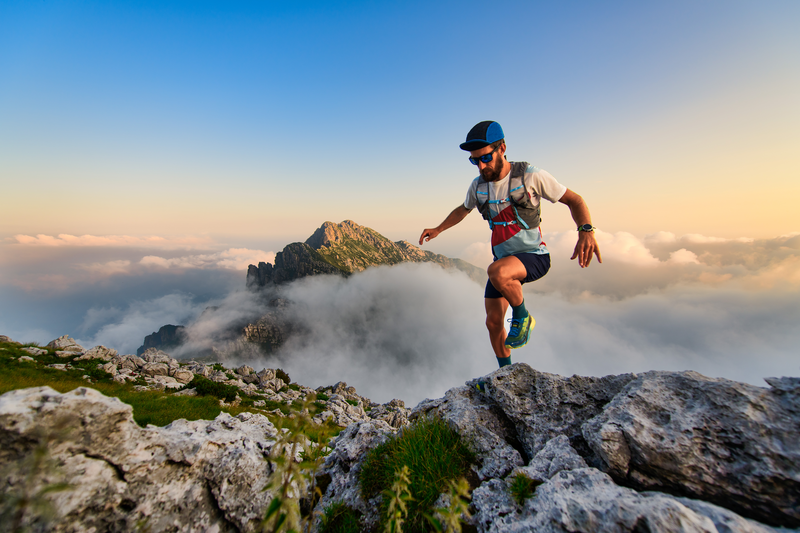
95% of researchers rate our articles as excellent or good
Learn more about the work of our research integrity team to safeguard the quality of each article we publish.
Find out more
ORIGINAL RESEARCH article
Front. Chem. , 04 January 2023
Sec. Medicinal and Pharmaceutical Chemistry
Volume 10 - 2022 | https://doi.org/10.3389/fchem.2022.1040216
Cyclization and glycosylation serve as effective approaches for enhancing the drug properties of peptides. Distinct from typical glycosylation, atypical arginine N-glycosylation has drawn increasing attention due to its fundamental role in various cellular procedures and signaling pathways. We previously developed a robust strategy for constructing arginine N-glycosylated peptides characterized by silver-promoted solid-phase guanidinylation. Modeled after cyclic octapeptide Samoamide A, an antitumor peptide composed of eight hydrophobic amino acids extracted from cyanobacteria, herein we first performed arginine scanning to determine an optimal position for replacement with arginine. Consequently, the first synthesis of arginine glycosylated Samoamide A cyclopeptide analogue was described combining solid-phase glycosylation with solution-phase cyclization. The resultant SA-HH-TT displayed enhanced water solubility compared with the non-glycosylated SA-HH-TT. Notably, our method provides a universal strategy for synthesizing arginine N-glycosylated cyclopeptides.
Polypeptide drugs, a research hotspot in recent years, have been marketed as approximately 80 therapeutics worldwide due to their various biological activities, strong receptor affinity, low toxicity and low accumulation (Du QS et al., 2015; Muttenthaler et al., 2021), such as the well-known cyclosporin (Fahr, 1993), GLP-1 receptor agonists (Nauck et al., 2021) exenatide, liraglutide, lixiniatide, and the recently approved cyclopeptide drug Pegcetacoplan (Hoy, 2021). However, problems exist, including their short half-life and suboptimal stability, such as their susceptibility to proteolytic degradation, and their sensitivity to acid, alkali, high temperature or organic solvents can deactivate them (Smart et al., 2014). These defects have become tremendous obstacles to the clinical application of peptide drugs, therefore, peptide modification has been utilized as a top priority in the fight against the above shortcomings.
The common modification methods include modification of both ends of peptides, such as amidation and methylation (Chatterjee et al., 2013; Mura et al., 2016; Alonso-Bastida and Encarnacion-Guevara, 2019); introduction of non-toxic, non-immunogenic and highly water-soluble PEG groups (Pasut and Veronese, 2012); and cyclization strategies (Craik, 2006; Zorzi et al., 2017). Cyclic peptides are often more rigid than linear peptides and thus have strong resistance to the digestive system; they also have a large specific surface area, which provides high affinity and selectivity for protein targets.
Glycosylation is also one of the common modification methods. The composition of glycosyl can affect its key pharmacological and pharmacokinetic properties in terms of water solubility, stability or biological activity (Johnson et al., 2014). Almost all natural glycosylation modifications can be classified as N- or O-glycosides, among which O-glycosides are mainly linked to the hydroxyl groups of serine and threonine. In addition, a small amount of O-glycosylation occurs on tyrosine residues (Iwashkiw et al., 2013). The main connection mode of N-glycosides is that N-acetyl glucosamine is connected with the side chain of asparagine. It was later discovered that the NleB family of type III secretory system effector proteins from enteropathogenic Escherichia coli (EPEC) can induce a novel posttranslational modification of N-glycosylated proteins, arginine-N-acetyl glucosamine (Arg-N-GlcNAc), which can act on host death receptors, such as TNFR and FAS, and their connectors, such as FADD, TRADD, and RIPK1, and inhibit the death receptor-induced apoptosis and necrosis of host cells by blocking the formation of the DISC (death inducing signaling complex) complex mediated by the death domain (Li et al., 2013; Pearson et al., 2013; Ding et al., 2019).
In the method of chemical synthesis of glycopeptides, O-linked glycopeptides are mainly constructed by glycosylated amino acids and then introduced into polypeptides. For N-linked glycopeptides, the main research is focused on the glycosylation of asparagine. In addition to using preformed glycosyl amino acids to synthesize glycopeptides, we can also use convergence strategies to directly glycosylate peptide chains to produce glycosylated peptides, called Lansbury’s aspartylation (Li and Zhu, 2016; Lin and Liu, 2020). Later, with the discovery that arginine can also be induced glycosylation, researchers also began to study the method of synthesizing arginine glycosylation. Pan et al. (2014) developed a robust strategy for constructing Arg N-glycosylated peptides, which was characterized by the use of chemical synthesis to obtain feasible S-alkyl-isothiourea glycosyl donors and then through silver-promoted solid-phase guanidine acylation to synthesize Arg N-GlcNAc peptides. This method is suitable for the efficient preparation of glycopeptides at single or multiple Arg-GlcNAc sites. Similarly, Xiang Li and his partners used this silver-promoted solid-phase synthesis method to successfully obtain a kind of anti-rhamnosyl arginine specific antibody, which was able to diagnose infections caused by pathogens, such as P. aeruginosa or N. meningitidis (Li et al., 2016). Not only that, this method was also applied to the fully synthetic TRADD death domain (residues 195-312) with Arg235 N-GlcNAcylation. Wu et al. (2020) first obtained two longish peptidyl fragments of the wild-type primary sequence by microwave-assisted efficient solid-phase synthesis (SPPS). The N-GlcNAcylated portion was constructed by total synthesis and bound to the resin of an unprotected ornithine residue to achieve guanidinylation directly on the resin via a silver-promoted method. Then, the two fragments were linked by hydrazide-based native chemical ligation (NCL) to obtain Arg-GlcNAc TRADD (195-312). In addition to the synthesis of arginine-N-glycosylated glycopeptides, this method can also be applied to the synthesis of other peptides, such as stictamide A. According to retrosynthetic analysis, researchers found that it was not easy to obtain by either the liquid or solid-phase methods because of the N-prenyl-modified arginine. Therefore, inspired by the arginine-N-glycosylated glycopeptide synthesis method, they finally chose to directly synthesize the amino acid side chains on the solid-phase carrier, and the silver-promoted solid-phase synthesis method successfully synthesized stictamide A (Li et al., 2015). However, the application of silver-promoted solid-phase guanidinylation in cyclic peptides has not yet been reported.
Samoamide A Figure 1 is an antitumor peptide consisting of eight hydrophobic amino acids extracted from cyanobacteria (Naman et al., 2017). Herein, using it as a template, after determining its inactive site by arginine scanning, the silver-promoted solid-phase arginine glycosylation method was applied to the cyclic peptide for the first time (Figure 2), and we designed and synthesized a series of Samoamide A derivatives and measured their activity to identify an excellent drug candidate for a novel antitumor drug.
2-Chlorotrityl chloride resin (0.53 mmol·g−1 loading) was purchased from Jiangsu Shenlang Biotech Co., (Jiangsu, China). Fmoc-protected amino acids were purchased from Shanghai GL Biochem Ltd., and Jiangsu Shenlang Biotech Co., (Shanghai and Jiangsu, China). Phenol, DIC, TIPS, DIEA, HoBt, PyBOP, and HCTU were obtained from Adamas-beta (Shanghai, China). DMF, DCM, petroleum ether, ethyl ether, AgNO3, TFA, piperidine and other common reagents were purchased from CHRON Chemical Co., Ltd., (Chengdu, China). HCT116 cells were obtained from Shanghai Cellular Institute of Chinese Academy of Sciences (Shanghai, China). The CCK-8 value-added test kit was purchased from Shanghai Tongren Group (Shanghai, China). RPMI-1640, phosphate buffer solution, 0.25% trypsin, penicillin–streptomycin solution, and fetal bovine serum were purchased from Gibco Thermo Fisher Scientific (United States).
First, 2-chlorotrityl chloride resin (0.75 g, 0.53 mmol·g−1 loading capacity) was swollen with DCM (10 ml) for 25 min, mixed with Fmoc-Val-OH (2 mmol), DIEA (6 mmol) and DCM (8 ml), and added to the resin for 2 h to complete the coupling of the first amino acid, followed by washing with DMF (5 times), DCM (5 times) and DMF (2 times) to afford 1a. Then, the resin was treated with methanol for 20 min to block the residual active sites of the resin. Afterward, we deprotected Fmoc with 20% piperidine in DMF twice for 10 min every time to afford 1b. The steps of coupling, deprotection, and washing were repeated until all the amino acids of the Samoamide A sequence we designed were coupled to the resin to afford 1c. Reagents (88% TFA, 5% H2O, 5% phenol and 2% TIPS) were reacted at room temperature for 4 h to disconnect the crude straight-chain peptide from the resin. After filtering, a large amount of ethyl ether was added to crystallize and then centrifuged to obtain crude linear peptide 1d. PyBOP (3 eq), HoBt (3 eq) and DIPEA (6 eq) were dissolved in DCM and slowly added to the DCM solution of the peptide at 0 °C and stirred at room temperature for 14 h to afford crude oily Samoamide A 1e. Representative HPLC, HR-MS and NMR data are shown, and complete data can be found in the Supplementary Materials.
Cyclic peptides were purified by preparative high-performance liquid chromatography with a reverse C18 column and gradient elution (mobile phase A was 0.1% TFA in acetonitrile; mobile phase B was 0.1% TFA in water). SA-HH-0 [Cyclo-(VFPIFPPL)]. 58 mg, 15% purified yield. ESI-MS m/z calcd. For C50H70N8O8 910.53; found [M + H]+ = 911.53; [M + Na]+ = 933.51.
The synthesis method of straight-chain peptide was the same as that of SA-HH-0, but the cleavage reagent was different. After obtaining Compound 2a, reagent (20% TFE, 80% DCM) was used to cleave the linear peptide. After concentration, a large amount of petroleum ether was added to crystallize, and then the liquid was removed by rotary steaming to obtain crude linear peptide 2b. PyBOP (3 eq), HoBt (3 eq) and DIPEA (6 eq) were dissolved in DCM, and the DCM solution of the peptide was slowly added to the DCM solution of PyBOP/HoBt/DIPEA at 0 °C and stirred at room temperature for 14 h to afford 2c. Then, the side chain protective group was removed, the reagents TFA and DCM were added, the volume ratio was 1:3, the reaction time was 4 h at room temperature, and we obtained the crude oily derivative 2d. The purification method was the same as that for SA-HH-0. Representative HPLC, HR-MS and NMR data are shown, and complete data can be found in the Supplementary Materials.
SA-HH-1 [Cyclo-(RFPIFPPL)]. 12 mg, 6% purified yield. ESI-MS m/z calcd. For C51H73N11O8 967.56; found [M + H]+ = 968.56. SA-HH-2 [Cyclo-(VRPIFPPL)]. 8 mg, 4% purified yield. ESI-MS m/z calcd. For C47H73N11O8 919.56; found [M + H]+ = 920.56. SA-HH-3 [Cyclo-(VFRIFPPL)]. 10 mg, 5% purified yield. ESI-MS m/z calcd. For C51H75N11O8 969.568; found [M + H]+ = 970.57. SA-HH-4 [Cyclo-(VFPRFPPL)]. 12 mg, 6% purified yield. ESI-MS m/z calcd. For C50H71N11O8 953.53; found [M + H]+ = 954.55. SA-HH-5 [Cyclo-(VFPIRPPL)]. 15 mg, 8% purified yield. ESI-MS m/z calcd. For C47H73N11O8 919.56; found [M + H]+ = 920.49. SA-HH-6 [Cyclo-(VFPIFRPL)]. 20 mg, 10% purified yield. ESI-MS m/z calcd. For C51H75N11O8 969.58; found [M + H]+ = 970.58. SA-HH-7 [Cyclo-(VFPIFPRL)]. 23 mg, 10% purified yield. ESI-MS m/z calcd. For C51H75N11O8 969.58; found [M + H]+ = 970.58. SA-HH-8 [Cyclo-(VFPIFPPR)].18 mg, 9% purified yield. ESI-MS m/z calcd. For C50H71N11O8 953.53; found [M + H]+ = 954.55.
We also synthesized the linear peptide of SA-HH-TT according to the method of solid-phase synthesis to afford 3a and then used a reagent (10 ml DMF containing 2% NH2NH2) to remove the protective base Dde for 6 min in a constant temperature shaker at 30°C; this process was repeated 3 times. The samples were washed with DMF 3 times to afford 3b. After dissolving the glycosyl donor we prepared previously (0.6 mmol) and AgNO3 (0.6 mmol) with an appropriate amount of anhydrous DMF, Et3N (2 mmol) was added and reacted at room temperature for more than 12 h. After the reaction, the mixture was washed with DMF 5 times, MeOH 5 times and DCM 2 times. Linear glycopeptide 3c was cleaved with reagent (88% TFA, 5% H2O, 5% phenol and 2% TIPS) in the same way as arginine-scanned Samoamide A derivatives. After filtration, a large amount of ice diethyl ether was added for crystallization, centrifuged and blown with nitrogen to obtain crude linear peptide 3d. Then, using the same method as for 1e, cyclization yielded 3e. It was necessary to further remove the acetyl protective group from the glycosyl donor with methanol sodium methanol solution, react it at room temperature for 1 h, adjust the pH to neutral with dilute hydrochloric acid, and concentrate it to obtain glycosylated crude Samoamide A 3f. The purification method was the same as that for the other derivatives. Representative HPLC, HR-MS and NMR data are shown, and complete data can be found in the Supplementary Materials.
SA-HH-TT [Cyclo-(VFPIFPR(GlcNAc)L)]. 46 mg, 20% purified yield. ESI-MS m/z calcd. For C59H88N12O13 1,172.66; found [M + H]+= 1,173.67; [M + Na]+= 1,195.65.
The human colon cancer cell line HCT116 was maintained in RPMI-1640 medium supplemented with 10% heat-inactivated FBS and 1% penicillin‒streptomycin at 37°C, 5% CO2, and complete humidification conditions. Cells (5 × 103 cells/well) were inoculated in 96-well plates and treated with different concentrations of polypeptides for 48 h. The absorbance at 450 nm was then measured by adding the CCK8 kit, and the percentage of cell survival was calculated based on the ratio of the sample well to the reference well OD450 (Adan et al., 2016).
Samoamide A was precisely weighed, and 1 mg was placed in a 10 ml centrifuge tube and diluted to scale with 95% ethanol. Then, 0, 200, 400, 600, 800, 1,000 μl aliquots were pipetted in a 5 ml centrifuge tube and adjusted to 1 ml with 95% ethanol, and the aqueous solutions were measured on a scale, sonicated for 5 min, and placed at room temperature. An additional 1 ml of 95% ethanol was added, and water was added to the same method as the blank. The absorbance values were measured at 214 nm to obtain the linear regression equation of the standard curve.
Sufficient amounts of Samoamide A and its glycosylated derivatives were dissolved in aqueous solutions at a constant temperature of 25°C, sonicated for approximately 10 min, centrifuged and allowed to settle to remove the insoluble matter from the solution, and the upper layer of supernatant was taken, that is, the saturated solution. After the saturated solution was properly diluted, its absorbance value was measured at 214 nm, and the concentration of the saturated solution was calculated by substituting the above equation (Chevalier et al., 2001).
Samoamide A is cytotoxic to a variety of cancer cells, including non-small cell lung cancer cells and colorectal cancer cells (Naman et al., 2017; Chang et al., 2019). However, its low content and poor water solubility in nature limit its application in the development of new drugs. Hence, we manually synthesized Samoamide A by solid-phase synthesis (Figure 3). Using 2-chlorotrityl chloride resin as the carrier and HCTU and DIEA as the condensation system, the straight-chain peptide was synthesized by solid-phase synthesis. Then, PyBOP, HoBt, and DIPEA were used as cyclization systems and cyclized in DCM solution. Finally, we obtained Samoamide A, and its relative molecular weight was determined by mass spectrometry (Table 1).
Then, we scanned the cyclic octapeptide using arginine because the introduction of cationic amino acids can improve the properties of the peptide, but more importantly, to identify its inactive site and introduce arginine to guarantee further arginine-N-glycosylation. Taking the synthesis of SA-HH-1 as an example, the synthesis route is shown in Figure 4. Using 2-chlorotrityl chloride resin as the carrier and HCTU and DIEA as the condensation system, the arginine-scanned straight-chain peptide was synthesized by solid-phase synthesis. Then, PyBOP, HoBt, and DIPEA were used as cyclization systems and cyclized in DCM solution. Finally, we successfully obtained eight derivatives (Figure 5), and their relative molecular weights were determined by mass spectrometry (Table 1). Since Arg has two amino groups, when cyclizing, it should be noted that we need to retain the protective group of the side chain amino group and then remove the amino protection group of the arginine side chain group after cyclization so that the amino group at the end of the peptide chain is correctly connected to the carboxyl group; therefore, we chose TFE (Muhajir et al., 2021).
When we identified the inactive site, we decided to further modify the arginine glycosylation at this site (Figure 5). Using the SSG method, we attached the preprepared S-alkyl isothiocyanate glycosyl donor (Figure 6) to ornithine, assembled the two parts, and achieved arginine glycosylation modifications (Figure 7). We confirmed the target product by mass spectrometry. In the process of obtaining the product, we found that in accordance with the Fmoc solid-phase synthesis method, when removing the ornithine side chain protection group, the use of NH2NH2 will also shed the Fmoc protection group of the amino acid at the end of the peptide chain so that the glycosyl donor cannot be correctly connected to the corresponding position. Using NH2OH HCl and imidazole (Díaz-Mochón et al., 2004), we were able to remove only the Dde protective group and retain the Fmoc protective group. After glycosylation, cyclization was carried out after the Fmoc protective base was removed. However, this method is cumbersome; therefore, we found another way to replace the last amino acid of the coupling with the amino acid protected by Boc because the Boc protective group is sensitive to acidity but is not sensitive to the alkaline environment. Therefore, when using NH2NH2, the Boc protective group will not fall off. When cutting the resin after glycosylation, the TFA can remove the Boc, and then the glycosylated straight chain is successfully cyclized. Similarly, to ensure the correct cyclization of cyclic peptides, we removed the hydroxyl protective acetyl group from the glycosyl donor after cyclization.
At present, the method of arginine glycosylation is roughly divided into two types: one is to construct glycosyl arginine and then introduce it into the solid-phase synthesis; the other is the direct solid-phase glycosylation of the peptide side chain functional group after the synthesis of the peptide chain (Wang et al., 2017; Rodriguez-Mayor et al., 2020). The limitation of the former is that after the construction of glycosyl arginine, a tedious purification step is needed, which can lead to a significant reduction in yield; the reaction also introduces Hg2+ harmful to the human body, which may limit the application of this method to arginine glycosylation (Rodriguez-Mayor et al., 2020). In the latter, in addition to the method described herein, silver-promoted direct solid-phase glycosylation is performed to obtain arginine glycosylated peptides (Pan et al., 2014); Yang et al. (2021) used palladium complexes as catalysts to achieve stereoselective arginine glycosylation. Different from our method, we combined thiourea glycosides and ornithine-bearing peptides, and they constructed glycopeptides directly from natural arginine peptides. However, to obtain high-yield products, a variety of complex metal palladium catalysts are needed, in which Pd(PPh3)4 is used, which is difficult to preserve. Some palladium catalysts are expensive and may even cause harm to the environment and the human body, limiting the development of their applications.
The activity of the derivatives scanned by arginine was determined, and the inactive site of cyclic octapeptide was confirmed again (Figure 8). However, in the cytotoxicity test of arginine-glycosylated Samoamide A, we regrettably found that we did not obtain new compounds with better activity or the same activity as we expected (Figure 9), compared to the parent peptide, the activity decreased slightly. At 100 μM, the survival rate of cells treated with Samoamide A was 17%, while that treated with SA-HH-TT was 28%. We speculate that steric hindrance may fold the structure differently from the original structure, affecting its antitumor activity. However, the specific cause requires further confirmation. Representative activity data are shown, and complete data can be found in the Supplementary Materials.
In the water solubility experiment, the hydrophilic group was introduced into the original structure because of glycosylation modification, and we found that the water solubility of the product was significantly improved. After drawing and calculation, the linear regression equation of the standard curve was determined to be C = 0.4646A - 0.0201 (Figure 10). Then, we measured the absorbance value after dilution and calculated that the solubility increased from the original 0.73 mg/ml to the present 34.14 mg/ml. Representative solubility test data are shown, and complete data can be found in the Supplementary Materials.
To sum up, we designed and synthesized a series of Samoamide A derivatives, including eight cyclic peptide derivatives scanned by arginine and glycosylation of arginine to modify inactive sites. Although it failed to show better antitumor activity, its water solubility was greatly improved. However, it is worth noting that we provide a general method of arginine N-glycosylation to modify cyclic peptides, using silver to promote solid-phase glycosylation; the subsequent use of this method can also replace glycosyl donors of glycosyl cyclic peptides, such as galactose, glucose, and rhamnose, to explore whether different glycosyl donors are related to their activity. This method provides a modification strategy for improving the properties of peptides. It is believed that with the gradual deepening of research, polypeptide drugs will have brighter application prospects after improving their properties.
The datasets presented in this study can be found in online repositories. The names of the repository/repositories and accession number(s) can be found in the article/Supplementary Material.
XL, HL, and CS designed the experiments. BL and RL conducted the experiments. LL and MT revised the manuscript.
This work was supported by Key R & D projects of the Science and Technology Department of Sichuan Province (2019YFS0097).
The authors declare that the research was conducted in the absence of any commercial or financial relationships that could be construed as a potential conflict of interest.
All claims expressed in this article are solely those of the authors and do not necessarily represent those of their affiliated organizations, or those of the publisher, the editors and the reviewers. Any product that may be evaluated in this article, or claim that may be made by its manufacturer, is not guaranteed or endorsed by the publisher.
The Supplementary Material for this article can be found online at: https://www.frontiersin.org/articles/10.3389/fchem.2022.1040216/full#supplementary-material
Adan, A., Kiraz, Y., and Baran, Y. (2016). Cell proliferation and cytotoxicity assays. Curr. Pharm. Biotechno. 17, 1213–1221. doi:10.2174/1389201017666160808160513
Alonso-Bastida, R., and Encarnacion-Guevara, S. (2019). Proteomic insights into lysine acetylation and the implications for medical research. Expert Rev. Proteomics 16, 1–3. doi:10.1080/14789450.2019.1557050
Chang, Q., Li, Y. L., and Zhao, X. (2019). Total synthesis and cyclization strategy of samoamide A, a cytotoxic cyclic octapeptide rich in proline and phenlalanine isolated from marine cyanobacterium. J. Asian Nat. Prod. Res. 21, 171–177. doi:10.1080/10286020.2018.1450391
Chatterjee, J., Rechenmacher, F., and Kessler, H. (2013). N-Methylation of peptides and proteins: An important element for modulating biological functions. Angew. Chem. Int. Ed. Engl. 52, 254–269. doi:10.1002/anie.201205674
Chevalier, F., Chobert, J., Popineau, Y., Nicolas, M. G., and Haertlé, T. (2001). Improvement of functional properties of β-lactoglobulin glycated through the Maillard reaction is related to the nature of the sugar. Int. Dairy J. 11, 145–152. doi:10.1016/S0958-6946(01)00040-1
Craik, D. J. (2006). Seamless proteins tie up their loose ends. Science 311, 1563–1564. doi:10.1126/science.1125248
Díaz-Mochón, J. J., Bialy, L., and Bradley, M. (2004). Full orthogonality between Dde and Fmoc: The direct synthesis of PNA−Peptide conjugates. Org. Lett. 6, 1127–1129. doi:10.1021/ol049905y
Ding, J. J., Pan, X., Du, L. J., Yao, Q., Xue, J., Yao, H. W., et al. (2019). Structural and functional insights into host death domains inactivation by the bacterial arginine GlcNAcyltransferase effector. Mol. Cell. 74, 922–935.e6. doi:10.1016/j.molcel.2019.03.028
Du, Q. S., Xie, N. Z., and Huang, R. B. (2015). Recent development of peptide drugs and advance on theory and methodology of peptide inhibitor design. Med. Chem. 11, 235–247. doi:10.2174/1573406411666141229163355
Fahr, A. (1993). Cyclosporin clinical pharmacokinetics. Clin. Pharmacokinet. 24, 472–495. doi:10.2165/00003088-199324060-00004
Hoy, S. M. (2021). Pegcetacoplan: First approval. Drugs 81, 1423–1430. doi:10.1007/s40265-021-01560-8
Iwashkiw, J. A., Vozza, N. F., Kinsella, R. L., and Feldman, M. F. (2013). Pour some sugar on it: The expanding world of bacterial protein O-linked glycosylation. Mol. Microbiol. 89, 14–28. doi:10.1111/mmi.12265
Johnson, Q. R., Lindsay, R. J., Raval, S. R., Dobbs, J. S., Nellas, R. B., and Shen, T. Y. (2014). Effects of branched O-glycosylation on a semiflexible peptide linker. J. Phys. Chem. B 118, 2050–2057. doi:10.1021/jp410788r
Li, S., Zhang, L., Yao, Q., Li, L., Dong, N., Rong, J., et al. (2013). Pathogen blocks host death receptor signalling by arginine GlcNAcylation of death domains. Nature 501, 242–246. doi:10.1038/nature12436
Li, X., Krafczyk, R., Macošek, J., Li, Y., Zou, Y., Simon, B., et al. (2016). Resolving the α-glycosidic linkage of arginine-rhamnosylated translation elongation factor P triggers generation of the first ArgRha specific antibody. Chem. Sci. 7, 6995–7001. doi:10.1039/C6SC02889F
Li, X., Li, Y., Chen, Y., Zou, Y., Zhuo, X., Wu, Q., et al. (2015). A silver-promoted solid-phase guanidylation process enables the first total synthesis of stictamide A. RSC Adv. 5, 94654–94657. doi:10.1039/C5RA20976E
Li, X., and Zhu, J. (2016). Glycosylation via transition-metal catalysis: Challenges and opportunities. Eur. J. Org. Chem. 2016, 4724–4767. doi:10.1002/ejoc.201600484
Lin, J. D., and Liu, X. W. (2020). Recent development in ligation methods for glycopeptide and glycoprotein synthesis. Chem.-Asian J. 15, 2548–2557. doi:10.1002/asia.202000566
Muhajir, M. I., Hardianto, A., Al-Anshori, J., Sumiarsa, D., Mayanti, T., NurlelasariHarneti, D., et al. (2021). Total synthesis of nocardiotide A by using a combination of solid- and solution-phase methods. CHEMISTRYSELECT 6, 12941–12946. doi:10.1002/slct.202103441
Mura, M., Wang, J., Zhou, Y., Pinna, M., Zvelindovsky, A. V., Dennison, S. R., et al. (2016). The effect of amidation on the behaviour of antimicrobial peptides. Eur. Biophys. J. 45, 195–207. doi:10.1007/s00249-015-1094-x
Muttenthaler, M., King, G. F., Adams, D. J., and Alewood, P. F. (2021). Trends in peptide drug discovery. Nat. Rev. Drug Discov. 20, 309–325.
Naman, C. B., Rattan, R., Nikoulina, S. E., Lee, J., Miller, B. W., Moss, N. A., et al. (2017). Integrating molecular networking and biological assays to target the isolation of a cytotoxic cyclic octapeptide, samoamide A, from an American Samoan marine cyanobacterium. J. Nat. Prod. 80, 625–633. doi:10.1021/acs.jnatprod.6b00907
Nauck, M. A., Quast, D. R., Wefers, J., and Meier, J. J. (2021). GLP-1 receptor agonists in the treatment of type 2 diabetes - state-of-the-art. Mol. Metab. 46, 101102. doi:10.1016/j.molmet.2020.101102
Pan, M., Li, S., Li, X., Shao, F., Liu, L., and Hu, H. G. (2014). Synthesis of and specific antibody generation for glycopeptides with arginine N-GlcNAcylation. Angew. Chem. Int. Ed. 53, 14517–14521. doi:10.1002/anie.201407824
Pasut, G., and Veronese, F. M. (2012). State of the art in PEGylation: The great versatility achieved after forty years of research. J. Control. Release 161, 461–472. doi:10.1016/j.jconrel.2011.10.037
Pearson, J. S., Giogha, C., Ong, S. Y., Kennedy, C. L., Kelly, M., Robinson, K. S., et al. (2013). A type III effector antagonizes death receptor signalling during bacterial gut infection. Nature 501, 247–251. doi:10.1038/nature12524
Rodriguez-Mayor, A. V., Peralta-Camacho, G. J., Cardenas-Martinez, K. J., and Garcia-Castaneda, J. E. (2020). Development of strategies for glycopeptide synthesis: An overview on the glycosidic linkage. Curr. Org. Chem. 24, 2475–2497. doi:10.2174/1385272824999200701121037
Smart, A. L., Gaisford, S., and Basit, A. W. (2014). Oral peptide and protein delivery: Intestinal obstacles and commercial prospects. Expert Opin. Drug Deliv. 11, 1323–1335. doi:10.1517/17425247.2014.917077
Wang, S. Y., Corcilius, L., Sharp, P. P., and Payne, R. J. (2017). Synthesis of a GlcNAcylated arginine building block for the solid phase synthesis of death domain glycopeptide fragments. Bioorgan. Med. Chem. 25, 2895–2900. doi:10.1016/j.bmc.2017.03.012
Wu, Y., Li, Y., Cong, W., Zou, Y., Li, X., and Hu, H. (2020). Total synthesis of TRADD death domain with arginine N-GlcNAcylation by hydrazide-based native chemical ligation. Chin. Chem. Lett. 31, 107–110. doi:10.1016/j.cclet.2019.05.010
Yang, J., Dai, Y., Bartlett, R., and Zhang, Q. (2021). Convergent palladium-catalyzed stereospecific arginine glycosylation using glycals. Org. Lett. 23, 4008–4012. doi:10.1021/acs.orglett.1c01218
Keywords: cyclopeptide synthesis, arginine N-glycosylation, solid-phase glycosylation, Samoamide A, antitumor biological activity
Citation: Liang B, Li R, Li L, Tang M, Li X, Su C and Liao H (2023) Silver-promoted solid-phase guanidinylation enables the first synthesis of arginine glycosylated Samoamide A cyclopeptide analogue. Front. Chem. 10:1040216. doi: 10.3389/fchem.2022.1040216
Received: 09 September 2022; Accepted: 19 December 2022;
Published: 04 January 2023.
Edited by:
Kaushik Chanda, VIT University, IndiaReviewed by:
Michal Szostak, Rutgers University, Newark, United StatesCopyright © 2023 Liang, Li, Li, Tang, Li, Su and Liao. This is an open-access article distributed under the terms of the Creative Commons Attribution License (CC BY). The use, distribution or reproduction in other forums is permitted, provided the original author(s) and the copyright owner(s) are credited and that the original publication in this journal is cited, in accordance with accepted academic practice. No use, distribution or reproduction is permitted which does not comply with these terms.
*Correspondence: Xiang Li, eGlhbmdsaUBzbW11LmVkdS5jbg==; Chunli Su, MjIwOTQ5MTdAcXEuY29t; Hongli Liao, bGlhb2hsMjEzQDEyNi5jb20=
†These authors have contributed equally to this work
Disclaimer: All claims expressed in this article are solely those of the authors and do not necessarily represent those of their affiliated organizations, or those of the publisher, the editors and the reviewers. Any product that may be evaluated in this article or claim that may be made by its manufacturer is not guaranteed or endorsed by the publisher.
Research integrity at Frontiers
Learn more about the work of our research integrity team to safeguard the quality of each article we publish.