- 1Department of Chemistry, Ardabil Branch, Islamic Azad University, Ardabil, Iran
- 2Department of Chemistry, Karaj Branch, Islamic Azad University, Karaj, Iran
- 3Department of Chemistry, Qaemshahr Branch, Islamic Azad University, Qaemshahr, Iran
- 4Department of Chemistry, Zahedan Branch, Islamic Azad University, Zahedan, Iran
We applied the Petasites hybridus rhizome water extract as green media so that Ag/Fe3O4/CdO@ multi-walled carbon nanotubes magnetic nanocomposites (Ag/Fe3O4/CdO@MWCNTs MNCs) could be prepared. We also evaluated its activity by using in the one-pot multicomponent reaction of acetophenones, diethyl oxalate, ammonium acetate, and activated carbonyl compounds such as ninhydrin, isatin and acenaphthylene-1,2-dione, and malononitrile and hydrazoyl chlorides in an aqueous medium at room temperature for the generation of spiro-1,2,4-triazines as new derivatives with tremendous output. Moreover, reducing organic pollutants from 4-nitrophenol (4-NP) was carried out by generating Ag/Fe3O4/CdO@MWCNTs in water at room temperature. The results displayed that Ag/Fe3O4/CdO@MWCNTs reduced pollutants of organic compounds in a short time. The synthesized spiro-1,2,4-triazines have NH and OH functional groups having acidic hydrogen with high antioxidant power. Also, the spiro-1,2,4-triazines exhibited antimicrobial ability. For this purpose, the disk diffusion method was applied and two kinds of bacteria, Gram-positive and Gram-negative, were employed for the analysis. Furthermore, we applied functional theory-based quantum chemical methods in order to better understand reaction mechanism density. To generate spiro-1,2,4-triazines, the applied process showed many properties such as reactions with short time, products with good yields, and simple extraction of catalyst from a mixture of reactions.
Introduction
Among organic compounds, heterocyclic organic compounds are important because of their application in medicinal chemistry and having many biological activities (Goel et al., 2004; Amir et al., 2007; Siddiqui et al., 2011; Lamberth and Dinges, 2012; Abdolmohammadi et al., 2013; Abdolmohammadi 2014; Martins et al., 2015; Desai et al., 2016; Zhao et al., 2017; Fouad et al., 2018; Kalaria et al., 2018; Khattab and Rehan, 2018; Li et al., 2018; Sokolova et al., 2018). Thus, due to the importance of these compounds, many procedures have been reported for the synthesis of heterocyclic compounds. One common procedure to synthesize these compounds with biological activity is multicomponent reactions (MCRs) (Ibarra et al., 2018; Zhi et al., 2019; Borah et al., 2021; Kumar et al., 2022). MCRs are significant due to having benefits such as atom effectiveness and synthesis of heterocyclic compounds with high yields compared with other procedures for the synthesis of heterocyclic compounds (Weber et al., 1999; Tietze et al., 2006; Herrera and Marqués-López, 2015; Borah, et al., 2021). In some procedures for the synthesis of heterocyclic compounds, a catalyst is needed. The transition metal oxide nanostructures with a high active surface area could be used as a catalyst in these reactions. Also, these catalysts are employed in technology and applied science (Sahay et al., 2012; Zhao et al., 2019; Chen et al., 2020). MWCNTs have been widely investigated, due to their large surface area and high adsorption ability (Zhang et al., 2013; Abdolmohammadi 2018; Abdolmohammadi and Hossaini., 2019; Abdolmohammadi et al., 2020). Recently, the supported catalyst and bimetallic oxide or trimetallic oxide catalysts have drawn attention owing to their high capabilities to carry out high-selectivity and efficient organic reactions (Wachs 2005; Dastan et al., 2012; Guo et al., 2014; Janitabar-Darzi and Abdolmohammadi, 2019; Abdolmohammadi et al., 2022). Metal oxides possess great crystalline structure and catalytic efficiency (Shi 2013; Jablonska and Palkovits, 2016). This is the reason why the mixture of two or more metals and their curing mechanisms allow the change in the properties of material surfaces for the optimization of the properties for a particular goal (Zhang et al., 2012; Lin-Bing et al., 2015). Accordingly, the combination of metal oxide catalysts and their nanocomposite structure has exhibited the production of heterocyclic compounds according to green rules with high efficiency (Kalantari et al., 2020; Abdolmohammadi et al., 2021; Khalilzadeh et al., 2021). Among the metal oxide nanoparticles, Fe3O4 magnetic nanoparticles (MNPs) are important because of their high surface area, simply removed from reaction, and their application in MCRs several times. Another subject addressed by this research was biological abilities such as the antioxidant and antimicrobial activity of synthesized spiro pyridoindole pyrrolidines. Compounds with antioxidant activity could eliminate the negative effect of free radicals due to having a reduced chemical structure. Also, these compounds could be employed as transitional metals chelators and they have been effective in improving or treating many illnesses (Halliwell 1999; Liu and Meydani, 2002; Babizhayev et al., 2004; Ahmadi et al., 2007; Djurišić et al., 2012). Another area of investigation on biological activity is the antimicrobial power of synthesized compounds. Some bacteria cannot be killed by utilizing drugs and they cause many diseases in humans and animals. For this reason, it is of great significance to find out procedures with good yield for decreasing this problem and investigating the antimicrobial properties of synthesized compounds. Dyes and pigments are two important components that are used in generating processes of food, drug, textile, and print. Producing dyes and pigments amount to about ∼7 × 105 tons in 1 year, which is hazardous for aquatic system organisms (Mandel et al., 2013). For this reason, discovering green and eco-friendly procedures for removing dyes and pigment pollutants from the environment is very important. Most of the procedures that have been reported in the literature have used much energy and generated adverse by-products. Therefore, high-efficiency methods or active synthesized compounds are required for eliminating or decreasing these problems. Over recent years, enhancing new and easy processes for the generation of essential heterocyclic compounds have been dealt with (Yavari et al., 2007; Yavari et al., 2008; Yavari et al., 2009; Yavari et al., 2010; Hajinasiri et al., 2011; Rostami Charati et al., 2011; Rustaiyan, and Ezzatzadeh, 2011; Ezzatzadeh et al., 2012; Tavakolinia et al., 2012; Hossaini et al., 2014; Rajabi et al., 2015; Rostami-Charati et al., 2015; Rezayati et al., 2016; Sajjadi-Ghotbabadi et al., 2016; Rostami-charati et al., 2017a; Rostami-Charati et al., 2017b; Hossaini et al., 2017; Ezzatzadeh 2018; Rezayati et al., 2018; Ezzatzadeh and Hossaini, 2019a; Ezzatzadeh and Hossaini 2019b; Seifi Mansour et al., 2019; Ezzatzadeh and Hossaini 2020a; Aghahosseini, and Ramazani, 2020; Ahmadi et al., 2020; Baghernejad and Fiuzat 2020; Ezzatzadeh and Hossaini, 2020b; Ebrahimi et al., 2020; Ghanaat et al., 2020; Haddazadeh and Mohammadi, 2020; Hakimi et al., 2020; Khazaei et al., 2020; Mostaghni and Taat, 2020; Nikpassand and Zare Fekri, 2020; Raoufi et al., 2020; Rohaniyan et al., 2020; Sajjadifar et al., 2020; Taran et al., 2020; Tayebee and Gohari, 2020; Baghernejad and Nazari, 2021; Baghernejad and Rostami Harzevili, 2021; Kamali and Shirini, 2021; Karimi et al., 2021; Mohammed Abd Al-Mohson 2021; Salih and Al-Messri, 2021). In this research, initially, a green procedure was employed for the generation of new spiro-1,2,4-triazine 7 via MCRs of activated carbonyl compound 1, acetophenones 2, diethyl oxalate 3, ammonium acetate 4, malononitrile 5, and hydrazoyl chloride 6 in the aqueous form at room temperature in the vicinity of Ag/Fe3O4/CdO@MWCNT MNCs as an organometallic catalyst in aqueous media at room temperature (Scheme 1).
Experimental
General
In this research work, all of the starting materials needed for the synthesis of spiro-1,2,4-triazines and also reagents and solvents were prepared by Fluka and Merck Company with no further purification. For the synthesis of the nanocatalyst, the MWCNTs were used with 8 nm for diameter, 30 μm ling, and 95% of purity, prepared by Merck Company. For approving the construction of synthesized catalyst Ag/Fe3O4/CdO@MWCNT MNCs, spectroscopy analysis such as XRD, SEM, EDX, and VSM was utilized. The FT-IR (KBr medium) of synthesized spiro-1,2,4-triazine was prepared by Shimadzu IR-460 spectrometer instrument. Additionally, another way to confirm the makeup of synthesized compounds was by applying 1H-NMR and 13C-NMR with Bruker DRX-500 AVANCE spectrometer instrument with 500 MHz NMR in CDCl3 as solvent and TMS as the internal standard. Mass spectra for synthesized compounds were given by Finnigan, MAT 8430 spectrometer with an ionization potential of 70 eV. To determine the element in the prepared compounds, we used a Heraeus CHN–O-Rapid analyzer.
Producing Petasites hybridus rhizome water extract
After drying the Petasites hybridus rhizome, 10 g of it was poured into a two-neck round bottom flask (250 ml), followed by adding water (100 ml) and stirring the new mixture at 100°C and filtered after 2 h. For the production of the nanocomposite, the water extract of Petasites hybridus rhizome and other compounds were employed as follows:
Generation of Ag/Fe3O4/CdO@MWCNT MNCs
After dissolving the mixture of Cd (NO3)2 (1.5 g) and FeCl2.4H2O (1.5 g) in water (10 ml), the next step was to add the Petasites hybridus rhizome water extract (5 ml) to the previous mixture, and the temperature of the mixture was enhanced to 100°C in a round bottom flask and mixed for 5 h. When the reaction was completed, the temperature of the reaction was reduced to room temperature. Next, after cooling, to remove the unwanted organic compounds, the mixture experienced sonication for 30 min and was centrifuged at 7,000 rpm for about 10 min. Then, we poured AgNO3 (1.5 g) into the previous mixture and continued the sonication of the new mixture at 100°C for 45 min, and thus, Ag//Fe3O4/CdO MNCs were synthesized. To synthesize Ag//Fe3O4/CdO@MWCNTs MNCs, the MWCNTs (0.1 g) and prepared Ag//Fe3O4/CdO MNCs (0.1 g) in the previous section were added to 100 ml water extract of Petasites hybridus rhizome and mixed at 150°C for 1 h. We used a centrifuge to separate the colloid, then we washed it with water, dried it, and calcinated it at 300°C for 45 min. After this, the Ag//Fe3O4/CdO @MWCNT magnetic nanocomposite was produced, which needed to be cooled to room temperature and washed with a mixture of water and ethanol (50:50) several times. After washing the solid, by employing an external magnet, the catalyst was separated and dried at room temperature for 24 h (Rajendran and Sengodan, 2017).
Preparation process of spiro-1,2,4-triazines 7a–n
Acetophenones 2 (2 mmol), diethyl oxalate 3 (2 mmol), and Ag//Fe3O4/CdO@MWCNT (0.02 g) were added to water as solvent at room temperature and mixed for 30 min. We poured ammonium acetate 4 (2 mmol) into the previous mixture after 30 min and mixed the new mixture for 30 min, followed by adding activated carbonyl compound 1 (2 mmol) and malononitrile 5 (2 mmol) together into another pot in the presence of the catalyst for 45 min. It was then added to the previous mixture and stirred for 30 min. Finally, hydrazoyl chloride 6 (2 mmol) was added and the ultimate mixture was stirred for 1 h in the presence of the catalyst. The reaction was accomplished after 3 h and it was monitored by TLC; in this stage, the separation took place using an external magnet, and the solid residue was washed by EtOH and Et2O to prepare purified spiro-1,2,4-triazines 7.
7a-Hydroxy-5-(4-methoxybenzoyl)-3- (4-methoxyphenyl)-1′,3′-dioxo-1-phenyl-1,1′,3′,7a- tetrahydrospiro[cyclopenta[e][1,2,4]triazine-6,2′-indene]-7,7(4H)-dicarbonitrile (7a): yellow powder, m.p. 138–140°C. Yield: 95%. IR (KBr) (νmax/cm−1): 3,565, 3,348, 2,195, 1,728, 1,725, 1,697, 1,485, 1,378, and 1,292 cm−1. 1H NMR (500 MHz, CDCl3): δ ppm: 3.85 (3 H, s, OMe), 3.78 (3 H, s, OMe), 6.56 (1 H, s, OH), 6.95 (2 H, d, 3J = 7.7 Hz, 2 CH), 7.18–7.23 (4 H, m, 4 CH), 7.28–7.32 (3 H, m, 3 CH), 7.35–7.42 (4 H, m, 4 CH), 7.52–7.57 (3 H, m, 3 CH), 7.87 (2 H, d, 3J = 7.7 Hz, 2 CH), and 10.35 (1 H, s, NH) ppm. 13C NMR (125.7 MHz, CDCl3): δ 50.9, 55.3, 67.9, 95.4, 112.7, 114.7, 118.7, 122.7, 123.7, 126.4, 128.4, 128.8, 129.3, 131.6, 130.2, 133.7, 135.4, 137.0, 137.0, 138.8, 138.8, 140.4, 163.9, 191.7,198.5, and 198.7. MS, m/z (%): 605 (M+, 10), 147 (48), and 31 (100). Anal. Calcd for C36H23N5O5 (605.61): C, 71.40; H, 3.83; and N, 11.56; found: C, 71.35; H, 3.78; N, and 11.48%.
7a-Hydroxy-3-(4-methoxyphenyl)-5-(4-methyl benzoyl)-1′,3′-dioxo-1-phenyl-1,1′,3′,7a- tetrahydrospiro[cyclopenta[e][1,2,4]triazine-6,2′-indene]-7,7(4H)-dicarbonitrile (7b): yellow powder, m.p. 143–145°C. Yield: 0.96 g (95%). IR (KBr) (νmax/cm−1): 3,456, 3,357, 2,232, 1,729, 1,727, 1,698, 1,589, 1,487, 1,358, and 1,296 cm−1. 1H NMR (500 MHz, CDCl3): δ ppm: 2.25 (3 H, s, Me), 3.86 (3 H, s, OMe), 6.57 (1 H, s, OH), 6.85 (2 H, d, 3J = 7.7 Hz, 2 CH), 7.16 (2 H, d, 3J = 7.7 Hz, 2 CH), 7.25–7.29 (3 H, m, 3 CH), 7.32–7.37 (4 H, m, 4 CH), 7.42 (2 H, m, 2 CH), 7.45 (2 H, d, 3J = 7.8 Hz, 2 CH), 7.63 (2 H, d, 3J = 7.8 Hz, 2 CH), and 10.45 (1 H, s, NH). 13C NMR (125.7 MHz, CDCl3): δ 21.3, 50.9, 55.3, 67.9, 95.4, 114.1,114.7, 118.6, 122.6, 123.6, 126.4, 126.9, 127.7, 128.3,129.2, 129.4, 135.4, 136.4, 137.5, 137.8, 138.5, 138.9, 140.4, 142.9, 161.2, 191.9, 198.5, and 198.5. MS, m/z (%): 619 (M+, 10), 147 (48), and 31 (100). Anal. Calcd for C37H25N5O5 (619.64): C, 71.72; H, 4.07; and N, 11.30; found: C, 71.78; H, 4.12; and N, 11.36; %.
5-(4-Ethyl benzoyl)-7a-hydroxy-3-(4-methoxyphenyl)-1′,3′-dioxo-1-phenyl-1,1′,3′,7a- tetrahydrospiro[cyclopenta[e][1,2,4]triazine-6,2′-indene]-7,7(4H)-dicarbonitrile (7c): pale yellow powder, m.p. 153–154°C. Yield: 92%. IR (KBr) (νmax/cm−1): 3,467, 3,348, 2,175, 1,732, 1,727, 1,996, 1,586, 1,485, 1,368, and 1,297 cm−1. 1H NMR (500 MHz, CDCl3): δ ppm: 1.35 (3 H, t, 3JHH = 7.4 Hz, Me), 2.75 H, q, 3JHH = 7.4 Hz, CH2), 3.78 (3 H, s, OMe), 6.63 (1 H, s, OH), 6.94 (2 H, d, 3J = 7.8 Hz, 2 CH), 7.16 (2 H, d, 3J = 7.8 Hz, 2 CH), 7.23 (2 H, d, 3J = 7.8 Hz, 2 CH), 7.26–7.29 (3 H, m, 3 CH), 7.34–7.36 (3 H, m, 3 CH), 7.42–7.45 (4 H, m, 4 CH), 7.52 (2 H, d, 3J = 7.8 Hz, 2 CH), and 10.48 (1 H, s, NH). 13C NMR (125.7 MHz, CDCl3): 15.3, 28.6, 50.9, 55.3, 67.9, 95.4, 114.1, 114.7, 118.6, 122.6, 123.6, 126.4, 126.9, 127.5, 127.7, 129.3, 129.4, 135.4, 136.3, 137.2, 137.5, 138.5, 138.8, 140.4, 146.8, 161.2, 191.8, 197.3, and 198.5. MS, m/z (%): 633 (M+, 10), 147 (48), and 31 (100). Anal. Calcd for C38H27N5O5 (633.66): C, 72.03; H, 4.30; and N, 11.05; found: C, 72.12; H, 4.39; and N, 11.12%.
7a-Hydroxy-5-(4-methyl benzoyl)-1′,3′-dioxo-1,3-diphenyl-1,1′,3′,7a-tetrahydrospiro [cyclopenta[e][1,2,4]triazine-6,2′-indene]-7,7(4H)-dicarbonitrile (7d): yellow powder, m.p. 152–154°C. Yield: 90%. IR (KBr) (νmax/cm−1): 3,478, 3,365, 2,195, 1,734, 1,728, 1,698, 1,595, 1,485, 1,376, and 1,293 cm−1. 1H NMR (500 MHz, CDCl3): δ ppm: 2.36 (3 H, s, Me), 6.52 (1 H, s, OH), 6.85–7.03 (4 H, m, 4 CH), 7.12–7.16 (4 H, m, 4 CH), 7.25 (2 H, d, 3JHH = 7.6 Hz, 2 CH), 7.28–7.32 (3 H, m, 3 CH), 7.42–7.47 (3 H, m, 3 CH), 7.56 (2 H, d, 3J = 7.8 Hz, 2 CH), and 10.68 (1 H, s, NH). 13C NMR (125.7 MHz, CDCl3): δ 21.3, 50.9, 67.6, 95.4, 114.7, 118.6, 122.7, 123.6, 126.4, 127.7, 128.2, 129.2, 130.4, 131.2, 132.3, 133.2, 135.4, 136.4, 137.2, 137.8, 138.8, 139.3, 140.4, 142.9, 191.9, 197.6, and 198.5. MS, m/z (%): 589 (M+, 10), 147 (48), and 31 (100). Anal. Calcd for C36H23N5O4 (589.61): C, 73.34; H, 3.93; and N, 11.88; found: C, 73.42; H, 4.02; and N, 11.93%.
7a-Hydroxy-5-(4-methyl benzoyl)-1′,3′-dioxo-1-phenyl-3-(p-tolyl)-1,1′,3′,7a-tetrahydrospiro [cyclopenta[e][1,2,4]triazine-6,2′-indene]-7,7(4H)-dicarbonitrile (7e): yellow powder, m.p. 163–165°C. Yield: 90%. IR (KBr) (νmax/cm−1): 3,546, 3,384, 1,732, 1,725, 1,695, 1,588, 1,498, 1,378, and 1,295 cm−1. 1H NMR (500 MHz, CDCl3): δ ppm: 2.35 (3 H, s, Me), 2.43 (3 H, s, Me), 6.38 (1 H, s, OH), 6.94 (2 H, d, 3J = 7.6 Hz, 2 CH), 7.02 (2 H, d, 3JHH = 7.6 Hz, 2 CH), 7.06–7.09 (3 H, m, 3 CH), 7.18–7.24 (4 H, m, 4 CH), 7.36 (2 H, d, 3J = 7.8 Hz, 2 CH), 7.42 (2 H, d, 3J = 7.6 Hz, 2 CH), 7.45–7.47 (2 H, m, 2 CH), and 10.45 (1 H, s, NH). 13C NMR (125.7 MHz, CDCl3): δ 21.2, 21.3, 50.9, 67.9, 95.3, 114.7, 118.6, 122.6, 123.6, 124.5, 125.3, 126.4, 127.5, 128.3, 129.2, 129.6, 135.4, 136.3, 137.0, 137.6, 138.7, 138.8, 140.4, 141.4, 142.9, 191.9, 198.4, and 198.5. MS, m/z (%): 603 (M+, 10), 147 (48), and 31 (100). Anal. Calcd for C37H25N5O4 (603.64): C, 73.34; H, 3.93; and N, 11.88; found: C, 73.42; H, 4.02; and N, 11.93%.
7a-Hydroxy-5-(4-methoxybenzoyl)-1′,3′- dioxo-1,3-diphenyl-1,1′,3′,7a-tetrahydrospiro [cyclopenta[e][1,2,4]triazine-6,2′-indene]-7,7(4H)-dicarbonitrile (7f): yellow powder, m.p. 171–173°C. Yield: 92%. IR (KBr) (νmax/cm−1): 3,547, 3,368, 1,734, 1,726, 1,697, 1,597, 1,488, 1,387, and 1,292 cm−1. 1H NMR (500 MHz, CDCl3): δ ppm: 2.38 (3 H, s, Me), 6.57 (1 H, s, OH), 6.92 (2 H, d, 3J = 7.8 Hz, 2 CH), 7.05–7.08 (3 H, m, 3 CH), 7.12–7.16 (4 H, m, 4 CH), 7.23–7.28 (4 H, m, 4 CH), 7.34 (2 H, d, 3J = 7.8 Hz, 2 CH), 7.37–7.42 (3 H, m, 3 CH), and 10.84 (1 H, s, NH). 13C NMR (125.7 MHz, CDCl3): δ 21.3, 50.9, 67.9, 95.3, 114.7, 118.6, 122.6, 123.6, 126.4, 127.7, 128.2, 128.3, 128.9, 129.2, 130.1, 131.5, 135.4, 136.3, 137.0, 137.04, 138.8, 138.9, 140.4, 142.9, 191.9, 198.4, and 198.5. MS, m/z (%): 589 (M+, 10), 147 (48), and 31 (100). Anal. Calcd for C36H23N5O4 (589.61): C, 73.34; H, 3.93; and N, 11.88; found: C, C, 73.43; H, 4.06; and N, 11.95%.
7a-Hydroxy-5-(4-nitrobenzoyl)-1′,3′- dioxo-1-phenyl-3-(p-tolyl)-1,1′,3′,7a-tetrahydrospiro [cyclopenta[e][1,2,4]triazine-6,2′-indene]-7,7(4H)-dicarbonitrile (7g): yellow powder, m.p. 172–174°C. Yield: 87%. IR (KBr) (νmax/cm−1): 3,452, 3,365, 1,729, 1,726, 1,694, 1,584, 1,469, and 1,297 cm−1. 1H NMR (500 MHz, CDCl3): δ ppm: 2.47 (3 H, s, Me), 6.58 (1 H, s, OH), 6.92 (2 H, d, 3J = 7.7 Hz, 2 CH), 7.06–7.11 (4 H, m, 4 CH), 7.26 (2 H, d, 3J = 7.7 Hz, 2 CH), 7.34–7.37 (2 H, m, 2 CH), 7.45–7.48 (3 H, m, 3CH), 7.53 (2 H, d, 3J = 7.7 Hz, 2 CH), 8.06 (2 H, d, 3J = 7.8 Hz, 2 CH), and 10.24 (1 H, s, NH). 13C NMR (125.7 MHz, CDCl3): δ 21.3, 50.9, 67.9, 95.3, 114.7, 118.6, 121.4, 122.6, 123.6, 126.4, 128.3, 129.2, 130.4, 132.5, 133.2, 135.4, 137.2, 137.8, 138.7, 138.8, 139.2, 140.8, 141.3, 149.0, 191.5, 198.4, and 198.5. MS, m/z (%): 634 (M+, 10), 147 (48), and 31 (100). Anal. Calcd for C36H22N6O6 (634.61): C, 68.14; H, 3.49; and N, 13.24; found: C, 68.23; H, 3.58; and N, 13.42%.
7a-Hydroxy-5-(4-methoxybenzoyl)-1′,3′-dioxo-1-phenyl-3-(p-tolyl)-1,1′,3′,7a-tetrahydrospiro [cyclopenta[e][1,2,4]triazine-6,2′-indene]-7,7(4H)-dicarbonitrile (7h): pale yellow powder, m.p. 149–151°C. Yield: 92%. IR (KBr) (νmax/cm−1): 3,458, 3,364, 1,732, 1,726, 1,698, 1,578, 1,364, and 1,286 cm−1. 1H NMR (500 MHz, CDCl3): δ ppm: 2.37 (3 H, s, Me), 3.87 (3 H, s, OMe), 6.62 (1 H, s, OH), 6.93 (2 H, d, 3J = 7.7 Hz, 2 CH), 7.14 (2 H, d, 3J = 7.7 Hz, 2 CH), 7.22–7.26 (3 H, m, 3 CH), 7.30–7.35 (4 H, m, 4 CH), 7.38 (2 H, m, 2 CH), 7.43 (2 H, d, 3J = 7.8 Hz, 2 CH), 7.56 (2 H, d, 3J = 7.8 Hz, 2 CH), and 10.63 (1 H, s, NH). 13C NMR (125.7 MHz, CDCl3): δ 21.5, 51.3, 55.6, 68.3, 95.7, 114.6, 115.3, 118.5, 123.4, 124.5, 127.2, 128.3, 129.5, 130.2, 131.2, 132.3, 134.2, 135.3, 136.2, 137.5, 138.6, 139.3, 141.2, 143.2, 162.3, 192.4, 197.2, and 198.6. MS, m/z (%): 619 (M+, 10), 147 (48), and 31 (100). Anal. Calcd for C37H25N5O5 (619.64): C, 71.72; H, 4.07; and N, 11.30; found: C, 71.78; H, 4.12; and N, 11.36; %.
7a-Hydroxy-5-(4-methoxybenzoyl)-3-(4-methoxyphenyl)-2′-oxo-1-phenyl-1,7a-dihydrospiro[cyclopenta[e][1,2,4]triazine-6,3′-indoline]-7,7(4H)-dicarbonitrile (7i): yellow powder, m.p. 179–181°C. Yield: 1.01 g (92%). IR (KBr) (νmax/cm−1): 3,478, 3,374, 1,697, 1,732, 1,727, 1,595, 1,478, 1,376, and 1,284 cm−1. 1H NMR (500 MHz, CDCl3): δ ppm: 3.78 (3 H, s, OMe), 3.85 (3 H, s, OMe), 6.43 (1 H, s, OH), 6.89 (2 H, d, 3J = 7.7 Hz, 2 CH), 7.02 (2 H, d, 3J = 7.8 Hz, 2 CH), 7.05–7.09 (4 H, m, 4 CH), 7.17–7.22 (3 H, m, 3 CH), 7.32 (2 H, d, 3J = 7.7 Hz, 2 CH), 7.38 (2 H, d, 3J = 7.8 Hz, 2 CH), 7.42–7.45 (2 H, m, 2 CH), 9.87 (1 H, s, NH), and 10.25 (1 H, s, NH). 13C NMR (125.7 MHz, CDCl3): δ 55.2, 55.3, 55.3, 66.9, 94.9, 111.5, 112.7, 113.8, 114.2, 118.6, 122.5,123.7,124.4,124.9,126.7, 127.4, 128.6, 129.3, 130.4, 131.2, 133.6, 138.4, 139.2, 140.3, 141.2, 161.0, 163.9, 175.8, and 192.2. MS, m/z (%): 619 (M+, 10), 147 (48), and 31 (100). Anal. Calcd for C37H26N6O5 (622.64): C, 69.45; H, 4.21; and N, 13.50; found: C, 69.53; H, 4.32; and N, 13.65%.
7a-Hydroxy-3-(4-methoxyphenyl)-5- (4-methylbenzoyl)-2′-oxo-1-phenyl-1,7a-dihydrospiro[cyclopenta [e][1,2,4]triazine-6,3′-indoline]-7,7(4H)-dicarbonitrile (7j): yellow powder, m.p. 182–184°C. Yield: 87%. IR (KBr) (νmax/cm−1): 3,487, 3,369, 2,195, 1,735, 1,728, 1,689, 1,587, 1,486, 1,387, and 1,268 cm−1. 1H NMR (500 MHz, CDCl3): δ ppm: 2.32 (3 H, s, Me), 3.89 (3 H, s, OMe), 6.63 (1 H, s, OH), 6.93 (2 H, d, 3J = 7.7 Hz, 2 CH), 7.13 (2 H, d, 3J = 7.7 Hz, 2 CH), 7.19–7.24 (3 H, m, 3 CH), 7.28–7.33 (4 H, m, 4 CH), 7.38–7.42 (2 H, m, 2 CH), 7.47 (2 H, d, 3J = 7.8 Hz, 2 CH), 7.54 (2 H, d, 3J = 7.8 Hz, 2 CH), 9.85 (1 H, s, NH), and 10.67 (1 H, s, NH). 13C NMR (125.7 MHz, CDCl3): δ 21.6, 51.3, 55.7, 68.3, 96.3, 113.7, 114.2, 118.7, 121.8, 123.4, 125.6, 126.5, 127.4, 128.2, 129.7, 130.4, 134.8, 136.5, 137.2, 138.4, 139.3, 140.7, 141.2, 142.7, 161.4, 163.4, and 191.7. MS, m/z (%): 606 (M+, 10), 147 (48), and 31 (100). Anal. Calcd for C36H26N6O4 (606.64): C, 71.28; H, 4.32; and N, 13.85; found: C, 71.36; H, 4.43; and N, 13.97%.
7a-Hydroxy-5-(4-nitrobenzoyl)-2′-oxo-1,3 -diphenyl-1,7a-dihydrospiro[cyclopenta[e] [1,2,4]triazine-6,3′-indoline]-7,7(4H)-dicarbonitrile (7k): yellow powder, m.p. 192–194°C. Yield: 87%. IR (KBr) (νmax/cm−1): 3,458, 3,345, 2,197, 1,736, 1,727, 1,695, 1,587, 1,463, 1,357, and 1,296 cm−1. 1H NMR (500 MHz, CDCl3): δ ppm: 6.67 (1 H, s, OH), 6.95 (2 H, d, 3J = 7.8 Hz, 2 CH), 7.04–7.08 (4 H, m, 4 CH), 7.18 (2 H, d, 3J = 7.7 Hz, 2 CH), 7.22–7.25 (2 H, m, 2 CH), 7.33–7.37 (3 H, m, 3CH), 7.48 (2 H, d, 3J = 7.7 Hz, 2 CH), 8.06 (2 H, d, 3J = 7.8 Hz, 2 CH), 9.68 (1 H, s, NH), and 10.37 (1 H, s, NH). 13C NMR (125.7 MHz, CDCl3): δ 21.7, 51.3, 68.3, 95.5, 115.8, 119.3, 121.7, 122.5, 123.7, 126.6, 128.7, 129.5, 131.2, 132.7, 133.5, 135.6, 136.8, 137.5, 138.3, 139.3, 140.5, 141.3, 142.4, 148.4, 164.3, and 191.7. MS, m/z (%): 621 (M+, 10), 147 (48), and 31 (100). Anal. Calcd for C35H23N7O5 (621.61): C, 67.63; H, 3.73; and N, 15.77; found: C, 67.74; H, 3.86; and N, 15.92%.
7a′-Hydroxy-5′-(4-methoxybenzoyl)-2-oxo-1′,3′- diphenyl-1′,7a′-dihydro-2H-spiro [acenaphthylene-1,6′-cyclopenta[e][1,2,4]triazine]-7′,7′(4′H)-dicarbonitrile (7l): yellow powder, m.p. 186–188°C. Yield: 85%. IR (KBr) (νmax/cm−1): 3,574, 3,358, 2,185, 1,735, 1,727, 1,694, 1,489, 1,368, and 1,297 cm−1. 1H NMR (500 MHz, CDCl3): δ ppm: 3.78 (3 H, s, OMe), 6.82 (1 H, s, OH), 6.97 (2 H, d, 3J = 7.8 Hz, 2 CH), 7.03–7.07 (5 H, m, 5 CH), 7.12–7.16 (3 H, m, 3 CH), 7.23–7.27 (4 H, m, 4 CH), 7.38 (2 H, d, 3J = 7.8 Hz, 2 CH), 7.43–7.47 (4 H, m, 4 CH), and 10.37 (1 H, s, NH). 13C NMR (125.7 MHz, CDCl3): δ 55.3, 56.7, 66.5, 95.4, 112.7, 113.4, 114.5, 115.2, 116.3, 117.6, 118.5, 119.4, 120.3, 121.8, 122.3, 122.8, 123.4, 124.5, 125.3, 126.7, 128.5, 127.2, 129.3, 130.8, 131.6, 132.4, 133.2, 136.5, 137.4, 138.5, 139.2, 140.3, 163.9, 192.2, and 195.3. MS, m/z (%): 627 (M+, 10), 147 (48), and 31 (100). Anal. Calcd for C39H25N5O4 (627.66): C, 74.63; H, 4.01; and N, 11.16; found: C, 74.73; H, 4.14; and N, 11.28%.
7a′-Hydroxy-5′-(4-nitrobenzoyl)-2-oxo-1′-phenyl-3′-(p-tolyl)-1′,7a′-dihydro-2H-spiro[acenaphthylene-1,6′- cyclopenta[e][1,2,4]triazine]-7′,7′(4′H)-dicarbonitrile (7m): yellow powder, m.p. 193–195°C. Yield: 80%. IR (KBr) (νmax/cm−1): 3,547, 3,378, 2,186, 1,736, 1,726, 1,692, 1,568, 1,367, and 1,297 cm−1. 1H NMR (500 MHz, CDCl3): δ ppm: 2.42 (3 H, s, Me), 6.57 (1 H, s, OH), 6.95 (2 H, d, 3J = 7.7 Hz, 2 CH), 7.12 (2 H, d, 3J = 7.7 Hz, 2 CH), 7.16–7.21 (4 H, m, 4 CH), 7.27–7.32 (4 H, m, 4 CH), 7.38 (2 H, d, 3J = 7.7 Hz, 2 CH), 7.42–7.46 (3 H, m, 3 CH), 8.06 (2 H, d, 3J = 7.8 Hz, 2 CH), and 10.38 (1 H, s, NH). 13C NMR (125.7 MHz, CDCl3): δ 21.3, 56.7, 66.67, 95.5, 112.3, 113.6, 114.2, 115.8, 116.4, 117.6, 118.7, 119.2, 120.8, 121.5, 122.8, 123.5, 124.2, 125.7, 127.3, 128.5, 129.3, 129.7, 130.8, 132.4, 136.5, 137.4, 138.5, 139.3, 143.4, 145.3, 146.3, 149.2, 191.3, and 196.3. MS, m/z (%): 656 (M+, 10), 147 (48), and 31 (100). Anal. Calcd for C39H24N6O5 (656.66): C, 71.34; H, 3.68; and N, 12.80; found: C, 71.45; H, 3.78; and N, 12.92; %.
7a′-Hydroxy-5′-(4-methoxybenzoyl)-2-oxo-1′- phenyl-3′-(p-tolyl)-1′,7a′-dihydro-2H-spiro[acenaphthylene-1,6′- cyclopenta[e][1,2,4]triazine]-7′,7′(4′H)-dicarbonitrile (7n): yellow powder, m.p. 181–183°C. Yield: 87%). IR (KBr) (νmax/cm−1): 3,486, 3,367, 2,234, 1,738, 1,728, 1,697, 1,587, 1,492, 1,387, and 1,265 cm−1. 1H NMR (500 MHz, CDCl3): δ ppm: 2.42 (3 H, s, Me), 3.85 (3 H, s, OMe), 6.57 (1 H, s, OH), 6.92 (2 H, d, 3J = 7.7 Hz, 2 CH), 7.12 (2 H, d, 3JHH = 7.8 Hz, 2 CH), 7.14–7.17 (5 H, m, 5 CH), 7.23–7.27 (3 H, m, 3 CH), 7.38 (2 H, d, 3J = 7.8 Hz, 2 CH), 7.43–7.47 (3 H, m, 3 CH), 7.54 (2 H, d, 3J = 7.8 Hz, 2 CH), and 10.64 (1 H, s, NH). 13C NMR (125.7 MHz, CDCl3): δ 21.3, 55.3, 56.7, 66.5, 95.4, 110.4, 112.7, 114.0, 115.2, 116.3, 117.7, 118.2, 119.5, 120.4, 121.5, 122.7, 123.3, 124.2, 125.3, 127.2, 128.9, 129.3, 129.7, 130.2, 130.9, 132.4, 133.2, 134.2, 137.4, 138.2, 138.7, 139.4, 140.4, 163.9, 192.2, and 196.3. MS, m/z (%): 641 (M+, 10), 147 (48), and 31 (100). Anal. Calcd for C40H27N5O4 (641.69): C, 74.87; H, 4.24; and N, 10.91; found: C, C, 74.96; H, 4.34; and N, 11.02%.
Evaluation of antioxidant property via DPPH
As mentioned above, in this research, the antioxidant property of some synthesized spiro-1,2,4-triazine such as 7a–7d was investigated using DPPH free radical utilizing Shimada et al. (1992)’s procedures. According to the Shimada method, the concentration of spiro-1,2,4-triazine 7a–7d was selected between 200 and 1,000 ppm, and a methanolic solution of DPPH (1 mmol/L) in equivalent volume was added to the spiro-1,2,4-triazine solution. The new mixture was mixed at room temperature, followed by placing it in a dark room after 30 min, where the absorbance of the mixture reached 517 nm. We compared the antioxidant activity of synthesized spiro-1,2,4-triazine 7a–7d butylated hydroxytoluene (BHT) and 2-tertbutylhydroquinone (TBHQ) and instead of synthesized compounds, methanol (3 ml) was used. To measure the percentage of inhibition of the DPPH radical trapping experiment, the equation in the work of Yen and Duh (1994) was used.
Evaluating the FRAP process of spiro-1,2,4-triazine antioxidant activity
Another way to look at the antioxidant properties of spiro-1,2,4-triazine is using the FRAP process that measures the amounts of iron (III) reduction by synthesized spiro-1,2,4-triazine 7a–7d employing Yildirim et al.’s (2001) procedure. In this experiment, the spiro-1,2,4-triazine solution (1 ml), potassium ferricyanide (2.6 ml), and phosphate buffer (2.6 ml) were used to evaluate antioxidant activity according to Yildirim et al. (2001)’s procedure. The temperature of the mixture was maintained at 55°C for 35 min, followed by adding trichloroacetic acid (2.5 ml) to the new mixture and stirring it for 10 min. Finally, the absorbance of FeCl3 (0.6 ml) and the supernatant (2.5 ml) mixture in aqueous media (2.6 ml) as a sample was measured at 700 nm. The results showed that compounds with a high reducing ability have a greater power of absorbance. To confirm the calculations, they were computed three times. We ran the SPSS software version 18.0 to compute the analysis of variance (ANOVA) for synthesizing spiro-1,2,4-triazine data, which approved samples and standard variation. We also applied Duncan multiple-range experiments for separation with 95% (p < 0.05).
Examining the antibacterial activity of the prepared spiro-1,2,4-triazine
To study the antibacterial activity of the prepared spiro-1,2,4-triazine, we prepared a Persian-type culture collection (PTCC) of Gram-positive and Gram-negative bacteria in Tehran, Iran, and for this reason, the disk diffusion procedure was utilized. For evaluating the antimicrobial ability of spiro-1,2,4-triazine, the two types of bacteria concentrations were similarly prepared according to the McFarland Standard No. 0.5 and were cultured for 16–24 h at 37°C. We used two standard drugs, namely, streptomycin and gentamicin, that killed bacteria. We prepared the suspension of bacteria with a sterile swab cultured on Mueller Hinton agar consistent with the McFarland Standard No. 0.5 (1.5 × 108 CFU/ml). Then, to deal with antibacterial properties, spiro-1,2,4-triazine (25 μg/ml) was added on sterile blank disks and the ready sample was placed for 24 h at 37°C in an incubator. We measured the diameter of inhibition and compared it with the standard sample.
Ag/Fe3O4/CdO@MWCNT MNC application in the reduction of 4-NP
For this purpose, the mixture of Ag/Fe3O4/CdO@MWCNT MNCs (0.005 g) and 4-nitrophenol solution (25 ml, 2.5 mM) was stirred for 2 min at room temperature in the beaker and the newly produced NaBH4 (25 ml, 0.25 M) was added to the previous mixture as reducing agent, which could remove pollutants in the presence of the catalyst. After adding the aqueous NaBH4 to the first mixture, the solution color varied from pale yellow to lemon-colored. The stirring of the mixture was continued until the mixture became colorless. Next, for measuring the UV-Vis absorption, 1 ml of the solution was diluted to 25 ml at sure times. The concentration of 4-nitrophenol varied between 200 and 700 nm at room temperature and it was checked by the UV-Vis absorption spectra. The main point in the catalyst is its reusability in the same reactions. To confirm this point, the catalyst was removed from the mixture of reaction and washed with ethanol and finally dried to be reused in the same reaction.
Results and discussion
In the current study, the new spiro-1,2,4-triazines 7 were produced with high efficiency by applying six component reactions of activated carbonyl compound 1, methyl ketones 2, diethyl oxalate 3, ammonium acetate 4, malononitrile 5, and hydrazoyl chlorides 6 in aqueous media at room temperature in the vicinity of Ag/Fe3O4/CdO@MWCNT MNCs as a new reusable organometallic nanocatalyst. The catalytic activities of Ag/Fe3O4/CdO@MWCNT MNCs MNCs were evaluated via the synthesis of spiro-1,2,4-triazine derivatives in the presence of Ag/Fe3O4/CdO@MWCNT MNCs. The important issue in all organic reactions lies in achieving the best condition for conducting the reactions. To achieve this purpose, we initially chose the multicomponent reaction of 4-methoxyacethophenone 1a, diethyl oxalate 2, ammonium acetate 3, ninhydrin 4, malononitrile 5, and hydrazoyl chloride 6a as a model reaction (Table 1). Without the catalyst even after 10 h, the production of compound 7a was not carried out (entry 1, Table 1). To optimize the temperature of the sample reaction and achieve the best temperature, the reaction temperature was increased to 100°C but it did not exhibit significant variation in the efficiency of spiro-1,2,4-triazine 7a (Entry 2, Table 1). Also, such reactions did not take place without the catalyst. To confirm this point, CdO-NPs (0.01 g) as catalysts were added to the mixture of reaction. After 4 h, spiro-1,2,4-triazine 7a was generated with good efficiency (entry 4, Table 1). Therefore, these reactions needed a catalyst for their performance. To find out the best catalyst for the model reaction, we considered many nanocatalysts, including Ag NPs, Fe3O4 MNPs, CdO NPs, Fe3O4/CdO NPs, Fe3O4/CdO/MWCNTs, Ag@MWCNTs, MWCNTs, Ag NPs, and Ag/Fe3O4/CdO@MWCNTs. Among these catalysts, Ag/Fe3O4/CdO@MWCNTs was selected as the nanocatalyst for the synthesis of spiro-1,2,4-triazine 7a, and the production efficiency was increased by this catalyst. Ag/Fe3O4/CdO@MWCNTs as a catalyst have two sites in their structure. The three sites in the synthesized catalyst (Ag, Fe, and Cd) are Lewis acids and are caused by the activation of carbonyl groups. According to the results shown in Table 1, Ag NPs are more useful compared with Fe3O4, CdO, Fe3O4/CdO, and Fe3O4/CdO/MWCNT. Ag is a stronger Lewis acid than Fe3O4 and TiO2 and is very significant by which these reactions were conducted with catalytic amounts of Ag/Fe3O4/CdO/MWCNTs-MNCs. Therefore, increasing Ag/Fe3O4/CdO/MWCNTs-MNCs amounts from 0.02 to 0.03 g did not show any remarkable variation in the efficiency of the reaction. So, 0.02 g of Ag/Fe3O4/CdO/MWCNTs-MNCs was needed for the preparation of spiro-1,2,4-triazine with high efficiency (entry 11, Table 1) and the yield of compound 7a is 95% after 3 h (entry 11, Table 1). The role of nanocatalysts in the preparation of spiro-1,2,4-triazine derivatives are Lewis acid and Lewis base. Ag, Cd, and Fe as Lewis acids activate the carbonyl group for nucleophilic attack. As displayed in Table 1, among Lewis acids, Ag is more effective compared with Cd and Fe.
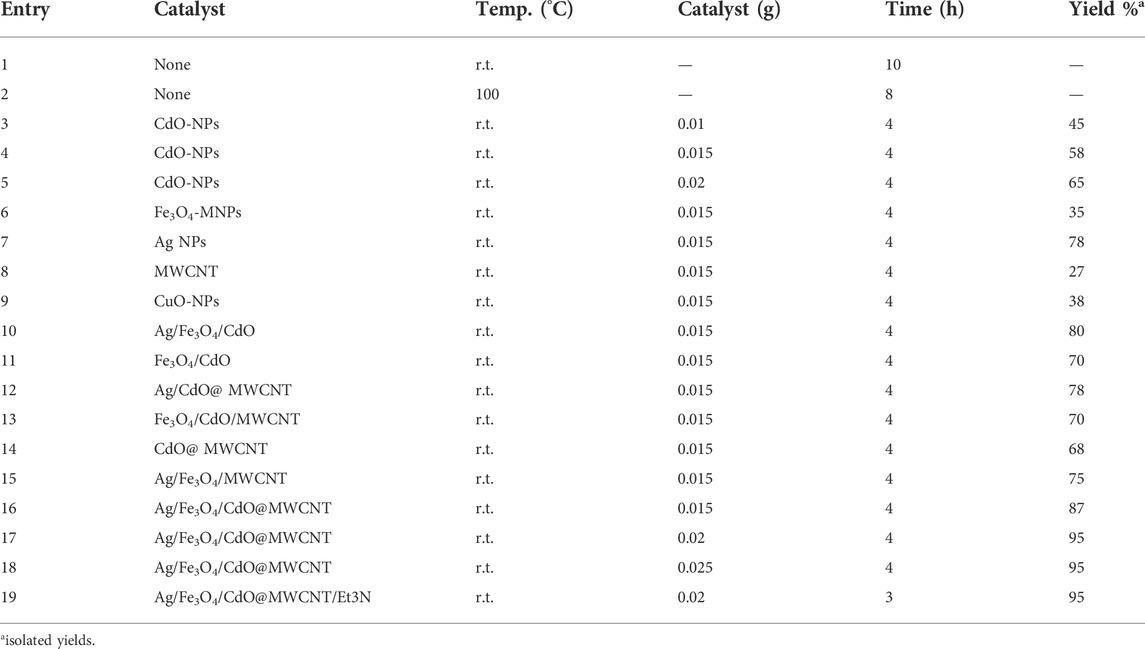
TABLE 1. Determining the most optimal conditions, including catalyst, amount of catalyst, and temperature for the synthesis of 7a.
Because of the easy and simple extraction of Fe3O4 magnetic nanoparticles (MNPs) from the mixture of reactions, their application in several reactions is very significant. Additionally, in this inquiry, the solvent’s effects on the synthesis of compound 7a in the presence of Ag/Fe3O4/CdO@MWCNT (0.02 g) were investigated. The results in Table 2 display that water is the best solvent to carry out the reaction.
As illustrated in Tables 1 and 2, Ag/Fe3O4/CdO@MWCNT-MNC (0.02 g) as an organometallic catalyst, room temperature, and aqueous media are the suitable conditions for the generation of spiro-1,2,4-triazine 7. Reusing the synthesized catalysts is an important factor in the synthesis of organic compounds. In this research, the synthesized nanocatalyst was reused five times for the synthesis of spiro-1,2,4-triazine 7a (Table 3). The final results attested that the catalyst could be used again five times with negligible change in its power (Table 3). To use the magnetic nanocatalyst again, we require the exterior magnet to separate the catalyst from the mixture of reactions, followed by washing the catalyst with water and drying it at room temperature for 24 h, and using it another time.
After each run, to synthesize compound 7a, the catalyst was removed from the mixture of reactions, washed, and used again. For this reason, the yield of compound 7a decreased after five times because of the reduced amount of catalyst and its separation after each run. It should be mentioned that after the separation of the catalyst, the amount of the catalyst might be changed but not its form and size. Lowering the ratios of the catalyst has the most impact on the efficiency of compound 7a. For confirmation, the structure of synthesized spiro-1,2,4-triazine 7, 1H NMR, 13C NMR, and IR, elemental analysis and mass spectrum were employed. At 3.78, 3.83, and 3.87 ppm in the 1H NMR spectra of spiro-1,2,4-triazine 7a displayed three singlets for methoxy protons. The one singlet appeared at 10.32 ppm for NH proton and several signals for aromatic protons at 6.98–8.22 ppm. The carbonyl moiety displayed four resonances in the 13C NMR spectra of 7a at 164.2, 165.2, 167.3, and 187.2 ppm. Also, another route for confirming the existence of carbonyl groups in the construction of synthesized compounds is the IR spectrum. The preparation mechanism for synthesized compounds 7 is recommended as in Scheme 2.
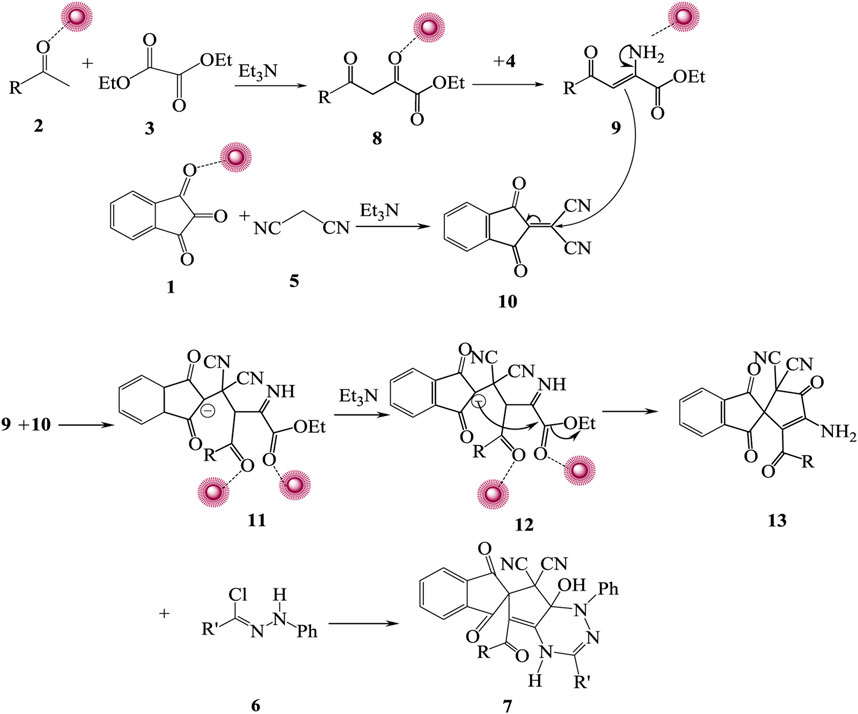
SCHEME 2. Recommended mechanism for preparation of 7. First, acetophenones 2 and diethyl oxalate 3 reacted at room temperature accompanied by Ag/Fe3O4/CdO@MWCNT MNCs, and 1,3-dicarbonyl 8 was generated. Ammonium acetate 4 reacted with the carbonyl group of intermediate 8 and produced iminium ion 9 that reacted with additional intermediate 10 from the reaction of carbonyl compound 1 reacting with malononitrile 5 and generating intermediate 11. Intermolecular cyclization of intermediate 12 made intermediate 13 that reacted with hydrazoyl chloride 6 produced spiro-1,2,4-triazine 7.
Evaluating the antioxidant property of prepared spiro-1,2,4-triazine by DPPH
Furthermore, this investigation aimed at examining the antioxidant property of synthesized spiro-1,2,4-triazines, and DPPH was used to realize this goal. It should be mentioned that we applied DPPH radical scavenging test for many purposes such as the antioxidant activity of synthesized organic compounds, foods, and biological structures (Bidchol et al., 2011; Saundane and Nandibeoor, 2015) by taking electron or hydrogen atoms by free radical of DPPH. The synthesized spiro-1,2,4-triazine loses the hydrogen atom or one electron in the presence of the DPPH radical, meaning that these compounds have antioxidant properties. The percentage of trapping the DPPH free radical by the synthesized spiro-1,2,4-triazine displays the order of antioxidant activity. Through this inquiry, we examined the antioxidant activity of some synthesized compounds such as 7a–7d and compared them with standard synthesized antioxidant BHT and TBHQ, where the electron or hydrogen absorbance of these compounds via DPPH free radical proved their antioxidant activity. If an electron or hydrogen atom is adsorbed via DPPH, its absorbance decreases by 517 nm. Overall, the antioxidant ability of spiro-1,2,4-triazine derivatives 7a–7d obtained was as TBHQ ≈ BHT>7a > 7d > 7c > 7b (Figure 1).
Figure 1 showed that good differences existed between the spiro-1,2,4-triazine concentration of BHT and TBHQ as standard antioxidants. Compound 7a showed a good ability relative to BHT and TBHQ among experimented spiro-1,2,4-triazine 7a–7d.
Assessment of spiro-1,2,4-triazine antioxidant activity using Fe3+ reduction
The antioxidant property of spiro-1,2,4-triazine 7a–7d was tested by another procedure for confirming it. Spiro-1,2,4-triazine caused the reduction of ferric ions (Fe3+) and amounts of reduction were measured based on the reduction of Fe3+/ferricyanide to the Fe2+/ferrous at 700 nm[51] and spiro-1,2,4-triazine 7a exhibited good effect compared with BHT and TBHQ. Figure 2 shows the order of spiro-1,2,4-triazine 7a–7d antioxidant activity as TBHQ > BHT > 7a > 7d > 7c > 7b.
Antibacterial activity evaluation of synthesized spiro-1,2,4-triazine
To study the antibacterial activity of synthesized compounds, we used two antibiotic drugs, namely, streptomycin and gentamicin and compared the results of the antimicrobial synthesized compounds with two standards as displayed in Table 4. For the evaluation of this experiment, two suitable and significant factors that affect the diameter and inhibition zone are the type of bacteria and the concentration of synthesized spiro-1,2,4-triazines. Among the Gram-positive and negative bacteria, spiro-1,2,4-triazines 7b, 7d, 7f, and 7g affect Escherichia coli owing to a good diameter of the inhibition zone.
Conclusion
This study approached effective, green, and environment-friendly reactions, namely, acetophenones, diethyl oxalate, ammonium acetate, ninhydrin, malononitrile, and hydrazoyl chlorides in aqueous media at an ambient temperature in the presence of the new organometallic nanocatalyst Ag/Fe3O4/CdO@MWCNT-MNC, which generated new derivatives of spiro-1,2,4-triazines. Also, two methods were employed for the evaluation of the antioxidant power of the synthesized spiro-1,2,4-triazines 7a–7d. DPPH radical trapping and reducing Fe+3 by synthesized compounds, these two procedures confirmed that the synthesized compounds had good antioxidant abilities relative to standard antioxidants. Moreover, to examine the antibacterial activity of synthesized compounds, we utilized the Gram-positive and negative bacteria, and to confirm the antimicrobial ability of the produced spiro-1,2,4-triazines, we relied on the disk diffusion process. The results of the antioxidant and antimicrobial activity investigations display that synthesized spiro-1,2,4-triazines have good biological activity and could prevent bacterial growth. Therefore, this procedure that is used for the synthesis of spiro-1,2,4-triazines carries several advantages, including reactions with high rate, high yield output, green processes, using low amounts of catalyst, easy separation of organometallic catalyst from the mixture of reaction, and easy product purification, which are the important aspects in these reactions.
Data availability statement
The original contributions presented in the study are included in the article/Supplementary Material; further inquiries can be directed to the corresponding author.
Author contributions
All authors listed have made a substantial, direct, and intellectual contribution to the work and approved it for publication.
Acknowledgments
The authors gratefully acknowledge the support of the Islamic Azad University of Ardabil.
Conflict of interest
The authors declare that the research was conducted in the absence of any commercial or financial relationships that could be construed as a potential conflict of interest.
Publisher’s note
All claims expressed in this article are solely those of the authors and do not necessarily represent those of their affiliated organizations, or those of the publisher, the editors, and the reviewers. Any product that may be evaluated in this article, or claim that may be made by its manufacturer, is not guaranteed or endorsed by the publisher.
Supplementary Material
The Supplementary Material for this article can be found online at: https://www.frontiersin.org/articles/10.3389/fchem.2022.1001707/full#supplementary-material
References
Abdolmohammadi, S. (2014). α-ZrP: A highly efficient catalyst for solvent-free synthesis of pyrimido[ 5', 4':5, 6]pyrido[2, 3-d]pyrimidinetetraone and 4-arylacridinedione derivatives. Lett. Org. Chem. 11, 465–469. doi:10.2174/1570178611666140124002242
Abdolmohammadi, S., Balalaie, S., Barari, M., and Rominger, F. (2013). Three-Component green reaction of arylaldehydes, 6-amino-1, 3-dimethyluracil and active methylene compounds catalyzed by Zr(HSO4)4 under solvent-free conditions. Comb. Chem. High. Throughput Screen. 16 (2), 150–159. doi:10.2174/1386207311316020009
Abdolmohammadi, S., and Hossaini, Z. S. (2019). Fe3O4 MNPs as a green catalyst for syntheses of functionalized [1, 3]-oxazole and 1H-pyrrolo-[1, 3]-oxazole derivatives and evaluation of their antioxidant activity. Mol. Divers. 23 (4), 885–896. doi:10.1007/s11030-019-09916-9
Abdolmohammadi, S., Hossaini, Z. S., and Poor Heravi, R. (2022). PANI-Fe3O4@ZnO nanocomposite as magnetically recoverable organometallic nanocatalyst promoted synthesis of new azo chromene dyes and evaluation of their antioxidant and antimicrobial activities. Mol. Divers. 26, 1983–1993. In press. doi:10.1007/s11030-021-10309-0
Abdolmohammadi, S., Shariati, S., Elmi Fard, N., and Samani, A. (2020). Aqueous‐Mediated green synthesis of novel spiro[indole‐quinazoline] derivatives using kit-6 mesoporous silica coated Fe3 O4 nanoparticles as catalyst. J. Heterocycl. Chem. 57 (7), 2729–2737. doi:10.1002/jhet.3981
Abdolmohammadi, S., Shariati, S., and Mirza, B. (2021). Ultrasound promoted and Kit-6 mesoporous silica supported Fe 3 O 4 magnetic nanoparticles catalyzed cyclocondensation reaction of 4‐hydroxycoumarin, 3, 4‐methylenedioxyphenol, and aromatic aldehydes. Appl. Organomet. Chem. 35 (3), e6117. doi:10.1002/aoc.6117
Abdolmohammadi, S. (2018). TiO2 NPs-coated carbone nanotubes as a green and efficient catalyst for the synthesis of [1]benzopyrano[b] [1]benzopyranones and xanthenols in water. Comb. Chem. High. Throughput Screen. 21 (8), 594–601. doi:10.2174/1386207321666181018164739
Aghahosseini, H., and Ramazani, A. (2020). Magnetite L-proline as a reusable nano-biocatalyst for efficient synthesis of 4H-benzo[b]pyrans in water: A green protocol. Eurasian Chem. Commun. 2 (3), 410–419. doi:10.33945/SAMI/ECC.2020.3.11
Ahmadi, F., Kadivar, M., and Shahedi, M. (2007). Antioxidant activity of Kelussia odoratissima Mozaff. in model and food systems. Food Chem. x. 105, 57–64. doi:10.1016/j.foodchem.2007.03.056
Ahmadi, N., Ramazani, A., Rezayati, S., and Hosseini, F. (2020). Synthesis of silica nanoparticles and study of its catalytic properties in the preparation of carboxylic esters. Eurasian Chem. Commun. 2 (8), 862–874. doi:10.22034/ecc.2020.108366
Amir, M., Javed, S. A., and Kumar, H. (2007). Pyrimidine as antiinflammatory agent: A review. Indian J. Pharm. Sci. 69 (3), 337–343. doi:10.4103/0250-474X.34540
Babizhayev, M. A., Deyev, A. I., Yermakovea, V. N., Brikman, I. V., and Bours, J. (2004). Lipid peroxidation and cataracts: N-Acetylcarnosine as a therapeutic tool to manage age-related cataracts in human and in canine eyes. Drugs R D 5, 125–139. doi:10.2165/00126839-200405030-00001
Baghernejad, B., and Fiuzat, M. (2020). A new strategy for the synthesis of 2-amino-4H-pyran derivatives in aqueous media using DABCO-Cucl complex as a novel and efficient catalyst. Eurasian Chem. Commun. 2 (11), 1088–1092.
Baghernejad, B., and Nazari, L. (2021). Synthesis of indeno [1, 2-b] pyridine derivatives in the precense of Nano CeO2/ZnO. Eurasian Chem. Commun. 3 (5), 319–326. doi:10.22034/ecc.2021.277002.1145
Baghernejad, B., and Rostami Harzevili, M. (2021). Nano-cerium oxide/aluminum oxide: An efficient and useful catalyst for the synthesis of tetrahydro[a]xanthenes-11-one derivatives. Chem. Methodol. 5 (2), 90–95. doi:10.22034/chemm.2021.119641
Bidchol, A. M., Wilfred, A., Abhijna, P., and Harish, R. (2011). Free radical scavenging activity of aqueous and ethanolic extract of Brassica oleracea L. Var. italica. Food bioproc. Tech. 4, 1137–1143. doi:10.1007/s11947-009-0196-9
Borah, B., Dhar Dwivedi, K., and Chowhan, L. R. (2021). 4-Hydroxycoumarin: A versatile substrate for transition‐metal‐free multicomponent synthesis of bioactive heterocycles. Asian J. Org. Chem. 10 (12), 3101–3126. doi:10.1002/ajoc.202100550
Chen, X., Mao, J., Liu, C., Chen, C., Cao, H., and Yu, L. (2020). An unexpected generation of magnetically separable Se/Fe3O4 for catalytic degradation of polyene contaminants with molecular oxygen. Chin. Chem. Lett. 31 (12), 3205–3208. doi:10.1016/j.cclet.2020.07.031
Dastan, A., Kulkarnia, A., and Torok, B. (2012). Environmentally benign synthesis of heterocyclic compounds by combined microwave-assisted heterogeneous catalytic approaches. Green Chem. 14, 17–37. doi:10.1039/C1GC15837F
Desai, N., Trivedi, A., Pandit, U., Dodiya, A., Rao, V. K., and Desai, P. (2016). Hybrid bioactive heterocycles as potential antimicrobial agents: A review. Mini. Rev. Med. Chem. 16, 1500–1526. doi:10.2174/1389557516666160609075620
Djurišić, A. B., Chen, X., Leung, Y. H., and Ching Ng, A. M. (2012). ZnO nanostructures: Growth, properties and applications. J. Mat. Chem. 22, 6526–6535. doi:10.1039/C2JM15548F
Ebrahimi, Z., Davoodnia, A., Motavalizadehkakhky, A., and Mehrzad, J. (2020). Synthesis, characterization, and molecular structure investigation of new tetrahydrobenzo[b]thiophene-based schiff bases: A combined experimental and theoretical study. Eurasian Chem. Commun. 2, 170–180. doi:10.33945/SAMI/ECC.2020.2.2
Ezzatzadeh, E., Farjam, M. H., and Rustaiyan, A. (2012). Comparative evaluation of antioxidant and antimicrobial activity of crude extract and secondary metabolites isolated from Artemisia kulbadica. Asian pac. J. Trop. Dis. 2, S431–S434. doi:10.1016/S2222-1808(12)60198-4
Ezzatzadeh, E. (2018). Green synthesis of α-aminophosphonates using ZnO-nanoparticles as an efficient catalyst. Z. für Naturforsch. B 73, 179–184. doi:10.1515/znb-2017-0177
Ezzatzadeh, E., and Hossaini, Z. S. (2020a). 2D ZnO/Fe3O4 nanocomposites as a novel catalyst promoted green synthesis of novel quinazoline Phosphonate derivatives. Appl. Organomet. Chem. 34, e5596. doi:10.1002/aoc.5596
Ezzatzadeh, E., and Hossaini, Z. S. (2020b). A novel one-pot three-component synthesis ofbenzofuran derivatives via Strecker reaction: Study of antioxidant activity. Nat. Prod. Res. 34, 923–929. doi:10.1080/14786419.2018.1542389
Ezzatzadeh, E., and Hossaini, Z. S. (2019a). Four-component green synthesis of benzochromene derivatives using nano-KF/clinoptilolite as basic catalyst: Study of antioxidant activity. Mol. Divers. 24, 81–91. doi:10.1007/s11030-019-09935-6
Ezzatzadeh, E., and Hossaini, Z. S. (2019b). Green Synthesis and antioxidant activity of novel series of benzofurans from euparin extracted of Petasites hybridus. Nat. Prod. Res. 33, 1617–1623. doi:10.1080/14786419.2018.1428598
Fouad, M. M., El-Bendary, E. R., Suddek, G. M., Shehata, I. A., and El-Kerdawy, M. M. (2018). Synthesis and in vitro antitumor evaluation of some new thiophenes and thieno[2, 3-d] pyrimidine derivatives. Bioorg. Chem. 81, 587–598. doi:10.1016/j.bioorg.2018.09.022
Ghanaat, J., Khalilzadeh, M. A., and Zareyee, D. (2020). KF/CP NPs as an efficient nanocatalyst for the synthesis of 1, 2, 4-triazoles: Study of antioxidant and antimicrobial activity. Eurasian Chem. Commun. 2, 202–212. doi:10.33945/SAMI/ECC.2020.2.6
Goel, A., Agarwal, N., Singh, F. V., Sharon, A., Tiwari, P., Dixit, M., et al. (2004). Antihyperglycemic activity of 2-methyl-3, 4, 5-triaryl-1H-pyrroles in SLM and STZ models. Bioorg. Med. Chem. Lett. 14 (5), 1089–1092. doi:10.1016/j.bmcl.2004.01.009
Guo, Z., Liu, B., Zhang, Q., Deng, W., Wang, Y., and Yang, Y. (2014). Recent advances in heterogeneous selective oxidation catalysis for sustainable chemistry. Chem. Soc. Rev. 43, 3480–3524. doi:10.1039/C3CS60282F
Haddazadeh, E., and Mohammadi, M. K. (2020). One-pot synthesize of phenyl phenanthro imidazole derivatives catalyzed by Lewis acid in the presence of ammonium acetate. Chem. Methodol. 4, 324–332. doi:10.33945/SAMI/CHEMM/2020.3.9
Hajinasiri, R., Hossaini, Z. S., and Rostami-Charati, F. (2011). Efficient synthesis of α aminophosphonates via one pot reactions of aldehydes, amines, and phosphates in ionic liquid. Heteroat. Chem. 22, 625–629. doi:10.1002/hc.20724
Hakimi, F., Fallah-Mehrjardi, M., and Golrasan, E. (2020). Yttrium aluminum garnet (YAG: Al5Y3O12) as an efficient catalyst for the synthesis of benzimidazole and benzoxazole derivatives. Chem. Methodol. 4, 234–244. doi:10.33945/SAMI/CHEMM/2020.3.2
Halliwell, B. (1999). Antioxidant defence mechanisms: From the beginning to the end (of the beginning). Free Radic. Res. 31, 261–272. doi:10.1080/10715769900300841
Herrera, R. P., and Marqués-López, E. (2015). Multicomponent reactions: Concepts and applications for design and synthesis. Hoboken: Wiley.
Hossaini, Z. S., Sheikholeslami-Farahani, F., and Rostami-Charati, F. (2014). Green synthesis of phosphoryl-2-oxo-2H-pyran via three component reaction of trialkyl phosphites. Comb. Chem. High. Throughput Screen. 17, 804–807. doi:10.2174/1386207317666141106155038
Hossaini, Z. S., Zareyee, D., Sheikholeslami-Farahani, F., Vaseghi, S., and Zamani, A. (2017). ZnO-NR as the efficient catalyst for the synthesis of new thiazole and cyclopentadienone phosphonate derivatives in water. Heteroat. Chem. 28, e21362. doi:10.1002/hc.21362
Ibarra, I. A., Islas-Jácome, A., and González-Zamora, E. (2018). Synthesis of polyheterocycles via multicomponent reactions. Org. Biomol. Chem. 16, 1402–1418. doi:10.1039/C7OB02305G
Jabłonska, M., and Palkovits, R. (2016). Nitrogen oxide removal over hydrotalcite-derived mixed metal oxides. Catal. Sci. Technol. 6, 49–72. doi:10.1039/C5CY00646E
Janitabar-Darzi, S., and Abdolmohammadi, S. (2019). TiO2-SiO2 nanocomposite as a highly efficient catalyst for the solvent-free cyclocondensation reaction of isatins, cyclohexanones, and urea. Z. für Naturforsch. B 74, 559–564. doi:10.1515/znb-2019-0059
Kalantari, E., Khalilzadeh, M. A., Zareyee, D., and Shokouhimehr, M. (2020). Catalytic degradation of organic dyes using green synthesized Fe3O4-cellulose-copper nanocomposites. J. Mol. Struct. 1218, 128488. doi:10.1016/j.molstruc.2020.128488
Kalaria, P. N., Karad, S. C., and Raval, D. K. (2018). A review on diverse heterocyclic compounds as the privileged scaffolds in antimalarial drug discovery. Eur. J. Med. Chem. 158, 917–936. doi:10.1016/j.ejmech.2018.08.040
Kamali, F., and Shirini, F. (2021). Effective and convenient synthesis of 2-amino-4H-chromenes promoted by melamine as a recyclable organocatalyst. Eurasian Chem. Commun. 3, 278–290. doi:10.22034/ecc.2021.272326.1136
Karimi, A. H., Hekmat-Ara, A., Zare, A., Barzegar, M., Khanivar, R., and Sadeghi-Takallo, M. (2021). Producing, characterizing and utilizing a novel magnetic catalyst to promote construction of N, N′-alkylidene bisamides. Eurasian Chem. Commun. 3, 360–368. doi:10.22034/ecc.2021.277415.1149
Khalilzadeh, M. A., Hosseini, S., Rad, A. S., and Venditti, R. A. (2021). Synthesis of grafted nanofibrillated cellulose-based hydrogel and study of its thermodynamic, kinetic, and electronic properties. J. Agric. Food Chem. 68, 8710–8719. doi:10.1021/acs.jafc.0c03500
Khattab, T. A., and Rehan, M. A. (2018). A review on synthesis of nitrogen-containing heterocyclic dyes for textile fibers - Part 2: Fused heterocycles. Egypt. J. Chem. 61, 0–1018. doi:10.21608/ejchem.2018.4131.1363
Khazaei, A., Gohari-Ghalil, F., Tavasoli, M., Rezaei-Gohar, M., and Moosavi-Zare, A. R. (2020). Fe3O4 bonded pyridinium-3-carboxylic acid-N-sulfonic acid chloride as an efficient catalyst for the synthesis of 3, 4-dihydropyrimidin-2(1H)-ones. Chem. Methodol. 4, 543–553. doi:10.22034/chemm.2020.106433
Kumar, B., Babu, N. J., and Chowhan, R. L. (2022). Sustainable synthesis of highly diastereoselective & fluorescent active spirooxindoles catalyzed by copper oxide nanoparticle immobilized on microcrystalline cellulose. Appl. Organomet. Chem. 36 (7), e6742. doi:10.1002/aoc.6742
Lamberth, C., and Dinges, J. (2012). Bioactive heterocyclic compound classes: Agrochemicals. KGaA: Wiley-VCH Verlag GmbH & Co.
Li, W., Zhao, S. J., Gao, F., Lv, Z. S., Tu, J. Y., and Xu, Z. (2018). Synthesis and in vitro anti-tumor, anti-mycobacterial and anti-HIV activities of diethylene-glycol-tethered bis-isatin derivatives. ChemistrySelect 3, 10250–10254. doi:10.1002/slct.201802185
Lin-Bing, S., Xiao-Qin, L., and Hong-Cai, Z. (2015). Design and fabrication of mesoporous heterogeneous basic catalysts. Chem. Soc. Rev. 44, 5092–5147. doi:10.1039/C5CS00090D
Liu, L., and Meydani, M. (2002). Combined vitamin C and E supplementation retards early progression of arteriosclerosis in heart transplant patients. Nutr. Rev. 60, 368–371. doi:10.1301/00296640260385810
Mandel, K., Hutter, F., Gellermann, C., and Sextl, G. (2013). Reusable superparamagnetic nanocomposite particles for magnetic separation of iron hydroxide precipitates to remove and recover heavy metal ions from aqueous solutions. Sep. Purif. Technol. 109, 144–147. doi:10.1016/j.seppur.2013.03.002
Martins, P., Jesus, J., Santos, S., Raposo, L. R., Roma-Rodrigues, C., Baptista, P. V., et al. (2015). Heterocyclic anticancer compounds: Recent advances and the paradigm shift towards the use of nanomedicine's tool box. Molecules 20, 16852–16891. doi:10.3390/molecules200916852
Mohammed Abd Al-Mohson, Z. (2021). Synthesis of novel pyrazole derivatives containing tetrahydrocarbazole, antimicrobail evaluation and molecular properties. Eurasian Chem. Commun. 3, 425–434. doi:10.22034/ecc.2021.284257.1173
Mostaghni, F., and Taat, F. (2020). CoFe2O4 as green and efficient catalyst for synthesis of multisubstituted imidazoles. Eurasian Chem. Commun. 2, 427–432. doi:10.33945/SAMI/ECC.2020.4.1
Nikpassand, M., and Zare Fekri, L. (2020). Catalyst-free synthesis of mono and bis spiro pyrazolopyridines in DSDABCO as a novel media. Chem. Methodol. 4, 437–446. doi:10.33945/SAMI/CHEMM.2020.4.6
Rajabi, M., Hossaini, Z. S., Khalilzadeh, M. A., Datta, Sh., Halder, M., and Mousa, Sh. A. (2015). Synthesis of a new class of furo[3, 2-C]coumarins and its anticancer activity. J. Photochem. Photobiol. B Biol. 148, 66–72. doi:10.1016/j.jphotobiol.2015.03.027
Rajendran, S. P., and Sengodan, K. (2017). Synthesis and characterization of zinc oxide and iron oxide nanoparticles using Sesbania grandiflora leaf extract as reducing agent. J. Nanosci. 2017, 1–7. doi:10.1155/2017/8348507
Raoufi, F., Aghaei, H., and Ghaedi, M. (2020). Cu-metformin grafted on multi walled carbon nanotubes: Preparation and investigation of catalytic activity. Eurasian Chem. Commun. 2, 226–233. doi:10.33945/SAMI/ECC.2020.2.8
Rezayati, S., Hajinasiri, R., Hossaini, Z. S., and Abbaspour, S. (2018). Chemoselective synthesis of 1, 1-diacetates (acylals) using 1, 1'‐ butylenebispyridinium hydrogen sulfate as a new, halogen‐free and environmental-friendly catalyst under solvent-freeconditions. Asian J. Green Chem. 2, 268–280. doi:10.22034/ajgc.2018.61810
Rezayati, S., Sheikholeslami-Farahani, F., Hossaini, Z. S., Hajinasiri, R., and Afshari Sharif Abad, S. (2016). Regioselctive thiocyanation of aromatic and heteroaromatic compounds using a novel bronsted acidic ionic liquid. Comb. Chem. High. Throughput Screen. 19 (9), 720–727. doi:10.2174/1386207319666160709191851
Rohaniyan, M., Davoodnia, A., and Beyramabadi, S. A. (2020). Successful application of a keplerate-type, giant-ball nanoporous isopolyoxomolybdate as a reusable green catalyst for atom-economy synthesis of tetrahydrobenzo[a]xanthene-11-ones. Chem. Methodol. 4, 285–296. doi:10.33945/SAMI/CHEMM/2020.3.6
Rostami Charati, F., Hossaini, Z. S., and Hosseini-Tabatabaei, M. R. (2011). A simple synthesis of oxaphospholes. Phosphorus Sulfur Silicon Relat. Elem 186, 1443–1448. doi:10.1080/10426507.2010.515953
Rostami-Charati, F., Hossaini, Z. S., Rostamian, R., Zamani, A., and Abdoli, M. (2017a). Green synthesis of indol-2-one derivatives from N-alkylisatins in the presence of KF/clinoptilolite nanoparticles. Chem. Heterocycl. Comp. 53, 480–483. doi:10.1007/s10593-017-2077-x
Rostami-Charati, F., Hossaini, Z. S., Sheikholeslami-Farahani, F., Azizi, Z., and Siadati, S. A. (2015). Synthesis of 9H-furo [2, 3-f] chromene derivatives by promoting ZnO nanoparticles. Comb. Chem. High. Throughput Screen. 18 (8), 872–880. doi:10.2174/1386207318666150525094109
Rostami-charati, F., Hossaini, Z. S., Zareyee, D., Afrashteh, S., and Hosseinzadeh, M. (2017b). ZnO‐nanorods as an efficient catalyst for the synthesis of 1, 3‐thiazolidine derivatives by aqueous multicomponent reactions of isothiocyanates. J. Heterocycl. Chem. 54, 1937–1942. doi:10.1002/jhet.2789
Rustaiyan, A., and Ezzatzadeh, E. (2011). Sesquiterpene lactones and penta methoxylated flavone from Artemisia kulbadica. Asian J. Chem. 23, 1774–1776.
Sahay, R., Sundaramurthy, J., Suresh Kumar, P., Thavasi, V., Mhaisalkar, S. G., and Ramakrishna, S. (2012). Synthesis and characterization of CuO nanofibers, and investigation for its suitability as blocking layer in ZnO NPs based dye sensitized solar cell and as photocatalyst in organic dye degradation. J. Solid State Chem. 186, 261–267. doi:10.1016/j.jssc.2011.12.013
Sajjadi-Ghotbabadi, H., Javanshir, S. H., and Rostami-Charati, F. (2016). Nano KF/clinoptilolite: An effective heterogeneous base nanocatalyst for synthesis of substituted quinolines in water. Catal. Lett. 146, 338–344. doi:10.1007/s10562-015-1652-y
Sajjadifar, S., Amini, I., Mansouri, G., and Alimohammadi, S. (2020). Fe3O4@APTES@isatin-SO3H as heterogeneous and efficient catalyst for the synthesis of quinoxaline derivatives. Eurasian Chem. Commun. 2, 626–633. doi:10.33945/SAMI/ECC.2020.5.9
Salih, A. R., and Al-Messri, Z. A. K. (2021). Synthesis of pyranopyrazole and pyranopyrimidine derivatives using magnesium oxide nanoparticles and evaluation as corrosion inhibitors for lubricants. Eurasian Chem. Commun. 3, 533–541. doi:10.22034/ecc.2021.291144.1189
Saundane, A. R., and Nandibeoor, M. K. (2015). Synthesis, characterization, and biological evaluation of Schiff bases containing indole moiety and their derivatives. Monatsh. Chem. 146, 1751–1761. doi:10.1007/s00706-015-1440-9
Seifi Mansour, S., Ezzatzadeh, E., and Safarkar, R. (2019). In vitro evaluation of its antimicrobial effect of the synthesized Fe3O4 nanoparticles using Persea Americana extract as a green approach on two standard strains. Asian J. Green Chem. 3, 353–365. doi:10.22034/ajgc.2018.154682.1113
Shi, J. (2013). On the synergetic catalytic effect in heterogeneous nanocomposite catalysts. Chem. Rev. 113, 2139–2181. doi:10.1021/cr3002752
Shimada, K., Fujikawa, K., Yahara, K., and Nakamura, T. (1992). Antioxidative properties of xanthan on the autoxidation of soybean oil in cyclodextrin emulsion. J. Agric. Food Chem. 40, 945–948. doi:10.1021/jf00018a005
Siddiqui, N., AndalipBawa, S., Ali, R., Afzal, O., Akhtar, M. J., Kumar, R., et al. (2011). Antidepressant potential of nitrogen-containing heterocyclic moieties: An updated review. J. Pharm. Bioallied Sci. 3, 194–212. doi:10.4103/0975-7406.80765
Sokolova, A. S., Yarovaya, O. I., Bormotov, N. I., Shishkina, L. N., and Salakhutdinov, N. F. (2018). Synthesis and antiviral activity of camphor-based 1, 3-thiazolidin-4-one and thiazole derivatives as Orthopoxvirus-reproduction inhibitors. Medchemcomm 9, 1746–1753. doi:10.1039/C8MD00347E
Taran, J., Ramazani, A., and Atrak, K. (2020). Silica nanoparticles as highly efficient catalyst for the one-pot synthesis of α-aminophosphonate derivatives from primary amines, quinoline-4-carbaldehyde and phosphite under solvent-free conditions. Eurasian Chem. Commun. 2, 257–264. doi:10.33945/SAMI/ECC.2020.2.11
Tavakolinia, F., Baghipour, T., Hossaini, Z. S., Zareyee, D., Khalilzadeh, M. A., and Rajabi, M. (2012). Antiproliferative activity of novel thiopyran analogs on MCF-7 breast and HCT-15 colon cancer cells: Synthesis, cytotoxicity, cell cycle analysis, and DNA-binding. Nucleic Acid. Ther. 22, 265–270. doi:10.1089/nat.2012.0346
Tayebee, R., and Gohari, A. (2020). The dual role of ammonium acetate as reagent and catalyst in the synthesis of 2, 4, 5-triaryl-1H-imidazoles. Eurasian Chem. Commun. 2, 581–586. doi:10.33945/SAMI/ECC.2020.5.3
Tietze, L. F., Bsasche, C., and Gericke, K. M. (2006). Domino reactions in organic synthesis. Weinheim: Wiley VCH.
Wachs, I. E. (2005). Recent conceptual advances in the catalysis science of mixed metal oxide catalytic materials. Catal. Today 100, 79–94. doi:10.1016/j.cattod.2004.12.019
Weber, L., Illgen, K., and Almstetter, M. (1999). Discovery of new multi component reactions with combinatorial methods. Synlett 3, 366–374. doi:10.1055/s-1999-2612
Yavari, I., Hossaini, Z. S., Sabbaghan, M., and Ghazanfarpour-Darjani, M. (2007). Efficient synthesis of functionalized spiro-2, 5-dihydro-1, 2-λ5-oxaphospholes. Tetrahedron 63, 9423–9428. doi:10.1016/j.tet.2007.06.102
Yavari, I., Hossaini, Z. S., Souri, S., and Seyfi, S. (2009). Diastereoselective synthesis of fused [1, 3]thiazolo [1, 3] oxazins and [1, 3] oxazino [2, 3-b] [1, 3] benzothiazoles. Mol. Divers. 13, 439–443. doi:10.1007/s11030-009-9128-x
Yavari, I., Nematpour, M., and Hossaini, Z. S. (2010). Ph3P-mediated one-pot synthesis of functionalized 3, 4-dihydro-2H-1, 3-thiazines from N, N′-dialkylthioureas and activated acetylenes in water. Monatsh. Chem. 141, 229–232. doi:10.1007/s00706-009-0247-y
Yavari, I., Sabbaghan, M., and Hossaini, Z. S. (2008). Proline-promoted efficient synthesis of 4-aryl-3, 4-dihydro-2h, 5h-pyrano[3, 2-c]chromene-2, 5-diones in aqueous media. Synlett 8, 1153–1154. doi:10.1055/s-2008-1072656
Yen, G. C., and Duh, P. D. (1994). Scavenging effect of methanolic extracts of peanut hulls on free-radical and active-oxygen species. J. Agric. Food Chem. 42, 629–632. doi:10.1021/jf00039a005
Yildirim, A., Mavi, A., and Kara, A. A. (2001). Determination of antioxidant and antimicrobial activities of Rumex crispus L. extracts. J. Agric. Food Chem. 49, 4083–4089. doi:10.1021/jf0103572
Zhang, B.-T., Zheng, X., Li, H.-F., and Lin, J.-M. (2013). Application of carbon-based nanomaterials in sample preparation: A review. Anal. Chim. Acta X. 784, 1–17. doi:10.1016/j.aca.2013.03.054
Zhang, Q., Vigier, K. D. V., Royer, S., and Jerome, F. (2012). Deep eutectic solvents: Syntheses, properties and applications. Chem. Soc. Rev. 41, 7108–7146. doi:10.1039/C2CS35178A
Zhao, X., Chaudhry, S. T., and Mei, J. (2017). Heterocyclic building blocks for organic semiconductors. Heterocycl. Chem. 21st Century a Tribute Alan Katritzky 121, 133–171. doi:10.1016/bs.aihch.2016.04.009
Zhao, Y., Xu, L., Yang, Ch., Chen, T., and Yu, L. (2019). Design and preparation of magnetic mesoporous melamine–formaldehyde resin: A novel material for pre-concentration and determination of silver. Appl. Organomet. Chem. 33, e5112. doi:10.1002/aoc.5112
Keywords: Ag/Fe3O4/CdO@MWCNTs MNCs, spiro-1,2,4-triazines, antioxidant activity, ninhydrin, isatin, acenaphthene
Citation: Ezzatzadeh E, Soleimani-Amiri S, Hossaini Z and Khandan Barani K (2022) Synthesis and evaluation of the antioxidant activity of new spiro-1,2,4-triazine derivatives applying Ag/Fe3O4/CdO@MWCNT MNCs as efficient organometallic nanocatalysts. Front. Chem. 10:1001707. doi: 10.3389/fchem.2022.1001707
Received: 23 July 2022; Accepted: 02 September 2022;
Published: 29 September 2022.
Edited by:
Pezhman Shiri, Shiraz University of Medical Sciences, IranReviewed by:
L. Raju Chowhan, Central University of Gujarat, IndiaBubun Banerjee, Akal University, India
Shahrzad Abdolmohammadi, Islamic Azad University South Tehran Branch, Iran
Faramarz Rostami Charati, Research institute of cultural Heritage and Tourism, Iran
Copyright © 2022 Ezzatzadeh, Soleimani-Amiri, Hossaini and Khandan Barani. This is an open-access article distributed under the terms of the Creative Commons Attribution License (CC BY). The use, distribution or reproduction in other forums is permitted, provided the original author(s) and the copyright owner(s) are credited and that the original publication in this journal is cited, in accordance with accepted academic practice. No use, distribution or reproduction is permitted which does not comply with these terms.
*Correspondence: Elham Ezzatzadeh, dr.ezzatzadeh@yahoo.com