- 1Department of Orthopaedic Surgery, Union Hospital, Tongji Medical College, Huazhong University of Science and Technology, Wuhan, China
- 2School of Nursing, Tongji Medical College, Huazhong University of Science and Technology, Wuhan, China
The importance of reactive oxygen species (ROS) in the occurrence and development of orthopaedic related diseases is becoming increasingly prominent. ROS regulation has become a new method to treat orthopaedic related diseases. In recent years, the application of nanomaterials has become a new hope for precision and efficient treatment. However, there is a lack of reviews on ROS-regulated nanomaterials for orthopaedic related diseases. Based on the key significance of nanomaterials for the treatment of orthopaedic related diseases, we searched the latest related studies and reviewed the nanomaterials that regulate ROS in the treatment of orthopaedic related diseases. According to the function of nanomaterials, we describe the scavenging of ROS related nanomaterials and the generation of ROS related nanomaterials. In this review, we closely integrated nanomaterials with the treatment of orthopaedic related diseases such as arthritis, osteoporosis, wound infection and osteosarcoma, etc., and highlighted the advantages and disadvantages of existing nanomaterials. We also looked forward to the design of ROS-regulated nanomaterials for the treatment of orthopaedic related diseases in the future.
1 Introduction
The common orthopaedic diseases mainly traumatic injury orthopaedic disease, metabolic immune orthopaedic disease and abnormal proliferative orthopaedic disease. Some other diseases including prosthesis/wound infection and wound repair are also closely related to orthopaedics. Traumatic orthopaedic disease includes fracture, osteonecrosis, bone defect and so on (Einhorn and Gerstenfeld, 2015; El-Rashidy et al., 2017; Lespasio et al., 2019). Metabolic immune orthopaedic diseases include osteoporosis, osteoarthritis, rheumatoid arthritis, etc. (Scott et al., 2010; Pereira et al., 2015; Zhao et al., 2018a). Abnormal proliferative orthopaedic diseases are represented by hyperostosis and some kinds of bone tumors. However, an orthopaedic disease may correspond to different classifications, such as a bone tumor that may cause bone defects after resection (Zhao et al., 2018b). In general, clinicians will choose surgical treatment for local bone lesions and medical treatment for systemic bone lesions. However, it should be pointed out that for the current various orthopaedic diseases, almost none of the diseases have a treatment plan that can make the patient recover back to “total normal state”. Most patients show dysfunction or morphological changes after treatment, and may even have no obvious therapeutic effect. This has prompted us to explore mechanisms and innovate treatment options for a variety of orthopaedic diseases.
The occurrence of orthopaedic diseases is usually due to the disturbance of local metabolic level, in which REDOX reaction plays a great role. Many metabolic processes are inseparable from REDOX reactions. Common REDOX reactions related to metabolism include citric acid cycle, electron transfer and so on (Akram, 2014; Garrido-Pérez et al., 2020). In the study of the regulation of metabolic process, there is a kind of REDOX reaction intermediates-free radicals has attracted more attention. Free radicals are associated with a variety of biological processes, including aging, etc. (Harman, 2003). Reactive oxygen species (ROS), lipid free radicals are common free radicals (Valko et al., 2006; Matsuoka and Yamada, 2021). Their essence is that the molecules contain unpaired electrons and have high reactivity. ROS, as a major free radical type, plays an important role in regulating various physiological and pathological processes in cells and organisms, and has become one of the most cutting-edge and important research fields in many orthopaedic diseases. Generally speaking, ROS can be divided into endogenous and exogenous sources. Under normal circumstances, ROS produced spontaneously by cells are endogenous ROS, such as those produced in the process of oxidative phosphorylation in mitochondria (Berry et al., 2018). ROS increased by treatment, environmental exposure and other factors are generally exogenous ROS, including ROS caused by exposure to some nanoparticles (NPs), exposure to ionizing or non-ionizing radiation, chemotherapy drugs and microbial infection (Rimal et al., 2005; Wang et al., 2019; Li et al., 2020a; Ludovici et al., 2020; Golovynska et al., 2022). Under normal conditions, ROS plays an important role in the regulation of metabolism, but excessive ROS usually leads to cell damage and even apoptosis (Madreiter-Sokolowski et al., 2020; Tsubata, 2020). ROS is associated with the development and prognosis of many orthopaedic diseases, such as osteoporosis, osteoarthritis and bone defects (Ilyas et al., 2019; Li et al., 2020b; Zhou et al., 2022a). ROS has also been used as a therapeutic target or treatment for many orthopaedic diseases, such as osteosarcoma treated by photodynamic regimens and osteoarthritis treated by reducing ROS levels (Ding et al., 2021; Lu et al., 2021). The controlled regulation of ROS may be the key to breakthrough in the treatment of orthopaedic diseases.
Theoretically, whether ROS acts as a damaging molecule or a signaling molecule depends on the concentration of ROS in cell (He et al., 2021). Therefore, how to accurately regulate ROS is a key issue for ROS as a target or means to treat diseases. In recent years, great progress has been made in the study of ROS regulation. In particular, nanomaterials have been introduced into the field of ROS regulation, which makes the related fields develop rapidly. ROS-based nano therapeutic methods or nano medicines may be applied to regulate the ROS process. This depends on the inherent biophysical and biochemical properties of nanomaterials, such as appropriate size, high surface area, etc. Nanomaterials have great potential to modulate ROS compared with traditional drug molecules because they can be modified to achieve lesion targeting, and they can show satisfactory catalytic efficiency due to their special 3D structure. Nanomaterials have made some progress in the treatment of orthopaedic diseases by regulating ROS, such as bone tumors, osteoarthritis, bone defects and so on. Therefore, it is important and necessary to review this topic, which may provide new possibilities for the further development of ROS-based nanomaterials for the treatment of orthopaedic diseases. The search results of existing studies show that photodynamic therapy (PDT), sonodynamic therapy (SDT) and chemodynamic therapy (CDT) are important research directions related to orthopaedic diseases, and the published articles are increasing year by year. Antioxidant therapy or ROS toxicity therapy is the Frontier of nanomaterials in the treatment of orthopaedic diseases, and its application direction in the treatment of orthopaedic diseases is shown in Figure 1.
2 An overview of the association of ROS with orthopaedic diseases
ROS that play a key role in the pathogenesis of orthopaedic diseases include H2O2, hydroxyl radical, superoxide anion, etc. Normally, ROS levels are kept low in normal joints (Lepetsos and Papavassiliou, 2016). The occurrence of many orthopaedic diseases may be related to the abnormal increase of ROS level. For patients with osteoarthritis, ROS such as H2O2, O2− are significantly increased in joints (Yao et al., 2019). The over generation of ROS triggers a series of reactions, such as excessive oxidation and DNA damage, which is considered to be one of reasons of chondrocyte loss and tissue damage (Hosseinzadeh et al., 2016). ROS produced by oxidative stress is also closely related to the pathogenesis of osteoporosis. For example, the study of Toker et al. showed that ROS is closely related to diabetic osteoporosis (Toker et al., 2012). An et al. showed that activation of ROS/MAPKs/NF-κB/NLRP3 was the important cause of osteoporosis in Diabetes Mellitus (An et al., 2019). In addition, the occurrence and development of orthopaedic diseases such as rheumatoid arthritis and osteonecrosis of the femoral head are closely related to the abnormal production of ROS((Chen et al., 2022), (Chen et al., 2020)). There are few studies on the relationship between ROS and the occurrence of bone tumors or bone metastases. Whether this association exists still needs to be proved. Some representative studies on ROS and orthopaedic diseases are shown in Table 1.
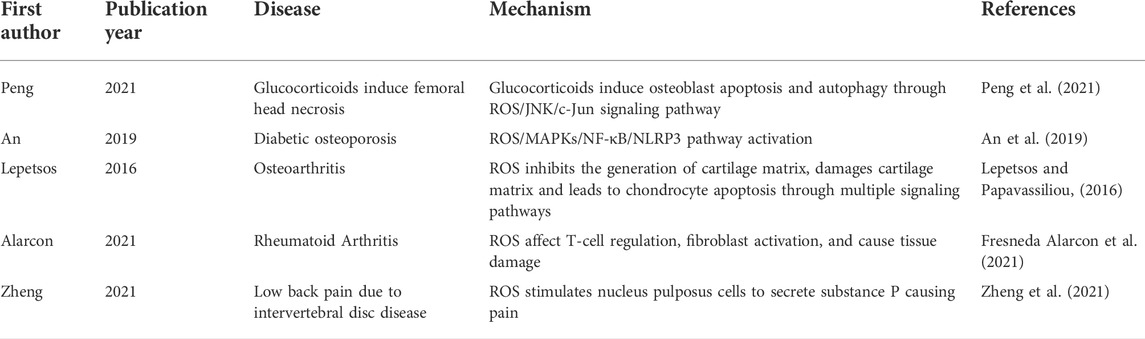
TABLE 1. Some representative studies on the association between ROS and the occurrence and development of orthopaedic diseases.
3 ROS regulation nanomaterials and the related mechanism of ROS production/elimination
Although the occurrence and development of most orthopaedic diseases are related to the abnormal increase of ROS in intracellular or local microenvironment, both down-regulation and up-regulation of ROS can be used as therapeutic ideas when using nanomaterials to treat different orthopaedic diseases. These has become antioxidant therapy and ROS-mediated cytotoxic therapy, respectively. Like other disease therapy, in the aspect of nanomaterials mediated antioxidant therapy for orthopaedic diseases, ROS scavenging nanomaterials are mainly developed, such as nanomaterials equipped with ROS scavengers or forming structures that catalyze ROS elimination (Huang et al., 2021). The purpose of nanomaterial therapy is to remove excess ROS and maintain normal physiological processes. The vast majority of cells in the body, including osteoblasts and osteoclasts, have their own endogenous ROS scavenging system. However, due to the large amount of ROS produced by various stimuli, the damage will occur when the endogenous ROS scavenging system cannot complete the removal of ROS. At this point, exogenous ROS clearance is very important. At present, many ROS-scavenging nanomaterials have been developed that can effectively alleviate the abnormal ROS increase to stabilize normal physiological functions (Li et al., 2021a). At present, some of them have been used in the research of the treatment of orthopaedic diseases, and some of them are expected to become “new weapons” for the treatment of refractory orthopaedic diseases. Based on the current studies, these nano-ROS scavengers show great potential for the remission and treatment of orthopaedic disease by inhibiting ROS.
Up-regulation of ROS in cells or microenvironment by nanoparticles is called nanoparticle mediated ROS cytotoxic therapy. The main idea of this treatment is to use ROS up-regulated Nano platforms to enhance ROS production at sites such as tumors or bacterial infections, thereby exerting the toxic effects. This treatment idea has been widely recognized in the treatment of osteosarcoma, bacterial infection and so on (Chu et al., 2019; Seo et al., 2021). A large amount of ROS is generated by nanomaterials to form oxidative stress in the cell or microenvironment, which will far exceed the endogenous ROS clearance level of cells or bacteria themselves, thus leading to cell apoptosis or bacterial death. The advantage of using nanoparticles as agents of ROS toxicity is that the nanoparticles can be modified to achieve site-specific and controlled toxicity treatment. At present, there are mainly three kinds of nanoparticles that exert ROS-toxic effects 1): ROS generated by carrying photosensitizer, sonosensitizer and other molecules (Ocsoy et al., 2016; Lee et al., 2021); 2) ROS synthesis catalyzed by a special structure independent of conventional photosensitizer/acoustic sensitizer (Yi et al., 2020); 3) Combined application of the two mechanisms. The common treatment methods of ROS toxicity using nanomaterials include chemodynamic therapy (CDT), photodynamic therapy (PDT), sonodynamic therapy (SDT), etc. (McHale et al., 2016; Kwiatkowski et al., 2018; Yu et al., 2021). These treatment ideas have been reflected in the treatment research of related orthopaedic diseases. In this section, we summarize the currently known strategies and approaches for the treatment of ROS-related orthopaedic diseases, including antioxidant therapy and ROS-induced toxicity.
3.1 ROS scavenging nanomaterials for antioxidant treatment of orthopaedic diseases
As mentioned above, the production of ROS in cells or microenvironment increases significantly under significant stress, and endogenous antioxidants cannot remove all ROS, resulting in serious damage to biomolecules including DNA, lipids and proteins, and triggering a series of reactions such as ER stress and mitochondrial damage related apoptosis (Tian et al., 2020; Tu et al., 2021). This is an important mechanism by which ROS promotes the occurrence and development of orthopaedic diseases. At this time, it is particularly important to introduce exogenous substances to remove ROS. However, it must be admitted that the current drugs used to remove ROS still face toxicity problems, stability problems and difficulties in clinical translation (Zhong et al., 2019). In recent years, with the development of nanotechnology, novel ROS scavenging strategies based on multifunctional nanomaterials have been widely studied in the design of ROS scavengers, providing a new opportunity for the treatment of related orthopaedic diseases. Current studies indicate that a great deal of effort has been invested in the development of ROS-scavenging nanomaterials for the treatment of orthopaedic diseases, some representative studies are shown in Table 2. Nanomaterials can be equipped with ROS scavengers to achieve the purpose of ROS elimination. This approach can improve the lack of targeting and poor water solubility of traditional ROS scavenger drug molecules. In addition to being drug carriers, some nanomaterials have direct elimination effect on ROS, which is usually related to the REDOX reaction ability of nanomaterials themselves or the special catalytic ability of nanomaterials. In addition, some nanomaterials can inhibit ROS production by regulating endogenous ROS scavenging pathway. A schematic illustration of mechanism is summarized in Figure 2. The first two kinds of ROS scavenging nanomaterials are described in detail below.
3.1.1 Nanomaterials loaded with ROS scavenger
Introduction of ROS scavengers is a common strategy to remove excess ROS in cells. However, these ROS scavengers have inevitable drawbacks, including lack of cell targeting and possible instability. The introduction of nanocarriers may accelerate the further development of ROS scavengers for the treatment of orthopaedic diseases. Nanomaterials as carriers of ROS scavengers can improve the stability of ROS scavengers, and can also give these molecules the ability to target and reduce their side effects (Lee et al., 2019; Khalil et al., 2020). The types of ROS scavengers loaded may include some small molecules of drugs, or some large molecules, such as enzymes that can eliminate ROS.
The use of nanoparticles to deliver small molecules capable of scavenging ROS is a common research idea for the treatment of related orthopaedic diseases. These small molecules are usually involved in REDOX reactions to achieve the purpose of scavenging ROS. However, it is important to point out that this delivery does not necessarily depend on the “loading” process, and ROS scavengers may become part of the nanomaterial. For example, Zhao et al. designed a nanoparticle P (DMA-co-MPC) in the study on the treatment of osteoarthritis, in which DMA is N-(3,4-dihydroxyphenethyl) methacrylamide, which acts as ROS scavenger (Zhao et al., 2020). DMA contains reducing phenolic hydroxyl groups, which may be the reason for its ROS scavenging ability. Through experiments, the authors proved that nanoparticles can regulate inflammation, pain and other related pathways by removing ROS, which may be a potential drug for the treatment of osteoarthritis. Zhou et al. used nanoparticles synthesized by Fe3+ and curcumin to treat osteoarthritis through the anti-oxidative stress effect of curcumin (Zhou et al., 2022c). In addition to being loaded with small molecules, nanoparticles can also be loaded with enzymes or short peptides to scavenge ROS to treat orthopaedic diseases. Common enzymes that can remove ROS include SOD and so on (Saxena et al., 2022). Some short peptides, such as GSH, are also involved in the removal of intracellular ROS((Yang et al., 2016)). For example, Gui et al. mentioned a SOD-loaded porous polymer nanoparticle that could be targeted to the synovial membrane as a means of treating osteoarthritis (Gui et al., 2022). Gao et al. also designed a nanomaterial containing SOD as a treatment for arthritis (Gao et al., 2020). To date, the use of nanoparticles to deliver ROS scavengers in the treatment of orthopaedic diseases still has great room for development. We believe that the key is how to design the structure of the nanomaterial carrier so that it could be targeted, low toxicity, and high drug loading ability. The use of engineered liposomes or exosomes for ROS scavenger delivery may be a valuable research direction. As for the ROS scavengers that could be loaded, not only some small molecule ROS scavengers, but also some biological molecules such as coenzyme Q10 may be suitable choices. Further experiments are needed to explore which ROS scavengers have more advantages. It is well known that the use of nanomaterials as the carrier of ROS scavengers indeed makes the application prospect of ROS scavengers more promising, but there is still a certain gap between nanoparticles and clinical practice due to its stability and how to produce them on a large scale.
3.1.2 Nanomaterials with ROS quenching effect independent of traditional ROS scavenging molecules
The aforementioned nanoparticles equipped with ROS scavengers can effectively improve the pharmacokinetics, targeting and other deficiencies of some ROS scavengers. However, they still have some shortcomings, such as stability in circulation. At the same time, the complexity of nanoparticle synthesis also makes the application of nanoparticles equipped with ROS scavenger limited. Therefore, it is necessary to design new antioxidant strategies that do not rely on traditional ROS scavenging molecules. In recent years, more studies have focused on the use of nanomaterials themselves to remove ROS. This kind of nanomaterials often have special structure or inherent catalytic properties, including nanozymes, etc. Some nanomaterials that can remove ROS depend on their ability to participate in REDOX reactions, such as dopamine nanomaterials (Newland et al., 2016). Interestingly, some metal oxide nanoparticles seem to exhibit good ROS scavenging ability. For example, cerium oxide nanoparticles are a typical type of nanoparticles with ROS scavenging ability. Vacancy-oxygen defect existing in the cerium oxide nanoparticle shows an advantage in ROS removal (Li et al., 2020c). In their review, Li et al. summarized the synthesis, structure and properties of cerium dioxide nanoparticles in detail, and introduced the mechanism of ROS scavenging by cerium dioxide nanoparticles, as well as some applications in bone tissue engineering (Li et al., 2020c). The ROS scavenging mechanism of nanoparticles fully demonstrates the importance of the microstructure of nanomaterials. Another popular nanoparticle with ROS scavenging ability is MnO2 related nanoparticle, which is often studied as a “nanozyme”. Some studies have shown that MnO2 nanoparticles have the ability to catalyze the reaction of hydrogen peroxide (Gordijo et al., 2015). In the application study of orthopaedic disease treatment, Chen et al. designed a hollow MnO2 nanoparticle with good ROS scavenging ability for the treatment of osteoarthritis (Chen et al., 2021). Kumar et al. mentioned that MnO2 nanoparticles can protect cartilage from oxidative stress caused by inflammation (Kumar et al., 2019). Zhu et al. designed a hollow MnO2 nanoparticles loaded with TGF-β3 to treat intervertebral disc degeneration, and the authors indicated that the hollow MnO2 nanoparticles played an important role in the antioxidation (Zhu et al., 2022). In addition to cerium oxide and manganese dioxide nanoparticles, other metal oxides such as molybdenum oxide and nickel oxide nanoparticles may also be used as functional nanoparticles for ROS scavenging (Mu et al., 2016; Duan et al., 2022). Some of these metal oxide nanoparticles have not been used in orthopaedic disease treatment research, and more relevant exploration can be carried out in the future.
In addition to the above metal oxide nanoparticles, some other types of nanoparticles, such as noble metal nanoparticles (gold, silver, platinum, etc.), polydopamine nanoparticles, etc., may have high activity of ROS scavenging activity (Zhao et al., 2018c; Yang et al., 2018). For example, Sul et al. mentioned in their study that gold nanoparticles can reduce ROS level and affect osteoclast formation (Sul et al., 2010). Nomura et al. showed that Pt nanoparticles could inhibit RANKL-stimulated osteoclast differentiation through ROS scavenging properties (Nomura et al., 2011). It is worth noting that each of these nanoparticles has its own potential applications, for example, the unique microstructure of some metal oxide nanoparticles, the high catalytic efficiency of some metal-organic framework nanomaterials, the specific surface area advantage of noble metal nanoparticles, and the modifiability of some organic nanomaterials. Future research should focus on combining the advantages of different types of nanoparticles to achieve the effect of “learning from each other”. In addition, future studies should focus on the specific mechanism of ROS elimination by nanomaterials, including the possible potential reaction mechanism and influencing factors, so as to provide a solid theoretical basis for ROS elimination of nanomaterials in the treatment of orthopaedic diseases.
3.2 Nanomaterials used to generate ROS toxicity for the treatment of orthopaedic diseases
ROS is a double-edged sword (Lu et al., 2018). As mentioned above, a certain amount of ROS is usually maintained in cells as a “messenger” for the regulation of some signaling pathways (Checa and Aran, 2020). However, excessive ROS can cause cell death. In the case of normal cells or bacteria, excessive ROS is very difficult to tolerate. This brings a new solution to some abnormal or proliferative orthopaedic diseases, such as bone tumors and infection after orthopaedic surgery. In recent years, the use of nanomaterials to increase the intracellular ROS content to kill target cells has become a popular method. Common ROS mainly include H2O2, •O2−, •OH, 1O2, etc., and most strategies focus on improving these ROS with the help of nanomaterials. Depending on the different stimulating methods, these strategies are often classified as photodynamic therapy, sonodynamic therapy, and chemodynamic therapy (Wang et al., 2021). A large amount of ROS produced can cause DNA damage, endoplasmic reticulum stress and mitochondrial apoptosis, etc. ROS can also induce cell apoptosis by influencing other related cell signaling pathways. For example, Huang et al. designed nanomaterials to inhibit autophagy and lead to apoptosis through endoplasmic reticulum stress related to ROS,etc. (Huang et al., 2020). However, it should be pointed out that the regulation of many signaling pathways by ROS has various possibilities, which showing certain complexity, so the regulation of autophagy and other cellular pathways by ROS needs to be careful. The relevant mechanism is shown in Figure 3. Meanwhile, around the topic of ROS generation, the designed nanomaterials need to have some supporting functions, such as increasing the local oxygen content to maintain the continuous supply of ROS((Cheng et al., 2015)). Considering the significance of nanomaterials leading to ROS overload in orthopaedic tumors and orthopaedic surgical infections, in this section we will review the treatment strategies leading to ROS overload by classification according to excitation mode. Some representative ROS-enhanced nanomedicines are listed in Table 3.
3.2.1 Photodynamic therapy
Photodynamic therapy refers to the generation of ROS by photosensitizer under the excitation of a certain wavelength laser to achieve the purpose of disease treatment. Photodynamic therapy was first realized by some drug molecules, including ICG, Ce6 and so on (Ara et al., 2021; Luo et al., 2021). However, similar to ROS scavengers, these photodynamic therapy drug molecules also have a series of problems such as toxicity and lack of targeting (Mitsunaga et al., 2011). The introduction of nanomaterials solves the shortage of existing photodynamic therapy drug molecules and endows photodynamic therapy with more abundant characteristics. In the research of orthopaedic diseases, especially osteosarcoma, it is a common research idea to use nanoparticles to load common photodynamic therapy drug molecules. Zeng et al. designed an ICG-loaded GO nanoparticle and modified it to achieve osteosarcoma aggregation and generate ROS to kill tumor through photodynamic effect (Zeng et al., 2021). Photodynamic therapy can also be combined with chemodynamic therapy to achieve the purpose of combined treatment to kill osteosarcoma. For example, in the study of Huang et al., a graphene equipped with ICG and doxorubicin was designed to target osteosarcoma, and the mechanism of its killing osteosarcoma was studied in detail (Huang et al., 2020). Specifically, ROS produced by photodynamic therapy is the key to enhanced therapy, which can kill osteosarcoma by affecting DNA integrity, autophagy related pathways and apoptosis-related pathways. In addition to the treatment of osteosarcoma, nanoparticles loaded with photodynamic therapeutic drug molecules have also achieved remarkable results in antimicrobial applications. After orthopaedic surgery, the potential infection risk of wound or prosthesis is one of the problems that must be faced by patients and doctors (Backes et al., 2018; Ronin et al., 2022). Among the pathogens that may lead to infection, Staphylococcus aureus is the common one, which can form bacterial film. Based on this problem, Yuan et al. designed a nanoparticle equipped with ICG, which could effectively remove biofilms formed by bacteria by photodynamic therapy under NIR irradiation (Yuan et al., 2020). According to the existing research results, nanomaterials equipped with photosensitizer can indeed achieve better photodynamic therapy effect. However, the similar problem faced by nanomaterials equipped with ROS scavengers is the circulation stability of the nanomaterials. Moreover, the loading load of nanomaterials increases after the photosensitizer is installed, which may affect the further modification of nanomaterials or the loading of chemotherapy drugs. Therefore, it is still necessary to design new photosensitive nanomaterials that do not rely on traditional photosensitizers to achieve more efficient photodynamic therapy.
Whether paying attention to the crystal structure or the 2D/3D structure, nanomaterials have the potential to form special catalytic structures. This catalytic potential can be used for ROS generation. There are already some nanomaterials being developed that have photodynamic effects of their own. The design of these novel photodynamic nanomaterials may be based on the structural characteristics of some traditional photosensitizers, such as porphyrins. These structures can also be directly used in integrated nanomaterials. Cai et al. designed a metal-organic framework material Au@MOF that incorporates porphyrin structures to enable photodynamic therapy (Cai et al., 2021). The use of metal-organic framework nanomaterials to catalyze REDOX reactions and generate ROS is one of the research hotspots. Xie et al. designed a biosafe material based on Ti-MOF to achieve antimicrobial resistance (Xie et al., 2022). In the future research of orthopaedic disease treatment, similar nanomaterials can be more modified in the future to achieve more functional integration, such as the integration of anti-tumor and anti-bacterial, etc. At the same time, this kind of nanomaterials can be combined with other biological materials such as hydrogels to enrich the function. Based on the summary of existing studies, the study of ROS generation by photodynamic therapy is relatively mature, and its application is increasingly widespread. However, we must also recognize the limitations of photodynamic therapy in orthopaedic diseases. It is important to note that the vast majority of orthopaedic diseases have lesions located deep in the body. However, most of the lasers required in photodynamic therapy, such as 808 nm near-infrared laser, do not show good tissue penetration ability. In addition, because human muscle tissues are rich in heme, showing a darker color, which may indicate that these normal human tissues also have certain photothermal capacity. When stimulated by the 808 nm laser, the temperature of these tissues may increase even though there is no aggregation of nanomaterials, which may cause unexpected tissue damage. This is an important reason why the application of photodynamic research in the treatment of orthopaedic diseases is limited. Therefore, new ways of generating ROS from nanomaterials should be developed.
3.2.2 Sonodynamic therapy
Sonodynamic therapy is a new concept emerging in recent years, which is a good supplement to photodynamic therapy. Sonodynamic therapy is to use ultrasound to stimulate a high concentration of sonosensitizer concentrated in the target cells to produce a large number of ROS to kill the target cells. Here sonosensitizer includes some small molecules, such as IR-780 ((Li et al., 2016)). For deep lesions, sonodynamic therapy is a suitable alternative to photodynamic therapy, because the tissue penetration of ultrasound is better than that of NIR laser, and it is less likely to cause non-specific damage to normal human tissue (Huang et al., 2017; Ouyang et al., 2020). There have been some studies on the application of nanomaterials in the treatment of orthopaedic tumors. For example, Fu et al. used degradable hollow mesoporous silica based nanomaterials as the carrier to obtain a multifunctional nanomaterial with acoustic dynamic effect, which can accumulate in osteosarcoma and generate a large amount of ROS to kill the tumor under the action of ultrasound (Fu et al., 2019). In the aspect of antibacterial treatment, some interesting results have been obtained by utilizing the sonodynamic effect of nanomaterials. For example, Wu et al. developed an Au@BTO nanoparticle based on piezoelectric electronics. By virtue of ultrasound, the electron-hole effect leads to the occurrence of REDOX reactions and the generation of ROS, so as to achieve the purpose of antibacterial and promoting tissue repair (Wu et al., 2021). At present, the research on the use of nanomaterials for the sonodynamic treatment of orthopaedic diseases is not enough, which may be related to the difficulty in the research and development of new materials. The key to the development of sonodynamic therapeutic nanomaterials is the microstructure of the nanomaterials, especially the design of the catalytic core. At present, there is still a lack of sufficient research basis in related fields. In addition, the use of nanomaterials in the sonodynamic treatment of orthopaedic diseases also has potential shortcomings. The first is the targeting and stability of nanomaterials, which is a common problem in the treatment of orthopaedic diseases with biological nanomaterials. Sonodynamic therapeutic nanomaterials require more microstructure, which makes further modification of the nanomaterials may need to be more careful. Furthermore, although ultrasound can reach deep lesions through tissues, attenuation of ultrasound energy must be considered. In addition, in order to ensure the stability of the effect, the selection of additional materials such as ultrasonic coupling agent also needs to be careful.
3.2.3 Chemodynamic therapy
Chemodynamic therapy is a new treatment for cancer in recent years, which is mainly based on Fenton response or Fenton-like response (Li et al., 2021b). Based on the slightly acidic environment of the tumor area, chemodynamic therapeutic nanoparticles can rapidly generate large amounts of ROS by Fenton reaction or Fenton-like reaction, without the need for activation by external energy (such as laser, ultrasound, etc.) (Li et al., 2021b). According to the related theory of chemodynamic therapy, nanoparticles that usually activate chemodynamic therapy include necessary metal elements, such as Fe, Cu, etc. (Wu et al., 2019; Koo et al., 2022). Chemodynamic therapy is often used in conjunction with other therapies such as photothermal, photodynamic, or immunotherapy to achieve synergistic effects. In the treatment of orthopaedic related diseases, chemodynamic therapy has more advantages for malignant bone tumors. Fu et al. designed a nanomaterial relying on manganese ions to catalyze the generation of hydroxyl radicals from hydrogen peroxide (Fu et al., 2022). They combined immunotherapy with chemodynamic therapy and provided a new idea for the treatment of osteosarcoma. On the antimicrobial side, chemodynamic therapy also shows more promise when used in conjunction with other therapies. Yang et al. developed a Cu2O based nanoparticle that catalyzes Fenton-like reactions to generate hydroxyl radicals with strong antimicrobial activity, while also promoting tissue repair (Yang et al., 2021b). The development of such nanoparticles is of great significance for the treatment of methicillin-resistant Staphylococcus aureus, a common intractable pathogen in wound infection, and for the treatment innovation of orthopaedic trauma. Combined with the theoretical basis of Chemodynamic therapy, when the microenvironment of the lesion is acidic, chemodynamic therapy will show great advantages. When the focal environment is weakly alkaline (usually normal tissue), the rate of Fenton/Fenton-like response is limited, which may be indicative of limited damage to normal tissue by chemodynamic therapy. However, nanomaterials undergoing chemodynamic therapy are mostly used as catalysts, which can continuously produce ROS. When combined with chemotherapy drugs or other therapies such as photothermal and photodynamic therapies, they often achieve complementary and synergistic effects by affecting autophagy, mitochondrial apoptosis, DNA damage and other pathways. However, chemodynamic therapy also needs to be concerned, that is, for osteosarcoma, the lesion may present a state of hypoxia locally, which is also a common feature of some solid tumors (Zhang et al., 2020). Hypoxic environments may affect ROS production. Therefore, the future design of related nanomaterials should also be taken into account the problem of oxygen supply.
4 Considerations for the design of nanomaterials related to REDOX reactions
In conclusion, the related nanomaterials based on ROS-regulated REDOX reactions show great application value for the treatment of orthopaedic diseases. We reviewed the relevant studies and believe that the following considerations should be paid attention to in the future research and development of nanomaterials for orthopaedic diagnosis and treatment:
1) Design nanomaterials with a clear purpose. Nanomaterials based on ROS regulation should focus on ROS generation or elimination. In the process of designing nanomaterials, the structure of nanomaterials and the corresponding function should be considered, rather than blindly designing a material to verify the function. In general, the catalytic core or functional unit of the nanomaterial should be given sufficient attention.
2) Design nanomaterials based on clinical and pathological features. Different orthopaedic diseases have different etiology, location, microenvironment characteristics of local lesions, and special gene expression/signal pathway changes. These characteristics should be fully integrated in the design of nanomaterials.
3) Good biocompatibility and circulation stability. This is a common standard for biological nanomaterials, which is the first step of nanomaterials research and development to application. Since the vast majority of nanomaterials used in orthopaedic diseases require systemic administration, any relevant research needs to verify the toxicity and circulatory stability of the developed nanomaterials. Studies of toxicity and circulatory stability in vivo are essential. If there is a potential toxicity, the study should be discontinued immediately. If possible, nanomaterials of the corresponding structure and composition should be reported by means of reports.
4) “Simple” design. The design of biological nanomaterials should aim to perform the relevant function in the simplest possible structure. The complex multilayer structure, repeated drug loading and modification of various macromolecules may cause the structure of nanomaterials to be confused and unstable, and the functional study of nanomaterials will become more complicated.
5) Pay attention to the combination of multiple treatment methods. The combination of treatment methods is the common goal of orthopaedic disease treatment. Nanomaterials can be loaded with therapeutic drugs and control their release in cells, and enhance the efficacy of therapeutic drugs by regulating ROS. At the same time, the combination of ROS-based nanomaterials and other treatment methods, such as radiotherapy and photothermal therapy, can also achieve better results. Under the premise of meeting the design principle of “simplification”, the combination of multiple treatment methods may achieve better treatment effect.
5 Reflection and prospect
It is undeniable that the occurrence or development of the vast majority of orthopaedic diseases are related to the disorder of local metabolic microenvironment. Taking some key metabolic reaction products such as ROS as disease regulatory targets has become an important idea for the prevention and treatment of orthopaedic diseases. In recent years, nanomaterials related to ROS regulation have been developed more. Because the preparation process is mature and can be reasonably modified, nanomaterials are considered as the symbol of precision, efficiency and safety in the treatment of orthopaedic diseases. We believe that the synthesis of nanomaterials with therapeutic function is the most promising solution for some refractory orthopaedic related diseases, such as malignant primary bone tumors, bone metastases, and wound infection after orthopaedic surgery. Although many nanomaterials still face many problems, such as lack of specific targets and so on. There are some problems in photodynamic therapy and acoustic dynamic therapy, such as insufficient penetration and energy attenuation. But these problems have a chance to be solved. The first is to strengthen the mechanism research of orthopaedic related diseases, find the specific pathways of diseases through high-throughput sequencing and other means, and explore the specific targets of diseases. At the same time, research in the field of materials science should be strengthened, especially the development of suitable acoustic/laser dielectric materials for therapeutic use.
However, there are still some problems worth reflecting upon when reviewing the existing related researches. For example, how to reasonably judge the specific regulatory effect of a structure or component on ROS and its influencing factors is a key issue. Interestingly, we often see studies in which different research come to different conclusions about the same structure or composition. For example, for the regulation of ROS by curcumin, some studies propose that it is a ROS scavenger, while others show that it is a molecule that promotes ROS production (Zeng et al., 2020; Wu et al., 2022). What are the influencing factors in this need to be clarified, which may include concentration, action time and so on. In addition, the non-specific phagocytosis and targeting effects of nanomaterials still need to be discussed. We have to admit that the application of biological nanomaterials in the treatment of orthopaedic diseases is still relatively not wide, one of the important reasons is that nanomaterials in the circulation may still be non-specific phagocytosed by the liver and spleen, so the targeting effect is limited. While reviewing published studies, we found that some of the proposed nanoparticles seem to have shown better targeting and very low nonspecific phagocytosis, and the reasons for this should be further explored and elucidated. Finally, ethics is an issue that must be considered. Some structures of nanomaterials mentioned in some studies may have certain risks, including nanomaterials synthesized by membrane of bacteria and viruses, etc. The safety of nanomaterials should be fully evaluated during the study, including whether they may activate unexpected immune reactions, etc. When similar nanomaterials are prepared for clinical application in the future, the ethical review and pre-clinical trials must be more rigorous. However, more reflection is for the better development. We always believe that nanomaterials will play an increasingly important role in the treatment of orthopaedic diseases in the future.
Author contributions
ZS and HH are responsible for determining the topic and revising the manuscript. WY, QZ, and QP jointly reviewed the studies, summarized the data, and wrote the manuscript. WH was responsible for the revision of the manuscript.
Acknowledgments
Thanks to the National Natural Science Foundation of China (Project Approval No. 82002318) And the Natural Science Foundation of Hubei Province (Project Approval No. 2020CFB422) for the help in literature search and expert consultation.
Conflict of interest
The authors declare that the research was conducted in the absence of any commercial or financial relationships that could be construed as a potential conflict of interest.
Publisher’s note
All claims expressed in this article are solely those of the authors and do not necessarily represent those of their affiliated organizations, or those of the publisher, the editors and the reviewers. Any product that may be evaluated in this article, or claim that may be made by its manufacturer, is not guaranteed or endorsed by the publisher.
References
Akram, M. (2014). Citric acid cycle and role of its intermediates in metabolism. Cell biochem. Biophys. 68 (3), 475–478. doi:10.1007/s12013-013-9750-1
An, Y., Zhang, H., Wang, C., Jiao, F., Xu, H., Wang, X., et al. (2019). Activation of ROS/MAPKs/NF-κB/NLRP3 and inhibition of efferocytosis in osteoclast-mediated diabetic osteoporosis. FASEB J. 33 (11), 12515–12527. doi:10.1096/fj.201802805rr
Ara, E. S., Noghreiyan, A. V., and Sazgarnia, A. (2021). Evaluation of photodynamic effect of Indocyanine green (ICG) on the colon and glioblastoma cancer cell lines pretreated by cold atmospheric plasma. Photodiagnosis Photodyn. Ther. 35, 102408. doi:10.1016/j.pdpdt.2021.102408
Backes, M., Spijkerman, I. J., de Muinck-Keizer, R. O., Goslings, J. C., and Schepers, T. (2018). Determination of pathogens in postoperative wound infection after surgically reduced calcaneal fractures and implications for prophylaxis and treatment. J. Foot Ankle Surg. 57 (1), 100–103. doi:10.1053/j.jfas.2017.08.016
Berry, B. J., Trewin, A. J., Amitrano, A. M., Kim, M., and Wojtovich, A. P. (2018). Use the protonmotive force: Mitochondrial uncoupling and reactive oxygen species. J. Mol. Biol. 430 (21), 3873–3891. doi:10.1016/j.jmb.2018.03.025
Cai, X., Zhao, Y., Wang, L., Hu, M., Wu, Z., Liu, L., et al. (2021). Synthesis of Au@MOF core-shell hybrids for enhanced photodynamic/photothermal therapy. J. Mat. Chem. B 9 (33), 6646–6657. doi:10.1039/d1tb00800e
Checa, J., and Aran, J. M. (2020). Reactive oxygen species: Drivers of physiological and pathological processes. J. Inflamm. Res. 13, 1057–1073. doi:10.2147/jir.s275595
Chen, K., Liu, Y., He, J., Pavlos, N., Wang, C., Kenny, J., et al. (2020). Steroid-induced osteonecrosis of the femoral head reveals enhanced reactive oxygen species and hyperactive osteoclasts. Int. J. Biol. Sci. 16 (11), 1888–1900. doi:10.7150/ijbs.40917
Chen, L., Tiwari, S. R., Zhang, Y., Zhang, J., and Sun, Y. (2021). Facile synthesis of hollow MnO(2) nanoparticles for reactive oxygen species scavenging in osteoarthritis. ACS Biomater. Sci. Eng. 7 (4), 1686–1692. doi:10.1021/acsbiomaterials.1c00005
Chen, M. W., Lu, Q. J., Chen, Y. J., Hou, Y. K., Zou, Y. M., Zhou, Q., et al. (2022). NIR-PTT/ROS-Scavenging/Oxygen-Enriched synergetic therapy for rheumatoid arthritis by a pH-responsive hybrid CeO(2)-ZIF-8 coated with polydopamine. ACS Biomater. Sci. Eng. 8 (8), 3361–3376. doi:10.1021/acsbiomaterials.2c00592
Cheng, Y., Cheng, H., Jiang, C., Qiu, X., Wang, K., Huan, W., et al. (2015). Perfluorocarbon nanoparticles enhance reactive oxygen levels and tumour growth inhibition in photodynamic therapy. Nat. Commun. 6, 8785. doi:10.1038/ncomms9785
Chu, X., Mao, L., Johnson, O., Li, K., Phan, J., Yin, Q., et al. (2019). Exploration of TiO(2) nanoparticle mediated microdynamic therapy on cancer treatment. Nanomedicine Nanotechnol. Biol. Med. 18, 272–281. doi:10.1016/j.nano.2019.02.016
Crivelli, B., Bari, E., Perteghella, S., Catenacci, L., Sorrenti, M., Mocchi, M., et al. (2019). Silk fibroin nanoparticles for celecoxib and curcumin delivery: ROS-scavenging and anti-inflammatory activities in an in vitro model of osteoarthritis. Eur. J. Pharm. Biopharm. 137, 37–45. doi:10.1016/j.ejpb.2019.02.008
Ding, Y., Li, Z., Hu, W., Feng, X., Chen, Y., Yan, G., et al. (2021). Carbazate-modified cross-linked dextran microparticles suppress the progression of osteoarthritis by ROS scavenging. Biomater. Sci. 9 (18), 6236–6250. doi:10.1039/d1bm00743b
Duan, G., Wen, L., Sun, X., Wei, Z., Duan, R., Zeng, J., et al. (2022). Healing diabetic ulcers with MoO3−X nanodots possessing intrinsic ROS-scavenging and bacteria-killing capacities. Small (Weinheim der Bergstrasse, Ger. 18 (10), e2107137. doi:10.1002/smll.202107137
Einhorn, T. A., and Gerstenfeld, L. C. (2015). Fracture healing: Mechanisms and interventions. Nat. Rev. Rheumatol. 11 (1), 45–54. doi:10.1038/nrrheum.2014.164
El-Rashidy, A. A., Roether, J. A., Harhaus, L., Kneser, U., and Boccaccini, A. R. (2017). Regenerating bone with bioactive glass scaffolds: A review of in vivo studies in bone defect models. Acta biomater. 62, 1–28. doi:10.1016/j.actbio.2017.08.030
Fresneda Alarcon, M., McLaren, Z., and Wright, H. L. (2021). Neutrophils in the pathogenesis of rheumatoid arthritis and systemic lupus erythematosus: Same foe different M.O. Front. Immunol. 12, 649693. doi:10.3389/fimmu.2021.649693
Fu, J., Li, T., Zhu, Y., and Hao, Y. (2019). Ultrasound-Activated oxygen and ROS generation nanosystem systematically modulates tumor microenvironment and sensitizes sonodynamic therapy for hypoxic solid tumors. Adv. Funct. Mat. 29 (51), 1906195. doi:10.1002/adfm.201906195
Fu, L., Zhang, W., Zhou, X., Fu, J., and He, C. (2022). Tumor cell membrane-camouflaged responsive nanoparticles enable MRI-guided immuno-chemodynamic therapy of orthotopic osteosarcoma. Bioact. Mater. 17, 221–233. doi:10.1016/j.bioactmat.2022.01.035
Gao, X., Ma, Y., Zhang, G., Tang, F., Zhang, J., Cao, J., et al. (2020). Targeted elimination of intracellular reactive oxygen species using nanoparticle-like chitosan- superoxide dismutase conjugate for treatment of monoiodoacetate-induced osteoarthritis. Int. J. Pharm. 590, 119947. doi:10.1016/j.ijpharm.2020.119947
Garrido-Pérez, N., Vela-Sebastián, A., López-Gallardo, E., Emperador, S., Iglesias, E., Meade, P., et al. (2020). Oxidative phosphorylation dysfunction modifies the cell secretome. Int. J. Mol. Sci. 21 (9), 3374. doi:10.3390/ijms21093374
Golovynska, I., Golovynskyi, S., and Qu, J. (2022). Comparing the impact of NIR, visible and UV light on ROS upregulation via photoacceptors of mitochondrial complexes in normal, immune and cancer cells. Photochem. Photobiol. [Online ahead of print] doi:10.1111/php.13661
Gordijo, C. R., Abbasi, A. Z., Amini, M. A., Lip, H. Y., Maeda, A., Cai, P., et al. (2015). Design of hybrid MnO2-polymer-lipid nanoparticles with tunable oxygen generation rates and tumor accumulation for cancer treatment. Adv. Funct. Mat. 25 (12), 1858–1872. doi:10.1002/adfm.201404511
Gui, T., Luo, L., Chhay, B., Zhong, L., Wei, Y., Yao, L., et al. (2022). Superoxide dismutase-loaded porous polymersomes as highly efficient antioxidant nanoparticles targeting synovium for osteoarthritis therapy. Biomaterials 283, 121437. doi:10.1016/j.biomaterials.2022.121437
Harman, D. (2003). The free radical theory of aging. Antioxidants redox Signal. 5 (5), 557–561. doi:10.1089/152308603770310202
He, Z., Xu, Q., Newland, B., Foley, R., Lara-Sáez, I., Curtin, J. F., et al. (2021). Reactive oxygen species (ROS): Utilizing injectable antioxidative hydrogels and ROS-producing therapies to manage the double-edged sword. J. Mat. Chem. B 9 (32), 6326–6346. doi:10.1039/d1tb00728a
Hosseinzadeh, A., Kamrava, S. K., Joghataei, M. T., Darabi, R., Shakeri-Zadeh, A., Shahriari, M., et al. (2016). Apoptosis signaling pathways in osteoarthritis and possible protective role of melatonin. J. Pineal Res. 61 (4), 411–425. doi:10.1111/jpi.12362
Huang, P., Qian, X., Chen, Y., Yu, L., Lin, H., Wang, L., et al. (2017). Metalloporphyrin-encapsulated biodegradable nanosystems for highly efficient magnetic resonance imaging-guided sonodynamic cancer therapy. J. Am. Chem. Soc. 139 (3), 1275–1284. doi:10.1021/jacs.6b11846
Huang, X., Chen, J., Wu, W., Yang, W., Zhong, B., Qing, X., et al. (2020). Delivery of MutT homolog 1 inhibitor by functionalized graphene oxide nanoparticles for enhanced chemo-photodynamic therapy triggers cell death in osteosarcoma. Acta biomater. 109, 229–243. doi:10.1016/j.actbio.2020.04.009
Huang, X., He, D., Pan, Z., Luo, G., and Deng, J. (2021). Reactive-oxygen-species-scavenging nanomaterials for resolving inflammation. Mater. today Bio 11, 100124. doi:10.1016/j.mtbio.2021.100124
Ilyas, A., Velton, M., Shah, A., Monte, F., Kim, H. K. W., Aswath, P. B., et al. (2019). Rapid regeneration of vascularized bone by nanofabricated amorphous silicon oxynitrophosphide (SiONP) overlays. J. Biomed. Nanotechnol. 15 (6), 1241–1255. doi:10.1166/jbn.2019.2779
Khalil, I., Yehye, W. A., Etxeberria, A. E., Alhadi, A. A., Dezfooli, S. M., Julkapli, N. B. M., et al. (2020). Nanoantioxidants: Recent trends in antioxidant delivery applications. Antioxidants (Basel). 9 (1), 24. doi:10.3390/antiox9010024
Kim, J., Kim, H. Y., Song, S. Y., Go, S. H., Sohn, H. S., Baik, S., et al. (2019). Synergistic oxygen generation and reactive oxygen species scavenging by manganese ferrite/ceria Co-decorated nanoparticles for rheumatoid arthritis treatment. ACS Nano 13 (3), 3206–3217. doi:10.1021/acsnano.8b08785
Kiyani, M. M., Butt, M. A., Rehman, H., Ali, H., Hussain, S. A., Obaid, S., et al. (2019). Antioxidant and anti-gout effects of orally administered zinc oxide nanoparticles in gouty mice. J. Trace Elem. Med. Biol. 56, 169–177. doi:10.1016/j.jtemb.2019.08.012
Koo, S., Park, O. K., Kim, J., Han, S. I., Yoo, T. Y., Lee, N., et al. (2022). Enhanced chemodynamic therapy by Cu-Fe peroxide nanoparticles: Tumor microenvironment-mediated synergistic Fenton reaction. ACS Nano 16 (2), 2535–2545. doi:10.1021/acsnano.1c09171
Kumar, S., Adjei, I. M., Brown, S. B., Liseth, O., and Sharma, B. (2019). Manganese dioxide nanoparticles protect cartilage from inflammation-induced oxidative stress. Biomaterials 224, 119467. doi:10.1016/j.biomaterials.2019.119467
Kwiatkowski, S., Knap, B., Przystupski, D., Saczko, J., Kędzierska, E., Knap-Czop, K., et al. (2018). Photodynamic therapy - mechanisms, photosensitizers and combinations. Biomed. Pharmacother. 106, 1098–1107. doi:10.1016/j.biopha.2018.07.049
Lee, H. R., Kim, D. W., Jones, V. O., Choi, Y., Ferry, V. E., Geller, M. A., et al. (2021). Sonosensitizer-Functionalized graphene nanoribbons for adhesion blocking and sonodynamic ablation of ovarian cancer spheroids. Adv. Healthc. Mat. 10 (13), e2001368. doi:10.1002/adhm.202001368
Lee, P. C., Zan, B. S., Chen, L. T., and Chung, T. W. (2019). Multifunctional PLGA-based nanoparticles as a controlled release drug delivery system for antioxidant and anticoagulant therapy. Int. J. Nanomedicine 14, 1533–1549. doi:10.2147/ijn.s174962
Lepetsos, P., and Papavassiliou, A. G. (2016). ROS/oxidative stress signaling in osteoarthritis. Biochimica Biophysica Acta - Mol. Basis Dis. 1862 (4), 576–591. doi:10.1016/j.bbadis.2016.01.003
Lespasio, M. J., Sodhi, N., and Mont, M. A. (2019). Osteonecrosis of the hip: A primer. Perm. J. 23, 18. doi:10.7812/tpp/18-100
Li, H., Bai, Z., Li, C., Sheng, C., and Zhao, X. (2020). EV71 infection induces cell apoptosis through ROS generation and SIRT1 activation. J. Cell. Biochem. 121 (10), 4321–4331. doi:10.1002/jcb.29628
Li, H., Xia, P., Pan, S., Qi, Z., Fu, C., Yu, Z., et al. (2020). The advances of ceria nanoparticles for biomedical applications in orthopaedics. Int. J. Nanomedicine 15, 7199–7214. doi:10.2147/ijn.s270229
Li, J. X., Zhang, L. M., Liu, C. C., Wu, Q. N., Li, S. P., Lei, X. P., et al. (2021). Doxorubicin-loaded hydrogen peroxide self-providing copper nanodots for combination of chemotherapy and acid-induced chemodynamic therapy against breast cancer. J. colloid interface Sci. 593, 323–334. doi:10.1016/j.jcis.2021.02.085
Li, M., Hao, L., Li, L., Liu, L., Chen, G., Jiang, W., et al. (2020). Cinnamtannin B-1 prevents ovariectomy-induced osteoporosis via attenuating osteoclastogenesis and ROS generation. Front. Pharmacol. 11, 1023. doi:10.3389/fphar.2020.01023
Li, Y., Chen, M., Yan, J., Zhou, W., Gao, S., Liu, S., et al. (2021). Tannic acid/Sr(2+)-coated silk/graphene oxide-based meniscus scaffold with anti-inflammatory and anti-ROS functions for cartilage protection and delaying osteoarthritis. Acta biomater. 126, 119–131. doi:10.1016/j.actbio.2021.02.046
Li, Y., Zhou, Q., Deng, Z., Pan, M., Liu, X., Wu, J., et al. (2016). IR-780 dye as a sonosensitizer for sonodynamic therapy of breast tumor. Sci. Rep. 6, 25968. doi:10.1038/srep25968
Lin, Y., Yi, O., Hu, M., Hu, S., Su, Z., Liao, J., et al. (2022). Multifunctional nanoparticles of sinomenine hydrochloride for treat-to-target therapy of rheumatoid arthritis via modulation of proinflammatory cytokines. J. Control. Release 348, 42–56. doi:10.1016/j.jconrel.2022.05.016
Lu, J., Wang, Z., Cao, J., Chen, Y., and Dong, Y. (2018). A novel and compact review on the role of oxidative stress in female reproduction. Reprod. Biol. Endocrinol. 16 (1), 80. doi:10.1186/s12958-018-0391-5
Lu, S. L., Wang, Y. H., Liu, G. F., Wang, L., Li, Y., Guo, Z. Y., et al. (2021). Graphene oxide nanoparticle-loaded ginsenoside Rg3 improves photodynamic therapy in inhibiting malignant progression and stemness of osteosarcoma. Front. Mol. Biosci. 8, 663089. doi:10.3389/fmolb.2021.663089
Ludovici, G. M., Oliveira de Souza, S., Chierici, A., Cascone, M. G., d'Errico, F., and Malizia, A. (2020). Adaptation to ionizing radiation of higher plants: From environmental radioactivity to chernobyl disaster. J. Environ. Radioact. 222, 106375. doi:10.1016/j.jenvrad.2020.106375
Luo, M., Li, H., Han, D., Yang, K., and Kang, L. (2021). Inhibition of autophagy enhances apoptosis induced by Ce6-photodynamic therapy in human colon cancer cells. Photodiagnosis Photodyn. Ther. 36, 102605. doi:10.1016/j.pdpdt.2021.102605
Madreiter-Sokolowski, C. T., Thomas, C., and Ristow, M. (2020). Interrelation between ROS and Ca(2+) in aging and age-related diseases. Redox Biol. 36, 101678. doi:10.1016/j.redox.2020.101678
Matsuoka, Y., and Yamada, K. I. (2021). Detection and structural analysis of lipid-derived radicals in vitro and in vivo. Free Radic. Res. 55 (4), 441–449. doi:10.1080/10715762.2021.1881500
McHale, A. P., Callan, J. F., Nomikou, N., Fowley, C., and Callan, B. (2016). Sonodynamic therapy: Concept, mechanism and application to cancer treatment. Adv. Exp. Med. Biol. 880, 429–450. doi:10.1007/978-3-319-22536-4_22
Mitsunaga, M., Ogawa, M., Kosaka, N., Rosenblum, L. T., Choyke, P. L., and Kobayashi, H. (2011). Cancer cell-selective in vivo near infrared photoimmunotherapy targeting specific membrane molecules. Nat. Med. 17 (12), 1685–1691. doi:10.1038/nm.2554
Mu, J., Zhao, X., Li, J., Yang, E. C., and Zhao, X. J. (2016). Novel hierarchical NiO nanoflowers exhibiting intrinsic superoxide dismutase-like activity. J. Mat. Chem. B 4 (31), 5217–5221. doi:10.1039/c6tb01390b
Newland, B., Wolff, P., Zhou, D., Wang, W., Zhang, H., Rosser, A., et al. (2016). Synthesis of ROS scavenging microspheres from a dopamine containing poly(β-amino ester) for applications for neurodegenerative disorders. Biomater. Sci. 4 (3), 400–404. doi:10.1039/c5bm00542f
Nomura, M., Yoshimura, Y., Kikuiri, T., Hasegawa, T., Taniguchi, Y., Deyama, Y., et al. (2011). Platinum nanoparticles suppress osteoclastogenesis through scavenging of reactive oxygen species produced in RAW264.7 cells. J. Pharmacol. Sci. 117 (4), 243–252. doi:10.1254/jphs.11099fp
Ocsoy, I., Isiklan, N., Cansiz, S., Özdemir, N., and Tan, W. (2016). ICG-Conjugated magnetic graphene oxide for dual photothermal and photodynamic therapy. RSC Adv. 6 (36), 30285–30292. doi:10.1039/c6ra06798k
Ouyang, J., Tang, Z., Farokhzad, N., Kong, N., Kim, N. Y., Feng, C., et al. (2020). Ultrasound mediated therapy: Recent progress and challenges in nanoscience. Nano Today 35, 100949. doi:10.1016/j.nantod.2020.100949
Peng, P., Nie, Z., Sun, F., and Peng, H. (2021). Glucocorticoids induce femoral head necrosis in rats through the ROS/JNK/c-Jun pathway. FEBS open bio 11 (1), 312–321. doi:10.1002/2211-5463.13037
Pereira, D., Ramos, E., and Branco, J. (2015). Acta Med. Port. 28 (1), 99–106. doi:10.20344/amp.5477
Pinna, A., Torki Baghbaderani, M., Vigil Hernández, V., Naruphontjirakul, P., Li, S., McFarlane, T., et al. (2021). Nanoceria provides antioxidant and osteogenic properties to mesoporous silica nanoparticles for osteoporosis treatment. Acta biomater. 122, 365–376. doi:10.1016/j.actbio.2020.12.029
Rimal, B., Greenberg, A. K., and Rom, W. N. (2005). Basic pathogenetic mechanisms in silicosis: Current understanding. Curr. Opin. Pulm. Med. 11 (2), 169–173. doi:10.1097/01.mcp.0000152998.11335.24
Ronin, D., Boyer, J., Alban, N., Natoli, R. M., Johnson, A., and Kjellerup, B. V. (2022). Current and novel diagnostics for orthopedic implant biofilm infections: A review. APMIS acta pathologica, Microbiol. Immunol. Scand. 130 (2), 59–81. doi:10.1111/apm.13197
Saxena, P., Selvaraj, K., Khare, S. K., and Chaudhary, N. (2022). Superoxide dismutase as multipotent therapeutic antioxidant enzyme: Role in human diseases. Biotechnol. Lett. 44 (1), 1–22. doi:10.1007/s10529-021-03200-3
Scott, D. L., Wolfe, F., and Huizinga, T. W. (2010). Rheumatoid arthritis. Lancet 376 (9746), 1094–1108. doi:10.1016/s0140-6736(10)60826-4
Seo, J. J., Mandakhbayar, N., Kang, M. S., Yoon, J. Y., Lee, N. H., Ahn, J., et al. (2021). Antibacterial, proangiogenic, and osteopromotive nanoglass paste coordinates regenerative process following bacterial infection in hard tissue. Biomaterials 268, 120593. doi:10.1016/j.biomaterials.2020.120593
Sul, O. J., Kim, J. C., Kyung, T. W., Kim, H. J., Kim, Y. Y., Kim, S. H., et al. (2010). Gold nanoparticles inhibited the receptor activator of nuclear factor-κb ligand (RANKL)-induced osteoclast formation by acting as an antioxidant. Biosci. Biotechnol. Biochem. 74 (11), 2209–2213. doi:10.1271/bbb.100375
Tian, J., Mo, J., Xu, L., Zhang, R., Qiao, Y., Liu, B., et al. (2020). Scoulerine promotes cell viability reduction and apoptosis by activating ROS-dependent endoplasmic reticulum stress in colorectal cancer cells. Chemico-biological Interact. 327, 109184. doi:10.1016/j.cbi.2020.109184
Toker, H., Ozdemir, H., Balcı, H., and Ozer, H. (2012). N-acetylcysteine decreases alveolar bone loss on experimental periodontitis in streptozotocin-induced diabetic rats. J. periodontal Res. 47 (6), 793–799. doi:10.1111/j.1600-0765.2012.01497.x
Tsubata, T. (2020). Involvement of reactive oxygen species (ROS) in BCR signaling as a second messenger. Adv. Exp. Med. Biol. 1254, 37–46. doi:10.1007/978-981-15-3532-1_3
Tu, Y., Zhou, Y., Zhang, D., Yang, J., Li, X., Ji, K., et al. (2021). Light-induced reactive oxygen species (ROS) generator for tumor therapy through an ROS burst in mitochondria and AKT-inactivation-induced apoptosis. ACS Appl. Bio Mat. 4 (6), 5222–5230. doi:10.1021/acsabm.1c00386
Valko, M., Rhodes, C. J., Moncol, J., Izakovic, M., and Mazur, M. (2006). Free radicals, metals and antioxidants in oxidative stress-induced cancer. Chem. Biol. Interact. 160 (1), 1–40. doi:10.1016/j.cbi.2005.12.009
Wang, N., Song, L., Xu, Y., Zhang, L., Wu, Y., Guo, J., et al. (2019). Loss of Scribble confers cisplatin resistance during NSCLC chemotherapy via Nox2/ROS and Nrf2/PD-L1 signaling. EBioMedicine 47, 65–77. doi:10.1016/j.ebiom.2019.08.057
Wang, X. Q., Wang, W., Peng, M., and Zhang, X. Z. (2021). Free radicals for cancer theranostics. Biomaterials 266, 120474. doi:10.1016/j.biomaterials.2020.120474
Wu, M., Zhang, Z., Liu, Z., Zhang, J., Zhang, Y., Ding, Y., et al. (2021). Piezoelectric nanocomposites for sonodynamic bacterial elimination and wound healing. Nano Today 37, 101104. doi:10.1016/j.nantod.2021.101104
Wu, W., Yu, L., Jiang, Q., Huo, M., Lin, H., Wang, L., et al. (2019). Enhanced tumor-specific disulfiram chemotherapy by in situ Cu(2+) chelation-initiated nontoxicity-to-toxicity transition. J. Am. Chem. Soc. 141 (29), 11531–11539. doi:10.1021/jacs.9b03503
Wu, X., Zhou, X., Lai, S., Liu, J., and Qi, J. (2022). Curcumin activates Nrf2/HO-1 signaling to relieve diabetic cardiomyopathy injury by reducing ROS in vitro and in vivo. FASEB J. 36 (9), e22505. doi:10.1096/fj.202200543rrr
Xie, L., Yang, H., Wu, X., Wang, L., Zhu, B., Tang, Y., et al. (2022). Ti-MOF-based biosafety materials for efficient and long-life disinfection via synergistic photodynamic and photothermal effects. Biosaf. Health 4 (2), 135–146. doi:10.1016/j.bsheal.2022.02.001
Yang, K. C., Wu, C. C., Chen, W. Y., Sumi, S., and Huang, T. L. (2016). l-Glutathione enhances antioxidant capacity of hyaluronic acid and modulates expression of pro-inflammatory cytokines in human fibroblast-like synoviocytes. J. Biomed. Mat. Res. A 104 (8), 2071–2079. doi:10.1002/jbm.a.35729
Yang, N., Guo, H., Cao, C., Wang, X., Song, X., Wang, W., et al. (2021). Infection microenvironment-activated nanoparticles for NIR-II photoacoustic imaging-guided photothermal/chemodynamic synergistic anti-infective therapy. Biomaterials 275, 120918. doi:10.1016/j.biomaterials.2021.120918
Yang, Q., Peng, J., Xiao, Y., Li, W., Tan, L., Xu, X., et al. (2018). Porous Au@Pt nanoparticles: Therapeutic platform for tumor chemo-photothermal Co-therapy and alleviating doxorubicin-induced oxidative damage. ACS Appl. Mat. Interfaces 10 (1), 150–164. doi:10.1021/acsami.7b14705
Yang, Y., Guo, L., Wang, Z., Liu, P., Liu, X., Ding, J., et al. (2021). Targeted silver nanoparticles for rheumatoid arthritis therapy via macrophage apoptosis and Re-polarization. Biomaterials 264, 120390. doi:10.1016/j.biomaterials.2020.120390
Yao, Y., Zhang, H., Wang, Z., Ding, J., Wang, S., Huang, B., et al. (2019). Reactive oxygen species (ROS)-responsive biomaterials mediate tissue microenvironments and tissue regeneration. J. Mat. Chem. B 7 (33), 5019–5037. doi:10.1039/c9tb00847k
Yi, C., Yu, Z., Ren, Q., Liu, X., Wang, Y., Sun, X., et al. (2020). Nanoscale ZnO-based photosensitizers for photodynamic therapy. Photodiagnosis Photodyn. Ther. 30, 101694. doi:10.1016/j.pdpdt.2020.101694
Yu, P., Zheng, L., Wang, P., Chai, S., Zhang, Y., Shi, T., et al. (2020). Development of a novel polysaccharide-based iron oxide nanoparticle to prevent iron accumulation-related osteoporosis by scavenging reactive oxygen species. Int. J. Biol. Macromol. 165, 1634–1645. doi:10.1016/j.ijbiomac.2020.10.016
Yu, Z., Hu, Y., Sun, Y., and Sun, T. (2021). Chemodynamic therapy combined with multifunctional nanomaterials and their applications in tumor treatment. Chem. Eur. J. 27 (56), 13953–13960. doi:10.1002/chem.202101514
Yuan, Z., Lin, C., He, Y., Tao, B., Chen, M., Zhang, J., et al. (2020). Near-infrared light-triggered nitric-oxide-enhanced photodynamic therapy and low-temperature photothermal therapy for biofilm elimination. ACS Nano 14 (3), 3546–3562. doi:10.1021/acsnano.9b09871
Zeng, W. N., Yu, Q. P., Wang, D., Liu, J. L., Yang, Q. J., Zhou, Z. K., et al. (2021). Mitochondria-targeting graphene oxide nanocomposites for fluorescence imaging-guided synergistic phototherapy of drug-resistant osteosarcoma. J. Nanobiotechnology 19 (1), 79. doi:10.1186/s12951-021-00831-6
Zeng, Y., Du, Q., Zhang, Z., Ma, J., Han, L., Wang, Y., et al. (2020). Curcumin promotes cancer-associated fibroblasts apoptosis via ROS-mediated endoplasmic reticulum stress. Archives Biochem. biophysics 694, 108613. doi:10.1016/j.abb.2020.108613
Zhang, X., Feng, L., Dong, Z., Xin, X., Yang, Z., Deng, D., et al. (2020). Protein-drug conjugate programmed by pH-reversible linker for tumor hypoxia relief and enhanced cancer combination therapy. Int. J. Pharm. 582, 119321. doi:10.1016/j.ijpharm.2020.119321
Zhao, H., Li, X., Zhang, D., Chen, H., Chao, Y., Wu, K., et al. (2018). Integrative bone metabolomics-lipidomics strategy for pathological mechanism of postmenopausal osteoporosis mouse model. Sci. Rep. 8 (1), 16456. doi:10.1038/s41598-018-34574-6
Zhao, H., Zeng, Z., Liu, L., Chen, J., Zhou, H., Huang, L., et al. (2018). Polydopamine nanoparticles for the treatment of acute inflammation-induced injury. Nanoscale 10 (15), 6981–6991. doi:10.1039/c8nr00838h
Zhao, K., Wang, Y., Lu, M., Yao, K., Xiao, C., Zhou, Y., et al. (2018). Progress in repair and reconstruction of large segmental bone tumor defect in distal tibia. Zhongguo xiu fu chong jian wai ke za zhi = Zhongguo xiufu chongjian waike zazhi = Chin. J. reparative Reconstr. Surg. 32 (9), 1211–1217. doi:10.7507/1002-1892.201803007
Zhao, W., Wang, H., Han, Y., Wang, H., Sun, Y., and Zhang, H. (2020). Dopamine/phosphorylcholine copolymer as an efficient joint lubricant and ROS scavenger for the treatment of osteoarthritis. ACS Appl. Mat. Interfaces 12 (46), 51236–51248. doi:10.1021/acsami.0c14805
Zheng, J., Zhang, J., Zhang, X., Guo, Z., Wu, W., Chen, Z., et al. (2021). Reactive oxygen species mediate low back pain by upregulating substance P in intervertebral disc degeneration. Oxidative Med. Cell. Longev. 2021, 1–11. doi:10.1155/2021/6681815
Zhong, G., Yang, X., Jiang, X., Kumar, A., Long, H., Xie, J., et al. (2019). Dopamine-melanin nanoparticles scavenge reactive oxygen and nitrogen species and activate autophagy for osteoarthritis therapy. Nanoscale 11 (24), 11605–11616. doi:10.1039/c9nr03060c
Zhou, T., Yang, X., Chen, Z., Yang, Y., Wang, X., Cao, X., et al. (2022). Prussian blue nanoparticles stabilize SOD1 from ubiquitination-proteasome degradation to rescue intervertebral disc degeneration. Adv. Sci. (Weinheim, Baden-Wurttemberg, Ger. 9 (10), e2105466. doi:10.1002/advs.202105466
Zhou, X., Zhang, Y., Hou, M., Liu, H., Yang, H., Chen, X., et al. (2022). Melatonin prevents cartilage degradation in early-stage osteoarthritis through activation of miR-146a/NRF2/HO-1 Axis. J. Bone Min. Res. 37 (5), 1056–1072. doi:10.1002/jbmr.4527
Zhou, Z., Gong, F., Zhang, P., Wang, X., Zhang, R., Xia, W., et al. (2022). Natural product curcumin-based coordination nanoparticles for treating osteoarthritis via targeting Nrf2 and blocking NLRP3 inflammasome. Nano Res. 15 (4), 3338–3345. doi:10.1007/s12274-021-3864-3
Keywords: nanomaterials, ROS regulation, therapy, review, orthopaedic related diseases
Citation: Yang W, Zeng Q, Pan Q, Huang W, Hu H and Shao Z (2022) Application and prospect of ROS-related nanomaterials for orthopaedic related diseases treatment. Front. Chem. 10:1035144. doi: 10.3389/fchem.2022.1035144
Received: 02 September 2022; Accepted: 16 September 2022;
Published: 05 October 2022.
Edited by:
Daoming Zhu, Southern Medical University, ChinaReviewed by:
Qiuyue Ding, Guizhou Provincial People’s Hospital, ChinaXiaowei Zeng, Sun Yat-sen University, China
Yongzhi Cui, Shanghai Jiao Tong University, China
Copyright © 2022 Yang, Zeng, Pan, Huang, Hu and Shao. This is an open-access article distributed under the terms of the Creative Commons Attribution License (CC BY). The use, distribution or reproduction in other forums is permitted, provided the original author(s) and the copyright owner(s) are credited and that the original publication in this journal is cited, in accordance with accepted academic practice. No use, distribution or reproduction is permitted which does not comply with these terms.
*Correspondence: Zengwu Shao, c3p3cHJvQDE2My5jb20=; Hongzhi Hu, MTM3MjAxMDU3NDZAcXEuY29t; Wei Huang, aHVhbmd3ZWlfeGhAMTI2LmNvbQ==
†These authors have contributed equally to this work