- Qiandongnan Engineering and Technology Research Center for Comprehensive Utilization of National Medicine, Kaili University, Kaili, China
In this study, using the botanical active components of carvacrol, thymol, guaiacol, and sesamol as the lead structures, 19 novel botanical active component derivatives containing carboxamide and 1,3,4-thiadiazole thioether moieties (5a−5s) were synthesized and structurally characterized by 1H NMR, 13C NMR, and HRMS. The antibacterial bioassay results in vitro showed that compound 2-(2-methoxyphenoxy)-N-(5-(methylthio)-1,3,4-thiadiazol-2-yl)acetamide (5k) revealed excellent inhibitory activities against Xanthomonas axonopodis pv. citri (Xac) and Xanthomonas oryzae pv. oryzicolaby (Xoc), with the median effective concentration (EC50) values of 22 and 15 μg/ml, respectively, which were even better than those of thiodiazole copper and bismerthiazol. Meanwhile, all the target compounds revealed lower in vitro inhibitory effects on Mucor bainieri (M. bainieri), Mucor fragilis (M. fragilis), and Trichoderma atroviride (T. atroviride), than carbendazim.
1 Introduction
As a serious threat to agricultural production, plant diseases can cause huge economic losses every year (Rosegrant and Cline, 2003; Neeraja et al., 2010; Opara, 2013; Bhattacharjee and Dey, 2014). Although the use of pesticides is an effective method to control plant diseases, the frequent use of traditional pesticides can lead to many negative effects such as pathogenic microorganism resistance, environmental contamination, and human health (Guo et al., 1998). As the improving of human living level and the demand for high-quality agricultural products, a limit on the use of traditional pesticides is required (Chávez-Dulanto et al., 2021).
In the 21st century and beyond, use of natural product pesticides to control plant diseases is an innovative approach of sustainable agricultural development (Cantrell et al., 2020; Souto et al., 2021). It is a critical approach to find new active components and to develop new pesticides by modifying the structure of natural products. Botanical active components of carvacrol, thymol, guaiacol, and sesamol (Figure 1) had a broad spectrum of pesticide biological properties, such as antifungal and insecticidal activity (Shen and He, 2022; Cui et al., 2022; Jia et al., 2007; Sharifi-Rad et al., 2018; Karina Kachur, 2020; Rathod et al., 2021). However, the inhibitory effects on plant pathogenic bacteria diseases of carvacrol, thymol, guaiacol, sesamol and their derivative had not been reported yet. Meanwhile, the carboxamide and 1,3,4-thiadiazole thioether moieties had extensive pesticide biological activities, including antibacterial, antifungal, antiviral, and insecticidal activity (Dalgaard, et al., 1994; Wu et al., 2016; Yang et al., 2018; Chen, et al., 2019; Yang et al., 2019; Tang et al., 2020; Chen et al., 2021). In our previous work, a series of novel thiochromanone derivatives containing carboxamide and 1,3,4-thiadiazole thioether moieties (Figure 2) were prepared and demonstrated to have suitable antibacterial and antifungal activity (Yu et al., 2020).
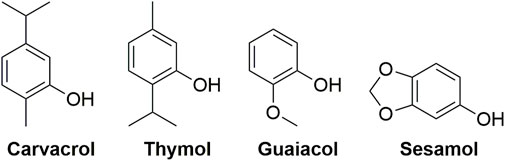
FIGURE 1. The structures of botanical active components of carvacrol, thymol, guaiacol, and sesamol.
To develop new lead compounds, in this study, we aimed to replace thiochromanone structure in the structure of our reported structures by carvacrol, thymol, guaiacol, and sesamol structures to build some new botanical active component derivatives containing carboxamide and 1,3,4-thiadiazole thioether moieties (Figure 2).
2 Materials and methods
2.1 Chemical synthesis
2.1.1 Preparation of intermediates 2 and 4
As shown in Scheme 1, using the botanical active components of carvacrol, thymol, guaiacol, and sesamol as the lead structures, intermediates 2 and 4 were prepared using the methods that have been previously reported (Friedrich et al., 2020; Yu et al., 2022).
2.1.2 Preparation of the target compounds 5a−5s
To a 25 ml round bottom flask, intermediates 2 (20 mmol) and 4 (20 mmol) dissolved in DMF (10 ml), DMAP (2 mmol), and EDCI (30 mmol) were added. After reacting overnight at room temperature, the precipitates obtained by adding distilled water (50 ml) were recrystallized from ethyl acetate to give the target compounds 5a−5s.
2.2 Bioactivity evaluation
The preliminary inhibitory effects results in vitro of compounds 5a−5s against Xanthomonas axonopodis pv. citri (Xac) and Xanthomonas oryzae pv. oryzicolaby (Xoc) as well as Mucor bainieri (M. bainieri), Mucor fragilis (M. fragilis), and Trichoderma atroviride (T. atroviride) were determined by the turbidimeter test (for antibacterial activity test) and mycelial growth rate method (for antifungal activity test) (Schaad et al., 1996; Wang et al., 2022). Meanwhile, the median effective concentration (EC50) values of compounds 5a, 5b, 5f, 5k, 5L, and 5n against Xac and Xoc were calculated using the SPSS 19.0 software (SPSS, Chicago, United States).
3 Results and discussion
3.1 Chemistry
Using the botanical active components of carvacrol, thymol, guaiacol, and sesamol as the lead structures, compounds 5a−5s were prepared in three steps, namely, substitution, thioetherification, and condensation reaction, with the yields of 68%–88% and the melting point ranges within two degrees centigrades. In the 1H NMR spectra of compounds 5a−5s, a singlet at 12.87–12.79 and 4.93–4.81 ppm indicated H atom in CONH and OCH2 groups, respectively. Meanwhile, a singlet at 168.07–167.79 ppm in the 13C NMR spectra indicated C atom in CONH group. In addition, the molecular weights of compounds 5a−5s were assigned by combining the [M + Na]+ ions with the confidence level of 100%. The physical and chemical properties and spectra data for compounds 5a−5s are presented in the following.
2-(5-Isopropyl-2-methylphenoxy)-N-(5-(methylthio)-1,3,4-thiadiazol-2-yl)acetamide (5a). White solid, yield 77%, mp 152–154°C; 1H NMR (400 MHz, DMSO-d6) δ (ppm): 12.85 (s, 1H), 7.06 (d, J = 8.0 Hz, 1H), 6.75 (d, J = 8.0 Hz, 1H), 6.72 (s, 1H), 4.93 (s, 2H), 2.83–2.76 (m, 1H), 2.72 (s, 3H), 2.99 (s, 3H), 1.15 (s, 3H), 1.13 (s, 3H); 13C NMR (100 MHz, DMSO-d6) δ (ppm): 167.99, 161.19, 158.17, 156.19, 147.85, 130.90, 123.95, 119.18, 110.14, 66.82, 33.77, 24.35, 16.38, 16.14; Anal. calcd. for m/z of C15H19N3O2S2 (HRMS [M + Na]+): 360.08109, found: 360.08046.
N-(5-(ethylthio)-1,3,4-thiadiazol-2-yl)-2-(5-isopropyl-2-methylphenoxy) acetamide (5b). White solid, yield 74%, mp 130–131°C; 1H NMR (400 MHz, DMSO-d6) δ (ppm): 12.86 (s, 1H), 7.06 (d, J = 8.0 Hz, 1H), 6.75 (d, J = 8.0 Hz, 1H), 6.71 (s, 1H), 4.93 (s, 2H), 3.23 (q, J1 = 8.0 Hz, J2 = 16.0 Hz, 2H), 2.83–2.76 (m, 1H), 2.18 (s, 3H), 1.34 (t, J = 8.0 Hz, 3H), 1.15 (s, 3H), 1.13 (s, 3H); 13C NMR (100 MHz, DMSO-d6) δ (ppm): 168.04, 159.49, 158.53, 156.18, 147.86, 130.91, 123.94, 119.18, 110.15, 66.82, 33.76, 28.52, 24.35, 16.15, 15.17; Anal. calcd. for m/z of C16H21N3O2S2 (HRMS [M + Na]+): 374.09674, found: 374.09643.
N-(5-(benzylthio)-1,3,4-thiadiazol-2-yl)-2-(5-isopropyl-2-methylphenoxy) acetamide (5c). White solid, yield 81%, mp 137–138°C; 1H NMR (400 MHz, DMSO-d6) δ (ppm): 12.86 (s, 1H), 7.40 (d, J = 8.0 Hz, 2H), 7.34–7.25 (m, 3H), 7.06 (d, J = 8.0 Hz, 1H), 6.75 (d, J = 8.0 Hz, 1H), 6.69 (s, 1H), 4.92 (s, 2H), 4.49 (s, 2H), 2.83–2.76 (m, 1H), 2.17 (s, 3H), 1.14 (s, 3H), 1.13 (s, 3H); 13C NMR (100 MHz, DMSO-d6) δ (ppm): 168.06, 158.90, 156.16, 147.85, 137.14, 130.91, 129.46, 129.01, 128.06, 123.91, 119.17, 110.11, 66.78, 38.02, 33.76, 24.35, 16.15; Anal. calcd. for m/z of C21H23N3O2S2 (HRMS [M + Na]+): 436.11239, found: 436.11185.
N-(5-((4-fluorobenzyl)thio)-1,3,4-thiadiazol-2-yl)-2-(5-isopropyl-2-methylphenoxy)acetamide (5d). Yellow solid, yield 86%, mp 140–141°C; 1H NMR (400 MHz, DMSO-d6) δ (ppm): 12.86 (s, 1H), 7.47–7.43 (m, 2H), 7.16 (d, J = 8.0 Hz, 2H), 7.05 (s, 1H), 6.75 (d, J = 8.0 Hz, 1H), 6.70 (s, 1H), 4.93 (s, 2H), 4.49 (s, 2H), 2.83–2.76 (m, 1H), 2.18 (s, 3H), 1.14 (s, 3H), 1.13 (s, 3H); 13C NMR (100 MHz, DMSO-d6) δ (ppm): 168.07, 161.98 (d, J = 243.0 Hz), 158.69, 158.16, 147.85, 133.50 (d, J = 3.0 Hz), 131.51 (d, J = 9.0 Hz), 130.91, 123.92, 119.18, 115.90, 115.69, 110.12, 66.80, 37.14, 33.76, 24.34, 16.14; Anal. calcd. for m/z of C21H22FN3O2S2 (HRMS [M + Na]+): 454.10297, found: 454.10241.
N-(5-((4-chlorobenzyl)thio)-1,3,4-thiadiazol-2-yl)-2-(5-isopropyl-2-methylphenoxy)acetamide (5e). Yellow solid, yield 74%, mp 132–134°C; 1H NMR (400 MHz, DMSO-d6) δ (ppm): 12.86 (s, 1H), 7.44–7.37 (m, 1H), 7.06 (d, J = 8.0 Hz, 1H), 6.75 (d, J = 8.0 Hz, 1H), 6.70 (s, 1H), 4.93 (s, 2H), 4.49 (s, 2H), 2.83–2.76 (m, 1H), 2.18 (s, 3H), 1.14 (s, 3H), 1.13 (s, 3H); 13C NMR (100 MHz, DMSO-d6) δ (ppm): 168.06, 158.97, 158.56, 156.16, 147.85, 136.45, 132.64, 131.30, 130.91, 128.94, 123.92, 119.18, 110.13, 66.80, 37.14, 33.76, 24.34, 16.14; Anal. calcd. for m/z of C21H22ClN3O2S2 (HRMS [M + Na]+): 470.07342, found: 470.07318.
2-(2-Isopropyl-5-methylphenoxy)-N-(5-(methylthio)-1,3,4-thiadiazol-2-yl)acetamide (5f). White solid, yield 79%, mp 158–160 °C; 1H NMR (400 MHz, DMSO-d6) δ (ppm): 12.84 (s, 1H), 7.09 (d, J = 8.0 Hz, 1H), 6.75 (d, J = 8.0 Hz, 1H), 6.66 (s, 1H), 4.91 (s, 2H), 2.72 (s, 3H), 2.23 (s, 3H), 1.17 (s, 3H), 1.16 (s, 3H); 13C NMR (100 MHz, DMSO-d6) δ (ppm): 167.83, 161.15, 158.21, 155.19, 136.33, 126.31, 122.32, 112.82, 66.73, 26.42, 23.15, 21.40, 16.43; Anal. calcd. for m/z of C15H19N3O2S2 (HRMS [M + Na]+): 360.08109, found: 360.08075.
N-(5-(ethylthio)-1,3,4-thiadiazol-2-yl)-2-(2-isopropyl-5-methylphenoxy)acetamide (5g). White solid, yield 78%, mp 168–170°C; 1H NMR (400 MHz, DMSO-d6) δ (ppm): 12.87 (s, 1H), 7.09 (d, J = 8.0 Hz, 1H), 6.75 (d, J = 8.0 Hz, 1H), 6.67 (s, 1H), 4.91 (s, 2H), 3.34–3.29 (m, 1H), 3.23 (q, J1 = 8.0 Hz, J2 = 16.0 Hz, 2H), 2.23 (s, 3H), 1.35 (t, J = 8.0 Hz, 3H), 1.17 (s, 3H), 1.16 (s, 3H); 13C NMR (100 MHz, DMSO-d6) δ (ppm): 167.88, 159.45, 158.57, 155.18, 136.34, 133.91, 126.31, 122.33, 112.80, 66.72, 28.54, 26.42, 23.15, 21.40, 15.20; Anal. calcd. for m/z of C16H21N3O2S2 (HRMS [M + Na]+): 374.09674, found: 374.09632.
N-(5-(benzylthio)-1,3,4-thiadiazol-2-yl)-2-(2-isopropyl-5-methylphenoxy)acetamide (5h). White solid, yield 88%, mp 133–135°C; 1H NMR (400 MHz, DMSO-d6) δ (ppm): 12.86 (s, 1H), 7.41 (d, J = 8.0 Hz, 2H), 7.35–7.25 (m, 3H), 7.09 (d, J = 8.0 Hz, 1H), 6.75 (d, J = 8.0 Hz, 1H), 6.66 (s, 1H), 4.91 (s, 2H), 4.50 (s, 2H), 3.34–3.27 (m, 1H), 2.23 (s, 3H), 1.17 (s, 3H), 1.15 (s, 3H); 13C NMR (100 MHz, DMSO-d6) δ (ppm): 167.89, 158.88, 155.17, 137.13, 136.33, 133.91, 129.45, 129.02, 128.06, 126.30, 122.34, 112.82, 66.74, 38.01, 26.40, 23.16, 21.40; Anal. calcd. for m/z of C21H23N3O2S2 (HRMS [M + Na]+): 436.11239, found: 436.11185.
N-(5-((4-fluorobenzyl)thio)-1,3,4-thiadiazol-2-yl)-2-(2-isopropyl-5-methylphenoxy)acetamide (5i). White solid, yield 78%, mp 129–130°C; 1H NMR (400 MHz, DMSO-d6) δ (ppm): 12.86 (s, 1H), 7.46 (q, J1 = 4.0 Hz, J2 = 8.0 Hz, 2H), 7.16 (t, J = 8.0 Hz, 2H), 7.09 (d, J = 8.0 Hz, 1H), 6.75 (d, J = 8.0 Hz, 1H), 6.66 (s, 1H), 4.91 (s, 2H), 4.49 (s, 2H), 3.34–3.27 (m, 1H), 2.23 (s, 3H), 1.17 (s, 3H), 1.15 (s, 3H); 13C NMR (100 MHz, DMSO-d6) δ (ppm): 167.91, 161.98 (d, J = 242.0 Hz), 158.98, 158.65, 155.17, 136.33, 133.91, 133.52 (d, J = 3.0 Hz), 131.51 (d, J = 8.0 Hz), 126.31, 122.34, 115.82 (d, J = 21.0 Hz), 112.82, 66.74, 37.13, 26.39, 23.15, 21.39; Anal. calcd. for m/z of C21H22FN3O2S2 (HRMS [M + Na]+): 454.10297, found: 454.10236.
N-(5-((4-chlorobenzyl)thio)-1,3,4-thiadiazol-2-yl)-2-(2-isopropyl-5-methylphenoxy)acetamide (5j). White solid, yield 82%, mp 138–140°C; 1H NMR (400 MHz, DMSO-d6) δ (ppm): 12.85 (s, 1H), 7.41 (q, J1 = 8.0 Hz, J2 = 16.0 Hz, 4H), 7.09 (d, J = 8.0 Hz, 1H), 6.75 (d, J = 8.0 Hz, 1H), 6.65 (s, 1H), 4.90 (s, 2H), 4.49 (s, 2H), 3.31–3.26 (m, 1H), 2.23 (s, 3H), 1.17 (s, 3H), 1.15 (s, 3H); 13C NMR (100 MHz, DMSO-d6) δ (ppm): 167.83, 158.53, 155.16, 136.49, 136.34, 133.91, 132.63, 131.31, 128.97, 126.32, 122.34, 112.82, 66.72, 37.11, 26.39, 23.16, 21.40; Anal. calcd. for m/z of C21H22ClN3O2S2 (HRMS [M + Na]+): 470.07342, found: 470.07319.
2-(2-Methoxyphenoxy)-N-(5-(methylthio)-1,3,4-thiadiazol-2-yl)acetamide (5k). Yellow solid, yield 72%, mp 135–136 °C; 1H NMR (400 MHz, DMSO-d6) δ (ppm): 12.79 (s, 1H), 7.02 (d, J = 4.0 Hz, 1H), 6.95 (q, J1 = 8.0 Hz, J2 = 16.0 Hz, 2H), 6.86 (t, J = 8.0 Hz, 1H), 4.88 (s, 2H), 3.79 (s, 3H), 2.72 (s, 3H); 13C NMR (100 MHz, DMSO-d6) δ (ppm): 167.82, 161.17, 158.14, 149.66, 147.64, 122.64, 121.12, 114.87, 113.03, 67.61, 56.03, 16.42; Anal. calcd. for m/z of C12H13N3O3S2 (HRMS [M + Na]+): 334.02905, found: 334.02896.
N-(5-(ethylthio)-1,3,4-thiadiazol-2-yl)-2-(2-methoxyphenoxy)acetamide (5l). White solid, yield 68%, mp 138–140°C; 1H NMR (400 MHz, DMSO-d6) δ (ppm): 12.81 (s, 1H), 7.02 (dd, J1 = 4.0 Hz, J2 = 8.0 Hz, 1H), 6.95 (qd, J1 = 4.0 Hz, J2 = 8.0 Hz, 2H), 6.89–6.84 (m, 1H), 4.89 (s, 2H), 3.79 (s, 3H), 3.23 (q, J1 = 8.0 Hz, J2 = 16.0 Hz, 2H), 1.34 (t, J = 8.0 Hz, 3H); 13C NMR (100 MHz, DMSO-d6) δ (ppm): 167.88, 159.45, 158.51, 149.65, 147.64, 122.63, 121.12, 114.84, 113.02, 67.59, 56.03, 28.54, 15.19; Anal. calcd. for m/z of C13H15N3O3S2 (HRMS [M + Na]+): 348.04470, found: 348.04468.
N-(5-(benzylthio)-1,3,4-thiadiazol-2-yl)-2-(2-methoxyphenoxy)acetamide (5m). White solid, yield 85%, mp 135–136°C; 1H NMR (400 MHz, DMSO-d6) δ (ppm): 12.80 (s, 1H), 7.41 (d, J = 8.0 Hz, 2H), 7.35–7.25 (m, 3H), 7.02–6.84 (m, 4H), 4.87 (s, 2H), 4.49 (s, 2H), 3.78 (s, 3H); 13C NMR (100 MHz, DMSO-d6) δ (ppm): 167.87, 158.89, 158.71, 149.63, 147.61, 137.14, 129.47, 129.03, 128.07, 122.63, 121.11, 114.80, 113.00, 67.55, 56.01, 37.99; Anal. calcd. for m/z of C18H17N3O3S2 (HRMS [M + Na]+): 410.06035, found: 410.06027.
N-(5-((4-fluorobenzyl)thio)-1,3,4-thiadiazol-2-yl)-2-(2-methoxyphenoxy)acetamide (5n). Yellow solid, yield 77%, mp 137–139°C; 1H NMR (400 MHz, DMSO-d6) δ (ppm): 12.81 (s, 1H), 7.47–7.43 (m, 2H), 7.19–7.14 (m, 2H), 7.03–6.84 (m, 4H), 4.88 (s, 2H), 4.49 (s, 2H), 3.79 (s, 3H); 13C NMR (100 MHz, DMSO-d6) δ (ppm): 167.90, 161.99 (d, J = 243.0 Hz), 158.93, 158.66, 149.65, 147.62, 133.53 (d, J = 3.0 Hz), 131.54 (d, J = 8.0 Hz), 122.64, 121.11, 115.82 (d, J = 22.0 Hz), 114.84, 113.01, 67.59, 56.02, 37.13; Anal. calcd. for m/z of C18H16FN3O3S2 (HRMS [M + Na]+): 428.05093, found: 428.05066.
N-(5-((4-chlorobenzyl)thio)-1,3,4-thiadiazol-2-yl)-2-(2-methoxyphenoxy)acetamide (5o). Yellow solid, yield 79%, mp 136–138 °C; 1H NMR (400 MHz, DMSO-d6) δ (ppm): 12.81 (s, 1H), 7.44–7.38 (m, 4H), 7.03–6.84 (m, 4H), 4.88 (s, 2H), 4.49 (s, 2H), 3.79 (s, 3H); 13C NMR (100 MHz, DMSO-d6) δ (ppm): 167.91, 158.97, 158.54, 149.65, 147.62, 136.49, 132.64, 131.32, 128.97, 122.64, 121.12, 114.85, 113.01; 67.59, 56.02, 37.13; Anal. calcd. for m/z of C18H16ClN3O3S2 (HRMS [M + Na]+): 444.02138, found: 444.02089.
2-(Benzo[d][1,3]dioxol-5-yloxy)-N-(5-(methylthio)-1,3,4-thiadiazol-2-yl)acetamide (5p). Pink solid, yield 78%, mp 171–172°C; 1H NMR (400 MHz, DMSO-d6) δ (ppm): 12.85 (s, 1H), 6.82 (d, J = 8.0 Hz, 1H), 6.70 (s, 1H), 6.40 (d, J = 8.0 Hz, 1H), 5.97 (s, 2H), 4.82 (s, 2H), 2.72 (s, 3H); 13C NMR (100 MHz, DMSO-d6) δ (ppm): 167.79, 161.19, 158.16, 153.47, 148.37, 142.21, 108.43, 106.36, 101.63, 98.61, 67.38, 16.41; Anal. calcd. for m/z of C12H11N3O4S2 (HRMS [M + Na]+): 348.00832, found: 348.00793.
2-(Benzo[d][1,3]dioxol-5-yloxy)-N-(5-(benzylthio)-1,3,4-thiadiazol-2-yl)acetamide (5q). Pink solid, yield 84%, mp 176–178°C; 1H NMR (400 MHz, DMSO-d6) δ (ppm): 12.82 (s, 1H), 7.41 (d, J = 8.0 Hz, 2H), 7.35–7.26 (m, 3H), 6.81 (d, J = 8.0 Hz, 1H), 6.70 (d, J = 4.0 Hz, 1H), 6.40 (s, 1H), 5.97 (s, 2H), 4.81 (s, 2H), 4.49 (s, 2H); 13C NMR (100 MHz, DMSO-d6) δ (ppm): 167.86, 158.93, 153.46, 148.38, 142.22, 137.13, 129.46, 129.03, 128.07, 108.43, 106.34, 101.64, 98.60, 67.36, 38.01; Anal. calcd. for m/z of C18H15N3O4S2 (HRMS [M + Na]+): 424.03962, found: 424.03902.
2-(Benzo[d][1,3]dioxol-5-yloxy)-N-(5-((4-fluorobenzyl)thio)-1,3,4-thiadiazol-2-yl)acetamide (5r). Yellow solid, yield 79%, mp 165–167°C; 1H NMR (400 MHz, DMSO-d6) δ (ppm): 12.83 (s, 1H), 7.47–7.44 (m, 2H), 7.17 (d, J = 8.0 Hz, 2H), 6.82 (d, J = 8.0 Hz, 1H), 6.40 (q, J1 = 4.0 Hz, J2 = 8.0 Hz, 1H), 5.97 (s, 2H), 4.82 (s, 2H), 4.49 (s, 2H); 13C NMR (100 MHz, DMSO-d6) δ (ppm): 167.87, 161.99 (d, J = 243.0 Hz), 158.94, 158.71, 153.45, 148.38, 142.22, 133.51 (d, J = 3.0 Hz), 131.52 (d, J = 9.0 Hz), 115.82 (d, J = 21.0 Hz), 108.42, 106.34, 101.63, 101.63, 98.60, 67.36, 37.36; Anal. calcd. for m/z of C18H14FN3O4S2 (HRMS [M + Na]+): 442.03020, found: 442.02960.
2-(Benzo[d][1,3]dioxol-5-yloxy)-N-(5-((4-chlorobenzyl)thio)-1,3,4-thiadiazol-2-yl)acetamide (5s). Yellow solid, yield 70%, mp 166–168°C; 1H NMR (400 MHz, DMSO-d6) δ (ppm): 12.84 (s, 1H), 7.45–7.38 (m, 4H), 6.82 (d, J = 8.0 Hz, 1H), 6.71 (d, J = 4.0 Hz, 1H), 6.40 (q, J1 = 4.0 Hz, J2 = 8.0 Hz, 1H), 5.97 (s, 2H), 4.82 (s, 2H), 4.49 (s, 2H); 13C NMR (100 MHz, DMSO-d6) δ (ppm): 167.88, 158.99, 158.58, 153.45, 148.38, 142.22, 136.46; Anal. calcd. for m/z of C18H14ClN3O4S2 (HRMS [M + Na]+): 458.00065, found: 458.00023.
3.2 Biological evaluations
Table 1 showed that, at 100 and 50 μg/ml, compounds 5a, 5b, 5d, 5e, 5f, 5g, 5k, 5L, and 5n showed significant in vitro inhibitory effect against Xac, with the inhibition rate ranges of 46%–84% and 33%–70%, respectively, which were higher than thiodiazole copper and bismerthiazol. Meanwhile, compounds 5a, 5b, 5f, 5k, 5L, and 5n exhibited excellent in vitro antibacterial activity against Xoc, with the inhibition rate ranges of 71%–92% and 53%–80% at 100 and 50 μg/ml, respectively, which were superior to thiodiazole copper and bismerthiazol. In particular, Table 2 showed that the EC50 values for compound 2-(2-methoxyphenoxy)-N-(5-(methylthio)-1,3,4-thiadiazol-2-yl)acetamide (5k) against Xac and Xoc were 22 and 15 μg/ml, respectively, which were higher than thiodiazole copper and bismerthiazol.
Table 3 showed that compounds 5a−5s revealed lower in vitro inhibitory effects against M. bainieri, M. fragilis, and T. atroviride, with the inhibition rate ranges of 0%–51%, 0%–47%, and 0%–21% at 50 μg/ml, respectively, than carbendazim.
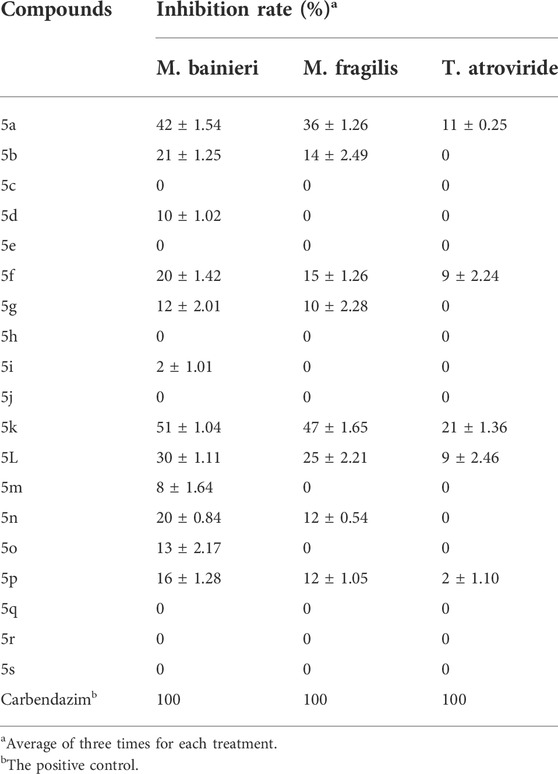
TABLE 3. In vitro antifungal activity test of compounds 5a−5s against M. bainieri, M. fragilis, and T. atroviride.
3.3 Structure-activity relationship analysis
The SAR analysis was analyzed based on the inhibitory activity listed in Tables 1 and 2. First, the presence of the 2-OCH3 group at R1 substituent group showed better inhibitory activity in the order of 5k > 5f, 5k > 5a, and 5k > 5p. Second, the CH3 group at the R2 substituent group could increase the inhibitory activity followed the order of 5a > 5b, 5f > 5g, and 5k > 5L.
4 Conclusion
In conclusion, using the botanical active components of carvacrol, thymol, guaiacol, and sesamol as the lead structures, 19 structurally characterized botanical active component derivatives containing carboxamide and 1,3,4-thiadiazole thioether moieties were prepared. Bioassay results demonstrated that compound 2-(2-methoxyphenoxy)-N-(5-(methylthio)-1,3,4-thiadiazol-2-yl)acetamide (5k) had the higher inhibitory activity against Xac and Xoc than thiodiazole copper and bismerthiazol. Meanwhile, the analysis of SAR results showed that the presence of the 2-OCH3 and CH3 groups at R1 and R2 substituent groups, respectively, could increase the inhibitory effects of the target compounds.
Data availability statement
The original contributions presented in the study are included in the article/Supplementary Material, further inquiries can be directed to the corresponding authors.
Author contributions
Methodology, PL; data analysis, CC, RZ, GY, MX, and GW; writing—review and editing, PL and XW; funding acquisition, PL and XW.
Funding
This research was funded by the Science and Technology Foundation of Guizhou Province, grant number ZK [2021] 137, the National Torch Base Project of Qiandongnan Miao-Dong Medicine Characteristic Industrial, grant number J [2018] 007, the Special Subject of Doctor and Professor Service Group of Kaili University, grant number BJFWT201909, and the 2022 Special Subject of Supporting the Quality Improvement Project of Municipal (Prefectural) Colleges and Universities in Qiandongnan, grant number QDNCJ [2022] 51klxy23.
Conflict of interest
The authors declare that the research was conducted in the absence of any commercial or financial relationships that could be construed as a potential conflict of interest.
Publisher’s note
All claims expressed in this article are solely those of the authors and do not necessarily represent those of their affiliated organizations, or those of the publisher, the editors and the reviewers. Any product that may be evaluated in this article, or claim that may be made by its manufacturer, is not guaranteed or endorsed by the publisher.
Supplementary material
The Supplementary Material for this article can be found online at: https://www.frontiersin.org/articles/10.3389/fchem.2022.1036909/full#supplementary-material
References
Bhattacharjee, R., and Dey, U. (2014). An overview of fungal and bacterial biopesticides to control plant pathogens/diseases. Afr. J. Microbiol. Res. 8 (17), 1749–1762. doi:10.5897/AJMR2013.6356
Cantrell, C. L., Dayan, F. E., and Duke, S. O. (2012). Natural products as sources for new pesticides. J. Nat. Prod. 75 (6), 1231–1242. doi:10.1021/np300024u
Chávez-Dulanto, P. N., Thiry, A. A. A., Glorio-Paulet, P., Vögler, O., and Carvalho, F. P. (2021). Increasing the impact of science and Technology to provide more people with healthier and safer food. Food Energy secur. 10, e259. doi:10.1002/fes3.259
Chen, J. V., Yi, C. F., Wang, S. B., Wu, S. K., Li, S. Y., Hu, D., et al. (2019). Novel amide derivatives containing 1, 3, 4-thiadiazole moiety: Design, synthesis, nematocidal and antibacterial activities. Bioorg. Med. Chem. Lett. 29 (10), 1203–1210. doi:10.1016/j.bmcl.2019.03.017
Chen, M. H., Zhang, X., Lu, D. W., Luo, H. R., Zhou, Z. Y., Qin, X., et al. (2021). Synthesis and bioactivities of novel 1, 3, 4-thiadiazole derivatives of glucosides. Front. Chem. 9, 645876. doi:10.3389/fchem.2021.645876
Cui, X., Zhu, J. Q., Hou, R., and Wang, J. (2022). Antibacterial activity and mechanism of eugenol, carvacrol and thymol against Fusarium graminearum. Food Sci. doi:10.7506/spkx1002-6630-20220107-051
Dalgaard, P., Ross, T., Kamperman, L., Neumeyer, K., and McMeekin, T. A. (1994). Estimation of bacterial growth rates from turbidimetric and viable count data. Int. J. Food Microbiol. 23 (3-4), 391–404. doi:10.1016/0168-1605(94)90165-1
Friedrich, L., Byrne, R., Treder, A., Singh, I., Bauer, C., Gudermann, T., et al. (2020). Shape Similarity by Fractal Dimensionality: An Application in the de novo Design of (-)-Englerin A Mimetics. ChemMedChem 15 (7), 566–570. doi:10.1002/cmdc.202000017
Guo, F., Zhang, Z. Q., and Zhao, Z. (1998). Pesticide resistance of tetranychus cinnabarinus (Acari: Tetranychidae) in China: A review. Syst. Appl. Acarol. 3 (1), 3–7. doi:10.11158/saa.3.1.1
Jia, X. Y., Xu, D. H., Xie, K. Y., and Ding, Y. L. (2017). Synthesis and antibacterial activity of sesamol amide derivatives. Chem. Res. 28 (1), 56–60. doi:10.14002/j.hxya.2017.01.009
Kachur, K., and Suntres, Z. (2020). The antibacterial properties of phenolic isomers, carvacrol and thymol. Crit. Rev. Food Sci. Nutr. 60 (18), 3042–3053. doi:10.1080/10408398.2019.1675585
Neeraja, C., Anil, K., Purushotham, P., Suma, K., Sarma, P., Moerschbacher, B. M., et al. (2010). Biotechnological approaches to develop bacterial chitinases as a bioshield against fungal diseases of plants. Crit. Rev. Biotechnol. 30 (3), 231–241. doi:10.3109/07388551.2010.487258
Opara, U. L. (2013). Perspective: The evolving dimensions and perspectives on food security – what are the implications for postharvest Technology research, policy and practice? Int. J. Postharvest Technol. Innov. 3 (3), 324–332. doi:10.1504/ijpti.2013.059340
Rathod, N. B., Kulawik, P., Ozogul, F., Regenstein, J. M., and Ozogul, Y. (2021). Biological activity of plant-based carvacrol and thymol and their impact on human health and food quality. Trends Food Sci. Technol. 116, 733–748. doi:10.1016/j.tifs.2021.08.023
Rosegrant, M. W., and Cline, S. R. (2003). Global food security: Challenges and policies. Science 302 (5652), 1917–1919. doi:10.1126/science.1092958
Schaad, N. W., Wang, Z. K., Di, M., McBeath, J., Peterson, G. L., and Bonde, M. (1996). An improved infiltration technique to test the pathogenicity of Xanthomonas oryzae pv. oryzae in rice seedlings. Seed Sci. Technol. 24 (3), 449–456.
Sharifi-Rad, M., Varoni, E. M., Iriti, M., Martorell, M., Setzer, W. N., del Mar Contreras, M., et al. (2018). Carvacrol and human health: A comprehensive review. Phytotherapy Res. 32 (9), 1675–1687. doi:10.1002/ptr.6103
Shen, Y. K., and He, Y. L. (2022). Agropharmaceutical evaluation of carvacrol aqueous solution on red spider mite of apple tree. J. Lanzhou Jiaot. Univ. 41 (3), 133–138. doi:10.3969/j.issn.1001-4373.2022.03.019
Souto, A. L., Sylvestre, M., Tölke, E. D., Tavares, J. F., Barbosa-Filho, J. M., and Cebrian-Torrejon, G. (2021). Plant-derived pesticides as an alternative to pest management and sustainable agricultural production: Prospects, applications and challenges. Molecules 26 (16), 4835. doi:10.3390/molecules26164835
Tang, X., Zhang, C., Chen, M., Xue, Y. N., Liu, T. T., and Xue, W. (2020). Synthesis and antiviral activity of novel myricetin derivatives containing ferulic acid amide scaffolds. New J. Chem. 44 (6), 2374–2379. doi:10.1039/C9NJ05867B
Wang, J. L., Zhang, J. F., Ma, J. X., Liu, L., Shen, T., and Tian, Y. Q. (2022). Antagonistic activity and defense mechanism of carvacrol and eugenol against Fusarium solani. Microbiol. China 49 (5), 1638–1650. doi:10.13344/j.microbiol.china.210891
Wu, W. N., Tai, A. Q., Chen, Q., and Ouyang, G. P. (2016). Synthesis and antiviral bioactivity of novel 2-substituted methlthio-5-(4-amino-2-methylpyrimidin-5-yl)-1, 3, 4-thiadiazole derivatives. J. Heterocycl. Chem. 53 (2), 626–632. doi:10.1002/jhet.2435
Yang, Z. B., Li, P., and Gan, X. H. (2018). Novel pyrazole-hydrazone derivatives containing an isoxazole moiety: Design, synthesis, and antiviral activity. Molecules 23 (7), 1798. doi:10.3390/molecules23071798
Yang, Z. B., Li, P., and He, Y. J. (2019). Design, synthesis, and bioactivity evaluation of novel isoxazole-amide derivatives containing an acylhydrazone moiety as new active antiviral agents. Molecules 24 (20), 3766. doi:10.3390/molecules24203766
Keywords: botanical active component, carboxamide, 1,3,4-thiadiazole thioether, antibacterial activity, antifungal activity
Citation: Li P, Chen C, Zhu R, Yang G, Xu M, Wan G and Wang X (2022) Novel botanical active component derivatives containing carboxamide and 1,3,4-Thiadiazole thioether moieties: Design, synthesis, and inhibitory activity. Front. Chem. 10:1036909. doi: 10.3389/fchem.2022.1036909
Received: 05 September 2022; Accepted: 12 September 2022;
Published: 27 September 2022.
Edited by:
Jian Wu, Guizhou University, ChinaReviewed by:
Xingang Meng, Jingdezhen University, ChinaGuo Ping Zhang, Huaibei Normal University, China
Zhenchao Wang, Guizhou University, China
Copyright © 2022 Li, Chen, Zhu, Yang, Xu, Wan and Wang. This is an open-access article distributed under the terms of the Creative Commons Attribution License (CC BY). The use, distribution or reproduction in other forums is permitted, provided the original author(s) and the copyright owner(s) are credited and that the original publication in this journal is cited, in accordance with accepted academic practice. No use, distribution or reproduction is permitted which does not comply with these terms.
*Correspondence: Pei Li, cGwxOTg5MDYyN0AxMjYuY29t; Xiang Wang, Z3pod3gwODI4QDEyNi5jb20=