- 1Department of Nuclear Medicine, Xiangya Hosptal, Central South University, Changsha, Hunan, China
- 2Key Laboratory of Nanobiological Technology of National Health Commission, Xiangya Hospital, Central South University, Changsha, Hunan, China
- 3National Clinical Research Center for Geriatric Disorders, Xiangya Hospital, Central South University, Changsha, Hunan, China
With high mortality, stroke has become a serious threat to human health. Nevertheless, the strategy for stroke therapy is quite limited in the clinic till now. In this research, we prepared a novel neuroprotective nanoformulation (OEA Liposomes) via encapsulating endogenous N-oleoylethanolamine (OEA) in liposomes for intravenous administration. The formulation largely increased the solubility and bioavailability of OEA. Then the following systematic experiments stated the excellent neuroprotective effect of OEA Liposomes in vivo. The survival rate of the nanodrug group was largely increased to 75%, while that of the Middle Cerebral Artery Occlusion (MCAO) group was only 41.7%. And the severe neurological functional deficit of the MCAO rats was also significantly improved. What’s more, the OEA Liposomes could inhibit the apoptosis of neurons and the inflammation of reperfusion to a very slight level, indicating their outstanding neuroprotective effect. These results indicated that the OEA Liposomes have a great potential for clinic anti-stroke application.
Introduction
With high mortality, stroke has been a leading cause of death worldwide. Plus the high rate of long-term disability, stroke has become a serious threat to human health (Centers for Disease Control and Prevention, 2015) (Centers for Disease Control and Prevention (CDC), 2009). What’s worse, the research in clinical stroke trials progressed slowly or stalled, and only a few effective formulations was available in the clinic now (Lo et al., 2003; Winstein et al., 2016).
When the research of neuroprotective drugs still remains elusive, our research group found that N-oleoylethanolane possessed an excellent neuroprotective effect (Zhou et al., 2012; Yang et al., 2016; Zhou et al., 2017a). As an endogenous peroxisome proliferator-activated receptor alpha (PPAR-α) agonist, OEA could protect against acute and chronic ischemic injury (Yang et al., 2019). The mechanism is that the activation of PPAR α could significantly inhibit the inflammation induced by ischemia reperfusion, which is one of the primary causes of severe brain damage (Zhou et al., 2017b; Luo et al., 2019). More strikingly, chronic administration of OEA improved spatial cognitive impairments and even promoted neurogenesis and neuroplasticity in MCAO rats (Yang et al., 2015a). Apart from other synthetic neuroprotective drugs, OEA is an endogenous compound and possesses superior effectiveness and safety. Therefore, OEA is a promising neuro-protector and may have potential applications in stroke therapy.
However, the solubility of OEA is extremely low, which has been a major obstacle to its absorption. And OEA could not take part in the salt-forming reaction, which is a common strategy to enhance the solubility of the drug candidate in formulation development (Lipinski, 2000). These poorly water-soluble candidates are usually dismissed at the beginning of pharmacological characterization (Porter et al., 2008). However, it is precious to find an endogenous compound with a nice neuroprotective effect. Hence, nanoparticles drug delivery system (NDDS) was employed to improve the pharmaceutical properties of OEA (Chen et al., 2018; Wu et al., 2022). Among all kinds of NDDS, liposomes or lipidic nanoparticles are the first nanomedicine delivery system passed the clinical trials, which has been an established technology platform with considerable clinical acceptance (Wicki et al., 2015). More and more liposomal formulations are in the pipeline now, which would enter the market in the near future (Tran et al., 2017). Through decades of research, many important technical advances in liposome preparation were developed, such as remote drug loading, long-circulating liposomes, nucleic acid loaded liposomes, and so on (Lukyanov and Torchilin, 2004). One particularly important advance was the extrusion for homogeneous size, which played a paramount role in the transition of the liposomes from concept to clinical application (Allen and Cullis, 2013; Bulbake et al., 2017). What’s more, many complex liposomes have been designed and prepared, such as ligand-targeted liposomes (Lv et al., 2018), and triggered release liposomes (Zhang et al., 2018; Ansari et al., 2021). Although they were not applied in the clinic now, these advances led to numerous clinical trials and more potential applications in the delivery of anti-cancer (Cao et al., 2016; Kiaie et al., 2020), anti-biotic (Shen et al., 2022), anesthetics (Ruokonen et al., 2017), and anti-inflammatory drugs (Mokdad et al., 2022).
In this paper, OEA was successfully encapsulated in PEGylated liposomes to improve its pharmaceutical properties. Through extrusion, the OEA Liposomes possessed a homogeneous and uniform size of 97.6 ± 4.8 nm, which met the standard of injection and could be intravenously administrated to the rats. The in vitro drug release study indicated the controlled and sustained drug release property of OEA Liposomes. And the in vivo neuroprotective effects were systemically evaluated. The results suggested that the OEA Liposomes could greatly increase the survival rate of MCAO rats and improve their neurological functional deficit scores. Significantly, the OEA Liposomes could inhibit the apoptosis of neurons and the inflammation of reperfusion to a very slight level, indicating their outstanding neuroprotective effect. The research stated that the OEA Liposomes might process the clinic application of OEA and become a promising anti-stroke NDDS.
Materials and methods
Materials
OEA (purity grade > 90.0%), SPC (purity grade >90%), and cholesterin (purity grade >90%) were purchased from Shanghai Macklin Biochemical Co., Ltd. (Shanghai, China). DSPE-PEG (MWCO 2000) was obtained from Ponsure Biotechnology Co., Ltd. (Shanghai, China). Trichloromethane was purchased from Sinopharm Chemical Reagent Co., Ltd. All chemical reagents were of analytical grade and used without further purification unless otherwise stated.
Preparation and characterization of OEA liposomes
The OEA Liposomes were prepared via the thin film hydration method coupled with sequential extrusion. Briefly, OEA (5 mg), cholesterin (5 mg), SPC (5 mg), and DSPE-PEG (35 mg) were dissolved in trichloromethane, which was evaporated under vacuum for 8 h to prepare a dry film. PBS (100 ml, pH 7.4) was added under sonication for 10 min, and the dispersion was intensely stirred for 2 h. Lastly, the dispersion was extruded through polycarbonate filters of decreasing pore diameters of 0.8 μm/0.4 μm/0.2 μm (three passages through each) to obtain liposomes with uniform size.
Morphology of the OEA Liposomes was examined by TEM (Tecnai G2 F20, United States). The Size of the OEA Liposomes was determined by Malvern Zetasizer Nano-ZS (Malvern Instruments, Malvern). The drug loading content of OEA in OEA Liposomes was determined by liquid chromatography-mass spectrometry (LCMS ORBITRAP VELOS PRO ETD, United States). The drug loading and encapsulation efficiency was calculated by Eqs 1, 2, respectively.
The in vitro drug release study
The in vitro drug release studies of OEA Liposomes were performed via the dialysis technique. Free OEA and OEA Liposomes were dispersed in PBS (10.0 ml) and placed in dialysis bags (MWCO 3500 Da). Then, the bags were immersed in PBS (150 ml, pH 7.4) containing 0.5% w/v Tween 80 and continuously oscillated in a shaker incubator (180 rpm, 37°C). All samples were assayed by HPLC.
Drug administration
The OEA Liposomes (0.5 mg/ml) were dissolved in saline under sonication. OEA (0.20 mg/ml) and Tween 80 were dissolved in saline under strong shaking. Drugs (1.5 mg/kg, iv) were administered with reperfusion, and daily for 14 consecutive days after reperfusion.
Preparation of the focal cerebral ischemia model
Focal cerebral ischemia was induced by middle cerebral artery occlusion in adult male SD rats (200–220 g), as previously described. Briefly, rats were anesthetized with chloral hydrate (350 mg/kg, ip). The silicon rubber-coated nylon monofilament was inserted into the left internal carotid artery (ICA) through the external carotid stump (ECA), and passed the ECA/ICA bifurcation to occlude the origin of the middle cerebral artery (MCA) at the junction of the circle of Willis. The monofilament was kept for 90 min and then withdrawn. Sham-operated rats were treated with an identical surgery without inserting the intraluminal filament. Throughout the procedure, body temperature was maintained at 37°C ± 0.5°C. Animals were excluded if hemorrhage was found in the brain slices or at the base of the circle of Willis during postmortem examination.
Measurement of the survival rate
The survival rate of the rats was recorded. (n = 12 rats for the sham group and the OEA Liposomes group, and n = 24 rats for the MCAO group and the free OEA group). The rats were involved in the following experiments.
Evaluation of neurological deficit
At 1, 3, 5, 7, and 14 days after reperfusion, rats (n = 8) were evaluated neurologically with the Garcia method (Garcia et al., 1995; Shimamura et al., 2006; Sozen et al., 2009) by one single examiner who was blinded to the animal groups.
Immunofluorescence staining and cell counting
The MCAO rats (21 days after reperfusion) were sacrificed and their brains were stained with Immunofluorescence (TUNEL, Iba-1 and GFAP). The number of TUNEL, GFAP, or Iba1 positive cells was obtained by fluorescence confocal microscopy (EX61, Olympus, Tokyo, Japan). The positive area was counted in three randomly chosen squares of identical size (460 μm × 460 μm).
Statistical analysis
The statistical significance of treatment outcomes was assessed using one-way/two-way analysis of variance for the differences within treatments followed by Tukey’s post hoc test (Prism 7 for Windows, GraphPad Software Inc., United States); p < 0.05 was considered statistically significant in all analyses (95% confidence level).
Results and discussions
Preparation and characterization of OEA liposomes
The OEA Liposomes were prepared via the thin film hydration method coupled with sequential extrusion. Simply, OEA (5 mg), cholesterin (5 mg), SPC (5 mg), and DSPE-PEG (35 mg) were dissolved in trichloromethane, which was evaporated under vacuum for 8 h to prepare a dry film. Then, the film was hydrated by adding buffer (pH 7.4) and intensely stirring. Last, the dispersion was extruded through polycarbonate filters of decreasing pore diameters of 0.8 μm/0.4 μm/0.2 μm (three passages through each) to obtain liposomes with uniform size. The TEM images indicated that the OEA Liposomes were nearly spherical in shape and possessed a size of approximately 70 nm (Figure 1). The DLS result indicated that the OEA Liposomes possessed a hydrodynamic size of 97.6 ± 4.8 nm and a PDI of 0.193 (Figure 2A). Since the retention of highly hydrophobic drugs in liposomes was always problematic (Zhigaltsev et al., 2010; Wu et al., 2021), the drug loading and loading efficiency of highly hydrophobic OEA was relatively low. The drug loading of OEA in the liposomes was 3.32% ± 0.46%. The loading efficiency of OEA in the liposomes were determined to be 28.3% ± 3. 4%.
In vitro drug release of OEA liposomes
The in vitro release study of OEA from OEA Liposomes was performed via a dialysis technique, alongside with free OEA. All samples were assayed by HPLC. Since OEA was a highly hydrophobic compound, 0.5% w/v Tween 80 was added to create a sink condition (Wang et al., 2011). As shown in Figure 2B, about 50% of the OEA was released at 4 h, indicating the severe burst release of free OEA. And the whole release of free OEA exhibited an evident “fast followed by slow” type. In comparison, the OEA Liposomes showed a remarkably prolonged and sustained release profile during the release period. Since the drug release was largely affected by the drug loading, the low content of OEA in OEA Liposomes also contributed to the sustained drug release (Yang et al., 2015b). Plus the controlled release property of liposomes, the in vitro release of OEA Liposomes was close to a zero-order release.
The survival rate
As an acute central nervous system disease, ischemic stroke possessed high mortality. Hence, reducing death rates must be the primary task to a formulation for stroke. So the survival rate of the MCAO rats injected with the indicated formulations {0.9% NaCl [MCAO], free OEA, and OEA Liposomes, [(OEA) = 1.5 mg/kg]} was recorded. As shown in Figure 3A, only 41.7% of the MCAO rats without treatment could survive for 14 days, indicating the severe damage and high mortality of MCAO. The injection of free OEA could not significantly improve this data, and there were still more than half of the MCAO rats who died within 14 days. In comparison, OEA Liposomes could obviously increase the survival rate to 75%. Only three MCAO rats injected with OEA Liposomes died from the cerebral injury. The result indicated that the administration of OEA Liposomes could build up resistance to cerebral ischemia significantly and extend the time window for beneficial reperfusion (Campbell et al., 2019).
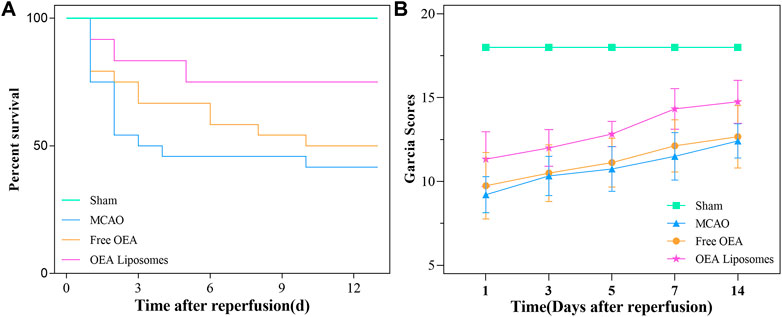
FIGURE 3. The survival rate (A) and the Garcia scores (B) of the MCAO rats administrated with the indicated formulations.
The behavioral assessment
In the clinic, the behavior of the patients was one of the clear indicators for illness assessment (Shea et al., 1992). Then the neurological functional deficit scores of the MCAO rats were assessed via the Garcia method. The Garcia scores of the MCAO rats administrated with the indicated formulations were evaluated at 1, 3, 5, 7, and 14 days after reperfusion. As shown in Figure 3B, the sham-operated rats got full marks throughout the estimate, indicating their normal brain condition. The MCAO rats without treatment got about nine points at 1 day after reperfusion, stating their deficient neurological function. Although the Garcia score of the rats in this group increased gradually, they did not get more than 13 points in the next assessments. The result suggested that the brain damage caused by MCAO was severe and hard to recover. And the MCAO rats injected with free OEA got almost the same scores as those without treatment, indicating that the administration of OEA did not obviously improve the neurological functional deficit caused by MCAO. On the contrary, the MCAO rats administrated with OEA Liposomes got significantly higher scores than the other operation rats (p < 0.01). The results illustrated that OEA could significantly mitigate and ameliorate the neurological function deficit caused by MCAO and the subsequent reperfusion.
The apoptosis of neurons
The neurological function deficit might come from the apoptosis of neurons, which were really hard to recover and would lead to intractable sequelae (Dirnagl et al., 1999; Tuo et al., 2022). Herein, TUNEL staining was employed to evaluate the ischemia reperfusion induced apoptosis in the cerebral cortex. As shown in Figures 4, 5, there was no apoptotic cell in the cerebral cortex of the sham-operated rats, suggesting their good brain condition. And significantly increased apoptotic cells were observed in the rats’ brains of the MCAO group and OEA group. The result indicated that the ischemia reperfusion indeed induced the apoptosis of the neurons, and this could not be improved by the injection of free OEA. On the contrary, the administration of OEA Liposomes could obviously protect the neurons from apoptosis, and only one apoptotic cell was observed in the field. The result indicated the excellent neuroprotective effect of OEA Liposomes.
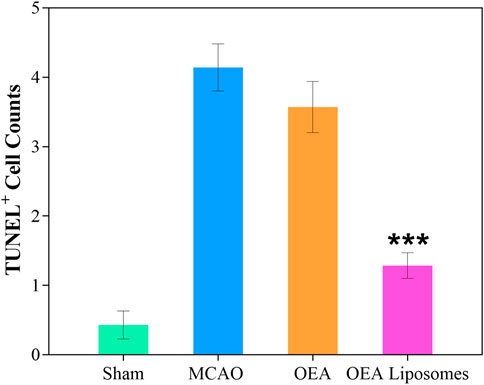
FIGURE 5. The statistical data (D) of the cortex stained with TUNEL. Data are expressed as mean ± SEM. *** = p < 0.001, compared to MCAO group).
The inflammation
According to our previous study, the OEA works as a neuroprotective candidate by inhibiting the inflammation, which is one of the primary causes of severe brain damage. The inflammation in the brain was mainly mediated by microglia and astrocyte, which would be specifically marked by Iba-1 and GFAP, respectively. Here, the Iba-1 and GFAP in the cortex were marked to access the inflammation after reperfusion. As depicted in Figures 6, 7, both markers indicated that the brain from the rats of the MCAO group or the OEA group possessed a largely increased inflammation compared with the Sham group. However, the administration of OEA Liposomes could significantly inhibit the inflammation caused by ischemia reperfusion, which was almost as slight as that of the Sham group. The result suggested that the OEA Liposomes could inhibit the inflammation of ischemia reperfusion to a very slight level, and therefore, provide significant neuroprotective effects.
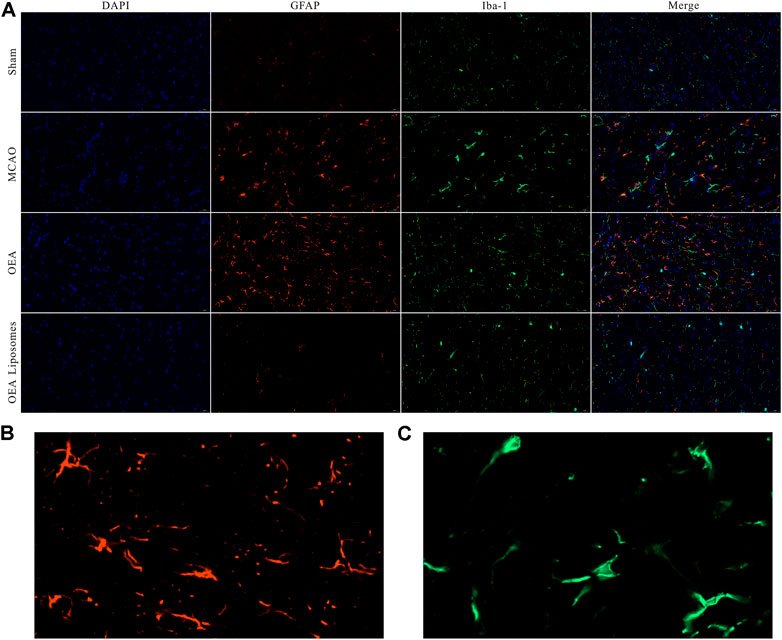
FIGURE 6. (A) The area of IBA-1 and GFAP positive stain from the cortex around the ischemic focus. (B–C) The enlarged pictures of IBA-1 and GFAP positive area.
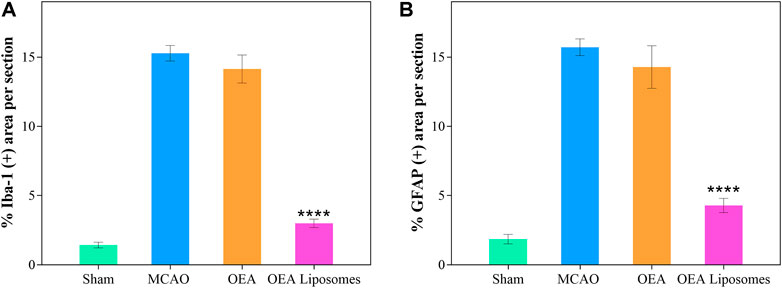
FIGURE 7. The statistical data of IBA-1 (A) and GFAP (B) positive stain from the cortex around the ischemic focus. Data are expressed as mean ± SEM. (**** = p < 0.0001, compared to MCAO group).
Conclusion
In summary, the study demonstrates OEA loaded liposomes for efficient stroke therapy. The highly hydrophobic property of OEA largely limited the clinic application of OEA. And the encapsulation of OEA into liposomes obviously increased its solubility and provide a sustained drug release property. Hence, the bioavailability was largely enhanced. The in vivo assessments indicated that the survival rate and the behavioral score of the MCAO rats were significantly improved via the administration of OEA Liposomes. What’s more, the apoptosis of the neurons and the inflammation in the cerebral cortex were significantly inhibited to a slight level. These results strongly suggested that the OEA Liposomes had potential applications for stroke therapy, and might give impetus to the clinic use of OEA.
Data availability statement
The raw data supporting the conclusion of this article will be made available by the authors, without undue reservation.
Ethics statement
The animal study was reviewed and approved by the ethics committee of Central South University.
Author contributions
XY and SW conceived and carried out experiments, analyzed data and wrote the paper. All authors read and approved the final manuscript.
Funding
This work was supported by the National Natural Science Foundation of China under Grant (81901221 and 82001273); and the Natural Science Foundation of Hunan province under Grant (2021JJ41031).
Conflict of interest
The authors declare that the research was conducted in the absence of any commercial or financial relationships that could be construed as a potential conflict of interest.
Publisher’s note
All claims expressed in this article are solely those of the authors and do not necessarily represent those of their affiliated organizations, or those of the publisher, the editors and the reviewers. Any product that may be evaluated in this article, or claim that may be made by its manufacturer, is not guaranteed or endorsed by the publisher.
References
Allen, T. M., and Cullis, P. R. (2013). Liposomal drug delivery systems: From concept to clinical applications. Adv. Drug Deliv. Rev. 65, 36–48. doi:10.1016/j.addr.2012.09.037
Ansari, M., Eslami, H., Javadpour, S., and Yousefi, E. (2021). Cancer therapy using a targeted magnetoliposomes encapsulated doxorubicin assisted ultrasound. Mater. Technol. 37, 858–865. doi:10.1080/10667857.2021.1903136
Bulbake, U., Doppalapudi, S., Kommineni, N., and Khan, W. (2017). Liposomal formulations in clinical use: An updated review. Pharmaceutics 9, 12. doi:10.3390/pharmaceutics9020012
Campbell, B. C. V., De Silva, D. A., Macleod, M. R., Coutts, S. B., Schwamm, L. H., Davis, S. M., et al. (2019). Ischaemic stroke. Nat. Rev. Dis. Prim. 5, 70. doi:10.1038/s41572-019-0118-8
Cao, H., Dan, Z., He, X., Zhang, Z., Yu, H., Yin, Q., et al. (2016). Liposomes coated with isolated macrophage membrane can target lung metastasis of breast cancer. ACS Nano 10, 7738–7748. doi:10.1021/acsnano.6b03148
Centers for Disease Control and Prevention (Cdc), (2009). Prevalence and most common causes of disability among adults: United States, 2005. MMWR. Morb. Mortal. Wkly. Rep. 58, 421–426.
Centers for Disease Control and Prevention (2015). National center for health statistics underlying cause of death 1999-2013 on CDC WONDER online database, released 2015. Data are from the multiple cause of death files, 1999-2013, as compiled from data provided by the 57 vital statistics jurisdictions through the vital statistics cooperative program. Available at: http://wonder.cdc.gov/ucd-icd10.html (Accessed on Feb 3, 2015).
Chen, H., Lui, Y. S., Zhao, J., Xu, L., and Tan, L. P. (2018). Effect of solvent composition of electrospun PLGA fibers on paclitaxel release. Mater. Technol. 33, 716–722. doi:10.1080/10667857.2018.1499271
Dirnagl, U., Iadecola, C., and Moskowitz, M. A. (1999). Pathobiology of ischaemic stroke: An integrated view. Trends Neurosci. 22, 391–397. doi:10.1016/s0166-2236(99)01401-0
Garcia, J. H., Wagner, S., Liu, K. F., and Hu, X. J. (1995). Neurological deficit and extent of neuronal necrosis attributable to middle cerebral artery occlusion in rats. Stroke Vasc. Interv. Neurol. 26, 627–635. ; discussion 35. doi:10.1161/01.str.26.4.627
Kiaie, S. H., Mojarad-Jabali, S., Khaleseh, F., Allahyari, S., Taheri, E., Zakeri-Milani, P., et al. (2020). Axial pharmaceutical properties of liposome in cancer therapy: Recent advances and perspectives. Int. J. Pharm., 119269. doi:10.1016/j.ijpharm.2020.119269
Lipinski, C. A. (2000). Drug-like properties and the causes of poor solubility and poor permeability. J. Of Pharmacol. Toxicol. Methods 44, 235–249. doi:10.1016/s1056-8719(00)00107-6
Lo, E. H., Dalkara, T., and Moskowitz, M. A. (2003). Mechanisms, challenges and opportunities in stroke. Nat. Rev. Neurosci. 4, 399–414. doi:10.1038/nrn1106
Lukyanov, A. N., and Torchilin, V. P. (2004). Micelles from lipid derivatives of water-soluble polymers as delivery systems for poorly soluble drugs. Adv. Drug Deliv. Rev. 56, 1273–1289. doi:10.1016/j.addr.2003.12.004
Luo, D., Zhang, Y., Yuan, X., Pan, Y., Yang, L., Zhao, Y., et al. (2019). Oleoylethanolamide inhibits glial activation via moudulating PPAR alpha and promotes motor function recovery after brain ischemia. Pharmacol. Res. 141, 530–540. doi:10.1016/j.phrs.2019.01.027
Lv, Z., Yang, Y., Wang, J., Chen, J., Li, J., and Di, L. (2018). Optimization of the preparation conditions of borneol-modified ginkgolide liposomes by response surface methodology and study of their blood brain barrier permeability. Molecules 23, 303. doi:10.3390/molecules23020303
Mokdad, R., Seguin, C., Fournel, S., Frisch, B., Heurtault, B., and Hadjsadok, A. (2022). Anti-inflammatory effects of free and liposome-encapsulated Algerian thermal waters in RAW 264.7 macrophages. Int. J. Pharm., 121452. doi:10.1016/j.ijpharm.2022.121452
Porter, C. J. H., Pouton, C. W., Cuine, J. F., and Charman, W. N. (2008). Enhancing intestinal drug solubilisation using lipid-based delivery systems. Adv. Drug Deliv. Rev. 60, 673–691. doi:10.1016/j.addr.2007.10.014
Ruokonen, S. K., Dusa, F., Rantamaki, A. H., Robciuc, A., Holma, P., Holopainen, J. M., et al. (2017). Distribution of local anesthetics between aqueous and liposome phases. J. Chromatogr. A 1479, 194–203. doi:10.1016/j.chroma.2016.12.005
Shea, S., Misra, D., Ehrlich, M. H., Field, L., and Francis, C. K. (1992). Predisposing factors for severe, uncontrolled hypertension in an inner-city minority population. N. Engl. J. Med. Overseas. Ed. 327, 776–781. doi:10.1056/nejm199209103271107
Shen, Y. Z., Wei, Y. L., Chen, H. H., Wu, Z. Y., Ye, Y. W., and Han, D. M. (2022). Liposome-encapsulated aggregation-induced emission fluorogen assisted with portable smartphone for dynamically on-site imaging of residual tetracycline. Sensors Actuators B Chem., 130871. doi:10.1016/j.snb.2021.130871
Shimamura, N., Matchett, G., Tsubokawa, T., Ohkuma, H., and Zhang, J. (2006). Comparison of silicon-coated nylon suture to plain nylon suture in the rat middle cerebral artery occlusion model. J. Of Neurosci. Methods 156, 161–165. doi:10.1016/j.jneumeth.2006.02.017
Sozen, T., Tsuchiyama, R., Hasegawa, Y., Suzuki, H., Jadhav, V., Nishizawa, S., et al. (2009). Role of interleukin-1 beta in early brain injury after subarachnoid hemorrhage in mice. Stroke 40, 2519–2525. doi:10.1161/strokeaha.109.549592
Tran, S., DeGiovanni, P-J., Piel, B., and Rai, P. (2017). Cancer nanomedicine: A review of recent success in drug delivery. Clin. Transl. Med. 6, 44. doi:10.1186/s40169-017-0175-0
Tuo, Q-z., Zhang, S-t., and Lei, P. (2022). Mechanisms of neuronal cell death in ischemic stroke and their therapeutic implications. Med. Res. Rev. 42, 259–305. doi:10.1002/med.21817
Wang, F., Zhang, D., Zhang, Q., Chen, Y., Zheng, D., Hao, L., et al. (2011). Synergistic effect of folate-mediated targeting and verapamil-mediated P-gp inhibition with paclitaxel -polymer micelles to overcome multi-drug resistance. Biomaterials 32, 9444–9456. doi:10.1016/j.biomaterials.2011.08.041
Wicki, A., Witzigmann, D., Balasubramanian, V., and Huwyler, J. (2015). Nanomedicine in cancer therapy: Challenges, opportunities, and clinical applications. J. Of Control. Release 200, 138–157. doi:10.1016/j.jconrel.2014.12.030
Winstein, C. J., Stein, J., Arena, R., Bates, B., Cherney, L. R., Cramer, S. C., et al. (2016). Guidelines for adult stroke rehabilitation and recovery A guideline for healthcare professionals from the American heart association/American stroke association. Stroke 47, E98–E169. doi:10.1161/str.0000000000000098
Wu, S., Liao, D., Li, X., Liu, Z., Zhang, L., Mo, F. M., et al. (2021). Endogenous oleoylethanolamide crystals loaded lipid nanoparticles with enhanced hydrophobic drug loading capacity for efficient stroke therapy. Int. J. Nanomedicine 16, 8103–8115. doi:10.2147/ijn.s344318
Wu, S. C., Yang, X. B., and Yang, X. R. (2022). Methotrexate and 10-hydroxycamptothecine loaded pullulan nanoparticles with the targeting property for efficient cancer therapy. Mater. Technol. doi:10.1080/10667857.2022.2075079
Yang, L., Guo, H., Li, Y., Meng, X., Yan, L., Zhang, D., et al. (2016). Oleoylethanolamide exerts anti-inflammatory effects on LPS-induced THP-1 cells by enhancing PPAR alpha signaling and inhibiting the NF-kappa B and ERK1/2/AP-1/STAT3 pathways. Sci. Rep. 6, 34611. doi:10.1038/srep34611
Yang, L-c., Guo, H., Zhou, H., Suo, D-q., Li, W-j., Zhou, Y., et al. (2015). Chronic oleoylethanolamide treatment improves spatial cognitive deficits through enhancing hippocampal neurogenesis after transient focal cerebral ischemia. Biochem. Pharmacol. 94, 270–281. doi:10.1016/j.bcp.2015.02.012
Yang, X., Wu, S., Li, Y., Huang, Y., Lin, J., Chang, D., et al. (2015). Integration of an anti-tumor drug into nanocrystalline assemblies for sustained drug release. Chem. Sci. 6, 1650–1654. doi:10.1039/c4sc03392b
Yang, X., Xu, L., Zhou, J., Ge, Y., Wu, S., Huang, J., et al. (2019). Integration of phospholipid-complex nanocarrier assembly with endogenous N-oleoylethanolamine for efficient stroke therapy. J. Nanobiotechnology 17, 8. doi:10.1186/s12951-019-0442-x
Zhang, K., Zhang, Y., Meng, X., Lu, H., Chang, H., Dong, H., et al. (2018). Light-triggered theranostic liposomes for tumor diagnosis and combined photodynamic and hypoxia-activated prodrug therapy. Biomaterials 185, 301–309. doi:10.1016/j.biomaterials.2018.09.033
Zhigaltsev, I. V., Winters, G., Srinivasulu, M., Crawford, J., Wong, M., Amankwa, L., et al. (2010). Development of a weak-base docetaxel derivative that can be loaded into lipid nanoparticles. J. Of Control. Release 144, 332–340. doi:10.1016/j.jconrel.2010.02.029
Zhou, H., Yang, W-s., Li, Y., Ren, T., Peng, L., Guo, H., et al. (2017). Oleoylethanolamide attenuates apoptosis by inhibiting the TLR4/NF-kappa B and ERK1/2 signaling pathways in mice with acute ischemic stroke. Schmiedeb. Arch. Pharmacol. 390, 77–84. doi:10.1007/s00210-016-1309-4
Zhou, J., Ren, T., Li, Y., Cheng, A., Xie, W., Xu, L., et al. (2017). Oleoylethanolamide inhibits alpha-melanocyte stimulating hormone-stimulated melanogenesis via ERK, Akt and CREB signaling pathways in B16 melanoma cells. Oncotarget 8, 56868–56879. doi:10.18632/oncotarget.18097
Keywords: OEA, stroke, neurprotective effect, ischemia reperfusion, drug delivery
Citation: Wu S and Yang X (2022) OEA loaded liposomes with the neuroprotective effect for stroke therapy. Front. Chem. 10:1014208. doi: 10.3389/fchem.2022.1014208
Received: 08 August 2022; Accepted: 19 August 2022;
Published: 08 September 2022.
Edited by:
Weiqi Zhang, Chinese Academy of Medical Sciences and Peking Union Medical College, ChinaReviewed by:
Hao Xu, University of Science and Technology of China, ChinaZhimin Zhou, Chinese Academy of Medical Sciences and Peking Union Medical College, China
Copyright © 2022 Wu and Yang. This is an open-access article distributed under the terms of the Creative Commons Attribution License (CC BY). The use, distribution or reproduction in other forums is permitted, provided the original author(s) and the copyright owner(s) are credited and that the original publication in this journal is cited, in accordance with accepted academic practice. No use, distribution or reproduction is permitted which does not comply with these terms.
*Correspondence: Xiangrui Yang, eWFuZ3hyQGNzdS5lZHUuY24=