- 1Department of Chemistry and CICECO-Aveiro Institute of Materials, University of Aveiro, Aveiro, Portugal
- 2Department of Biology and CESAM-Centre of Environmental and Marine Studies, University of Aveiro, Aveiro, Portugal
Water is the most important resource for all kind forms of live. It is a vital resource distributed unequally across different regions of the globe, with populations already living with water scarcity, a situation that is spreading due to the impact of climate change. The reversal of this tendency and the mitigation of its disastrous consequences is a global challenge posed to Humanity, with the scientific community assuming a major obligation for providing solutions based on scientific knowledge. This article reviews literature concerning the development of nanomaterials for water purification technologies, including collaborative scientific research carried out in our laboratory (nanoLAB@UA) framed by the general activities carried out at the CICECO-Aveiro Institute of Materials. Our research carried out in this specific context has been mainly focused on the synthesis and surface chemical modification of nanomaterials, typically of a colloidal nature, as well as on the evaluation of the relevant properties that arise from the envisaged applications of the materials. As such, the research reviewed here has been guided along three thematic lines: 1) magnetic nanosorbents for water treatment technologies, namely by using biocomposites and graphite-like nanoplatelets; 2) nanocomposites for photocatalysis (e.g., TiO2/Fe3O4 and POM supported graphene oxide photocatalysts; photoactive membranes) and 3) nanostructured substrates for contaminant detection using surface enhanced Raman scattering (SERS), namely polymers loaded with Ag/Au colloids and magneto-plasmonic nanostructures. This research is motivated by the firm believe that these nanomaterials have potential for contributing to the solution of environmental problems and, conversely, will not be part of the problem. Therefore, assessment of the impact of nanoengineered materials on eco-systems is important and research in this area has also been developed by collaborative projects involving experts in nanotoxicity. The above topics are reviewed here by presenting a brief conceptual framework together with illustrative case studies, in some cases with original research results, mainly focusing on the chemistry of the nanomaterials investigated for target applications. Finally, near-future developments in this research area are put in perspective, forecasting realistic solutions for the application of colloidal nanoparticles in water cleaning technologies.
1 Introduction
Research on colloidal inorganic nanomaterials has developed considerably in the last decades. Taking the last century transition period as a yearly timeline reference, it is possible to trace to about that period the development of important chemical methods for the synthesis of quantum dots, magnetic metal oxides and metals, just to mention a few relevant classes of nanomaterials (Brus, 1984; Ekimov et al., 1985; Bawendi et al., 1990; Steigerwald and Brus, 1990; Schmid, 1992; Weller, 1993; Alivisatos, 1996; Leslie-Pelecky and Rieke, 1996; López-Quintela and Rivas, 1996; Trindade and O’Brien, 1996; Weller, 1998; Manna et al., 2000; Peng and Peng, 2001; Trindade et al., 2001; Esteves and Trindade, 2002; Frey et al., 2009; Teitelboim et al., 2016). Associated to the development of synthetic methods of nanosized colloids has emerged a new paradigm in inorganic synthesis concerning the control of size and shape-dependent intrinsic properties of colloidal particles (Jun et al., 2006; Grzelczak et al., 2008; Tao et al., 2008; Xia et al., 2009; Guerrero-Martínez et al., 2011; Lim and Xia, 2011; Watt et al., 2013; Zhang et al., 2013). Indeed, it is at the nanoscale that several crystalline solids show the transition of the bulk-type behaviour to a cluster-like behaviour as particle size decreases, which scales with a critical dimension identified for that type of solid. Hence, it is now routine to employ chemical methods for preparing semiconductor nanocrystals, whose band gap can be tuned by adjusting the particle size distribution below a certain threshold defined as the Bohr radius of that semiconductor or to synthesise superparamagnetic iron oxides whose dimensions are below the size of Weiss-domain. The control of the optical, electronic and magnetic properties of materials using size (and shape) as additional parameters of chemically controlled characteristics of particles has important consequences in advancing the frontiers of knowledge and on new applications of materials, which is one of the reasons, among others, for which Chemistry is regarded as a central science also in Nanotechnology (Whitesides, 2005; Yina and Talapin, 2013). Furthermore, the properties, manipulation, processing and applications of colloidal inorganic nanoparticles are markedly dependent on their surface chemistry, which has been an active research topic in the last 2 decades, including in our laboratory (Yin and Alivisatos, 2005; Boles et al., 2016; Daniel-da-Silva and Trindade, 2021).
The unique properties of inorganic nanoparticles make them suitable for applications in diverse areas, which include nanomedicine (e.g., drug delivery, bioimaging, phototerapies) (Lin et al., 2007; Sokolova and Epple, 2008; Trindade and Daniel da Silva, 2011; Vega-Vásquez et al., 2020; Wang and Mattoussi, 2020; Ray and Bandyopadhyay, 2021), energy (e.g., solar cells, lightning) (Kamat, 2008; Cheng et al., 2016; Kumar et al., 2017; Chen et al., 2018; Choe et al., 2021), environment (e.g., water and air purification (Theron et al., 2008; Kumari et al., 2019; Weon et al., 2019; Wadhawan et al., 2020; Li et al., 2021), greenhouse gases capture (Kumar et al., 2020; Cheng et al., 2021), sustainable technologies (e.g., catalysis, biological synthesis) (Polshettiwar and Varma, 2010; Dutta et al., 2015; Kumari et al., 2020; Jeevanandam et al., 2022; Liu et al., 2022; Wani and Suresh, 2022), among many others. These endeavours require transdisciplinary approaches, among which the synthesis and surface modification of the materials are usually regarded at the upstream in the supply chain of functional materials for such devices and processes. A main aspect of our late research has been the exploration of chemical methods for modifying the surface of inorganic nanoparticles, as shown below with distinct systems. Mostly of these methods rely on colloid chemistry of inorganic particles establishing important bridges to other scientific domains, such as polymer chemistry and coordination chemistry. In this context, a series of functional inorganic nanoparticles have been investigated, depending on the target application.
Several water cleaning technologies have been developed, including adsorption, photocatalysis and membrane filtration. Adsorption is an efficient method, very attractive due to the affordable cost and easy operation. Photocatalysis enables water treatment in an eco-friendly manner because solar radiation can be used as an energy source. However, complete mineralization of the pollutants is challenging and may result in oxidation by products formation. The interest in membrane filtration technologies has grown significantly in the last years, but it still faces challenges in membrane fouling, concentrate disposal and high energy consumption. Together with remediation technologies, preventing measures should be also implemented namely by developing water quality monitoring protocols, that can be easily accessed in different regions of the globe. As an example of this approach, several sensors have been investigated, namely envisaging the optical detection of water contaminants using low-cost and disposable substrates. In this work, we focus on the research of colloidal inorganic nanomaterials and their composites, aiming at nanotechnologies for water cleaning and quality monitoring, which has been a common ground for some of the research carried out in our laboratory during the past years.
2 Nanomaterials for water cleaning nanotechnologies
2.1 General framework
Water and wastewater management is a major global concern considering an ever-increasing population, Human-driven water pollution and the scarcity of freshwater resources. Specifically, water pollution is critical since it may cause negative impacts on ecosystems and Human health, and it can as well adversely impact economic growth and the social perspectives of societies. Considering this framework, the Organization of United Nations (UN) acknowledged that the availability of good water quality is vital for all living creatures and must be considered a worldwide challenge for this century. The preface of the last edition of the “Guidelines for drinking-water quality” published by the World Health Organization begins with the undisputable sentence: “Access to safe drinking-water is essential to health, a basic human right and a component of effective policy for health protection” (WHO, 2022). Among the seventeen Sustainable Development Goals agreed by world leaders, the Goal 6 (SDG 6) aims to ensure access and sustainable use of water for everyone, while SDG 15 aims to protect, restore and promote sustainable use of ecosystems. Water contamination is a global problem affecting populations and ecosystems, putting at risk such goals, namely by limiting the access to safe drinking-water of large populations worldwide. The prioritisation of this issue has increased in the past decades due to several factors, which includes accentuated water scarcity due to climate changes and increasing levels of water contaminants of emerging concern (CEC). The complexity of this global problem requires actions and decisions at very diverse levels, engaging different actors, from the ordinary citizen to policy decision makers and, with obvious social responsibility, the scientific community.
In this context, several nanotechnologies have been developed with potential for implementation and contribute for water remediation and quality improvement. Most of these procedures rely on the use of nanoengineered materials, which have been surface modified accordingly to the envisaged application and the target contaminants to be eliminated. Probably future developments in this area should be better considered as complementary to conventional water cleaning technologies, which for several purposes are quite effective but less so for the elimination of CEC in certain aqueous environments. Also, water quality monitoring technologies that are easily implemented and communicated in different parts of the globe, including in remote regions, are regarded as important advancements, namely because they value prevention behaviour in relation to remediation practices.
The following article sections are built upon research lines that converge in providing colloidal nanomaterials for water quality improvement and monitoring. Figure 1 illustrates these thematic lines, which have been investigated in our research activity during the past years, as well as new research lines. Rather than providing an exhaustive literature survey on each topic, we favour the communication of our own perspectives on nanomaterials developments that have a great of deal to contribute for water cleaning nanotechnologies as identified in illustrative cases of study. Ideally, clean water for all should be supported in sustainable and clean technologies, favouring water contamination prevention rather than remediation measures, but in fact contaminated water is a real problem and purification nanotechnologies can contribute for its solution. The next paragraph provides a summary of topics approaching both general strategies, by following Figure 1 as an illustrative guide.
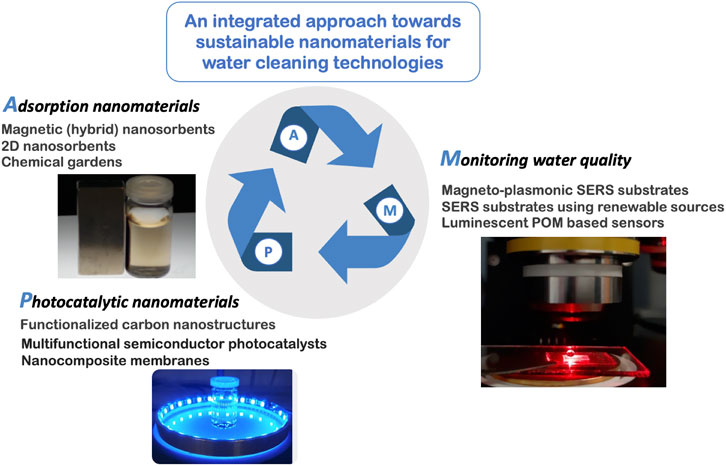
FIGURE 1. Research topics on sustainable nanomaterials for improving water quality by Adsorption, Photocatalysis and Monitoring by SERS sensors.
Adsorption techniques applied to water purification are well-established and carbon activated sorbents are ubiquitous in several procedures. However, the removal of certain water pollutants can be ineffective by using such processes, which requires non-conventional approaches. Surface modified nanosorbents for magnetic separation technologies and multifunctional nanostructures for photocatalysis are examples of nanomaterials that might have impact on new technologies for CEC removal or its prevention. Under this scope, chemical gardens have also attracted our attention. These are self-organizing structures created by nonequilibrium processes and have been known since the discoveries made by Johann Glauber (1646). Still, chemical gardens hide new possibilities for technological applications, including selective adsorption-desorption processes. It is known that these micro- or nano-tubules have internal reactive surfaces whose chemistry can be explored to develop sorbents for CEC.
Contexts of applications are diverse but typically might include water treatment stations of industries, hospitals, and municipalities. These applications are clearly dependent on the functionality and associated efficacy of the nanoengineered materials, for which surface chemistry plays a major role. The presence of CEC in water, even in vestigial amounts, poses serious threats to human health and jeopardizes the access of populations to safe drinking-water. Thus, not only water purification methods effective for pollutants’ diminutive amounts are required, but accessible and sensitive sensors for water quality monitoring would be a step forward for management of water supplies. Nanotechnology provides a myriad of possibilities for fabricating reliable sensors, which ideally should also be low-cost and user friendly. Together with technologies based on the internet of things, these sensors might offer on-time answers for water contamination events which might occur in remote zones. As it happens for water remediation and purification technologies, the nanosensors’ performance depends on the materials used, namely on their selectivity and nanoscale properties that can be explored for achieving lower detection limits in the field analysis. Optical sensors based on low-cost and versatile SERS substrates are among the most promising for several applications in this context. The risk-benefit balance of these nanomaterials in water cleaning technologies is crucial to decide on their practical use in real contexts. Studies on the assessment of the impact on health and environment of these materials have been carried out, providing a wealth of data that requires a critical and thoroughly analysis. Our research in this area has been mostly collaborative, in providing nanomaterials that have been found as promising for experts on nano- and eco-toxicology to assess, as is also illustrated in this work as an important part of research in this area.
2.1 Functionalized magnetic nanosorbents
2.2.1 Biocomposite particles
Biopolymers, i.e., natural polymers produced by living organisms, are sustainable materials with low toxicity, abundant and available, whose structure contains functional groups with an affinity towards diverse pollutants. The properties of biopolymers and their composites have prompted a focus on water treatment applications, namely as adsorbents for water decontamination (Nasrollahzadeh et al., 2021; Yaashikaa et al., 2022). One of the major issues with the adsorption-treatment process is the recovery of spent sorbents, which should be easy and cost-effective (Baskar et al., 2022). Combining biopolymers with magnetic iron oxide nanocrystals results in composites that are easily recovered using magnetic separation, making them very appealing for water treatment through adsorption (Abdel Maksoud et al., 2020; Soares et al., 2020). A rational modification of the surface of the magnetic nanocrystals with the biopolymer is necessary to attain pollutant specificity, high adsorption capacity and reusability. For example, covalent immobilization of the biopolymer onto the surface of the particles is critical to ensure the successful recycling and reuse of the biosorbents.
Our group has developed a method for preparing magnetic biosorbents that took in consideration the context above. The biopolymer is grafted onto a siliceous network that acts as a surface coating of Fe3O4 nanoparticles during the encapsulation process, yielding an organic-inorganic hybrid shell enriched in the biopolymer component. The encapsulation encompasses the hydrolysis and condensation of a mixture of tetraethyl orthosilicate (TEOS), as the SiO2 precursor, and a silicon alkoxide derivative of the biopolymer, in the presence of colloidal magnetic particles. Figure 2A illustrates the preparation of the magnetic biosorbents using the polysaccharide κ-carrageenan, encompassing the derivatization of the biopolymer with a silane coupling agent functionalized with isocyanate groups (3-isocyanatopropyl triethoxysilane-ICPTES) and the subsequent coating of the Fe3O4 nanoparticles. This method originates more robust coatings and with a higher degree of functionalization when compared to the conventional techniques of non-covalent coating and surface-grafting approach, which is desirable to obtain reusable sorbents with improved adsorption capabilities. A series of magnetic biosorbents have been prepared by using this route, from polysaccharides with distinct ionic character and variable chemical functionalities, which were efficient in the uptake of target pharmaceuticals (Soares et al., 2016; Soares et al., 2019a; Soares et al., 2019b; Soares et al., 2019c; Soares et al., 2021b; Soares et al., 2022), pesticides (Fernandes et al., 2017; Soares et al., 2021a), organic dyes (Soares et al., 2017b), and non-polar organic solvents from water (Soares et al., 2017a). The biopolymer selected allows the preparation of magnetic nanosorbents with various chemical functionalities and tunable surface charge (Figure 2B). This is a crucial step to attain high adsorption capacity because electrostatic interactions between oppositely charged groups of the biopolymer and the pollutant species play an essential role in their adsorption. Nevertheless, other interactions, such as H-bonding and hydrophobic interactions, may also contribute to the adsorption process (Crini et al., 2019; Badsha et al., 2021). Assessing the adsorptive performance in conditions closest to the final application is of utmost importance in developing effective treatments. This assessment frequently involves testing the removal of the pollutant in trace concentrations in aqueous matrices of complex nature. In this context, magnetic biosorbents prepared from trimethyl chitosan-based decreased environmentally realistic concentrations of the pesticide glyphosate in spiked effluents of wastewater treatment plants up to 80%, demonstrating their potential application in real conditions (Soares et al., 2021a).
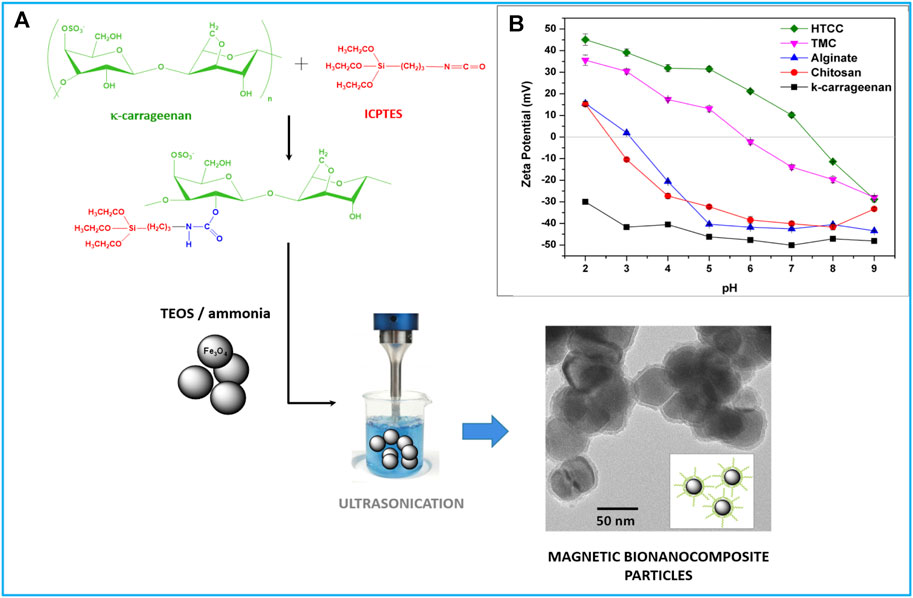
FIGURE 2. (A) Scheme illustrating the chemical route to prepare the silicon alkoxide derivative of the biopolymer k-carrageenan and the preparation of the magnetic biosorbents through coating the Fe3O4 nanoparticles with the bio-hybrid siliceous shell; (B) Potential zeta values of magnetic biosorbents prepared using distinct biopolymers (HTCC -N-(2-hydroxypropyl)-3-trimethylammonium chitosan; TMC- trimethylchitosan).
2.2.2 Carbon-based 2D materials
Carbon is one of the most abundant elements in the environment and human body (Rao et al., 2021), with the particularity of having an extraordinary ability to bind to other elements. Carbon has extensive allotropy but, until the eighties, few carbon allotropic forms were known, namely, diamond, graphite and fullerene (Kallumottakkal et al., 2021). Since then, many other allotropes have been discovered and produced, including ball shapes such as buckminsterfullerene and sheets such as graphene, opening a research window for a variety of carbon nanomaterials of distinct structural dimensionality.
Two-dimensional nanomaterials are considered the thinnest nanostructures among the different dimensional groups, and contrary to bulk materials, have a high surface-area-to-volume ratio (Kallumottakkal et al., 2021). In the 2D nanostructures, the relative number of external to internal atoms is higher, and their different functions lead to a change in the behavior of 2D nanomaterials, in comparison to the other dimensional groups, conferring them a variety of unique physical, chemical, and electrical properties (Rafiei-Sarmazdeh et al., 2019). Graphene is a 2D material with a hexagonal structure composed of sp2-hybridized C atoms, where each carbon atom is covalently bound with each other in the same plane (Kallumottakkal et al., 2021). For water compatible applications, pristine graphene faces some problems such as poor solubility and agglomeration tendency (Joseph et al., 2021). To overcome these limitations, researchers have come up with a handful of techniques to synthesize structurally similar compounds, like graphene oxide (GO) and graphite-like nanoplatelets (GNP), which can be produced from carbon sources by simple top-down methods (Rao et al., 2021).
Our research group has been interested in exploring GNP for their adsorption capability and ability to enhance the adsorption capacity of various composites, with the aim of removing toxic metal ions from water and/or recovering valuable elements, such as technology critical elements. There has been great interest in this topic (Cardoso et al., 2019) because emerging key technologies are intrinsically dependent of a limited number of elements often termed by technology critical elements (TCE), which includes all lanthanoids and the platinum group elements (Pinto et al., 2021). GNP are a viable and inexpensive materials that can be used as stable substrates for preparing magnetic nanocomposites for water adsorption treatment, thus allowing their use in magnetic separation technologies, as already discussed in the previous section. The GNP are decorated with nanoparticles of spinel ferrites (MFe2O4, M = Fe, Mn, Co.), thereby conferring ability for magnetic separation of the sorbents when exposed to an external magnetic gradient.
The magnetic nanostructures have been prepared by in situ alkaline hydrolysis or electrostatic assembly of particulates previously synthesized. Figure 3 shows TEM images of spinel ferrites, with average sizes below 100 nm, attached onto GNP substrates. All materials quickly approach saturation in the presence of an external magnetic field, and the estimated saturation magnetization, at 300 K, is about 45 emu/g for Fe3O4/GNP and 29 emu/g for the CoFe2O4/GNP and MnFe2O4/GNP composites. As expected, these estimated saturation magnetization values are lower than the values of the respective spinel ferrites at room temperature (Fe3O4 92 emu/g, CoFe2O4 55 emu/g and MnFe2O4 48 emu/g).
The Fe3O4/GNP nanocomposites were investigated to capture selected lanthanoids (La, Eu, and Tb), from unary and ternary solutions, and their performance was compared with the one observed for the isolated components (Fe3O4 and GNPs) (Afonso et al., 2019). After a 24 h period of exposition of equal amounts of GNP, Fe3O4 NPs and Fe3O4/GNPs to a ternary solution of La(III), Eu(III) and Tb(III), with an elemental concentration of 100 μg/L, the results show distinct affinity between materials and elements, with the Fe3O4/GNPs composite showing better capability to remove the target elements from water than any of its counterparts (La: 4.7% (GNPs), 3.1% (Fe3O4 NPs), and 15% (Fe3O4/GNPs); Eu: 1.2% (GNPs), 0.2% (Fe3O4 NPs) and 37.6% (Fe3O4/GNPs); Tb: 0.2% (GNPs), 0.1% (Fe3O4 NPs) and 35.0% (Fe3O4/GNPs)). The better performance of the nanocomposites can be explained by an increase of active sorption sites due to the introduction of oxygen moieties into the carbon lattice. Trivalent lanthanides are hard Lewis acids with strong chemical affinity for oxygen donors. More, the sorption process showed to be very dependent from solution pH, and only feasible for pH values higher than 5.2, which is the experimental isoelectric point of the composite (Afonso et al., 2019). Consecutive sorption and desorption cycles were also applied to ternary solutions of TCE (100 μg/L) and for the best experimental conditions studied (pH 7.5, 250 mg/L of Fe3O4/GNPs) and using 0.1 M HNO3 as eluent solution. During the consecutive cycles, the recovery efficiency of the target elements ranged from 82 to 92% for La (III), 88–104% for Eu (III) and 82–97% for Tb (III). These data elucidate that dilute nitric acid solution (0.1 mol/L HNO3) is an efficient eluent for the recovery of these elements from the magnetic composite. More, the capability of this nanostructure to remove the selected TCE from water was rarely affected since the values of the removal efficiency remained nearly constant during all cycles, also confirming the stability of the magnetic composite.
In other study, Fe3O4/GNPs, CoFe2O4/GNPs and MnFe2O4/GNPs composites were investigated to capture mercury and arsenic, from unary and binary solutions. Among the toxic substances of anthropogenic and natural origin, these elements pose greatest threat to the environment, due to their non-degradable nature, severe toxicity and bioaccumulative character (Lopes et al., 2008; Tavares et al., 2020). Arsenic and Hg occupy the 1st and 3rd positions, respectively, on the list of priority hazardous substances of 2019 provided by the Agency for Substances and Toxic Diseases, and so their effective removal from water is a priority to achieve the SDG6 of United Nations. The pH dependency, of the sorption process using these magnetic nanostructures was assessed for the range 4–9. After a 24 h period of exposition of equal amounts of MFe2O4/GNPs, to unary solutions of As(III) (1000 μg/L) and Hg(II) (50 μg/L), the results showed that 1) Fe3O4/GNPs were the least effective for removing As(III) or Hg(II) and, 2) As(III) removal was higher for the CoFe2O4/GNPs at pH 7 (90.4%), while MnFe2O4/GNPs displayed higher efficiency to remove Hg(II) at pH 6 (90.6%). In binary solutions, CoFe2O4/GNPs showed more efficiency to As(III) with removal percentages near 90% in ultra-pure water and around 80% in tap water, while MnFe2O4/GNPs removed preferably Hg(II) (85%) in both matrices. Although the above nanocomposites were prepared by similar methods, those containing Co and Mn ferrites performed better than the Fe3O4/GNPs but on the other hand show more potential for metal leaching (Tavares et al., 2020). Indeed, the ferrites nanoparticles display different metal leaching (20–50% of Co, 20–30% of Mn, and <8% of Fe). The free Mn, Co, or Fe sites located at the surfaces of the respective ferrites could exchange with ions in solution, working as active sorption sites, and the different degree of leaching could also explain the different affinity toward the ions.
These studies highlight that magnetic graphite-like nanoplatelets composites can be explored as effective sorbents for different elements. The nanocomposites are prepared using a simple and environmentally friendly procedure, with potential for future application in the removal of toxic elements and/or in the recovery of valuable elements, such as TCE, from industrial or other water systems.
2.3 Nanocomposites for photocatalysis
2.3.1 Metal oxide nanophotocatalysts
TiO2 has been the most used semiconductor photocatalyst for effluent treatment because it is relatively inexpensive, photostable over a wide range of pH and the photo-generated hole-electron pairs are efficient in producing highly reactive oxygen species (Kubacka et al., 2012). In particular, TiO2 colloidal nanoparticles have several advantages because of their high surface-to-volume ratio, increased number of delocalized charge carriers over the surface, improved charge transport and lifetime afforded by their dimensional anisotropy, and efficient contribution to the separation of photo-generated holes and electrons (Wang et al., 2014). Previous studies have shown that adsorbable organic halides (AOX) produced in the bleaching treatment stage in pulp and paper mills, can be photodegraded under UV radiation by using TiO2 or ZnO photocatalysts (Yeber et al., 2000; Ugurlu and Karaoglu, 2009; Kumar et al., 2011). Typically, the metal oxides are dispersed in the effluent as colloids and, at the end of the treatment, the separation and recovery of the photocatalysts are required, which in practice is a costly task and also poses raises issues concerning their effective separation. As discussed below, the immobilization of the catalyst in active supports is a promising strategy, but for conventional immobilized systems there is usually less efficiency in photodegradation due to a reduction in the specific surface area of the catalyst (Li et al., 2009). Alternative strategies have been investigated, such as coupling TiO2 semiconducting phases to magnetic nanostructures, enabling magnetic separation of the hybrid photocatalysts without need additional downstream treatment processes. However, these strategies have been mostly tested in degrading dye pollutants in synthetic solutions (Li et al., 2009; Abbas et al., 2014; Gómez-Pastora et al., 2017). There is a lack of studies regarding the applicability of magnetic TiO2 hybrids in the treatment of real effluents, namely regarding photodegradation of AOX in samples from pulp and paper mills. The following paragraphs in this sub-section describe our research on this scientific problem by using real effluents provided by an industrial unit localized in the Aveiro (Portugal) region.
First, the TiO2 photocatalysts were designed for their magnetic properties through the co-precipitation of magnetite (Fe3O4) in the presence of P25 TiO2. Magnetic water-dispersible TiO2 nanostructures, with a magnetite content of 86 wt% (hybrid A) and 67 wt% (hybrid B), have been prepared by varying the reactants ratio. The ensuing hybrid nanostructures were composed of spheroidal magnetite 12 ± 2 nm in diameter and a TiO2 nanoparticles (24 ± 9 nm), in a ratio 80/20 wt% of anatase/rutile phases. The Fe3O4/TiO2 hybrids were characterized by an absorption edge in the visible region, which is dominated by the Fe3O4 adsorption whose direct band-gap energy is 2.27 eV. The magnetic TiO2 hybrids were then tested for degrading AOX compounds present in the effluent of the bleaching stage from Kraft pulp mills (pH = 2), under UV light irradiation in the presence of H2O2 as the co-catalyst.
AOX photodegradation was observed regardless the amount of TiO2 content in the hybrid photocatalyst. Although the highest AOX removal was observed when using non-magnetic TiO2, the magnetic hybrids enabled 59–73% AOX removal after 60 min under UV irradiation (Figure 4), with the great advantage that could be separated from the treated effluent by magnetic separation and subsequently reused. The identity of the hybrids was intact after two photocatalytic cycles, even though a slight loss of photocatalytic activity and mass loss was observed.
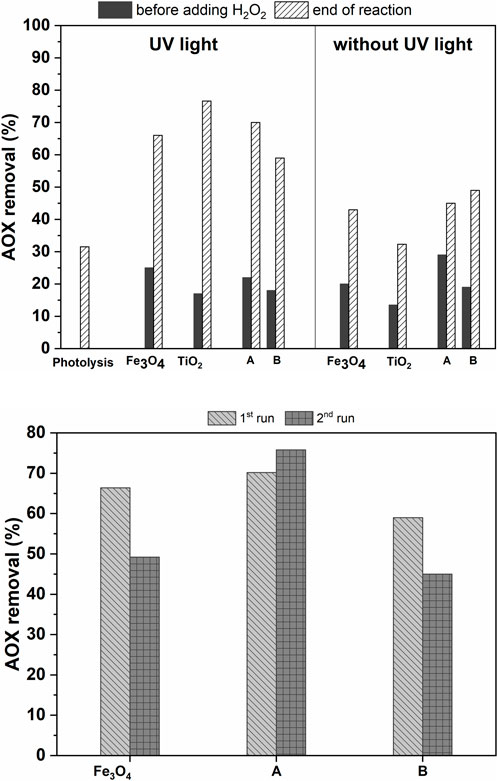
FIGURE 4. AOX removal in a real aqueous effluent as a function of photocatalyst used (A) in the presence or absence of UV light irradiation (top) and in two consecutive photocatalysis runs using Fe3O4 and Fe3O4/TiO2-A and B photocatalysts (bottom). Reaction conditions: 2 mg of photocatalyst; AOX effluent (200 ml); 30 wt%, H2O2 (9.8 mmol); 57°C; 1 h reaction time.
2.3.2 POM supported photocatalysts
Polyoxometalates (POMs) show multi-advantages for application as photocatalysts (Walsh et al., 2016), namely their switch-ability by visible light, the possibility of multi-electronic photoreduction, followed by reversible electron exchange with a substrate, the possibility of heterogenization and finally a low cost. The possibility of heterogenization without loss of POM properties is very important for the application of POM based nanomaterials in photocatalysis.
In this work we have been developing new nanostructured hybrid materials based on polyoxometalate clusters supported in graphene (Granadeiro et al., 2012). Graphene supports were obtained by graphene oxide reduction (rGO, reduced graphene oxide). These materials have been optimized for application in the photocatalytic degradation of water pollutants of pharmaceutical origin, one of the most important classes of emergent environmental contaminants (Lopes et al., 2021). The new electron transfer features resulting from the synergetic combination of POMs and rGO have been explored in photocatalysis.
To evaluate the photocatalytic activity in the visible of the polyoxometalate [PW11CoO39]5− supported in rGO (rGO-PW11Co), the photodegradation of Rhodamine B (RhB) under visible-light irradiation was conducted at room temperature. RhB was selected as an organic dye model because it shows strong absorption in the visible region (maximum at 554 nm, Figures 5, 0 h) and is photostable in the absence of photocatalyst. As control, identical experiments in the dark were also carried out. The results for a blank experiment, without catalyst, demonstrate that RhB concentration was practically unchanged, suggesting that RhB is stable under visible-light irradiation. Comparative photocatalytic studies using as catalyst the supported rGO-PW11Co material and the pure solid K5 [PW11CoO39], respectively, under the same experimental conditions, demonstrate that rGO-PW11Co shows higher photocatalytic activity than the polyoxometalate by itself. The photodegradation of RhB is closed to 95% after 600 min in the presence of rGO-PW11Co catalyst, under visible-light irradiation. The degradation of RhB is followed by the decrease in the characteristic absorbance at 554 nm as well as the maximum absorption shift over time (Figure 5).
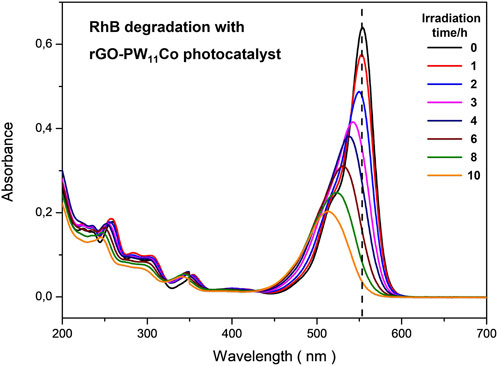
FIGURE 5. Absorption spectra recorded during the photocatalytic degradation of Rhodamine B (10 ppm) in the presence of 14 mg of rGO-PW11Co under irradiation of a 400 W halogen lamp.
The later example presents a POM based heterogeneous photocatalyst (rGO-PW11Co) that shows activity under promising conditions, in particular the use of visible-light for irradiation and room temperature.
2.3.3 Nanoparticles immobilized in/on membranes
Although photoactive nanoparticles have demonstrated effectiveness for the removal of CEC in wastewater, their direct application as free nanoparticles in suspension mode has also limitations. Nanoparticles tend to aggregate with time, due to Van der Walls interactions, which decrease their performance (Lofrano et al., 2016). Most importantly it requires a complex post-treatment step for the recovery/separation of the solid slurry, with a substantial impact on the overall process cost (Loeb et al., 2019). Thus, more effective strategies have been explored based on the immobilization of nanoparticles in/on membranes (Shen et al., 2020). Besides the fundamental economic advantage, coupling nanoparticles with membranes allow easy scaling and reusability with fewer environmental risks, thus contributing to their real application in wastewater treatments. In these systems, the membrane has the simultaneous role of catalyst support while acting as a barrier for the pollutants to be degraded (Iglesias et al., 2016). Retained molecules during filtration, depending on their physical and chemical properties, are forced towards the membrane increasing their concentration near the liquid/catalyst interface, thus boosting the degradation rate (Presumido et al., 2021). Moreover, nanocomposite membranes are of interest as it also reduces the membrane fouling effect, which remains the notorious drawback of micro- (MF), ultra- (UF) and nanofiltration (NF) (Xu et al., 2020).
The introduction of nanoparticles promotes changes in morphology and enhances membrane hydrophilicity due to their polarity. Following the solution–diffusion theory, an increase in the nature of the membrane benefits water diffusion and controls the transport process through the membrane, improving its permeability. Conversely, immobilized nanoparticles have reduced active surface area, which entails the maximization of crucial parameters such as light irradiation and mass transfer (Alipour Atmianlu et al., 2021). Different oxides nanoparticles (photocatalysts) have been explored, such as TiO2 (Martins et al., 2016), ZnO (Shen et al., 2020), ZrO2 (Huang et al., 2021), WO3 (Gondal et al., 2017), as well as carbon-based nanomaterials such as GO (Kusworo et al., 2021), g-C3N4 (Li et al., 2019) or SWCNTs (Jue et al., 2020).
Due to high thermal, chemical and mechanical stability, the most used membrane matrix materials are ceramic (Zhang et al., 2020) and polymeric, such as polyvinyl fluoride (PVDF) (Cui et al., 2019), polytetrafluoroethylene (PTFE) (Feng et al., 2018) and polyaniline (PANI) (Vijayakumar and Khastgir, 2018). Nanocomposite membranes can be prepared by nanoparticles surface coating (Figure 6A) or entrapping nanoparticles thru blending with the membrane substrate during the manufacturing process (Figure 6B), or even as a free-standing catalyst (Homocianu and Pascariu, 2022).
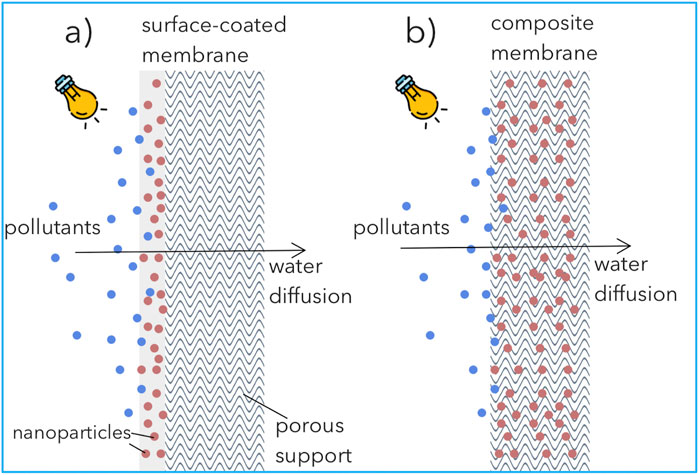
FIGURE 6. Schematic representation of nanoparticles immobilized in/on composite membranes: (A) surface-coated membrane; (B) nanoparticles matrix-blended membrane.
Surface-coated membranes can be obtained by dip-coating, electrospraying, sputter deposition or gas-phase deposition which generates a nanoparticles layer on its surface (Lakhotia et al., 2018; Alipour Atmianlu et al., 2021). Horovitz et al. (2016) reported the dip-coating of a commercial alumina membrane with N-doped TiO2 by the sol-gel method. Substantial gain in the carbamazepine removal was observed, however, with a loss in the permeability. Recently (Barati et al., 2021), observed that the in-situ growth of hydrophilic iron oxides nanoparticles (<4 nm) did not modify the morphology, porosity or the intrinsic hydraulic resistance of ceramic membranes, however, it significantly increased the rejection of humic acid. Higher loading of iron oxides promoted a porous obstruction, thus a drop in permeability.
Matrix-blended membranes obtained by blending nanoparticles in the membrane matrix allow to minimize the possible catalyst leaching. For fabrication, nanoparticles are dispersed by mixing in the polymer-solvent solution before phase inversion. Martins et al. (2016) immobilized 3-8 wt% TiO2 nanoparticles in porous PVDF/NaY-based membrane via solvent casting method. Interestingly, the authors reported a minor loss (∼3%) in pollutant removal efficiency compared to the nanoparticle suspension assays. Following a similar strategy, Luo et al. (2021) immobilized NCQDs/BiOBr/TiO2 in PVDF membranes with strong antifouling properties under visible light irradiation. The use of blended nanoparticles promoted the degradation of the pollutant while enhancing the filtration performance due to lower membrane fouling. Yet, the membrane permeability can be compromised due to porous hindrance when high nanoparticle loading is used.
In summary, nanoparticles immobilized in membranes is an effective strategy to overcome the disadvantages associated with conventional slurry-type systems. Surface-coated membranes can improve hydrophilicity and provide antifouling properties; however, the strategy can be ineffective in preventing catalyst leaching. Nanoparticles blended in the membrane matrix are more successful in retaining the catalyst, but the antifouling capacity can be inferior. Deeper optimization to assure porosity, surface dispersion, high photocatalytic activity and improve mass transfer is required to enlarge the broad market commercialization of self-cleaning nanocomposite membranes for environmental application.
2.4 Nanostructured platforms for SERS detection
Fleischmann and co-workers noticed the SERS effect for the first time in 1974 (Fleischmann et al., 1974), with its interpretation and deep understanding of underlying mechanisms developed in the subsequent years (Albrecht and Creighton, 1977; Jeanmaire and Van Duyne, 1977; Jensen et al., 2008; Stiles et al., 2008; Valley et al., 2013). However, it was mainly during the last decades that SERS has been regarded as an essential method for water quality monitoring, offering new possibilities for practical implementation and, in certain cases, lowering analytical detection limits in established analytical protocols (Pinheiro et al., 2018; Tang et al., 2018; Bodelón and Pastoriza-Santos, 2020; Ong et al., 2020). The increasing interest in the application of SERS to water quality monitoring is inseparable from last developments in terms of instrumentation (e.g., detection sensitivity and portability) and also on the nanofabrication methods of analytical substrates, in which surface chemistry plays a determinant role (Li et al., 2014; Yılmaz et al., 2022). In fact, our interest in this research topic has been mainly driven by exploring chemical routes to produce a variety of nanostructured materials with specific features for SERS analysis (Fateixa et al., 2011; Fateixa et al., 2013; Fateixa et al., 2015; Fateixa et al., 2016; Pinheiro et al., 2019b).
2.4.1 Nanometal assemblies in polymeric substrates
The fabrication of flexible, low-cost and sensitive SERS substrates has gained great attention in environmental monitoring (Yu and White, 2012; Gao et al., 2016; Fateixa et al., 2018b; Guo et al., 2019; Sun et al., 2021). Polymer-based filter membranes show important features for these purposes, namely robust mechanical resistance and tuneable pore size. These membranes are available in various materials that allow sample concentration by large-volume filtration, promoting the target analyte uptake and, consequently, easier detection by SERS (Xu et al., 2021b). The lightweight and flexibility of membrane SERS substrates can also be combined with portable Raman spectrometers and smartphones, providing on-site detection (Gao et al., 2016; Guo et al., 2019; Sun et al., 2021). Several filtering membranes have been used as SERS substrates, such as cellulose (paper) (Lee et al., 2010; Moram et al., 2018; Wu et al., 2018; Jiao et al., 2022), polyamide (PA) (Yu and White, 2012; Shi et al., 2014; Fateixa et al., 2018a; Yu et al., 2021), PVDF (Yu and White, 2012; Gao et al., 2016; Guo et al., 2019; Sun et al., 2021), and derived polymer-based composites (Fateixa et al., 2018b; Ankudze et al., 2019; Ye et al., 2020). Polymer-based membranes offer flexibility, porosity and extensive fibrillar networks for surface chemical functionalization, which are important features for substrates fabrication, but they are not SERS active themselves. In this regard, plasmonic nanoparticles such as Ag or Au NPs are often used as the SERS active phases present in the filter membranes. The attachment of metal NPs onto the filter membranes can be performed by different approaches, such as 1) filtering colloidal NPs through the membrane (Yu and White, 2012; Shi et al., 2014; Fateixa et al., 2018a); 2) blending or in situ growth of NPs within the filter membrane (Lee et al., 2010; Moram et al., 2018; Wu et al., 2018; Yu et al., 2021); 3) coating the filter membrane with Au/Ag assembled structures (Guo et al., 2016); 4) modification of the fibres with NPs prior the membrane fabrication (Guo et al., 2016; Fateixa et al., 2018b; Ankudze et al., 2019).
A straightforward method for SERS substrates based on filter membranes involves syringe filtration of metal sols, as demonstrated by the early work of Yu and White. (2012) on Ag colloidal NPs supported on PA and PVDF membranes. They have demonstrated that this simple, economic and fast method provides efficient SERS substrates, which not only pre-concentrate the target analyte but also decrease the analysis detection time, with improvements in the Raman signal reproducibility of the molecular probe used. This topic was further explored in other laboratories using distinct filter membranes and plasmonic NPs with diverse morphologies, such as spherical Ag and Au NPs (Guo et al., 2016; Fateixa et al., 2018b; Sun et al., 2021; Yu et al., 2021), nanorods and nanowires (Lee et al., 2010; Shi et al., 2014; Wu et al., 2018; Ankudze et al., 2019), nanostars and nanourchins (Mehn et al., 2013; Xu et al., 2021a), and Au/Ag alloys or core-shell (Moram et al., 2018; Sha et al., 2020; Khan et al., 2022). Yu et al. (2021) have investigated Au/PA filter membranes for pesticide detection by comparing distinct fabrication methods, including in situ reduction, immersion-adsorption, and filtration. The latter method provided the SERS substrates with the best stability, reproducibility, and the lowest detection limit for the selected pesticides, compared with those prepared using other methods. Gao and co-workers have developed a rapid and sensitive methodology to detect the fungicide ferbam in aqueous samples using a portable Raman spectrometer (Gao et al., 2016). In this way, Ag colloids were previously aggregated by electrolyte addition (NaCl) and then filtered through a PVDF filter membrane. The Ag-loaded PVDF membranes allowed the SERS detection of ferbam dissolved in distilled, tap, and pond water samples at a low concentration of 2.5 μg/L.
Our interest in this topic led us to explore the fabrication of filter membranes using PA and liquid crystal polymer (LCP) for water CEC monitoring (Fateixa et al., 2018a; Fateixa et al., 2018b; Fateixa et al., 2019). A simple strategy was reported to fabricate filter membranes based on composites containing plasmonic NPs and Vectran™, a manufactured aromatic poly (esther) that shows properties useful for filtering membranes (Fateixa et al., 2018b; Fateixa et al., 2019). In fact, Vectran™ is a thermotropic LCP fiber with high Young modulus and high strength, superior heat resistance and excellent chemical stability in comparison to other conventional polymers. Hence, Au and Ag NPs with different morphology (spheres and rods) have been prepared by wet chemical procedures and successfully attached to the LCP fibres previously modified with polyelectrolytes. In this strategy, the optical properties are fine-tuned through judicious selection of the metallic NPs which have been prepared previous the composite fabrication. The metal-loaded nanocomposites were then submitted to hydraulic pressing to fabricate the filter membranes. These SERS substrates were investigated to uptake and detect thiram and paraquat dissolved in water samples using SERS coupled with Raman imaging (Figure 7A).
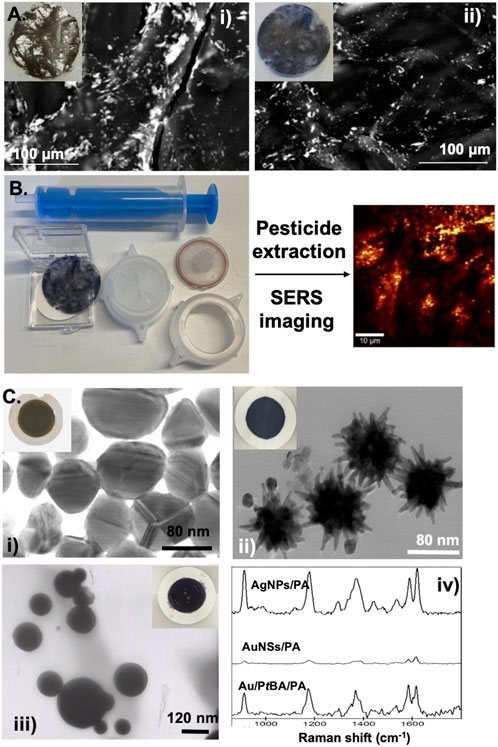
FIGURE 7. (A) SEM images of Ag/LCP (i) and Au/LCP (ii) composites prepared by layer-by-layer approach. Inset: digital photography of the MNPs/LCP fibres after pressed by hydraulic pressing (B) Schematic illustration of MNPs/LCP filter membrane preparation for SERS detection of pesticides dissolved in water. (C) TEM images of Ag NPs (i), Au nanostars (ii), and Au NPs coated with PtBA (iii) deposited on commercial PA filter membranes and used as SERS substrates for the detection of crystal violet (iv). Insets: digital photographs of PA filter membranes loaded with Ag NPs (i), AuNSs (ii) and Au/PtBA beads (iii).
Alternatively, the in situ citrate reduction method applied to Ag(I) dissolved in LCP aqueous suspensions resulted in Ag/LCP fibres, which were then pressed into filter membranes. As a proof of concept, the Ag/LCP filter membranes were supported on polyamide filters, which combine the SERS technique and share similarities with solid phase extraction due to the rapid collection of the molecular analyte for in situ detection (Figure 7B). These SERS substrates have shown better SERS response and selectivity for thiram molecules dissolved in water samples than other pesticides (e.g., paraquat). The SERS map shows a strong SERS signal of the thiram molecules, indicating not only the pesticide’s spatial distribution but also the active Raman regions (so-called hotspots) due to the presence of the Ag NPs (Fateixa et al., 2018b). These membranes applied to real aqueous matrices laboratorial spiked with thiram led to SERS signals with enhancement factors of 1.67 × 107 and 3.86 × 105, respectively, for Aveiro estuary water and fruit juices samples. The latter value was lower than the maximal residue limit of 5 ppm in fruit, as prescribed by European regulations (EU) 2016/1.
Other studies involved metal-loaded PA filter membranes fabricated by filtration under reduced pressure to detect crystal violet, a synthetic basic cationic dye used in veterinary medicine to treat fungal infections in aquacultures (Fateixa et al., 2018a; Fateixa et al., 2019). Commercial PA filter membranes with a porous size of 200 nm are suitable platforms to load metallic NPs. We have succeeded in depositing Ag and Au particles with different particle sizes and shapes (spheres and stars), and polymeric coated Au NPs (Au/poly (tert-butyl acrylate)) and used them as SERS substrates (Figure 7C). Using these metal-loaded PA filter membranes, we have reported, for the first time, the use of Raman imaging combined with SERS spectroscopy not only to optimize the fabrication process of such SERS substrates but also to achieve low detection limits. In more detail, SERS imaging was used to adjust operational parameters, namely the amount of NPs in the filter membrane surface and sample preparation method, and to monitor the active SERS sites’ formation in distinct areas of the PA membrane. The Ag/PA filter membranes led to the higher SERS response in the CV analysis with detection limits of 10 fM for Aveiro Estuary water (Fateixa et al., 2018a). This fabrication process can be easily scaled up, and large amounts of contaminated water can be used for in field measurements.
2.4.2 Inkjet printing of SERS substrates
Several methods for fabricating SERS substrates rely on the adaptation of available technologies for routine applications. Among such methods, inkjet printing has also been explored to produce substrates for SERS detection of water pollutants (Yu and White, 2010; Dai et al., 2014; Restaino et al., 2017; Godoy et al., 2020; Martins et al., 2021). This technique has several advantages such as simplicity, low-cost and suitability for large-scale production of substrates. Furthermore, it offers the possibility to adjust the SERS sensor to target applications by employing ink formulations of distinct chemical composition (Yorov et al., 2016; Martins et al., 2021; Fernandes et al., 2022). Another important feature of inkjet printing is that allows the fabrication of SERS sensors on diverse materials with different substrate shapes (e.g., cellulose paper, glass surfaces or silicon wafers) (Hoppmann et al., 2013; Yang et al., 2015; Miccichè et al., 2018). In particular, paper is a flexible, biodegradable, lightweight and low-cost material, therefore is highly attractive to develop disposable analytical platforms. Furthermore, paper-based SERS substrates can be employed in point-of-use applications in conjunction with handheld Raman instruments and smartphones (Sun et al., 2019; Zeng et al., 2019). Thus, the inkjet printing of colloidal metal NPs on paper is gaining considerable attention as a widely accessible method for SERS substrates fabrication (Dai et al., 2014; Godoy et al., 2020; Martins et al., 2021; Fernandes et al., 2022).
Earlier reports on the use of cellulose substrates demonstrated their potential for SERS detection using both vegetable cellulose and bacterial cellulose (Marques et al., 2008). The latter has some advantages, because the cellulose can be used either as dry substrates (paper sheets) or aqueous sponges, both with high level of metal loaded cellulosic nanofibrils. However, the demonstration of the inkjet printing method for fabricating SERS paper-based substrates was only reported later by Yu and White. (2010). These authors have used a commercial inkjet printer to pattern Ag NPs with microscale precision on paper to obtain SERS substrates. In this way, they could detect rhodamine 6G (R6G) dissolved in water in a concentration as low as 10 fmol/µL. Later, White et al. have shown that inkjet printed paper substrates are also sensitive for the detection of antibiotics (e.g., sulfapyridine, ciprofloxacin) (Restaino et al., 2017). Many other reports followed that apply the inkjet printing method using chemical and morphological distinct colloids as the inks, such as Au nanospheres (Godoy et al., 2020), Au nanorods (Dai et al., 2014), and Ag anisotropic NPs (Yorov et al., 2016). In fact, it is not an overstatement to consider the chemistry of nanoinks for printing materials a research topic on its own, such as in the fabrication of paper-based SERS substrates, considering the many possibilities to adjust the sensing properties by applying chemical reasoning to the ink composition.
We have been particularly interested in ink formulations for the preparation of paper-based SERS substrates that offers control of the surface hydrophobicity (Martins et al., 2021). In fact, it has been demonstrated that hydrophobic paper can improve the sensitivity of SERS sensors by preventing the spreading of aqueous droplets employed in the analysis (Lee et al., 2018; Godoy et al., 2020). As consequence, this strategy can be used for increasing the amount of plasmonic NPs in a small area of the substrate by using the respective hydrosols and also allows the concentration of the aqueous analyte in the sensing region. Figure 8A shows paper-based SERS substrates obtained through inkjet printing of aqueous emulsions containing Ag NPs (Figure 8D), as the SERS active phase, and polystyrene (PS) beads that confers hydrophobic properties to the coated paper. Substrates with different levels of hydrophobicity were obtained by varying the weight percentage of PS in the ink formulations and then evaluated using several aqueous samples. The best SERS performance of the Ag/PS coated papers was observed for substrates showing the highest hydrophobicity. It should be noted that this approach is not limited to common office paper; other substrates can be coated either varying the emulsion composition or the metal colloids used in the inkjet printing process, as illustrated in Figures 8A–C. The applicability of these substrates using different aqueous matrices was evaluated, such as in the SERS detection of thiram (LOD 0.024 pm) in spiked samples of mineral water and apple juice (Figure 8E) (Martins et al., 2021).
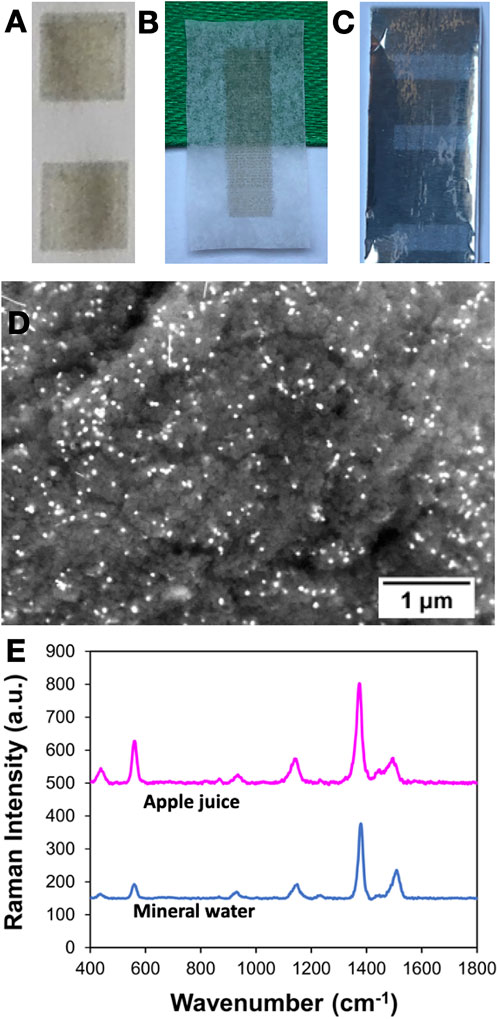
FIGURE 8. SERS substrates prepared by inkjet printing of metal colloids on different types of paper: (A) Ag/PS on office paper; (B) Ag on parchment paper (C) Au/PS on aluminium foil paper; (D) SEM image of office paper printed with Ag/PS ink, showing the metal NPs as white spots on a polymeric background.; (E) SERS spectra of thiram (10−6 M) spiked in apple juice and mineral water using Ag/PS as the substrate.
More recently, we have reported the use of dendrimer-stabilized Au:Ag nanoalloys of variable molar ratios for the inkjet printing of SERS substrates on office paper (Fernandes et al., 2022). Firstly, Au:Ag alloys were prepared in the presence of poly (amidoamine) dendrimer (PAMAM), resulting in nanoassemblies with plasmonic properties that depend on the chemical composition of the final materials (Fernandes et al., 2021; Fernandes et al., 2022). The dendrimer acts as a “molecular glue” for the clustering of Au and Ag nanocrystals, and also as a reducing and colloidal stabilizer. The dendrimer-stabilized Au:Ag nanoalloys were then used as colloidal inks for the inkjet printing of SERS substrates on paper. To further improve the SERS performance of the substrates, the paper surface was pre-treated with a hydrophobic coating of PS before the deposition of the nanoassemblies. The resulting substrates displayed good SERS sensitivity for the detection of the pesticide thiram in aqueous solutions, namely by using a portable Raman equipment.
2.4.3 Magneto-plasmonic substrates
Owing to their multifunctionality, there has been great interest in magneto-plasmonic systems where two distinct inorganic phases coexist in the same nanostructure, such as in a composite particle of a plasmonic metal and a magnetic iron oxide. These nanostructures can be produced by a variety of methods, leading to a wide assortment of magneto-plasmonic systems. In this regard, we have been interested in colloidal procedures because they can be easily implemented by adaptation of synthesis protocols developed for the single-component counterparts. Hence, two main synthetic approaches have been investigated: 1) the assembly of colloids of both phases and 2) the in situ chemical reduction of metal salts in the presence of the colloidal magnetic phase; the latter are typically ferrimagnetic Fe3O4 NPs. Although featuring distinct characteristics, both methods result in magneto-plasmonic nanosorbents whose basic configuration is observed in the electron microscopy images shown in Figure 9 (Lopes et al., 2016; Pinheiro et al., 2018). In Figures 9B, a detailed TEM image of Fe3O4-Au nanosorbents show Au spheroidal NPs (ca. 15 nm) assembled onto surface modified Fe3O4 NPs (ca. 80 nm); the Au NPs are responsible for SERS activity while Fe3O4 allows the separation of the multifunctional particles by applying an external magnetic gradient. The attachment of the Au NPs followed previous surface modification of the Fe3O4 particles with silica shells enriched in dithiocarbamate moieties by using a single-step route (Tavares et al., 2013). Hence, the modification of the nanosorbents’ surfaces is a practical consequence of the concept of soft acid (Au)-soft base (S ligand) affinity, which can be extended to the attachment of other noble metal NPs onto dithiocarbamate functionalized magnetites (Lopes et al., 2016).
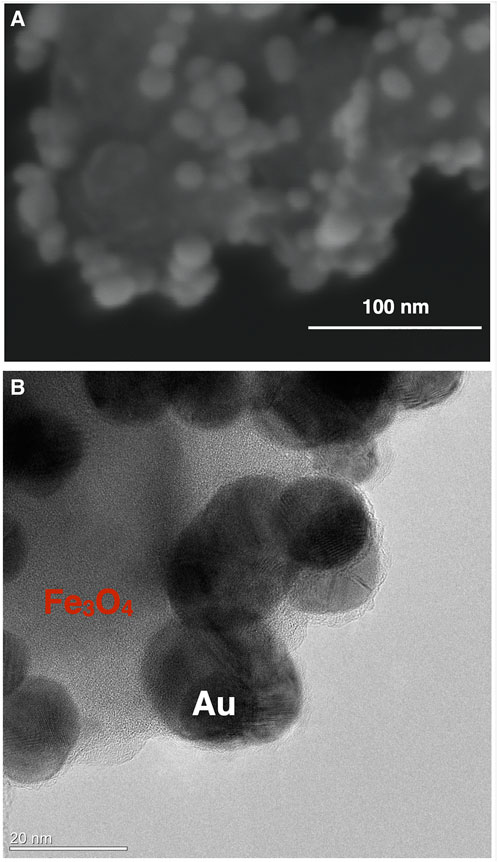
FIGURE 9. Electron microscopy images of multifunctional magneto-plasmonic nanosorbents: SEM (A) and TEM (B) images. The magnetic drivers are Fe3O4 particles decorated with SERS active Au nanoparticles.
There are few reports in the literature on the development of colloidal magneto-plasmonic nanomaterials applied to environmental applications, though their multifunctionality have been explored in other areas such as in bioapplications (Hao et al., 2010; Hou et al., 2017; Nguyen et al., 2018; Ovejero et al., 2018; Scaramuzza et al., 2019; Archana et al., 2021; Mukha et al., 2021). However, nanostructures like those shown in Figure 9 can be also explored as a new class of multifunctional nanosorbents for analytical purposes. While the magnetic iron oxide NPs confer the ability for magnetic separation from the aqueous medium, the plasmonic metal NPs allow the SERS monitoring of the target analyte. In this context, we have reported the application of Fe3O4-Au nanosorbents in the uptake and SERS detection of water contaminants of emergent concern, such as vestigial pharmaceutics (Pinheiro et al., 2019a; Pinheiro et al., 2019b). These multifunctional nanosorbents have great potential in analytical kits envisaging water quality monitoring protocols to be applied both in laboratory and on-site analysis. A great advantage in using magneto-plasmonic nanosorbents for trace analysis is the possibility of successive pre-concentration steps to facilitate the detection of the analyte. Nevertheless, research on this topic has been scarce and the potential of magneto-plasmonic materials is far from being well explored.
2.5 Environmental impact of nanomaterials
To overcome the global problem of water pollution, nanomaterials emerged as promising tools for monitoring water quality and for the remediation of wastewaters (Vikesland, 2018; Yaqoob et al., 2020; Davarazar et al., 2021). This is because some of their properties like nano size, large specific surface area, high reactivity, porosity, among others, can be explored for more effective processes. But, despite the beneficial potentials conferred by these properties for water and wastewater monitoring and remediation, these same properties may render them toxic to the biota (Nogueira et al., 2016; Monteiro et al., 2019; Costa et al., 2020; Ghadimi et al., 2020). Considering that it is inevitable that some of the nanoparticles used in water and wastewater treatments will be released into the aquatic ecosystem, concerns arise on their ecotoxicity to the environment. Therefore, targeting the safe use of nanomaterials in the remediation of water and wastewaters and promote the development of sustainable remediation nanotechnologies, in parallel to the study of its chemical efficiency (i.e., removal and/or monitoring of contaminants in water), it is important to address if the nanoparticles per se may constitute a risk to the environment. We have carried out this type of studies as exemplified by the case of study presented below.
We have characterized the ecotoxicity of three systems, mentioned previously in this manuscript, to the freshwater microalgae Raphidocelis subcapitata. The selected systems comprised colloidal gold nanostars (Au-NS) and biocomposite magnetic nanosorbents. The latter samples have distinct surface chemistry, one sample comprise coatings of k-carrageenan hybrid siliceous shells (Fe3O4@SiO2/SiCRG) and the other contains chitosan hybrid siliceous thin shells (Fe3O4@SiO2/SiCHT). In particular, the selected biocomposite sorbents are of particular interest because they have shown capacity to remove CEC from aqueous media as discussed above (Soares et al., 2016; Soares et al., 2017a). The R. subcapitata was used here as the model species because it is one of the recommended by REACH legislation to be tested when characterizing the ecotoxicity of new chemicals during the process for authorization to be placed in the market. Therefore, 72-h growth inhibition assays were performed by exposing R. subcapitata to several concentrations of Au-NS (125–200 mg/L), Fe3O4@SiO2/SiCRG (52.4–2000 mg/L) and Fe3O4@SiO2/SiCHT (52.4–2000 mg/L). Figure 10 apresents the results obtained in these assays. All the tested concentrations of the three selected nanomaterials induced a significant decrease in the yield and growth rate of R. subcapitata (p < 0.05). Regarding Au-NS, the concentration causing 20% (EC20, considered as the threshold for significant effects) and 50% (EC50) of reduction in the growth rate were 32.3 (95% confidence limits-95%CL: 8.84–7.4) and 294.8 (95%CL: 134.4–455.1 mg/L), respectively. Considering these ecotoxicity results, the safe use of Au NS depends on the amount employed for water quality monitoring applications. For example, in a study on magnetite decorated with Au-NS for SERS detection of tetracycline, only 0.250 mg/L of Au-NS were necessary (Pinheiro et al., 2019b), thus it is foreseen a safe use of these Au-NS in this context.
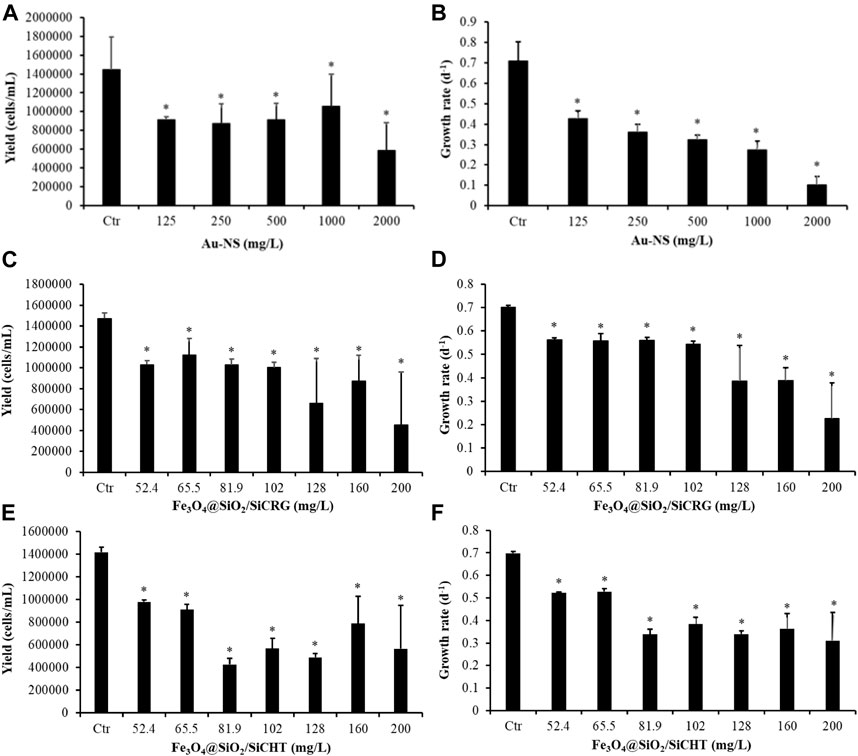
FIGURE 10. Average yield and growth rate of Raphidocelis subcapitata after being exposed, for 72 h, to several concentrations of gold nano-speres ((A) and (B) Au-NS) and of Fe3O4 nanoparticles coated with κ-carrageenan hybrid siliceous shells ((C) and (D) Fe3O4@SiO2/SiCRG) and Fe3O4 nanoparticles coated with chitosan hybrid siliceous thin shells ((E) and (F) Fe3O4@SiO2/SiCHT). * Indicates significant differences from the respective control (p < 0.05).
The two samples of magnetic biosorbents exhibited similar toxicity to the microalgae (Figure 10), with EC20 and EC50 of 84.8 (95% CL: 50.4–1119) mg/L and 164 (95% CL: 131-198) mg/L for Fe3O4@SiO2/SiCRG and of 29.7 (95% CL: 7.55–51.9) and 139 (93.8–183) mg/L for Fe3O4@SiO2/SiCHT, respectively. The ECx values computed for Fe3O4@SiO2/SiCRG were lower than the concentrations used by (Soares et al., 2016) to remove metoprolol from water. These authors used a concentration of 500 mg/L of Fe3O4@SiO2/SiCRG (by adding 10 mg of the sorbent to 2 ml of a metoprolol solution) to remove metoprolol from water and reported a removal higher than 30% after 2 h contact time. This removal efficiency occurred at concentrations of sorbent that may induce toxicity to freshwater biota, since it is higher than the concentration that impairs in 20 and 50% the growth rate of R. subcapitata. Given that microalgae are at the base of the trophic level, cascade effects at upper trophic levels may be expected to occur. A direct comparison of the ECx here computed for Fe3O4@SiO2/SiCHT could not be done with the amount needed to remove the non-polar compounds (Soares et al., 2017a) because different units were used in the two studies.
The preliminary results on the ecotoxicity of these three systems, suggest the potential for the safe use of Au-NS in the monitoring of water quality. For Fe3O4@SiO2/SiCRG it is expected that the amounts needed for an efficient remediation of contaminated waters will cause an ecological risk to microalgae, suggesting the need to remove these nanosorbents from the treated water before being released into the natural aquatic systems. In this regard, the ability for magnetic separation offered by these nanosorbents constitutes a good asset but that still require further research. In fact, it must be emphasized that these experiments were performed under controlled laboratory conditions and natural waters are much more complex systems than the aqueous media used in laboratory, which may alter the toxicity observed under laboratory conditions. Therefore, these types of studies should also be carried out under more realistic scenarios (e.g., test in media that are relevant/similar to the ones aimed to be remediated) to better understand how the nanosorbents will behave in natural freshwaters. Moreover, scientific evidence exists reporting how environmental characteristics may affect the fate and behaviour of nanoparticles, which, in turn, influences their toxicity to biota [e.g., (Ren et al., 2016; Arvidsson et al., 2020) and references therein]. Furthermore, the toxicity of these nanoparticles after being aged in the environmental matrices must be also explored since published works have reported a reduction in their ecotoxicity after ageing in water and soil matrices (Lehutso et al., 2020).
3 Conclusions and outlook
This review focused on recent research on nanomaterials of colloidal nature, to be used in this form or as starting components for other types of materials, envisaging applications that aim to prevent contamination of water sources and/or their treatment, mainly when CEC are involved. The revision of scientific work presented here was also framed by the research topics that our own group has developed on this subject. The several examples presented demonstrate the enormous potential of colloidal nanomaterials in applications aimed at water quality improvement. However, they are in distinct stages of potential application and, in certain cases, mainly regarded as interesting systems for acquiring new scientific knowledge. This is due not only to the nature of nanomaterials and their differentiated complexity, but also to the requirements that apply to each of the technologies in which nanomaterials are used. As an example, it should be noted that the scale at which nanomaterials are used differs between an application as nanosorbent in a water treatment plant and an analytical kit for laboratorial monitoring a target contaminant.
Although research on nanomaterials as addressed in this work is in different stages of maturation, it is possible to highlight some challenges and possible lines of investigation aimed at their solution. Nanosorbents have clear advantages over conventional materials, however aspects related to selectivity for CEC molecules and subsequent regeneration, if necessary, remain important challenges. The application of colloidal materials in photocatalysis has focused mainly on the use of the UV region of the electromagnetic spectrum, thus complementary and innovative strategies are needed aimed at extending the photocatalytic activity to the visible region. The study of chemical speciation of solutions submitted to photocatalytic treatment is of increasing importance, because total mineralization of pollutant molecules has not been always demonstrated. The use of nanomaterials as adsorbents and in photocatalysis, in a real context of water cleaning technology, must be preceded by environmental impact studies. The strategy of associating magnetic phases either to adsorbents or photocatalysts, is a possible solution to ensure the development of safe and more sustainable processes based on magnetic separation technologies. Another alternative strategy could be the immobilisation of nanomaterials in membranes using new processing technologies that take advantage of the properties of colloidal nanoparticles. With regard to the development of SERS sensors for CEC detection, in addition to the detection limit and selectivity for target analytes, there is a need for quantitative analytical data for specific scenarios. This aspect requires even more fundamental research that integrates, in addition to the aspects of synthesis of plasmonic nanomaterials, the mechanisms associated to their interaction with the analyte molecules, namely by taking into account the nanoscale characterization of the substrate, which impacts on the uniformity and reproducibility of the SERS signal.
In addition, there is a need for pilot projects aimed at water improvement applications for the most promising colloidal nanomaterials, in a realistic context, by selecting specific situations in which these materials can be part of the solution, in whole or in part. This type of approach also requires further ecotoxicity studies, also under realistic conditions, that allow the safe use of nanomaterials for the specific application for which they are intended and, in the required quantities. Finally, it should be noted that the unique properties observed in some of the nanomaterials reviewed here, can also be explored in other research contexts and innovative applications.
Author contributions
All authors have contributed to the manuscript. TT designed and coordinated the project.
Funding
This work was financed by Portugal 2020 through European Regional Development Fund (ERDF) in the frame of CENTRO2020 in the scope of the project BIOMAG, CENTRO-01-0145-FEDER-181268 and in the scope of the project CICECO–Aveiro Institute of Materials, UIDB/50011/2020 & UIDP/50011/2020 & LA/P/0006/2020, and CESAM-UIDB/50017/2020 + UIDP/50017/2020 + LA/P/0094/2020, financed by national funds through the FCT/MEC (PIDDAC). The work was also partially funded by Portugal 2020 through the European Regional Development Fund (ERDF) in the frame of Operational Competitiveness and Internationalization Program (POCI) in the scope of the project PROTEUS-POCI-01-0247-FEDER-017729. CM and MM thank the Fundação para a Ciência e Tecnologia (FCT) for the PhD grants PD/BD/150568/2020 and SFRH/BD/131433/2017, respectively. NG acknowledge the funding from the European Union’s Horizon 2020 research and innovation programme under the Marie Skłodowska-Curie grant agreement No 101065059. AE, NM, and SF acknowledge the costs of their research contracts (REF-078-88-ARH/2018; REF-069-88-ARH/2018) resulting from the FCT hiring funded by national funds (OE), through FCT, I. P, in the scope of the framework contract foreseen in 4, 5, and 6 of article 23 of the Decree-Law 57/2016, of 29 August, changed by the law 57/2017, of 19 July. CL acknowledges the funding by FCT through CEECIND/03739/2021.
Conflict of interest
The authors declare that the research was conducted in the absence of any commercial or financial relationships that could be construed as a potential conflict of interest.
Publisher’s note
All claims expressed in this article are solely those of the authors and do not necessarily represent those of their affiliated organizations, or those of the publisher, the editors and the reviewers. Any product that may be evaluated in this article, or claim that may be made by its manufacturer, is not guaranteed or endorsed by the publisher.
Abbreviations
AOX, adsorbable organic halides; CEC, contaminant of emerging concern; GNP, graphite-like nanoplatelets; GO, graphene oxide; ICPTES, 3-isocyanatopropyl triethoxysilane; LCP, liquid crystal polymer; MF, microfiltration; NF, nanofiltration; NP, nanoparticle; PA, polyamide; PANI, polyaniline; POM, polyoxometalate; PS, poly(styrene); PTFE, poly(tetrafluoroethylene); PVDF, poly(vinyl fluoride); rGO, reduced graphene oxide; RhB, Rhodamine B; SDG, Sustainable Development Goal; SEM, Scanning electron microscopy; SERS, surface enhanced Raman scattering; UN, United Nations; SWCNT, single-walled carbon nanotube; TCE, technology-critical element; TEM, Transmission electron microscopy; TEOS, tetraethylorthosilicate; UF, ultrafiltration.
References
Abbas, M., Parvatheeswara Rao, B., Reddy, V., and Kim, C. (2014). Fe3O4/TiO2 core/shell nanocubes: Single-batch surfactantless synthesis, characterization and efficient catalysts for methylene blue degradation. Ceram. Int. 40, 11177–11186. doi:10.1016/j.ceramint.2014.03.148
Abdel Maksoud, M. I. A., Elgarahy, A. M., Farrell, C., Al-Muhtaseb, A. H., Rooney, D. W., and Osman, A. I. (2020). Insight on water remediation application using magnetic nanomaterials and biosorbents. Coord. Chem. Rev. 403, 213096. doi:10.1016/j.ccr.2019.213096
Afonso, E. L., Carvalho, L., Fateixa, S., Amorim, C. O., Amaral, V. S., Vale, C., et al. (2019). Can contaminated waters or wastewater be alternative sources for technology-critical elements? The case of removal and recovery of lanthanides. J. Hazard. Mat. 380, 120845. doi:10.1016/J.JHAZMAT.2019.120845
Albrecht, M. G., and Creighton, J. A. (1977). Anomalously intense Raman spectra of pyridine at a silver electrode. J. Am. Chem. Soc. 99, 5215–5217. doi:10.1021/ja00457a071
Alipour Atmianlu, P., Badpa, R., Aghabalaei, V., and Baghdadi, M. (2021). A review on the various beds used for immobilization of nanoparticles: Overcoming the barrier to nanoparticle applications in water and wastewater treatment. J. Environ. Chem. Eng. 9, 106514. doi:10.1016/j.jece.2021.106514
Alivisatos, A. P. (1996). Semiconductor clusters, nanocrystals, and quantum dots. Science 271, 933–937. doi:10.1126/science.271.5251.933
Ankudze, B., Asare, B., Goffart, S., Koistinen, A., Nuutinen, T., Matikainen, A., et al. (2019). Hydraulically pressed silver nanowire-cotton fibers as an active platform for filtering and surface-enhanced Raman scattering detection of bacteria from fluid. Appl. Surf. Sci. 479, 663–668. doi:10.1016/j.apsusc.2019.02.067
Archana, V. N., Sam, S. A., Puthirath Balan, A., and Anantharaman, M. R. (2021). Magneto-optical properties of a magneto-plasmonic nanofluid based on superparamagnetic iron oxide and gold nanoparticles. J. Magn. Magn. Mat. 536, 168092. doi:10.1016/j.jmmm.2021.168092
Arvidsson, R., Hansen, S. F., and Baun, A. (2020). Influence of natural organic matter on the aquatic ecotoxicity of engineered nanoparticles: Recommendations for environmental risk assessment. NanoImpact 20, 100263. doi:10.1016/j.impact.2020.100263
Badsha, M. A. H., Khan, M., Wu, B., Kumar, A., and Lo, I. M. C. (2021). Role of surface functional groups of hydrogels in metal adsorption: From performance to mechanism. J. Hazard. Mat. 408, 124463. doi:10.1016/j.jhazmat.2020.124463
Barati, N., Husein, M. M., and Azaiez, J. (2021). Modifying ceramic membranes with in situ grown iron oxide nanoparticles and their use for oily water treatment. J. Memb. Sci. 617, 118641. doi:10.1016/j.memsci.2020.118641
Baskar, A. V., Bolan, N., Hoang, S. A., Sooriyakumar, P., Kumar, M., Singh, L., et al. (2022). Recovery, regeneration and sustainable management of spent adsorbents from wastewater treatment streams: A review. Sci. Total Environ. 822, 153555. doi:10.1016/j.scitotenv.2022.153555
Bawendi, M. G., Steigerwald, M. L., and Brus, L. E. (1990). The quantum mechanics of larger semiconductor clusters (“Quantum dots”). Annu. Rev. Phys. Chem. 41, 477–496. doi:10.1146/annurev.pc.41.100190.002401
Bodelón, G., and Pastoriza-Santos, I. (2020). Recent progress in surface-enhanced Raman scattering for the detection of chemical contaminants in water. Front. Chem. 8, 478. doi:10.3389/fchem.2020.00478
Boles, M. A., Ling, D., Hyeon, T., and Talapin, D. V. (2016). The surface science of nanocrystals. Nat. Mat. 15, 141–153. doi:10.1038/nmat4526
Brus, L. E. (1984). Electron-electron and electron-hole interactions in small semiconductor crystallites: The size dependence of the lowest excited electronic state. J. Chem. Phys. 80, 4403–4409. doi:10.1063/1.447218
Cardoso, C. E. D., Almeida, J. C., Lopes, C. B., Trindade, T., Vale, C., and Pereira, E. (2019). Recovery of rare earth elements by carbon-based nanomaterials—a review. Nanomater. (Basel). 9, 814. doi:10.3390/NANO9060814
Chen, F., Guan, Z., and Tang, A. (2018). Nanostructure and device architecture engineering for high-performance quantum-dot light-emitting diodes. J. Mat. Chem. C 6, 10958–10981. doi:10.1039/C8TC04028A
Cheng, Y., Arinze, E. S., Palmquist, N., and Thon, S. M. (2016). Advancing colloidal quantum dot photovoltaic technology. Nanophotonics 5, 31–54. doi:10.1515/nanoph-2016-0017
Cheng, Z., Xu, H., Wang, S., Liu, W., Li, Y., Jiang, L., et al. (2021). Effect of nanoparticles as a substitute for kinetic additives on the hydrate-based CO2 capture. Chem. Eng. J. 424, 130329. doi:10.1016/j.cej.2021.130329
Choe, H., Jeon, D., Lee, S. J., and Cho, J. (2021). Mixed or segregated: Toward efficient and stable mixed halide perovskite-based devices. ACS Omega 6, 24304–24315. doi:10.1021/acsomega.1c03714
Costa, B., Quintaneiro, C., Daniel-da-Silva, A. L., Trindade, T., Soares, A. M. V. M., and Lopes, I. (2020). An integrated approach to assess the sublethal effects of colloidal gold nanorods in tadpoles of Xenopus laevis. J. Hazard. Mat. 400, 123237. doi:10.1016/j.jhazmat.2020.123237
Crini, G., Lichtfouse, E., Wilson, L. D., and Morin-Crini, N. (2019). Conventional and non-conventional adsorbents for wastewater treatment. Environ. Chem. Lett. 17, 195–213. doi:10.1007/s10311-018-0786-8
Cui, Z., Li, W., Zeng, H., Tang, X., Zhang, J., Qin, S., et al. (2019). Fabricating PVDF hollow fiber microfiltration membrane with a tenon-connection structure via the thermally induced phase separation process to enhance strength and permeability. Eur. Polym. J. 111, 49–62. doi:10.1016/J.EURPOLYMJ.2018.12.009
Dai, Z., Xiao, X., Wu, W., Liao, L., Mei, F., Yu, X., et al. (2014). Side-to-side alignment of gold nanorods with polarization-free characteristic for highly reproducible surface enhanced Raman scattering. Appl. Phys. Lett. 105. , No 211902. doi:10.1063/1.4902812
Daniel-da-Silva, A. L., and Trindade, T. (2021). Surface chemistry of colloidal nanocrystals. Cambridge: Royal Society of Chemistry. doi:10.1039/9781788016568
Davarazar, M., Kamali, M., and Lopes, I. (2021). Engineered nanomaterials for (waste)water treatment - a scientometric assessment and sustainability aspects. NanoImpact 22, 100316. doi:10.1016/j.impact.2021.100316
Dutta, D. K., Borah, B. J., and Sarmah, P. P. (2015). Recent advances in metal nanoparticles stabilization into nanopores of montmorillonite and their catalytic applications for fine chemicals synthesis. Catal. Rev. 57, 257–305. doi:10.1080/01614940.2014.1003504
Ekimov, A. I., Efros, A. L., and Onushchenko, A. A. (1985). Quantum size effect in semiconductor microcrystals. Solid State Commun. 56, 921–924. doi:10.1016/S0038-1098(85)80025-9
Esteves, A. C. C., and Trindade, T. (2002). Synthetic studies on II/VI semiconductor quantum dots. Curr. Opin. Solid State Mat. Sci. 6, 347–353. doi:10.1016/S1359-0286(02)00079-7
Fateixa, S., Girão, A. V., Nogueira, H. I. S., and Trindade, T. (2011). Polymer based silver nanocomposites as versatile solid film and aqueous emulsion SERS substrates. J. Mat. Chem. 21, 15629–15636. doi:10.1039/c1jm12444g
Fateixa, S., Correia, M. R., and Trindade, T. (2013). Resizing of colloidal gold nanorods and morphological probing by SERS. J. Phys. Chem. C 117, 20343–20350. doi:10.1021/jp407216c
Fateixa, S., Soares, S. F., Daniel-Da-Silva, A. L., Nogueira, H. I. S., and Trindade, T. (2015). Silver-gelatine bionanocomposites for qualitative detection of a pesticide by SERS. Analyst 140, 1693–1701. doi:10.1039/c4an02105c
Fateixa, S., Wilhelm, M., Nogueira, H. I. S., and Trindade, T. (2016). SERS and Raman imaging as a new tool to monitor dyeing on textile fibres. J. Raman Spectrosc. 47, 1239–1246. doi:10.1002/jrs.4947
Fateixa, S., Nogueira, H. I. S., and Trindade, T. (2018a). Surface-enhanced Raman scattering spectral imaging for the attomolar range detection of crystal violet in contaminated water. ACS Omega 3, 4331–4341. doi:10.1021/acsomega.7b01983
Fateixa, S., Raposo, M., Nogueira, H. I. S., and Trindade, T. (2018b). A general strategy to prepare SERS active filter membranes for extraction and detection of pesticides in water. Talanta 182, 558–566. doi:10.1016/j.talanta.2018.02.014
Fateixa, S., Pinheiro, P. C., Nogueira, H. I. S., and Trindade, T. (2019). Gold loaded textile fibres as substrates for SERS detection. J. Mol. Struct. 1185, 333–340. doi:10.1016/j.molstruc.2019.03.001
Feng, S., Zhong, Z., Wang, Y., Xing, W., and Drioli, E. (2018). Progress and perspectives in PTFE membrane: Preparation, modification, and applications. J. Memb. Sci. 549, 332–349. doi:10.1016/J.MEMSCI.2017.12.032
Fernandes, T., Soares, S., Trindade, T., and Daniel-da-Silva, A. (2017). Magnetic hybrid nanosorbents for the uptake of paraquat from water. Nanomaterials 7, 68. doi:10.3390/nano7030068
Fernandes, T., Fateixa, S., Ferro, M., Nogueira, H. I. S., Daniel-da-Silva, A. L., and Trindade, T. (2021). Colloidal dendritic nanostructures of gold and silver for SERS analysis of water pollutants. J. Mol. Liq. 337, 116608. doi:10.1016/j.molliq.2021.116608
Fernandes, T., Martins, N. C. T., Fateixa, S., Nogueira, H. I. S., Daniel-da-Silva, A. L., and Trindade, T. (2022). Dendrimer stabilized nanoalloys for inkjet printing of surface-enhanced Raman scattering substrates. J. Colloid Interface Sci. 612, 342–354. doi:10.1016/j.jcis.2021.12.167
Fleischmann, M., Hendra, P. J., and McQuillan, A. J. (1974). Raman spectra of pyridine adsorbed at a silver electrode. Chem. Phys. Lett. 26, 163–166. doi:10.1016/0009-2614(74)85388-1
Frey, N. A., Peng, S., Cheng, K., and Sun, S. (2009). Magnetic nanoparticles: Synthesis, functionalization, and applications in bioimaging and magnetic energy storage. Chem. Soc. Rev. 38, 2532–2542. doi:10.1039/b815548h
Gao, S., Zhang, Z., and He, L. (2016). Filter-based surface-enhanced Raman spectroscopy for rapid and sensitive detection of the fungicide ferbam in water. Int. J. Environ. Anal. Chem. 96, 1495–1506. doi:10.1080/03067319.2016.1272677
Ghadimi, M., Zangenehtabar, S., and Homaeigohar, S. (2020). An overview of the water remediation potential of nanomaterials and their ecotoxicological impacts. WaterSwitzerl. 12, 1150. doi:10.3390/W12041150
Godoy, N. V., García-Lojo, D., Sigoli, F. A., Pérez-Juste, J., Pastoriza-Santos, I., and Mazali, I. O. (2020). Ultrasensitive inkjet-printed based SERS sensor combining a high-performance gold nanosphere ink and hydrophobic paper. Sensors Actuators B Chem. 320, 128412. doi:10.1016/j.snb.2020.128412
Gómez-Pastora, J., Dominguez, S., Bringas, E., Rivero, M. J., Ortiz, I., and Dionysiou, D. D. (2017). Review and perspectives on the use of magnetic nanophotocatalysts (MNPCs) in water treatment. Chem. Eng. J. 310, 407–427. doi:10.1016/j.cej.2016.04.140
Gondal, M. A., Sadullah, M. S., Qahtan, T. F., Dastageer, M. A., Baig, U., and McKinley, G. H. (2017). Fabrication and wettability study of WO3 coated photocatalytic membrane for oil-water separation: A comparative study with ZnO coated membrane. Sci. Rep. 7, 1686–1710. doi:10.1038/s41598-017-01959-y
Granadeiro, C., Cruz, S., Goncalves, G., Marques, P., Costa, P., Ferreira, R., et al. (2012). Photoluminescent bimetallic-3-hydroxypicolinate/graphene oxide nanocomposite. RSC Adv. 2, 9443–9447. doi:10.1039/C2RA21388E
Grzelczak, M., Pérez-Juste, J., Mulvaney, P., and Liz-Marzán, L. M. (2008). Shape control in gold nanoparticle synthesis. Chem. Soc. Rev. 37, 1783–1791. doi:10.1039/b711490g
Guerrero-Martínez, A., Barbosa, S., Pastoriza-Santos, I., and Liz-Marzán, L. M. (2011). Nanostars shine bright for you. Colloidal synthesis, properties and applications of branched metallic nanoparticles. Curr. Opin. Colloid Interface Sci. 16, 118–127. doi:10.1016/j.cocis.2010.12.007
Guo, H., Xing, B., and He, L. (2016). Development of a filter-based method for detecting silver nanoparticles and their heteroaggregation in aqueous environments by surface-enhanced Raman spectroscopy. Environ. Pollut. 211, 198–205. doi:10.1016/j.envpol.2015.12.049
Guo, H., Hamlet, L. C., He, L., and Xing, B. (2019). A field-deployable surface-enhanced Raman scattering (SERS) method for sensitive analysis of silver nanoparticles in environmental waters. Sci. Total Environ. 653, 1034–1041. doi:10.1016/j.scitotenv.2018.10.435
Hao, R., Xing, R., Xu, Z., Hou, Y., Gao, S., and Sun, S. (2010). Synthesis, functionalization, and biomedical applications of multifunctional magnetic nanoparticles. Adv. Mat. 22, 2729–2742. doi:10.1002/adma.201000260
Homocianu, M., and Pascariu, P. (2022). High-performance photocatalytic membranes for water purification in relation to environmental and operational parameters. J. Environ. Manage. 311, 114817. doi:10.1016/j.jenvman.2022.114817
Hoppmann, E. P., Yu, W. W., and White, I. M. (2013). Highly sensitive and flexible inkjet printed SERS sensors on paper. Methods 63, 219–224. doi:10.1016/j.ymeth.2013.07.010
Horovitz, I., Avisar, D., Baker, M. A., Grilli, R., Lozzi, L., Di Camillo, D., et al. (2016). Carbamazepine degradation using a N-doped TiO2 coated photocatalytic membrane reactor: Influence of physical parameters. J. Hazard. Mat. 310, 98–107. doi:10.1016/j.jhazmat.2016.02.008
Hou, X., Wang, X., Liu, R., Zhang, H., Liu, X., and Zhang, Y. (2017). Facile synthesis of multifunctional Fe3O4@SiO2@Au magneto-plasmonic nanoparticles for MR/CT dual imaging and photothermal therapy. RSC Adv. 7, 18844–18850. doi:10.1039/c7ra00925a
Huang, X., Tian, F., Chen, G., Wang, F., Weng, R., and Xi, B. (2021). Preparation and characterization of regenerated cellulose membrane blended with ZrO2 nanoparticles. Membr. (Basel). 12, 42. doi:10.3390/MEMBRANES12010042
Iglesias, O., Rivero, M. J., Urtiaga, A. M., and Ortiz, I. (2016). Membrane-based photocatalytic systems for process intensification. Chem. Eng. J. 305, 136–148. doi:10.1016/j.cej.2016.01.047
Jeanmaire, D. L., and Van Duyne, R. P. (1977). Surface Raman spectroelectrochemistry. J. Electroanal. Chem. Interfacial Electrochem. 84, 1–20. doi:10.1016/S0022-0728(77)80224-6
Jeevanandam, J., Kiew, S. F., Boakye-Ansah, S., Lau, S. Y., Barhoum, A., Danquah, M. K., et al. (2022). Green approaches for the synthesis of metal and metal oxide nanoparticles using microbial and plant extracts. Nanoscale 14, 2534–2571. doi:10.1039/d1nr08144f
Jensen, L., Aikens, C. M., and Schatz, G. C. (2008). Electronic structure methods for studying surface-enhanced Raman scattering. Chem. Soc. Rev. 37, 1061–1073. doi:10.1039/b706023h
Jiao, S., Liu, Y., Wang, S., Wang, S., Ma, F., Yuan, H., et al. (2022). Face-to-Face assembly of Ag nanoplates on filter papers for pesticide detection by surface-enhanced Raman spectroscopy. Nanomaterials 12, 1398. doi:10.3390/nano12091398
Joseph, A., Sajith, V., and Sarathchandran, C. (2021). Graphene: The magic material. Handb. Carbon-Based Nanomater., 517–549. doi:10.1016/B978-0-12-821996-6.00001-4
Jue, M. L., Buchsbaum, S. F., Chen, C., Park, S. J., Meshot, E. R., Wu, K. J. J., et al. (2020). Ultra-permeable single-walled carbon nanotube membranes with exceptional performance at scale. Adv. Sci. (Weinh). 7, 2001670. doi:10.1002/ADVS.202001670
Jun, Y., Choi, J., and Cheon, J. (2006). Shape control of semiconductor and metal oxide nanocrystals through nonhydrolytic colloidal routes. Angew. Chem. Int. Ed. 45, 3414–3439. doi:10.1002/anie.200503821
Kallumottakkal, M., Hussein, M. I., and Iqbal, M. Z. (2021). Recent progress of 2D nanomaterials for application on microwave absorption: A comprehensive study. Front. Mat. 8, 34. doi:10.3389/fmats.2021.633079
Kamat, P. V. (2008). Quantum dot solar cells. Semiconductor nanocrystals as light harvesters. J. Phys. Chem. C 112, 18737–18753. doi:10.1021/jp806791s
Khan, G. A., Demirtaş, O. Ö., Bek, A., Bhatti, A. S., and Ahmed, W. (2022). Facile fabrication of Au-Ag alloy nanoparticles on filter paper: Application in SERS based swab detection and multiplexing. Vib. Spectrosc. 120, 103359. doi:10.1016/j.vibspec.2022.103359
Kubacka, A., Fernández-García, M., and Colón, G. (2012). Advanced nanoarchitectures for solar photocatalytic applications. Chem. Rev. 112, 1555–1614. doi:10.1021/cr100454n
Kumar, P., Kumar, S., Bhardwaj, N. K., and Choudhary, A. K. (2011). Optimization of process parameters for the photocatalytic treatment of paper mill wastewater. Environ. Eng. Manag. J. 10, 595–601. doi:10.30638/eemj.2011.082
Kumar, S., Nehra, M., Deep, A., Kedia, D., Dilbaghi, N., and Kim, K. H. (2017). Quantum-sized nanomaterials for solar cell applications. Renew. Sustain. Energy Rev. 73, 821–839. doi:10.1016/j.rser.2017.01.172
Kumar, R., Mangalapuri, R., Ahmadi, M. H., Vo, D.-V. N., Solanki, R., and Kumar, P. (2020). The role of nanotechnology on post-combustion CO2 absorption in process industries. Int. J. Low-Carbon Technol. 15, 361–367. doi:10.1093/ijlct/ctaa002
Kumari, S., Tyagi, M., and Jagadevan, S. (2019). Mechanistic removal of environmental contaminants using biogenic nano-materials. Int. J. Environ. Sci. Technol. 16, 7591–7606. doi:10.1007/s13762-019-02468-3
Kumari, P., Singh, P., Singhal, A., and Alka, (2020). Cyclodextrin-based nanostructured materials for sustainable water remediation applications. Environ. Sci. Pollut. Res. 27, 32432–32448. doi:10.1007/s11356-020-09519-0
Kusworo, T., Nugraheni, R. E., and Aryanti, N. (2021). The effect of membrane modification using TiO 2 , ZnO, and GO nanoparticles: Challenges and future direction in wastewater treatment. IOP Conf. Ser. Mat. Sci. Eng. 1053, 012135. doi:10.1088/1757-899x/1053/1/012135
Lakhotia, S. R., Mukhopadhyay, M., and Kumari, P. (2018). Surface-modified nanocomposite membranes. Sep. Purif. Rev. 47, 288–305. doi:10.1080/15422119.2017.1386681
Lee, C. H., Tian, L., and Singamaneni, S. (2010). Paper-based SERS swab for rapid trace detection on real-world surfaces. ACS Appl. Mat. Interfaces 2, 3429–3435. doi:10.1021/am1009875
Lee, M., Oh, K., Choi, H.-K., Lee, S. G., Youn, H. J., Lee, H. L., et al. (2018). Subnanomolar sensitivity of filter paper-based SERS sensor for pesticide detection by hydrophobicity change of paper surface. ACS Sens. 3, 151–159. doi:10.1021/acssensors.7b00782
Lehutso, R. F., Tancu, Y., Maity, A., and Thwala, M. (2020). Aquatic toxicity of transformed and product-released engineered nanomaterials: An overview of the current state of knowledge. Process Saf. Environ. Prot. 138, 39–56. doi:10.1016/j.psep.2020.03.002
Leslie-Pelecky, D. L., and Rieke, R. D. (1996). Magnetic properties of nanostructured materials. Chem. Mat. 8, 1770–1783. doi:10.1021/cm960077f
Li, H., Zhang, Y., Wang, S., Wu, Q., and Liu, C. (2009). Study on nanomagnets supported TiO2 photocatalysts prepared by a sol-gel process in reverse microemulsion combining with solvent-thermal technique. J. Hazard. Mat. 169, 1045–1053. doi:10.1016/j.jhazmat.2009.04.040
Li, Z., Deen, M., Kumar, S., and Selvaganapathy, P. (2014). Raman spectroscopy for in-line water quality monitoring—instrumentation and potential. Sensors 14, 17275–17303. doi:10.3390/s140917275
Li, R., Ren, Y., Zhao, P., Wang, J., Liu, J., and Zhang, Y. (2019). Graphitic carbon nitride (g-C3N4) nanosheets functionalized composite membrane with self-cleaning and antibacterial performance. J. Hazard. Mat. 365, 606–614. doi:10.1016/J.JHAZMAT.2018.11.033
Li, R., Cui, L., Chen, M., and Huang, Y. (2021). Nanomaterials for airborne virus inactivation: A short review. Aerosol Sci. Eng. 5, 1–11. doi:10.1007/s41810-020-00080-4
Lim, B., and Xia, Y. (2011). Metal nanocrystals with highly branched morphologies. Angew. Chem. Int. Ed. 50, 76–85. doi:10.1002/anie.201002024
Lin, C. A. J., Liedl, T., Sperling, R. A., Fernández-Argüelles, M. T., Costa-Fernández, J. M., Pereiro, R., et al. (2007). Bioanalytics and biolabeling with semiconductor nanoparticles (quantum dots). J. Mat. Chem. 17, 1343–1346. doi:10.1039/b618902d
Liu, Z., McClements, D. J., Shi, A., Zhi, L., Tian, Y., Jiao, B., et al. (2022). Janus particles: A review of their applications in food and medicine. Crit. Rev. Food Sci. Nutr., 1–12. doi:10.1080/10408398.2022.2067831
Loeb, S. K., Alvarez, P. J. J., Brame, J. A., Cates, E. L., Choi, W., Crittenden, J., et al. (2019). The technology Horizon for photocatalytic water treatment: Sunrise or sunset? Environ. Sci. Technol. 53, 2937–2947. doi:10.1021/acs.est.8b05041
Lofrano, G., Carotenuto, M., Libralato, G., Domingos, R. F., Markus, A., Dini, L., et al. (2016). Polymer functionalized nanocomposites for metals removal from water and wastewater: An overview. Water Res. 92, 22–37. doi:10.1016/j.watres.2016.01.033
Lopes, C. B., Coimbra, J., Otero, M., Pereira, E., Duarte, A. C., Lin, Z., et al. (2008). Uptake of Hg2+ from aqueous solutions by microporous titano- and zircono-silicates. Quim. Nova 31, 321–325. doi:10.1590/S0100-40422008000200025
Lopes, J. L., Marques, K. L., Girão, A. V., Pereira, E., and Trindade, T. (2016). Functionalized magnetite particles for adsorption of colloidal noble metal nanoparticles. J. Colloid Interface Sci. 475, 96–103. doi:10.1016/j.jcis.2016.04.046
Lopes, J. L., Martins, M. J., Nogueira, H. I. S., Estrada, A. C., and Trindade, T. (2021). Carbon-based heterogeneous photocatalysts for water cleaning technologies: A review. Environ. Chem. Lett. 19, 643–668. doi:10.1007/s10311-020-01092-9
López-Quintela, M. A., and Rivas, J. (1996). Nanoscale magnetic particles: Synthesis, structure and dynamics. Curr. Opin. Colloid Interface Sci. 1, 806–819. doi:10.1016/s1359-0294(96)80085-9
Luo, H., Yan, M., Wu, Y., Lin, X., and Yan, Y. (2021). Facile synthesis of PVDF photocatalytic membrane based on NCQDs/BiOBr/TiO2 heterojunction for effective removal of tetracycline. Mater. Sci. Eng. B 265, 114996. doi:10.1016/j.mseb.2020.114996
Manna, L., Scher, Erik C., and Alivisatos, A. P. (2000). Synthesis of soluble and processable rod-arrow-teardrop-and tetrapod-shaped CdSe nanocrystals. J. Am. Chem. Soc. 122:12700–12706. doi:10.1021/JA003055+
Marques, P. A. A. P., Nogueira, H. I. S., Pinto, R. J. B., Neto, C. P., and Trindade, T. (2008). Silver-bacterial cellulosic sponges as active SERS substrates. J. Raman Spectrosc. 39, 439–443. doi:10.1002/jrs.1853
Martins, P. M., Miranda, R., Marques, J., Tavares, C. J., Botelho, G., and Lanceros-Mendez, S. (2016). Comparative efficiency of TiO2 nanoparticles in suspension vs. immobilization into P(VDF-TrFE) porous membranes. RSC Adv. 6, 12708–12716. doi:10.1039/c5ra25385c
Martins, N. C. T., Fateixa, S., Fernandes, T., Nogueira, H. I. S., and Trindade, T. (2021). Inkjet printing of Ag and polystyrene nanoparticle emulsions for the one-step fabrication of hydrophobic paper-based surface-enhanced Raman scattering substrates. ACS Appl. Nano Mat. 4, 4484–4495. doi:10.1021/acsanm.1c00112
Mehn, D., Morasso, C., Vanna, R., Bedoni, M., Prosperi, D., and Gramatica, F. (2013). Immobilised gold nanostars in a paper-based test system for surface-enhanced Raman spectroscopy. Vib. Spectrosc. 68, 45–50. doi:10.1016/j.vibspec.2013.05.010
Miccichè, C., Arrabito, G., Amato, F., Buscarino, G., Agnello, S., and Pignataro, B. (2018). Inkjet printing Ag nanoparticles for SERS hot spots. Anal. Methods 10, 3215–3223. Available at: http://xlink.rsc.org/?DOI=C8AY00624E.
Monteiro, C., Daniel-da-Silva, A. L., Venâncio, C., Soares, S. F., Soares, A. M. V. M., Trindade, T., et al. (2019). Effects of long-term exposure to colloidal gold nanorods on freshwater microalgae. Sci. Total Environ. 682, 70–79. doi:10.1016/j.scitotenv.2019.05.052
Moram, S. S. B., Byram, C., Shibu, S. N., Chilukamarri, B. M., and Soma, V. R. (2018). Ag/Au nanoparticle-loaded paper-based versatile surface-enhanced Raman spectroscopy substrates for multiple explosives detection. ACS Omega 3, 8190–8201. doi:10.1021/acsomega.8b01318
Mukha, I., Chepurna, O., Vityuk, N., Khodko, A., Storozhuk, L., Dzhagan, V., et al. (2021). Multifunctional magneto-plasmonic Fe3O4/Au nanocomposites: Approaching magnetophoretically-enhanced photothermal therapy. Nanomaterials 11, 1113. doi:10.3390/nano11051113
Nasrollahzadeh, M., Sajjadi, M., Iravani, S., and Varma, R. S. (2021). Starch, cellulose, pectin, gum, alginate, chitin and chitosan derived (nano)materials for sustainable water treatment: A review. Carbohydr. Polym. 251, 116986. doi:10.1016/j.carbpol.2020.116986
Nguyen, T. T., Mammeri, F., and Ammar, S. (2018). Iron oxide and gold based magneto-plasmonic nanostructures for medical applications: A review. Nanomaterials 8, 149. doi:10.3390/nano8030149
Nogueira, V. I. J. O., Gavina, A., Bouguerra, S., Andreani, T., Lopes, I., Rocha-Santos, T., et al. (2016). “Ecotoxicity and toxicity of nanomaterials with potential for wastewater treatment applications,” in Applying Nanotechnology for environmental sustainability, 294–329. doi:10.4018/978-1-5225-0585-3.ch013
Ong, T. T. X., Blanch, E. W., and Jones, O. A. H. (2020). Surface Enhanced Raman Spectroscopy in environmental analysis, monitoring and assessment. Sci. Total Environ. 720, 137601. doi:10.1016/j.scitotenv.2020.137601
Ovejero, J. G., Yoon, S. J., Li, J., Mayoral, A., Gao, X., O’Donnell, M., et al. (2018). Synthesis of hybrid magneto-plasmonic nanoparticles with potential use in photoacoustic detection of circulating tumor cells. Microchim. Acta 185, 130–138. doi:10.1007/s00604-017-2637-x
Peng, Z. A., and Peng, X. (2001). Formation of high-quality CdTe, CdSe, and CdS nanocrystals using CdO as precursor. J. Am. Chem. Soc. 123, 183–184. doi:10.1021/ja003633m
Pinheiro, P. C., Daniel-da-Silva, A. L., Nogueira, H. I. S., and Trindade, T. (2018). Functionalized inorganic nanoparticles for magnetic separation and SERS detection of water pollutants. Eur. J. Inorg. Chem. 2018, 3440. doi:10.1002/ejic.201800808
Pinheiro, P. C., Fateixa, S., Daniel-da-Silva, A. L., and Trindade, T. (2019a). An integrated approach for trace detection of pollutants in water using polyelectrolyte functionalized magneto-plasmonic nanosorbents. Sci. Rep. 9, 19647. doi:10.1038/s41598-019-56168-6
Pinheiro, P. C., Fateixa, S., Nogueira, H. I. S., and Trindade, T. (2019b). Magnetite-supported gold nanostars for the uptake and SERS detection of tetracycline. Nanomaterials 9, 31. doi:10.3390/nano9010031
Pinto, J., Lopes, C. B., Henriques, B., Couto, A. F., Ferreira, N., Carvalho, L., et al. (2021). Platinum-group elements sorption by living macroalgae under different contamination scenarios. J. Environ. Chem. Eng. 9, 105100. doi:10.1016/J.JECE.2021.105100
Polshettiwar, V., and Varma, R. S. (2010). Green chemistry by nano-catalysis. Green Chem. 12, 743–775. doi:10.1039/b921171c
Presumido, P. H., Santos, L. F. dos, Neuparth, T., Santos, M. M., Feliciano, M., Primo, A., et al. (2021). A novel ceramic tubular membrane coated with a continuous graphene-TiO2 nanocomposite thin-film for CECs mitigation. Chem. Eng. J. 430, 132639. doi:10.1016/J.CEJ.2021.132639
Rafiei-Sarmazdeh, Z., Zahedi-Dizaji, S. M., and Kang, A. K. (2019). “Two-Dimensional Nanomaterials,” in Nanostructures. Editors S. Ameen, M. S. Akhtar, and H. Shin (IntechOpen). doi:10.5772/intechopen.85263
Rao, N., Singh, R., and Bashambu, L. (2021). Carbon-based nanomaterials: Synthesis and prospective applications. Mater. Today Proc. 44, 608–614. doi:10.1016/J.MATPR.2020.10.593
Ray, S. S., and Bandyopadhyay, J. (2021). Nanotechnology-enabled biomedical engineering: Current trends, future scopes, and perspectives. Nanotechnol. Rev. 10, 728–743. doi:10.1515/ntrev-2021-0052
Ren, C., Hu, X., and Zhou, Q. (2016). Influence of environmental factors on nanotoxicity and knowledge gaps thereof. NanoImpact 2, 82–92. doi:10.1016/j.impact.2016.07.002
Restaino, S. M., Berger, A., and White, I. M. (2017). Inkjet-printed paper fluidic devices for onsite detection of antibiotics using surface-enhanced Raman spectroscopy. Methods Mol. Biol. 2, 525–540. doi:10.1007/978-1-4939-6911-1_33
Scaramuzza, S., Polizzi, S., and Amendola, V. (2019). Magnetic tuning of SERS hot spots in polymer-coated magnetic-plasmonic iron-silver nanoparticles. Nanoscale Adv. 1, 2681–2689. doi:10.1039/c9na00143c
Schmid, G. (1992). Large clusters and colloids. Metals in the embryonic state. Chem. Rev. 92, 1709–1727. doi:10.1021/cr00016a002
Sha, X., Han, S. Q., Gao, W., Zhao, H., Li, N., Zhang, C., et al. (2020). A rapid detection method for on-site screening of estazolam in beverages with Au@Ag core-shell nanoparticles paper-based SERS substrate. Anal. Sci. 36, 667–674. doi:10.2116/ANALSCI.19P361
Shen, L., Huang, Z., Liu, Y., Li, R., Xu, Y., Jakaj, G., et al. (2020). Polymeric membranes incorporated with ZnO nanoparticles for membrane fouling mitigation: A brief review. Front. Chem. 8, 224. doi:10.3389/fchem.2020.00224
Shi, Y. E., Li, L., Yang, M., Jiang, X., Zhao, Q., and Zhan, J. (2014). A disordered silver nanowires membrane for extraction and surface-enhanced Raman spectroscopy detection. Analyst 139, 2525–2530. doi:10.1039/c4an00163j
Soares, S. F., Simões, T. R., António, M., Trindade, T., and Daniel-da-Silva, A. L. (2016). Hybrid nanoadsorbents for the magnetically assisted removal of metoprolol from water. Chem. Eng. J. 302, 560–569. doi:10.1016/j.cej.2016.05.079
Soares, S. F., Rodrigues, M. I., Trindade, T., and Daniel-da-Silva, A. L. (2017a). Chitosan-silica hybrid nanosorbents for oil removal from water. Colloids Surfaces A Physicochem. Eng. Aspects 532, 305–313. doi:10.1016/j.colsurfa.2017.04.076
Soares, S. F., Simões, T. R., Trindade, T., and Daniel-da-Silva, A. L. (2017b). Highly efficient removal of dye from water using magnetic carrageenan/silica hybrid nano-adsorbents. Water Air Soil Pollut. 228, 87. doi:10.1007/s11270-017-3281-0
Soares, S. F., Daniel-da-Silva, A. L., and Trindade, T. (2019a). Trimethyl chitosan/siloxane-hybrid coated Fe3O4 nanoparticles for the uptake of sulfamethoxazole from water. Molecules 24, 1958. doi:10.3390/molecules24101958
Soares, S. F., Fernandes, T., Sacramento, M., Trindade, T., and Daniel-da-Silva, A. L. (2019b). Magnetic quaternary chitosan hybrid nanoparticles for the efficient uptake of diclofenac from water. Carbohydr. Polym. 203, 35–44. doi:10.1016/j.carbpol.2018.09.030
Soares, S. F., Rocha, M. J., Ferro, M., Amorim, C. O., Amaral, J. S., Trindade, T., et al. (2019c). Magnetic nanosorbents with siliceous hybrid shells of alginic acid and carrageenan for removal of ciprofloxacin. Int. J. Biol. Macromol. 139, 827–841. doi:10.1016/j.ijbiomac.2019.08.030
Soares, S. F., Fernandes, T., Trindade, T., and Daniel-da-Silva, A. L. (2020). Recent advances on magnetic biosorbents and their applications for water treatment. Environ. Chem. Lett. 18, 151–164. doi:10.1007/s10311-019-00931-8
Soares, S. F., Amorim, C. O., Amaral, J. S., Trindade, T., and Daniel-Da-Silva, A. L. (2021a). On the efficient removal, regeneration and reuse of quaternary chitosan magnetite nanosorbents for glyphosate herbicide in water. J. Environ. Chem. Eng. 9, 105189. doi:10.1016/j.jece.2021.105189
Soares, S. F., Trindade, T., and Daniel-Da-Silva, A. L. (2021b). Enhanced removal of non-steroidal inflammatory drugs from water by quaternary chitosan-based magnetic nanosorbents. Coatings 11, 964. doi:10.3390/coatings11080964
Soares, S. F., Nogueira, J., Trindade, T., and Daniel-da-Silva, A. L. (2022). Towards efficient ciprofloxacin adsorption using magnetic hybrid nanoparticles prepared with κ-ι-and λ-carrageenan. J. Nanostructure Chem., 1–20. doi:10.1007/s40097-022-00498-x
Sokolova, V., and Epple, M. (2008). Inorganic nanoparticles as carriers of nucleic acids into cells. Angew. Chem. Int. Ed. 47, 1382–1395. doi:10.1002/anie.200703039
Steigerwald, M. L., and Brus, L. E. (1990). Semiconductor crystallites: A class of large molecules. Acc. Chem. Res. 23, 183–188. doi:10.1021/ar00174a003
Stiles, P. L., Dieringer, J. A., Shah, N. C., and Van Duyne, R. P. (2008). Surface-enhanced Raman spectroscopy. Annu. Rev. Anal. Chem. Palo. Alto. Calif. 1, 601–626. doi:10.1146/annurev.anchem.1.031207.112814
Sun, M., Li, B., Liu, X., Chen, J., Mu, T., Zhu, L., et al. (2019). Performance enhancement of paper-based SERS chips by shell-isolated nanoparticle-enhanced Raman spectroscopy. J. Mat. Sci. Technol. 35, 2207–2212. doi:10.1016/j.jmst.2019.05.055
Sun, J., Zhang, Z., Liu, C., Dai, X., Zhou, W., Jiang, K., et al. (2021). Continuous in situ portable SERS analysis of pollutants in water and air by a highly sensitive gold nanoparticle-decorated PVDF substrate. Anal. Bioanal. Chem. 413, 5469–5482. doi:10.1007/s00216-021-03531-0
Tang, H., Zhu, C., Meng, G., and Wu, N. (2018). Review—surface-enhanced Raman scattering sensors for food safety and environmental monitoring. J. Electrochem. Soc. 165, B3098–B3118. doi:10.1149/2.0161808jes
Tao, A. R., Habas, S., and Yang, P. (2008). Shape control of colloidal metal nanocrystals. Small 4, 310–325. doi:10.1002/smll.200701295
Tavares, D. S., Daniel-Da-Silva, A. L., Lopes, C. B., Silva, N. J. O., Amaral, V. S., Rocha, J., et al. (2013). Efficient sorbents based on magnetite coated with siliceous hybrid shells for removal of mercury ions. J. Mat. Chem. A 1, 8134–8143. doi:10.1039/c3ta10914c
Tavares, D. S., Lopes, C. B., Almeida, J. C., Vale, C., Pereira, E., and Trindade, T. (2020). Spinel-type ferrite nanoparticles for removal of arsenic(V) from water. Environ. Sci. Pollut. Res. 27, 22523–22534. doi:10.1007/s11356-020-08673-9
Teitelboim, A., Meir, N., Kazes, M., and Oron, D. (2016). Colloidal double quantum dots. Acc. Chem. Res. 49, 902–910. doi:10.1021/acs.accounts.5b00554
Theron, J., Walker, J. A., and Cloete, T. E. (2008). Nanotechnology and water treatment: Applications and emerging opportunities. Crit. Rev. Microbiol. 34, 43–69. doi:10.1080/10408410701710442
Trindade, T., and Daniel da Silva, A. L. (2011). Nanocomposite particles for bio-applications: Materials and bio-interfaces. doi:10.4032/9789814267816
Trindade, T., and O’Brien, P. (1996). A single source approach to the synthesis of CdSe nanocrystallites. Adv. Mat. 8, 161–163. doi:10.1002/adma.19960080214
Trindade, T., O’Brien, P., and Pickett, N. L. (2001). Nanocrystalline semiconductors: Synthesis, properties, and perspectives. Chem. Mat. 13, 3843–3858. doi:10.1021/cm000843p
Ugurlu, M., and Karaoglu, M. H. (2009). Removal of AOX, total nitrogen and chlorinated lignin from bleached Kraft mill effluents by UV oxidation in the presence of hydrogen peroxide utilizing TiO2 as photocatalyst. Environ. Sci. Pollut. Res. 16, 265–273. doi:10.1007/s11356-008-0044-x
Valley, N., Greeneltch, N., Van Duyne, R. P., and Schatz, G. C. (2013). A look at the origin and magnitude of the chemical contribution to the enhancement mechanism of surface-enhanced Raman spectroscopy (SERS): Theory and experiment. J. Phys. Chem. Lett. 4, 2599–2604. doi:10.1021/jz4012383
Vega-Vásquez, P., Mosier, N. S., and Irudayaraj, J. (2020). Nanoscale drug delivery systems: From medicine to agriculture. Front. Bioeng. Biotechnol. 8, 79. doi:10.3389/fbioe.2020.00079
Vijayakumar, V., and Khastgir, D. (2018). Hybrid composite membranes of chitosan/sulfonated polyaniline/silica as polymer electrolyte membrane for fuel cells. Carbohydr. Polym. 179, 152–163. doi:10.1016/J.CARBPOL.2017.09.083
Vikesland, P. J. (2018). Nanosensors for water quality monitoring. Nat. Nanotechnol. 13, 651–660. doi:10.1038/s41565-018-0209-9
Wadhawan, S., Jain, A., Nayyar, J., and Mehta, S. K. (2020). Role of nanomaterials as adsorbents in heavy metal ion removal from waste water: A review. J. Water Process Eng. 33, 101038. doi:10.1016/j.jwpe.2019.101038
Walsh, J., Bond, A., Forster, R., and Keyes, T. (2016). Hybrid polyoxometalate materials for photo(electro-) chemical applications. Coord. Chem. Rev. 306, 217–234. doi:10.1016/j.ccr.2015.06.016
Wang, W., and Mattoussi, H. (2020). Engineering the bio-nano interface using a multifunctional coordinating polymer coating. Acc. Chem. Res. 53, 1124–1138. doi:10.1021/acs.accounts.9b00641
Wang, Y., He, Y., Lai, Q., and Fan, M. (2014). Review of the progress in preparing nano TiO2: An important environmental engineering material. J. Environ. Sci. 26, 2139–2177. doi:10.1016/j.jes.2014.09.023
Wani, T. A., and Suresh, G. (2022). Plant-mediated green synthesis of magnetic spinel ferrite nanoparticles: A sustainable trend in nanotechnology. Adv. Sustain. Syst. 6, 2200035. doi:10.1002/adsu.202200035
Watt, J., Cheong, S., and Tilley, R. D. (2013). How to control the shape of metal nanostructures in organic solution phase synthesis for plasmonics and catalysis. Nano Today 8, 198–215. doi:10.1016/j.nantod.2013.03.001
Weller, H. (1993). Colloidal semiconductor Q-particles: Chemistry in the transition region between solid state and molecules. Angew. Chem. Int. Ed. Engl. 32, 41–53. doi:10.1002/anie.199300411
Weller, H. (1998). Quantum size colloids: From size-dependent properties of discrete particles to self-organized superstructures. Curr. Opin. Colloid Interface Sci. 3, 194–199. doi:10.1016/S1359-0294(98)80013-7
Weon, S., He, F., and Choi, W. (2019). Status and challenges in photocatalytic nanotechnology for cleaning air polluted with volatile organic compounds: Visible light utilization and catalyst deactivation. Environ. Sci. Nano 6, 3185–3214. doi:10.1039/c9en00891h
Whitesides, G. (2005). Nanoscience, nanotechnology, and chemistry. Small 1, 172–179. doi:10.1002/smll.200400130
WHO (2022). Guidelines for drinking-water quality: Fourth edition incorporating the first and second addenda. World heal. Organ. 4, 1–614. Available at: https://www.who.int/publications/i/item/9789240045064 (Accessed July 28, 2022).
Wu, M., Li, P., Zhu, Q., Wu, M., Li, H., and Lu, F. (2018). Functional paper-based SERS substrate for rapid and sensitive detection of Sudan dyes in herbal medicine. Spectrochimica Acta Part A Mol. Biomol. Spectrosc. 196, 110–116. doi:10.1016/j.saa.2018.02.014
Xia, Y., Xiong, Y., Lim, B., and Skrabalak, S. E. (2009). Shape-controlled synthesis of metal nanocrystals: Simple chemistry meets complex physics? Angew. Chem. Int. Ed. 48, 60–103. doi:10.1002/anie.200802248
Xu, H., Xiao, K., Wang, X., Liang, S., Wei, C., Wen, X., et al. (2020). Outlining the roles of membrane-foulant and foulant-foulant interactions in organic fouling during microfiltration and ultrafiltration: A mini-review. Front. Chem. 8, 417. doi:10.3389/fchem.2020.00417
Xu, F., Shang, W., Ma, G., Zhu, Y., and Wu, M. (2021a). Metal organic framework wrapped gold nanourchin assembled on filter membrane for fast and sensitive SERS analysis. Sensors Actuators B Chem. 326, 128968. doi:10.1016/j.snb.2020.128968
Xu, F., Xuan, M., Ben, Z., Shang, W., and Ma, G. (2021b). Surface enhanced Raman scattering analysis with filter-based enhancement substrates: A mini review. Rev. Anal. Chem. 40, 75–92. doi:10.1515/revac-2021-0126
Yaashikaa, P. R., Senthil Kumar, P., and Karishma, S. (2022). Review on biopolymers and composites – evolving material as adsorbents in removal of environmental pollutants. Environ. Res. 212, 113114. doi:10.1016/j.envres.2022.113114
Yang, Q., Deng, M., Li, H., Li, M., Zhang, C., Shen, W., et al. (2015). Highly reproducible SERS arrays directly written by inkjet printing. Nanoscale 7, 421–425. Available at: http://xlink.rsc.org/?DOI=C4NR04656K.
Yaqoob, A. A., Parveen, T., Umar, K., and Ibrahim, M. N. M. (2020). Role of nanomaterials in the treatment of wastewater: A review. WaterSwitzerl. 12, 495. doi:10.3390/w12020495
Ye, T., Huang, Z., Zhu, Z., Deng, D., Zhang, R., Chen, H., et al. (2020). Surface-enhanced Raman scattering detection of dibenzothiophene and its derivatives without π acceptor compound using multilayer Ag NPs modified glass fiber paper. Talanta 220, 121357. doi:10.1016/j.talanta.2020.121357
Yeber, C. M., Rodríguez, J., Freer, J., Durán, N., and Mansilla, D. H. (2000). Photocatalytic degradation of cellulose bleaching effluent by supported TiO2 and ZnO. Chemosphere 41, 1193–1197. doi:10.1016/S0045-6535(99)00551-2
Yılmaz, D., Günaydın, B. N., and Yüce, M. (2022). Nanotechnology in food and water security: On-site detection of agricultural pollutants through surface-enhanced Raman spectroscopy. emergent Mat. 5, 105–132. doi:10.1007/s42247-022-00376-w
Yin, Y., and Alivisatos, A. P. (2005). Colloidal nanocrystal synthesis and the organic-inorganic interface. Nature 437, 664–670. doi:10.1038/nature04165
Yina, Y., and Talapin, D. (2013). The chemistry of functional nanomaterials. Chem. Soc. Rev. 42, 2484–2487. doi:10.1039/c3cs90011h
Yorov, K. E., Grigorieva, A. V., Sidorov, A. V., Polyakov, A. Y., Sukhorukova, I. V., Shtansky, D. V., et al. (2016). Inkjet printing of silver rainbow colloids for SERS chips with polychromatic sensitivity. RSC Adv. 6, 15535–15540. doi:10.1039/C5RA25907J
Yu, H., Lyu, Q., Chen, X., Guo, D., He, D., Jia, X., et al. (2021). Nylon membranes modified by gold nanoparticles as surface-enhanced Raman spectroscopy substrates for several pesticides detection. RSC Adv. 11, 24183–24189. doi:10.1039/d1ra03490a
Yu, W. W., and White, I. M. (2012). A simple filter-based approach to surface enhanced Raman spectroscopy for trace chemical detection. Analyst 137, 1168–1173. doi:10.1039/c2an15947c
Yu, W. W., and White, I. M. (2010). Inkjet printed surface enhanced Raman spectroscopy array on cellulose paper. Anal. Chem. 82, 9626–9630. doi:10.1021/ac102475k
Zeng, F., Mou, T., Zhang, C., Huang, X., Wang, B., Ma, X., et al. (2019). Paper-based SERS analysis with smartphones as Raman spectral analyzers. Analyst 144, 137–142. Available at: http://xlink.rsc.org/?DOI=C8AN01901K.
Zhang, H., Jin, M., Xiong, Y., Lim, B., and Xia, Y. (2013). Shape-controlled synthesis of Pd nanocrystals and their catalytic applications. Acc. Chem. Res. 46, 1783–1794. doi:10.1021/ar300209w
Keywords: colloids, nanomaterials, surface chemistry, inorganic nanoparticles, water quality
Citation: Estrada AC, Daniel-da-Silva AL, Leal C, Monteiro C, Lopes CB, Nogueira HIS, Lopes I, Martins MJ, Martins NCT, Gonçalves NPF, Fateixa S and Trindade T (2022) Colloidal nanomaterials for water quality improvement and monitoring. Front. Chem. 10:1011186. doi: 10.3389/fchem.2022.1011186
Received: 03 August 2022; Accepted: 06 September 2022;
Published: 27 September 2022.
Edited by:
Verónica de Zea Bermudez, University of Trás-os-Montes and Alto Douro, PortugalReviewed by:
Thibaud Coradin, UMR7574 Laboratoire de Chimie de la Matière Condensée de Paris (LCMCP), FranceRajapandiyan Panneerselvam, SRM University, India
Copyright © 2022 Estrada, Daniel-da-Silva, Leal, Monteiro, Lopes, Nogueira, Lopes, Martins, Martins, Gonçalves, Fateixa and Trindade. This is an open-access article distributed under the terms of the Creative Commons Attribution License (CC BY). The use, distribution or reproduction in other forums is permitted, provided the original author(s) and the copyright owner(s) are credited and that the original publication in this journal is cited, in accordance with accepted academic practice. No use, distribution or reproduction is permitted which does not comply with these terms.
*Correspondence: Tito Trindade, dGl0b0B1YS5wdA==