1016/j.bmcl.2018.03.070
Chen, H., Xu, B. B., Sun, T., Zhou, Z., Ya, H. Y., and Yuan, M. (2017). Synthesis and antitumor activity of novel arylpiperazine derivatives containing the saccharin moiety. Molecules 22, 1857. doi:10.3390/molecules22111857
Chen, H., Xu, F., Xu, B. B., Xu, J. Y., Shao, B. H., Huang, B. Y., et al. (2016). Design, synthesis and biological evaluation of novel arylpiperazine derivatives on human prostate cancer cell lines. Bioorg. Med. Chem. Lett. 27, 285–287. doi:10.1016/j.bmcl.2014.11.049
Chen, H., Yu, Y. Z., Tian, X. M., Wang, C. L., Qian, Y. N., Deng, Z. A., et al. (2019a). Synthesis and biological evaluation of arylpiperazine derivatives as potential anti-prostate cancer agents. Bioorg. Med. Chem. 27, 133–143. doi:10.1016/j.bmc.2018.11.029
Chen, H., Zhang, J. X., Hu, P. X., Qian, Y. N., Li, J., and Shen, J. L. (2019b). Synthesis, biological evaluation and molecular docking of 4-Amino-2Hbenzo[h]chromen-2-one (ABO) analogs containing the piperazine moiety. Bioorg. Med. Chem. 27, 115081. doi:10.1016/j.bmc.2019.115081
Chen, X., Sassano, M. F., Zheng, L. Y., Setola, V., Chen, M., Bai, X., et al. (2012). Structure–functional selectivity relationship studies of β-arrestin-biased dopamine D2Receptor agonists. J. Med. Chem. 55, 7141–7153. doi:10.1021/jm300603y
Culig, Z., Klocker, H., Bartsch, G., Hobishc, A., and Hobisch, A. (2002). Androgen receptors in prostate cancer. Endocr. Relat. Cancer 9, 155–170. doi:10.1677/erc.0.0090155
Dai, C., Heemers, H., and Sharifi, N. (2017). Androgen signaling in prostate cancer. Cold Spring Harb. Perspect. Med. 7, a030452. doi:10.1101/cshperspect.a030452
Dehm, S. M., and Tindall, D. J. (2007). Androgen receptor structural and functional elements: Role and regulation in prostate cancer. Mol. Endocrinol. 21, 2855–2863. doi:10.1210/me.2007-0223
Ding, J., Huang, S. L., Wu, S. Q., Zhao, Y. J., Liang, L. H., Yan, M. X., et al. (2010). Gain of miR-151 on chromosome 8q24.3 facilitates tumour cell migration and spreading through downregulating RhoGDIA. Nat. Cell Biol. 12, 390–399. doi:10.1038/ncb2039
Falciani, C., Brunetti, J., Pagliuca, C., Menichetti, S., Vitellozzi, L., Lelli, B., et al. (2010). Design and in vitro evaluation of branched peptide conjugates: Turning nonspecific cytotoxic drugs into tumor-selective agents. ChemMedChem 5, 567–574. doi:10.1002/cmdc.200900527
Feldman, B. J., and Feldman, D. (2001). The development of androgen-independent prostate cancer. Nat. Rev. Cancer 1, 34–45. doi:10.1038/35094009
Frydenberg, M., Stricker, P. D., and Kaye, K. W. (1997). Prostate cancer diagnosis and management. Lancet 349, 1681–1687. doi:10.1016/S0140-6736(96)07393-X
Gelmann, E. P. (2002). Molecular biology of the androgen receptor. J. Clin. Oncol. 20, 3001–3015. doi:10.1200/JCO.2002.10.018
Greenlee, R. T., Murray, T., Hill-Harmon, M. B., and Thun, M. J. (2001). Cancer statistics, 2001. CA A Cancer J. Clin. 51, 15–36. doi:10.3322/canjclin.51.1.15
Guo, F. J., Sun, J., Gao, L. L., Wang, X. Y., Zhang, Y., Qian, S. S., et al. (2015). Discovery of phenylpiperazine derivatives as IGF-1R inhibitor with potent antiproliferative properties in vitro. Bioorg. Med. Chem. Lett. 25, 1067–1071. doi:10.1016/j.bmcl.2015.01.011
Guo, Z. H., Shi, L. Q., Feng, H. Y., Yang, F., Li, Z. R., Zhang, J. J., et al. (2021). Reduction-sensitive nanomicelles: Delivery celastrol for retinoblastoma cells effective apoptosis. Chin. Chem. Lett. 32, 1046–1050. doi:10.1016/j.cclet.2020.03.066
Gupta, S., Pandey, D., Mandalapu, D., Bala, V., Sharma, V., Shukla, M., et al. (2016). Design, synthesis and biological profiling of aryl piperazine based scaffolds for the management of androgen sensitive prostatic disorders. MedChemComm 7, 2111–2121. doi:10.1039/C6MD00426A
He, X. J., Dai, L. X., YeSun, L. S. X. S., Enoch, O., Hu, R. D., Zan, X. J., et al. (2022b). A vehicle-free antimicrobial polymer hybrid gold nanoparticle as synergistically therapeutic platforms for staphylococcus aureus infected wound healing. Adv. Sci. (Weinh). 9 (14), 2105223. doi:10.1002/advs.202105223
He, X. J., Hou, J. T., Sun, X. S., Jangili, P., An, J., Qian, Y., et al. (2022a). Nir-ii photo-amplified sonodynamic therapy using sodium molybdenum bronze nanoplatform against subcutaneous staphylococcus aureus infection. Adv. Funct. Mat. 32 (38), 2203964. doi:10.1002/adfm.202203964
Heinlein, C. A., and Chang, C. (2004). Androgen receptor in prostate cancer. Endocr. Rev. 25, 276–308. doi:10.1210/er.2002-0032
Hu, F. Y., Song, B., Wang, X. H., Bao, S., Shang, S. Y., Lv, S. P., et al. (2022). Green rapid synthesis of Cu2O/Ag heterojunctions exerting synergistic antibiosis. Chin. Chem. Lett. 33, 308–313. doi:10.1016/j.cclet.2021.07.018
Isaacs, W. B., Carter, B. S., and Ewing, C. M. (1991). Wild-type p53 suppresses growth of human prostate cancer cells containing mutant p53 alleles. Cancer Res. 51, 4716–4720.
Kaspers, G. J., Pieters, R., Van Zantwijk, C. H., VanWering, E. R., Van Der Does-Van Den Berg, A., and Veerman, A. J. (1998). Prednisolone resistance in childhood acute lymphoblastic leukemia: Vitro-vivo correlations and cross-resistance to other drugs. Blood 92, 259–266. doi:10.1182/blood.V92.1.259.413k21_259_266
Kaspers, G. J., Veerman, A. J., Pieters, R., Van Zantwijk, C. H., Smets, L. A., Van Wering, E. R., et al. (1997). In vitro cellular drug resistance and prognosis in newly diagnosed childhood acute lymphoblastic leukemia. Blood 90, 2723–2729. doi:10.1182/blood.V90.7.2723.2723_2723_2729
Kinoyama, I., Taniguchi, N., Kawaminami, E., Nozawa, E., Koutoku, H., Furutani, T., et al. (2005). N-arylpiperazine-1-carboxamide derivatives: A novel series of orally active nonsteroidal androgen receptor antagonists. Chem. Pharm. Bull. 53, 402–409. doi:10.1248/cpb.53.402
Kinoyama, I., Taniguchi, N., Yoden, T., Koutoku, H., Furutani, T., Kudoh, M., et al. (2004). Synthesis and pharmacological evaluation of novel arylpiperazine derivatives as nonsteroidal androgen receptor antagonists. Chem. Pharm. Bull. 52, 1330–1333. doi:10.1248/cpb.52.1330
Lack, N. A., Axeriocilies, P., Tavassoli, P., Han, F. Q., Chan, K. H., Feau, C., et al. (2011). Targeting the binding function 3 (BF3) site of the human androgen receptor through virtual screening. J. Med. Chem. 54, 8563–8573. doi:10.1021/jm201098n
Lee, Y. B., Gong, Y. D., Yoon, H., Ahn, C. H., Jeon, M. K., and Kong, J. Y. (2010). Synthesis and anticancer activity of new 1-[(5 or 6-substituted 2-alkoxyquinoxalin-3-yl)aminocarbonyl]-4-(hetero)arylpiperazine derivatives. Bioorg. Med. Chem. 18, 7966–7974. doi:10.1016/j.bmc.2010.09.028
Leopoldo, M., Lacivita, E., Passafiume, E., Contino, M., Colabufo, N. A., Berardi, F., et al. (2007). 4-[ω-[4-Arylpiperazin-1-yl]alkoxy]phenyl)imidazo[1, 2-a]pyridine Derivatives: fluorescent high-affinity dopamine D3 receptor ligands as potential probes for receptor visualization. J. Med. Chem. 50, 5043–5047. doi:10.1021/jm070721+
Lin, H. H., Wu, W. Y., Cao, S. L., Liao, J., Ma, L., Gao, M., et al. (2013). Synthesis and antiproliferative evaluation of piperazine-1-carbothiohydrazide derivatives of indolin-2-one. Bioorg. Med. Chem. Lett. 23, 3304–3307. doi:10.1016/j.bmcl.2013.03.099
Liu, C., Zhu, Y., Lou, W., Nadiminty, N., Chen, X., Zhou, Q., et al. (2013b). Functional p53 determines docetaxel sensitivity in prostate cancer cells. Prostate 73, 418–427. doi:10.1002/pros.22583
Liu, W. H., Chang, J. X., Liu, Y., Luo, J. W., and Zhang, J. W. (2013a). Design, synthesis and activities of novel benzothiazole derivatives containing arylpiperazine. Acta Pharm. Sin. 48, 1259–1265. doi:10.16438/j.0513-4870.2013.08.002
Mashimo, T., Bandyopadhyay, S., Goodarzi, G., Watabe, M., Pai, S. K., Gross, S. C., et al. (2000). Activation of the tumor Metastasis suppressor gene, KAI1, by Etoposide is mediated by p53 and c-jun genes. Biochem. Biophys. Res. Commun. 274, 370–376. doi:10.1006/bbrc.2000.3139
Qi, Y. H., Chen, H., Chen, S. J., Shen, J. L., and Li, J. G. (2022). Synthesis, bioactivity, and molecular docking of novel arylpiperazine derivatives as potential AR antagonists. Front. Chem. 10, 947065. doi:10.3389/fchem.2022.947065
Romeiro, L. A., Da Silva Ferreira, M., Da Silva, L. L., Castro, H. C., Miranda, A. L., ilva, S. C. L., et al. (2011). Discovery of LASSBio-772, a 1, 3-benzodioxole N-phenylpiperazine derivative with potent alpha 1A/D-adrenergic receptor blocking properties. Eur. J. Med. Chem. 46, 3000–3012. doi:10.1016/j.ejmech.2011.04.032
Rose, P. W., Beran, B., Bi, C., Bluhm, W. F., Dimitropoulos, D., Goodsell, D. S., et al. (2011). The RCSB protein data bank: Redesigned web site and web services. Nucleic Acids Res. 39, D392–D401. doi:10.1093/nar/gkq1021
Shen, W. C., Hu, T. T., Liu, X. Y., Zha, J. J., Meng, F. Q., Wu, Z. K., et al. (2022). Defect engineering of layered double hydroxide nanosheets as inorganic photosensitizers for NIR-III photodynamic cancer therapy. Nat. Commun. 13, 3384. doi:10.1038/s41467-022-31106-9
Tannock, I. (1978). Cell kinetics and chemotherapy: A critical review. Cancer Treat. Rep. 62, 1117–1133.
Trott, O., and Olson, A. J. (2010). AutoDock Vina: Improving the speed and accuracy of docking with a new scoring function, efficient optimization, and multithreading. J. Comput. Chem. 31, 455–461. doi:10.1002/jcc.21334
Vinaya, K., Kavitha, C. V., Chandrappa, S., Prasanna, D. S., Raghavan, S. C., and Rangappa, K. S. (2011). Synthesis and antileukemic activity of novel 4-(3-(piperidin-4-yl) propyl)piperidine derivatives. Chem. Biol. Drug Des. 78, 622–630. doi:10.1111/j.1747-0285.2011.01184.x
Xu, F., Chen, H., He, X. L., Xu, J. Y., Xu, B. B., Huang, B. Y., et al. (2014). Identification of two novel α1-AR agonists using a high-throughput screening model. Molecules 19, 12699–12709. doi:10.3390/molecules190812699
Xu, F., Chen, H., Xu, J. Y., Liang, X., He, X. L., Shao, B. H., et al. (2015). Synthesis, structure-activity relationship and biological evaluation of novel arylpiperzines as α1A/1D-AR subselective antagonists for BPH. Bioorg. Med. Chem. 23, 7735–7742. doi:10.1016/j.bmc.2015.11.020
Xu, X., Ge, R., Li, L., Wang, J., Lu, X., Xue, S., et al. (2018). Exploring the tetrahydroisoquinoline thiohydantoin scaffold blockade the androgen receptor as potent anti-prostate cancer agents. Eur. J. Med. Chem. 143, 1325–1344. doi:10.1016/j.ejmech.2017.10.031
Yap, T. A., Smith, A. D., Ferraldeschi, R., Al-Lazikani, B., Workman, P., and de Bono, J. S. (2016). Drug discovery in advanced prostate cancer: Translating biology into therapy. Nat. Rev. Drug Discov. 15, 699–718. doi:10.1038/nrd.2016.120
Zhou, Z., Wang, Y. L., Peng, F., Meng, F. Q., Zha, J. J., Ma, L., et al. (2022). Intercalation-activated layered MoO3 nanobelts as biodegradable nanozymes for tumor-specific photo-enhanced catalytic therapy. Angew. Chem. Int. Ed. Engl. 61, e202115939. doi:10.1002/anie.202115939
Keywords: hydroxyzine derivatives, cytotoxic activity, antagonistic activity, docking study, AR antagonists
Citation: Qi Y, Xue B, Chen S, Wang W, Zhou H and Chen H (2022) Synthesis, biological evaluation, and molecular docking of novel hydroxyzine derivatives as potential AR antagonists. Front. Chem. 10:1053675. doi: 10.3389/fchem.2022.1053675
Received: 26 September 2022; Accepted: 20 October 2022;
Published: 03 November 2022.
Edited by:
Yu Huo, Sichuan Normal University, ChinaReviewed by:
Hang Gao, Taiyuan University of Technology, ChinaBingchao Qiang, Baiyao Group Co. Ltd. New medicine R&D, China
Copyright © 2022 Qi, Xue, Chen, Wang, Zhou and Chen. This is an open-access article distributed under the terms of the Creative Commons Attribution License (CC BY). The use, distribution or reproduction in other forums is permitted, provided the original author(s) and the copyright owner(s) are credited and that the original publication in this journal is cited, in accordance with accepted academic practice. No use, distribution or reproduction is permitted which does not comply with these terms.
*Correspondence: Haifeng Zhou, emhvdWhmQGN0Z3UuZWR1LmNu; Hong Chen, Y2hlbndleHBvQHNpbmEuY29t