- Department of Pharmacy, Zhuhai Campus of Zunyi Medical University, Zhuihai, Guangdong, China
Delphinium grandiflorum L. (family Ranunculaceae), one of the most important and widely distributed Delphinium species, has received considerable interest due to its extremely high medicinal value. The discovery of novel metabolites from D. grandiflorum supported and broadened its application as an herbal medicine. In this study, the whole herb of D. grandiflorum was phytochemically investigated to obtain fourteen C19-lycaconitine-type diterpenoid alkaloids (1–14), including six undescribed alkaloids, grandiflolines A–F (1–6). The structural elucidation of them was accomplished by detailed spectroscopic analyses, mainly including HR-MS, 1D and 2D NMR (1H–1H COSY, NOESY, HMBC and HSQC), and IR spectra. New alkaloids 1–3 and 5 possess a characteristic △2,3 functional group in the A ring, while compounds 5 and 6 feature a rare OH-16 substituent. In addition, known compounds 7–12 were isolated from D. grandiflorum for the first time. Moreover, according to its medicinal use, new alkaloids 1–6 were estimated for their potential in vitro anti-inflammatory effects, and some of them exhibited inhibitory effects on NO production in LPS-activated RAW 264.7 macrophages. Our work enriched the chemical diversity of D. grandiflorum and the genus Delphinium and presented beneficial information for further investigations.
Introduction
The genus Delphinium L., which belongs to the tribe Delphineae in the family Ranunculaceae, is an important species-rich genus comprising approximately 400 species of annual, biennial, or perennial herbs. Delphinium plants prefer cold and humid conditions and are mainly distributed in mountainous regions in the north temperate zone, including Asia, Europe, North America, and sporadically North Africa (Wang, 2019). China is regarded as the distribution center for this genus, as more than half of the confirmed Delphinium species were reported to be grown within this country (232 species, 200 endemic), mainly in the high mountain areas in northern Yunnan, eastern Tibet and western Sichuan (Chen et al., 2009; Wang, 2019). Delphinium plants are well-known ornamental plants with a long history around the world (Yin et al., 2020). Many Delphinium species, represented by D. grandiflorum, D. elatum, Delphinium × belladonna, feature showy flowers with various colors, including white, pink, blue, light blue, violet, purple, and lavender, which are particularly popular worldwide and have been widely cultivated as landscape and potted plants or cut flowers (Ichimura et al., 2009). On the other hand, in many countries and regions, such as China and India, Delphinium plants are commonly used as herbal medicines by natives for treating various diseases, mainly traumatic injury, enteritis, rheumatism, headache, toothache, neuralgia, and other kinds of pain. The multiple therapeutic effects of Delphinium-derived herbs could be attributed to the abundance of various active ingredients, including diterpenoid alkaloids (DAs), flavonoids, phenolic acids, and volatile oils (Marin, 2011). In particular, DAs, which have been acknowledged as the characteristic ingredients for this genus, have exhibited broad-spectrum biological activities, including analgesic, anti-inflammatory, antiarrhythmic, anticancer, antioxidant, and neuroprotective activities (Thawabteh et al., 2021; Liu et al., 2022). Further exploration and discovery of bioactive DAs with novel structures from Delphinium plants could support and broaden their application as medicinal plants.
D. grandiflorum L., one of the best-known Delphinium species, is widely distributed in China and other Asian countries, including Mongolia, Korea, and the Russian Far East (Siberia) (Liu et al., 2009; Chen et al., 2017; Xu et al., 2021). This plant has a particularly long history as an ornamental flower and has been cultivated as a horticultural plant in Beijing City of China for hundreds of years. In addition, its roots or whole herbs are used for treating traumatic injury, toothache, and asthma in traditional Chinese medicine (Li et al., 2019). In previous investigations, a number of DAs, mainly C19-lycoctonine-type DAs, have been reported in D. grandiflorum (Batbayar et al., 2003; Wang et al., 2021), and some of them possess unprecedented DA skeletons. For example, Chen et al. reported two novel DAs, grandiflodine A and B, from D. grandiflorum. The former compound showed a C19-lycaconitine-type DA skeleton with cleavage of N-C19 and C7-C17 bonds and linkage of the N-C7 bond, while the latter represents a rare hetisine-type C20-DA with a broken N–C7 bond (Chen et al., 2017). In addition, two DAs, grandiflonines A and B reported by (Xu et al., 2021), possess an undescribed C20-hetisine-type DA skeleton with an open E ring. These exciting discoveries highlight the significant chemical diversity of DAs in D. grandiflorum and promote further in-depth studies on them. Hence, as part of our ongoing research exploring bioactive DAs with novel structures from Delphinium plants (Yin et al., 2022), the whole herb of D. grandiflorum was phytochemically investigated to afford fourteen C19-lycaconitine-type DAs (1–14), including six undescribed DAs, grandiflolines A–F (1–6) (Figure 1). The structural elucidation was accomplished by detailed spectroscopic analyses, mainly including 1D and 2D NMR, HR-MS, and IR spectra. Moreover, new alkaloids 1–6 were estimated for their potential anti-inflammatory effects in LPS-activated RAW 264.7 macrophages. This paper describes the extraction and isolation, structural elucidation, and activity screening of these compounds.
Materials and methods
General experimental procedures
1D (1H, 13C, and DEPT) and 2D (HSQC, HMBC, 1H–1H COSY, and NOESY) NMR spectra were obtained on a Bruker AM-500 spectrometer (Bruker, Germany) in CDCl3 or CD3OD (Qingdao Tenglong, China), and TMS was used as an internal reference. IR spectra were scanned on a Nicolet Magna-IR 550 spectrometer (Thermo Nicolet, United States) with KBr pellets. Optical rotations were measured on a Jasco P-1020 digital polarimeter (Jasco, Japan). HR-ESI-MS spectra were obtained on an Agilent 6230 LC/TOF MS spectrometer (Agilent, United States). The prep-HPLC experiment was performed on an Agilent 1260 pump coupled with an analytical preparative ZORBAXSB column (21.2 × 500 m, 5 µm). Silica gel (300–400 mesh, Qingdao Haiyang, China) was used in column chromatography, and Dragendorff’s reagent was used in TLC analysis (GF254 TLC plates, Qingdao Haiyang, China).
Plant material
Whole herbs of D. grandiflorum were gathered in Longhua County of China in December 2020. The voucher specimen (2020-dg-1) identified by Zhang Jun from Kunming GenPHYTech Co., Ltd. is stored at Zunyi Medical University, China.
Extraction and isolation
The whole herbs of D. grandiflorum (20 kg) were air-dried, crushed, and then extracted with 95% ethanol three for 3 days at room temperature (three times). The extracted solutions were combined and evaporated under reduced pressure to obtain ethanol extracts (∼2 kg), which were completely dissolved in water (2 L) at 70°C, adjusted to pH 1 with HCl and extracted with EtOAc (3 L × 3). After adjusting the acidic aqueous solution to pH 10 with sodium hydroxide, it was extracted with CHCl3 to obtain the crude alkaloid (320 g).
The crude alkaloid was divided into five fractions (Fr.A–Fr.E) by silica gel CC eluted with a CHCl3-CH3OH gradient system (100:1 → 0:1). Fr.A (5.4 g) was separated by silica gel CC (CHCl3-CH3OH-NH4OH, 100:1:1) to yield two subfractions (Fr.A1 and Fr.A2). The former subfraction was purified on silica gel CC (CHCl3-CH3OH, 1:1) to afford compound 7 (3 mg), while the latter was purified on silica gel CC (CHCl3-CH3OH- NH4OH, 100:1:1) to afford compound 2 (168 mg). Fr.B (20.7 g) was further purified by semipreparative HPLC over a ZORBAXSB C18 column eluted with petroleum ether-acetone-diethylamine (4:1:1) to obtain compound 1 (7 mg). Further silica gel CC purification of Fr.C (25.3 g) was carried out by elution with CH3OH-H2O (30:70 → 80:20) to yield four subfractions (Fr.C1–Fr.C4). Subfraction Fr.C1 was separated on silica gel CC (CHCl3-CH3OH-NH3H2O, 30:1:1) to obtain compounds 5 (5 mg) and 10 (98 mg), while subfraction Fr.C2 was purified by semipreparative HPLC (CH3OH-H2O, 65:35, 0.5% NH4OH) to yield compound 3 (4 mg). Fr.C3 was subjected to silica gel CC (CHCl3-CH3OH-NH4OH, 100:1:1) to afford two subfractions (SFr.C3-1 and SFr.C3-2). Fr.C3-1 was further separated by silica gel CC (PE-acetone-NH3H2O, 2:1:1) to afford compounds 8 (6 mg) and 9 (15 mg), and Fr.C3-2 was purified by semipreparative HPLC (CH3OH-H2O, 70:30, 0.5% NH4OH) to afford compounds 6 (7 mg) and 11 (12 mg). Fr.C4 was separated on a silica gel CC (PE-acetone-diethylamine, 4:1:1) to give two subfractions (Fr.C4-1 and Fr.C4-2), which was purified by semipreparative HPLC (CH3OH-H2O, 70:30, 0.5% NH4OH) to yield compounds 4 (13 mg) and 13 (2 mg). Fr.D (12.9 g) was purified by silica gel CC (PE-acetone-NH3H2O, 3:1:1) and semipreparative HPLC (CH3OH-H2O, 70:30, 0.5% NH4OH) to afford compound 12 (28 mg). Fr.E (14.1 g) was separated by silica gel CC (CHCl3-CH3OH-NH4OH, 100:1:1 → 220:1:1) along with semipreparative HPLC (CH3OH-H2O, 70:30, 0.5% NH4OH) to afford compound 14 (43 mg).
Grandifloline A (1): white powder; IR (KBr, cm−1): 3520, 2917, 2751, 1745, 1675, 1278, 1057, 805;
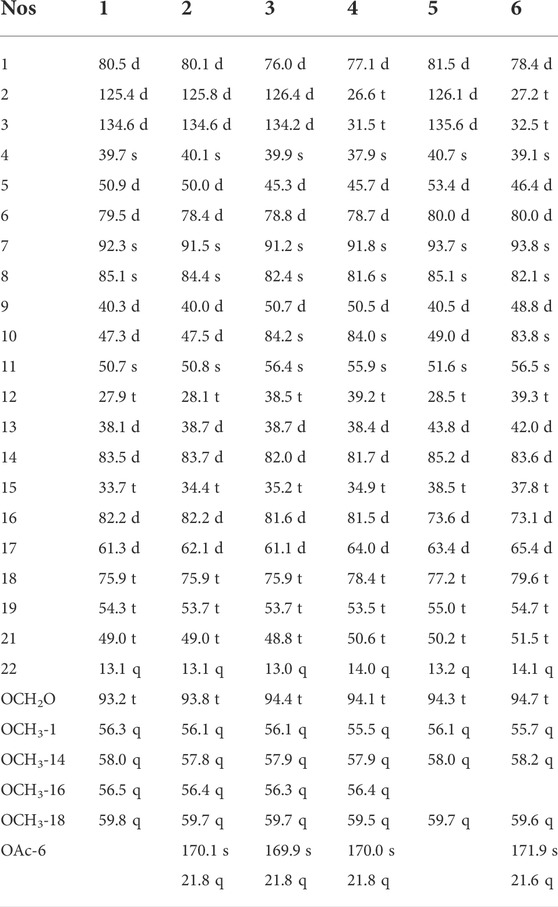
TABLE 1. 13C NMR (125 MHz) data of compounds 1–6 (compounds 1–4 in CDCl3 and compounds 5 and 6 in CD3OD).
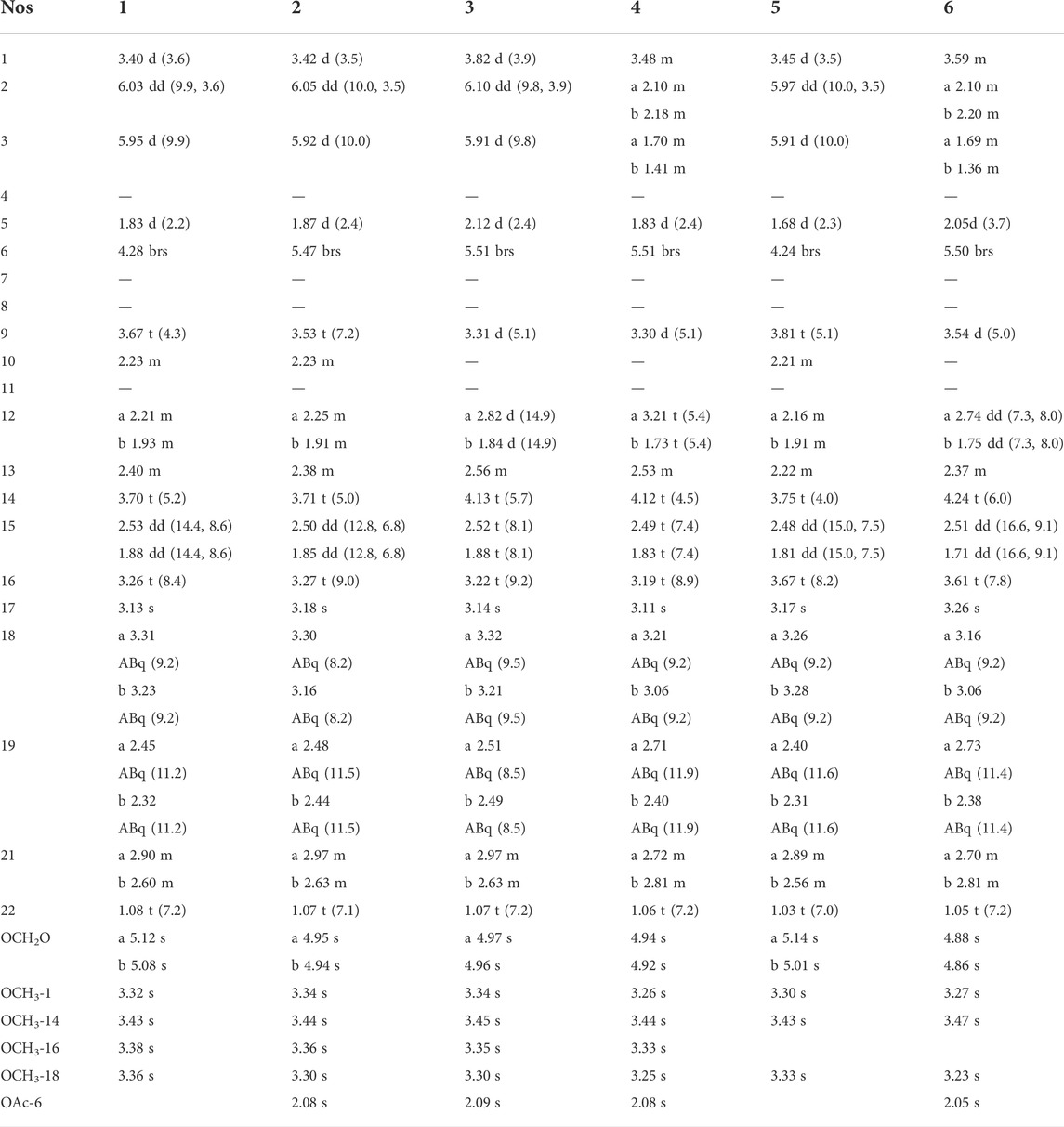
TABLE 2. 1H NMR (500 MHz, δH, Mult., J in Hz) data of compounds 1–6 (compounds 1–4 in CDCl3 and compounds 5 and 6 in CD3OD).
Grandifloline B (2): white powder; IR (KBr, cm−1): 2936, 2881, 1741, 1649, 1452, 1366, 1224, 853;
Grandifloline C (3): white powder; IR (KBr, cm−1): 3450, 2933, 2888, 1739, 1674, 1229, 1088, 819;
Grandifloline D (4): white powder; IR (KBr, cm−1): 3515, 2934, 2874, 1739, 1650, 1366, 1090, 852;
Grandifloline E (5): white powder; IR (KBr, cm−1): 3437, 2964, 2824, 1740, 1647, 1397, 1078, 813;
Grandifloline F (6): white amorphous powder; IR (KBr, cm−1): 3467, 2932, 2875, 1741, 1631, 1245, 1054, 852;
NO production in RAW264.7 macrophages
A previously reported method was adopted to evaluate the in vitro anti-inflammatory activities of the newly isolated alkaloids (Wang et al., 2019). RAW264.7 cells were plated into 96-well microplates, stimulated with 1 μg/ml LPS, and treated with the alkaloid under test. The nondrug group and L-NMMA-treated group were set as blank and positive controls, respectively. After the macrophages were cultivated overnight, the NO content in the medium and the absorbance of the solution were measured at 570 nm. To exclude the toxic effects of the compound on the cells, MTS was added to the remaining medium to detect cell survival. The inhibition rate on NO generation was calculated by the following equation: inhibition rate (%) = (ODnondrug group–ODsample group)/ODnondrug group × 100%).
Results and discussion
Structural identification of new compounds
Compound 1, a white powder, exhibited a pseudo ion peak at m/z 478.2799 [M+H]+ in its HR-MS spectrum, which corresponded to the molecular formula C26H39NO7, with an unsaturation degree of eight. Its 1H NMR spectrum revealed the existence of an NCH2CH3 group (δH 1.08, t, J = 7.2 Hz, 3H), a characteristic cis-trisubstituted double bond (δH 6.03, dd, J = 9.9 Hz, 3.6 Hz; 5.95, d, J = 9.9 Hz; each 1H), four methoxyl (OCH3) groups (δH 3.32, 3.36, 3.38, 3.43, s, each 3H), and a methylenedioxy (OCH2O) group (δH 5.08, 5.12, s, each 1H). The 13C NMR spectrum suggested that compound 1 possesses 19 carbons in addition to the NCH2CH3, methoxy, and methylenedioxy groups, including four diagnostic quaternary carbons at δH 39.7 s, 50.7 s, 85.1 s, and 92.3 s (Yin et al., 2022). Combining the above data with biogenetic considerations implied that compound 1 could be a C19-lycoctonine-type DA (Meng et al., 2017). Seven oxygenated carbons were observed in the 13C NMR spectrum (δC 75.9 t, 79.5 d, 80.5 d, 82.2 d, 83.5 d, 85.1 s, 92.3 s), in addition to the molecular formula, suggesting an extra OH group in addition to the OCH3 and OCH2O groups. According to the HMBC correlations from OCH3-1 (δH 3.32, s) to C-1 (δC 80.5 d), OCH3-14 (δH 3.43 s) to C-14 (δC 83.5 d), OCH3-16 (δH 3.38 s) to C-16 (δC 82.2 d), and OCH3-18 (δH 3.36 s) to C-18 (δC 75.9 t), four OCH3 groups were located at C-1, C-14, C-16, and C-18 (Figure 2). The OCH2O group was placed at C-7 and C-8 as revealed by the long-range correlations from the methylene protons to C-7 and C-8. In addition, on the basis of the HMBC correlation networks from H-6 (δH 4.28, brs) to C-5 (δC 50.9 d), C-7 (δC 92.3 s), and C-11 (δC 50.7 s), the hydroxyl group was determined to be connected to C-6 (δC 79.5). The double bound was located at C-2 and C-3 based on the 1H–1H COSY correlation between H-1 and H-2, which was further confirmed by the HMBC correlations from H-2 to C-1 and C-11 and from H-3 to C-4, C-5, and C-18. Finally, the relative configuration of 1 was deduced from the NOESY experiment (Figure 3). The α-orientation of OCH3-1 was confirmed by the NOESY correlation between H-1β and H-10β, and between H-1β and H-12β. The β-orientation of OH-6 was deduced from the NOESY correlation between H-1β and H-10β, and between H-1β and H-12β. The orientations of the remaining oxygenated substituents are identical for all lycoctonine-type DAs, namely, the α-orientation of OCH3-14 and the β-orientation of OCH3-16, OCH3-18, and OCH2O (Ablajan et al., 2018). Hence, the structure of 1 was established with the assigned NMR data in Tables 1, 2.
Compound 2 was afforded as a white powder, and its molecular formula was determined to be C28H41NO8 by HR-MS at m/z 520.2909 [M+H]+. A characteristic absorption spectrum for ester carbonyl groups (1741 cm−1) was observed in the IR spectrum. The 1H NMR spectrum revealed the presence of an NCH2CH3 group (δH 1.03 t, J = 7.0 Hz, 3H), four OCH3 groups (δH 3.30, 3.34, 3.36, 3.44, s, each 3 H), a cis-trisubstituted double bond (δH 5.92, d, J = 10.0 Hz; 6.05, dd, J = 10.0 Hz, 3.4 Hz; each 1H), an OCH2O group (δH 4.94, 4.95, s, each 1H) and an OAc functional group (δH 2.08, s, 3H). The above NMR features indicated a C19-lycoctonine-type DA for 2 (Lin et al., 2017). Comparison of NMR data between compounds 2 and 1 (Tables 1, 2) revealed identical substituent patterns. Exceptionally, alkaloid 2 possesses an extra OAc group, which was placed at C-6 according to the HMBC correlation from H-6 to the ester carbonyl carbon (Figure 2). This could be further supported by the fact that H-6 in 2 was significantly shifted downfield from δH 4.28 in compound 1 to δH 5.47 due to the substituted effect (OH→OAc). Therefore, the structure of 2 was determined, and its relative configuration was consistent with that of 1, as revealed by the NOESY experiment (Figure 3). All of the NMR data of 2 were assigned by 2D NMR and are listed in Tables 1, 2.
Compound 3 is a white powder, whose molecular formula was identified as C28H41NO9 by HR-MS at m/z 536.2855 [M+H]+. The IR spectrum indicated the existence of hydroxyl (3450 cm−1) and ester (1739 cm−1) groups. Compound 3 displayed characteristic NMR features for a C19-lycoctonine-type DA bearing an NCH2CH3 group (δH 1.07, t, J = 7.2 Hz, 3H), an OCH2O group (δH 4.96, 4.97, s, each 1H), four OCH3 groups (δH 3.30, 3.34, 3.35, 3.45, s, 3H), a cis-trisubstituted double bond (δH 6.10, dd, J = 9.8 Hz, 3.9 Hz; 5.91, d, J = 9.8 Hz; s, each 1H), and an OAc group (δH 2.09 s, 3H) (Ping et al., 2007). The NMR data of 3 were highly similar to those of 2 except that the chemical shift of C-10 in compound 3 was shielded downfield with approximately ΔδC 36. In addition, eight oxygenated carbons (δC 75.9 t, 76.0 d, 78.8 d, 81.6 d, 82.0 d, 82.4 s, 84.2 s, 91.2 s) were detected in the 13C NMR spectrum, in combination with the molecular formula, indicating the presence of an extra hydroxyl group in addition to the abovementioned substituents. The hydroxyl group should be positioned at C-10 due to the chemical shift of C-10, which could be further supported by the HMBC correlation networks from H-9, H-12, and H-17 to C-10 (Figure 2). The relative configuration of 3 was also deduced from the NOESY experiment (Figure 3), which was identical to those of compounds 1 and 2 (Meng et al., 2017). Thus, the structure of compound 3 was determined.
Compound 4 was afforded as a white powder. According to the protonated molecular ion at m/z 538.3012 [M+H]+ in the HR-MS spectrum, its molecular formula was identified as C28H43NO9. Analysis of its IR spectrum revealed hydroxyl (3515 cm−1) and ester (1739 cm−1) groups in the structure. In the 1H NMR spectrum, an NCH2CH3 group (δH 1.06, t, J = 7.4 Hz, 3 H), an OCH2O group (δH 4.92, 4.94, s, each 1H), four OCH3 groups (δH 3.25, 3.26, 3.33, 3.44, each 3H), and an OAc group (δH 2.08 s, 3H) were recognized. Apart from the above groups, compound 4 contains 19 carbons, including five diagnostic quaternary carbons (δC 37.9, 55.9, 81.6, 84.0, 91.8, s). These data, in combination with biogenetic consideration, suggested a C19-lycoctonine-type DA for 4 (Li et al., 2010). Moreover, a combined analysis of 1H and 13C NMR data suggested that 4 and 3 have similar structures. Correlations between OCH3-1 (δH 3.26, s) and C-1 (δC 77.1 d), OCH3-14 (δH 3.44, s) and C-14 (δC 81.7 d), OCH3-16 (δH 3.33, s) and C-16 (δC 81.5 d), OCH3-18 (δH 3.25, s) and C-18 (δC 78.4 d), and H-6 (δH 5.51, brs) with C=O (δC 170.0 s) in the HMBC spectrum confirmed the assignment of four OCH3 groups and the ester group. A hydroxyl group was placed at C-10 on the basis of the HMBC correlations from H-9 (δH 3.30, d), H-12 and H-13 (δH 2.53, m) to C-10 (δC 83.9) (Figure 2). Based on the long-range correlations of the OCH2O group with C-7 and C-8, this substituent group was located at C-7 and C-8. Furthermore, in a comparison between 4 and 3 in terms of their molecular formulas and NMR data, one of the most important differences was found to be the lack of the △2,3 in 4. Therefore, its structure was determined and further confirmed by analysing its 2D NMR spectra (Figure 2). Similarly, the orientations for several oxygenated substituents at flexible positions, including OCH3-1α and OAc-6β, were determined by using the NOESY experiment (Figure 3) (Meng et al., 2017). Thus, the structural elucidation of compound 4 was accomplished.
Compound 5 is a white powder, and its molecular formula was determined to be C25H37NO7 by HR-MS at m/z 464.2643 [M+H]+. The 1H NMR spectrum showed an NCH2CH3 group (δH 1.03, t, J = 7.0 Hz, 3H), an OCH2O group (δH 5.01, 5.14, s, each 1H), three OCH3 groups (δH 3.30, 3.33, 3.43, s, each 3H), and a cis-trisubstituted double bond (δH 5.97, dd, J = 10.0 Hz, 3.8 Hz; 5.91, d, J = 10.0 Hz; each 1H). There are nineteen carbons in the 13C NMR spectrum of 5 other than the abovementioned groups, including four characteristic quaternary carbons (δc 40.7, 51.6, 85.2, 93.7). Combining the data presented above with biogenetic considerations suggested that 5 might be a C19-lycoctonine-type DA (Zhang et al., 2016). In addition, seven oxygenated carbons (δC 73.6 d, 77.2 t, 80.0 d, 81.5 d, 85.1 s, 85.2 d, 93.7 s) were found in the 13C NMR spectrum, which corresponded to the molecular formula, implied two extra OH groups in 5. On the basis of the HMBC correlation networks from H-6 (δH 4.24, brs) to C-5 (δC 53.4 d), C-7 (δC 93.7 s), and C-11 (δC 51.6 s) and from H-16 (δH 3.67, t) to C-13 (δC 43.8 d) and C-15 (δC 38.5 t), these two hydroxyl groups were determined to connect to C-6 (δC 80.0) and C-16 (δC 73.6), respectively. The double bond was located at C-2 and C-3 based on the 1H–1H COSY correlation between H-1 and H-2, which was further supported by the HMBC correlations from H-2 to C-1 and C-11 and from H-3 to C-4, C-5, and C-18. Three OCH3 groups were placed at C-1, C-14, and C-18 on the basis of the HMBC correlations from OCH3-1 (δH 3.30, s) to C-1 (δc 81.5 d), OCH3-14 (δH 3.43 s) to C-14 (δc 85.2 d), and OCH3-18 (δH 3.36 s) to C-18 (δc 59.7 t), respectively. In addition, OCH2O was located at C-7 and C-8 due to the long-range correlations of the methylene group with C-7 (δc 91.3 q) and C-8 (δc 84.2 q) (Figure 2). The relative configurations of OCH3-1α and OH-6β were determined on the basis of the NOESY correlations between H-6 and H-18 and between H-1 and H-10, respectively (Liu et al., 2009). Thus, the structure of compound 5 was determined.
Compound 6 has the molecular formula C27H41NO9 as determined by the HR-MS experiment (m/z 524.2854 [M+H]+), suggesting eight degrees of unsaturation. Its IR spectrum showed the presence of hydroxyl (3467 cm−1) and ester (1741 cm−1) groups. The 1H NMR spectrum revealed the presence of an NCH2CH3 group (δH 1.05, t, J = 7.2 Hz, 3H), an OCH2O group (δH 4.86, 4.88, s, each 1H), an OAc group (δH 2.05, s, 3H; δC 21.6 q, 171.9 s), and three OCH3 groups (δH 3.23, 3.27, 3.47, s, each 3H). The 13C NMR spectrum showed 19 carbons in addition to the aforementioned groups, including four diagnostic quaternary carbons at δC 39.1, 56.5, 82.1, 93.8, thus revealing a C19-lycoctonine-type DA for 6 (Ablajan et al., 2018). Comparing all of the NMR data of 6 and 5, it was found that the major difference was the lack of the △2,3 group for 6 and an additional ester group and oxygenated carbon (δC 83.8 s), which can be attributed to an OAc group and an OH group. Eight oxygenated carbons (δC 73.1 d, 78.4 d, 79.6 d, 80.0 d, 82.1 s, 83.6 d, 83.8 d, 93.8 s) presented in the 13C NMR spectrum also implied the presence of two OH groups along with three OCH3 groups, an OAc group, and an OCH2O group in 6 (Xu et al., 2021). The extra OH group could be positioned at C-10 due to the HMBC correlations from H-9 (δH 3.54, d) and H-12 (δH 1.75, d) to C-10 (δC 83.8 s) (Figure 2). Additionally, the HMBC correlation from H-6 (δH 5.50, s) to the carbonyl carbon suggested that the OAc group is connected at C-6. Thus, the structure of compound 6 was determined.
The remaining compounds were identified by comparison of the 1H and 13C NMR data with those in the literature. Finally, they were identified as eight known C19-lycaconitine-type DAs, namely, tatsiensine (7) (Wang et al., 2003), deacetyltatsiensine (8) (Pelletier et al., 1983), siwanines A and B (9 and 10) (Zhang and Ou, 1998), elasine (11) (Pelletier et al., 1989), anthriscifoldine B (12) (Song et al., 2009), browniine (13) (Zhou et al., 2005), and lycoctonine (14) (Zeng et al., 2007). Among them, compounds 7–12 have not been found in D. grandiflorum in previous studies.
Biological activity
Traditionally, Delphinium plants have been extensively utilized to treat arthritis and other inflammatory diseases, which implies that their major constituents, namely, diterpenoid alkaloids, possess certain anti-inflammatory activity. It has been reported in previous studies that a certain number of diterpenoid alkaloids, mainly aconitine-type and lycoctonine-type alkaloids, have exhibited in vitro anti-inflammatory activity (Shen et al., 2020; Qasem et al., 2022). For example, two typical lycoctonine-type DAs, delbrunine and eldeline, from D. brunonianum Royle showed good anti-inflammatory activity in LPS-activated RAW 264.7 cells, which could significantly restrain the elevation of inflammatory factors, including NO, TNF-α (tumor necrosis factor-α), IL-6 (interleukin-6), COX-2 (cyclooxygenase 2), and iNOS (inducible nitric oxide synthase) through NF-κB signaling pathway (Wang et al., 2010). Another analogous Delphinium alkaloid A from D. giraldii Diels suppressed the overexpression of the proinflammatory factors TNF-α, IL-6 and IL-8 significantly in LPS-infected Caco2 cells (Liu et al., 2019). Our previous studies also indicated that three aconitine-type DAs, taronenines A, B and D, showed inhibitory effects on the production of IL-6 in LPS-activated RAW 264.7 cells, which exerted IC50 values of 29.6, 18.8, and 25.4 μg/ml, respectively (Yin et al., 2018). Accordingly, in the present study, all of the new compounds were screened for their inhibitory activities against NO in LPS-activated RAW 264.7 cells. As a result, all of the isolated new alkaloids exhibited a weak inhibitory effect, exerting an inhibition rate of approximately 20% at a concentration of 50 µM (Figure 4). This might be attributed to the lack of key pharmacophores responsible for their anti-inflammatory activity, such as aromatic ester groups at C-14 or C-8 (Tang et al., 2022).
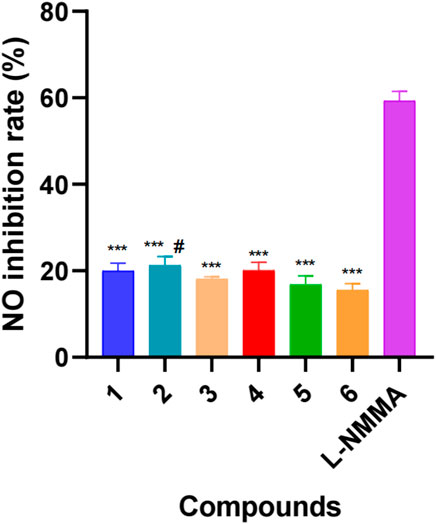
FIGURE 4. Inhibitory effects of six new new diterpenoid alkaloids on NO production in LPS-induced RAW264.7 cells. L-NMMA: L-NMMA was used as positive control. Error bars indicate SD, #, p < 0.05 vs. compound 6, ***, p < 0.001 vs. L-NMMA.
Conclusion
DAs have attracted increasing interest due to their complex and diverse structures and bioactivities. In the present study, six previously undescribed C19-lycoctonine-type DAs were isolated and identified from the whole plant of D. grandiflorum. New alkaloids 1–3 and 5 possess a characteristic △2,3 functional group in the A ring, while compounds 5 and 6 feature a rare OH-16 substituent. In addition, known compounds 7–12 were isolated from D. grandiflorum for the first time. The results of our work enriched the chemical diversity of D. grandiflorum and the genus Delphinium and presented beneficial information for further investigations. Compounds 1–6 only exhibit weak inhibition activities of NO in LPS-activated RAW 264.7 macrophages. The results suggested that the anti-inflammatory effect of D. grandiflorum might be attributed to the other DA compounds. Thus, further studies are still needed to elucidate the material basis of D. grandiflorum in inflammation-mediated diseases.
Data availability statement
The original contributions presented in the study are included in the article/Supplementary Material, further inquiries can be directed to the corresponding author.
Author contributions
TY conceived and designed the experiments and revised the manuscript. YY isolated the compounds and wrote the original draft. TY carried out structure elucidation. YY, HJ, and XY carried out the experiments and data analyses. ZD collected the plant material. All authors have read and approved the published version of the manuscript.
Funding
This work was financially supported by a grant from the National Natural Science Foundation of China (No. 31860095) and a grant from Chinese Medicine, Ethnic Medicine Science and Technology Research Project of Guizhou Provincial Administration of Traditional Chinese Medicine (No. QZYY-2022-020).
Conflict of interest
The authors declare that the research was conducted in the absence of any commercial or financial relationships that could be construed as a potential conflict of interest.
Publisher’s note
All claims expressed in this article are solely those of the authors and do not necessarily represent those of their affiliated organizations, or those of the publisher, the editors and the reviewers. Any product that may be evaluated in this article, or claim that may be made by its manufacturer, is not guaranteed or endorsed by the publisher.
Supplementary material
The Supplementary Material for this article can be found online at: https://www.frontiersin.org/articles/10.3389/fchem.2022.1012874/full#supplementary-material
References
Ablajan, N., Zhao, B., Xue, W.-J., Ruzi, Z., Zhao, J.-Y., and Aisa, H. A. (2018). Diterpenoid alkaloids from Delphinium aemulans. Nat. Prod. Commun. 13 (11), 1934578X1801301–1431. doi:10.1177/1934578x1801301104 |
Chen, F.-Z., Chen, D.-L., Chen, Q.-H., and Wang, F.-P. (2009). Diterpenoid alkaloids from Delphinium majus. J. Nat. Prod. 72 (1), 18–23. doi:10.1021/np800439a | |
Chen, N.-H., Zhang, Y.-B., Li, W., Li, P., Chen, L.-F., Li, Y.-L., et al. (2017). Grandiflodines A and B, two novel diterpenoid alkaloids from Delphinium grandiflorum. RSC Adv. 7 (39), 24129–24132. doi:10.1039/c7ra02869e |
Ichimura, K., Shimizu, Y., H., and Goto, R. (2009). Ethylene production by gynoecium and receptacle is associated with sepal abscission in cut Delphinium flowers. Postharvest Biol. Technol. 52 (3), 267–272. doi:10.1016/j.postharvbio.2008.12.008 |
Li, C., Hirasawa, Y., Arai, H., Aisa, H. A., and Morita, H. (2010). A new diterpenoid alkaloid, sharwuphinine A, from Delphinium sharwurense. Heterocycles 80 (1), 607–612. doi:10.3987/com-09-s(s)20 |
Li, G.-Q., Li, Y.-Y., Zhao, Y.-T., Xu, Q.-P., Li, Y.-L., and Wang, G.-C. (2019). Isolation and crystal structure of 4-((2-(methoxycarbonyl)phenyl)amino)-2-methyl-4-oxobutanoic acid from Delphinium Grandiflorum, C13H15NO5. Z. Krist. New Cryst. Struct. 234 (3), 521–522. doi:10.1515/ncrs-2018-0517 |
Lin, C.-Z., Liu, Z.-J., Zeren-Dawa, B., and Zhu, C.-C. (2017). A new diterpenoid alkaloid isolated from Delphinium caeruleum. Chin. J. Nat. Med. 15 (1), 45–48. doi:10.1016/s1875-5364(17)30007-9 | |
Liu, X.-Y., Chen, Q.-H., and Wang, F.-P. (2009). New C20‐diterpenoid alkaloids from Delphinium anthriscifolium var. savatieri. Helv. Chim. Acta 92 (4), 745–752. doi:10.1002/hlca.200800376 |
Liu, X.-Y., Ke, B.-W., Qin, Y., and Wang, F.-P. (2022). The diterpenoid alkaloids. Alkaloids. Chem. Biol. 87, 1–360. doi:10.1016/bs.alkal.2021.08.001 | |
Marin, C., Ramirez-Macias, I., Lopez-Cespedes, A., Olmo, F., Villegas, N., Diaz, J. G., et al. (2011). In vitro and in vivo trypanocidal activity of flavonoids from Delphinium staphisagria against Chagas disease. J. Nat. Prod. (Gorakhpur). 74 (4), 744–750. doi:10.1021/np1008043 | |
Meng, X.-H., Guo, Q.-L., Zhu, C.-G., and Shi, J.-G. (2017). Unprecedented C19-diterpenoid alkaloid glycosides from an aqueous extract of "fu zi": Neoline 14-O-L-arabinosides with four isomeric L-anabinosyls. Chin. Chem. Lett. 28 (8), 1705–1710. doi:10.1016/j.cclet.2017.04.026 |
Pelletier, S. W., Glinski, J. A., Joshi, B. S., and Chen, S.-Y. (1983). The diterpenoid alkaloids of Delphinium tatsienense Franch. Heterocycles 20 (7), 1347–1354. doi:10.3987/R-1983-07-1347 |
Pelletier, S. W., Ross, S. A., and Kulanthaivel, P. (1989). New alkaloids from Delphinium elatum L. Tetrahedron 45 (7), 1887–1892. doi:10.1016/S0040-4020(01)80053-3 |
Ping, T., Chen, D.-L., Chen, Q., -H., Jiang, X.-X., and Wang, F.-P. (2007). Three new C19-diterpenoid alkaloids from Delphinium laxicymosum var. pilostachyum. Chin. Chem. Lett. 18 (6), 700–703. doi:10.1016/j.cclet.2007.04.026 |
Qasem, A M. A., Zeng, Z., Rowan, M. G., and Blagbrough, I. S. (2022). Norditerpenoid alkaloids from aconitum and Delphinium: Structural relevance in medicine, toxicology, and metabolism. Nat. Prod. Rep. 39 (3), 460–473. doi:10.1039/D1NP00029B | |
Shen, Y., Liang, W. J., Shi, Y. N., Kennelly, E. J., and Zhao, D. K. (2020). Structural diversity, bioactivities, and biosynthesis of natural diterpenoid alkaloids. Nat. Prod. Rep. 37 (6), 763–796. doi:10.1039/d0np00002g | |
Song, L., Liu, X.-Y., Chen, Q.-H., and Wang, F.-P. (2009). New C19- and C18-diterpenoid alkaloids from Delphinium anthriscifolium var. Chem. Pharm. Bull. 57 (2), 158–161. doi:10.1248/cpb.57.158 | |
Tang, Q., Chen, S., Rizvi, S., Qu, J., Kang, W., Wang, S., et al. (2022). Two alkaloids from Delphinium brunonianum Royle, their anti-inflammatory and anti-oxidative stress activity via NF-κB signaling pathway. Front. Nutr. 8, 826957. doi:10.3389/fnut.2021.826957 | |
Thawabteh, A. M., Thawabteh, A., Lelario, F., Bufo, S. A., and Scrano, L. (2021). Classification, toxicity and bioactivity of natural diterpenoid alkaloids. Molecules 26 (13), 4103. doi:10.3390/molecules26134103 | |
Wang, F.-P., Chen, Q.-H., and Liu, X.-Y. (2010). Diterpenoid alkaloids. Nat. Prod. Rep. 27 (4), 529–570. doi:10.1039/B916679C | |
Wang, J.-L., Zhou, X.-L., and Wang, F.-P. (2003). Chemical studies on the diterpenoid alkaloids of Delphinium giraldii Diels. Nat. Prod. Res. Dev. 15 (6), 498509–499501.
Wang, J.-P., Shu, Y., Liu, S.-X., Hu, J.-T., Sun, C.-T., Zhou, H., et al. (2019). Expanstines A-D: Four unusual isoprenoid epoxycyclohexenones generated by Penicillium expansum YJ-15 fermentation and photopromotion. Org. Chem. Front. 6 (23), 3839–3846. doi:10.1039/c9qo01076a |
Wang, W. T. (2019). A revision of the genus Delphinium (Ranunculaceae) of China (I). Guihaia 39 (11), 1425–1469.
Xu, J.-B., Li, Y.-Z., Huang, S., Chen, L., Luo, Y.-Y., Gao, F., et al. (2021). Diterpenoid alkaloids from the whole herb of Delphinium grandiflorum. L. Phytochem. 190, 112866. doi:10.1016/j.phytochem.2021.112866 | |
Yin, T.-P., Hu, X.-F., Mei, R.-F., Shu, Y., Gan, D., Cai, L., et al. (2018). Four new diterpenoid alkaloids with anti-inflammatory activities from Aconitum taronense Fletcher et Lauener. Phytochem. Lett. 25, 152–155. doi:10.1016/j.phytol.2018.04.001 |
Yin, T.-P., Cai, L., and Ding, Z.-T. (2020). An overview of the chemical constituents from the genus Delphinium reported in the last four decades. RSC Adv. 10 (23), 13669–13686. doi:10.1039/D0RA00813C | |
Yin, T.-P., Yu, Y., Liu, Q.-H., Zhou, M.-Y., Zhu, G.-Y., Bai, L.-P., et al. (2022). 2D NMR-based MatchNat dereplication strategy enables explosive discovery of novel diterpenoid alkaloids. Chin. J. Chem. 40, 2169–2178. doi:10.1002/cjoc.202200250 |
Zeng, L.-G., Chen, D.-L., Wang, F.-P., and Shen, X.-L. (2007). Study on norditerpenoid alkaloids of Delphinium laxicymosum. West China J. Pharm. Sci. 22 (6), 299–300.
Zhang, J.-F., Dai, R.-Y., Shan, L.-H., Chen, L., Xu, L., Wu, M.-Y., et al. (2016). Iliensines A and B: Two new C19-diterpenoid alkaloids from Delphinium iliense. Phytochem. Lett. 17, 299–303. doi:10.1016/j.phytol.2016.08.014 |
Zhang, S., and Ou, Q. (1998). Norditerpenoid alkaloids from Delphinium siwanense var. Phytochemistry 48 (1), 191–196. doi:10.1016/S0031-9422(97)00579-7 |
Keywords: delphinium grandiflorum, ranunculaceae, diterpenoid alkaloid, grandiflolines A-F, anti-inflammatory activity
Citation: Yan Y, Jiang H, Yang X, Ding Z and Yin T (2022) Grandiflolines A–F, new anti-inflammatory diterpenoid alkaloids isolated from Delphinium grandiflorum. Front. Chem. 10:1012874. doi: 10.3389/fchem.2022.1012874
Received: 06 August 2022; Accepted: 05 September 2022;
Published: 19 September 2022.
Edited by:
Jian-Wei Dong, Qujing Normal University, ChinaReviewed by:
Zheng Li, Jiangsu Normal University, ChinaCheng-Ting Zi, Yunnan Agricultural University, China
Copyright © 2022 Yan, Jiang, Yang, Ding and Yin. This is an open-access article distributed under the terms of the Creative Commons Attribution License (CC BY). The use, distribution or reproduction in other forums is permitted, provided the original author(s) and the copyright owner(s) are credited and that the original publication in this journal is cited, in accordance with accepted academic practice. No use, distribution or reproduction is permitted which does not comply with these terms.
*Correspondence: Tianpeng Yin, ytp@zmu.edu.cn