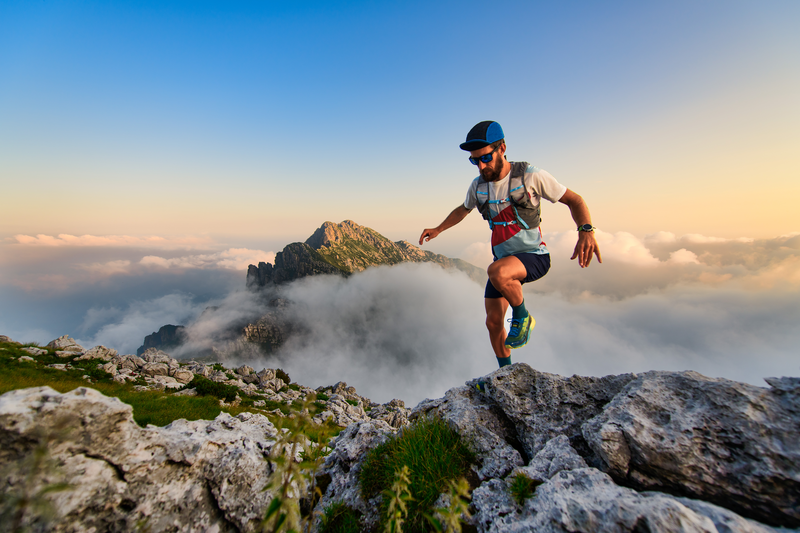
95% of researchers rate our articles as excellent or good
Learn more about the work of our research integrity team to safeguard the quality of each article we publish.
Find out more
ORIGINAL RESEARCH article
Front. Chem. , 21 January 2022
Sec. Green and Sustainable Chemistry
Volume 9 - 2021 | https://doi.org/10.3389/fchem.2021.826625
This article is part of the Research Topic Biomass Resources Utilization View all 9 articles
Lignin-carbohydrate complexes (LCCs) in the plant cell wall are responsible for providing resistance against biomass-degrading enzymes produced by microorganisms. Four major types of lignin-carbohydrate bonds are reported in the literature, namely, benzyl ethers, benzyl esters, phenyl glycosides, and acetyl ester linkages. Ester’s linkages in the plant cell wall are labile to alkaline pretreatments, such as ammonia fiber expansion (AFEX), which uses liquid or gaseous ammonia to cleave those linkages in the plant cell wall and reduce biomass recalcitrance. Two competing reactions, notably hydrolysis and ammonolysis, take place during AFEX pretreatment process, producing different aliphatic and aromatic acids, as well as their amide counterparts. AFEX pretreated grasses and agricultural residues are known to increase conversion of biomass to sugars by four- to five-fold when subjected to commercial enzyme hydrolysis, yielding a sustainable feedstock for producing biofuels, biomaterials, and animal feed. Animal feed trials on dairy cows have demonstrated a 27% increase in milk production when compared to a control feedstock. However, the presence of carboxamides in feedstocks could promote neurotoxicity in animals if consumed beyond a certain concentration. Thus, there is the need to overcome regulatory hurdles associated with commercializing AFEX pretreated biomass as animal feed in the United States. This manuscript demonstrates a modified pretreatment for increasing the digestibility of industrial byproducts such as Brewer’s spent grains (BSG) and high-fiber meal (HFM) produced from BSG and dry distillers grains with soluble (DDGS), while avoiding the production of carboxamides. The three industrial byproducts were first treated with calculated amounts of alkali such as NaOH, Ca(OH)2, or KOH followed by AFEX pretreatment. We found that 4% alkali was able to de-esterify BSG and DDGS more efficiently than using 2% alkali at both 10 and 20% solids loading. AFEX pretreatment of de-esterified BSG, HFM, and DDGS produced twofold higher glucan conversion than respective untreated biomass. This new discovery can help overcome potential regulatory issues associated with the presence of carboxamides in ammonia-pretreated animal feeds and is expected to benefit several farmers around the world.
Industrial lignocellulosic biomass byproducts such as Brewer’s spent grains (BSG) and high-fiber meal (HFM) produced in distilleries and dry distillers grains and soluble (DDGS) produced in corn ethanol plant are sustainable feedstocks for producing fuels, chemicals, biomaterials, and animal feed. Both BSG and DDGS are extensively used as ruminant animal feed. BSG contain a significant quantity of Tannins that are reported to interfere in the absorption of essential nutrients, amino acids, and digestive enzymes, thereby reducing feed utilization (Liu, 2011; Westendorf et al., 2014; Oryschak et al., 2010a; Oryschak et al., 2010b; Choct et al., 2010; Lacassagn et al., 1998). As the quantity of BSG in the diet increased, the feed conversion ratio decreased (Alabi et al., 2014; Ashour et al., 2019). Extensively using lignocellulosic biomass as animal feed will help displace grains, such as corn, soybean, and sorghum, and reduce global hunger (Salami et al., 2019). Lignocellulosic biomass recalcitrance has been attributed to high lignin content, acetylated hemicellulose, and highly crystalline cellulose (Marriott et al., 2016). Hence, using untreated lignocellulosic biomass as feedstock provides low metabolizable energy to animals. Four types of chemical linkages are reported in the literature between lignin and hemicellulose that form a lignin-carbohydrate complex (LCC). They include benzyl ethers, benzyl esters, phenyl glycoside, and acetyl linkages (Balakshin et al., 2011). Some of the ester linkages include monomeric p-coumarate (pCA) and ferulate-linkages on lignin, glucuronoarabinoxylan (GAX), eight de-hydro-diferulic acids (DFA) resulting from five possible coupling modes (8-5′-, 8-O-4′-, 8-8′-, 5-5′-, and 4-O-5′-coupling) connecting glucuronoarabinoxylan (GAX) and GAX-hemicellulose sugar polymer, monomeric ferulate connecting lignin to GAX, and acetylated sugars (Hatfield et al., 1999; Bunzel et al., 2004; Mnich et al., 2020). Particularly, pCA is mostly acylated to the lignin polymer at the γ-carbon of the side chain region, particularly in syringyl moieties and to a lesser extent on arabinosyl side chains of arabinoxylan (Hatfield et al., 2008). These linkages make the lignocellulosic biomass highly recalcitrant and less digestible in ruminant animals.
Several acids- and alkali-catalyzed pretreatment methods are widely used to produce highly digestible ruminant animal feed (Falls et al., 2017; Zhao et al., 2018; Liang et al., 2020). Chemical catalyzed pretreatment processes help open the forage plant cell wall, reduce the recalcitrance, and improve the digestibility of the feed. Some of the prominent pretreatments include dilute acid, NaOH or KOH catalyzed H2O2, ionic liquids, dilute acid or base catalyzed steam explosion, liquid hot water, CO2 explosion, organosolv, ammonia percolation, and lime pretreatment (Baruah et al., 2018). However, most of the catalyst used in pretreatment process is miscible in water and get embedded into the biomass, requiring expensive processing step to remove or neutralize them after pretreatment. Ammonia fiber expansion (AFEX) is one of the leading alkaline pretreatments processes that uses volatile ammonia as catalyst and up to 97% of ammonia can be recycled and used for subsequent processing cycle (da Costa Sousa et al., 2009; Balan et al., 2009a; Balan et al., 2011; Chundawat et al., 2011; da Costa Sousa et al., 2016; Balan et al., 2017; Bals et al., 2019; Mor et al., 2019; O Ribeiro et al., 2020; Xu et al., 2020). AFEX pretreatment of various feedstocks such as rice and wheat straw, corn stover, and oat hull followed by densification and drying helped produce dry biomass pellets (<10% moisture) with increased bulk density (588–634 kg/m3) like shelled corn grains (720 kg/m3) and offered the advantage to transportation and store biomass in grain silos (Hoover et al., 2014). Microorganisms in ruminant animals (dairy and beef cows, sheep, goats, buffalo, horse, donkey, mule, and camels) in the gut are capable of efficiently hydrolyzing the cellulose and hemicellulose in the AFEX pretreated lignocellulosic biomass and provide energy to animals (Mokomele et al., 2018). Some of the recently concluded AFEX pretreated densified wheat straw as animal feed trials, especially dairy cows and buffaloes has demonstrated increased metabolizable energy and increased milk production as high as 27% (Mor et al., 2018; Beauchemin et al., 2019; O Ribeiro et al., 2020). Pretreatments of monocot (e.g., corn stover, forage sorghum, rice straw, sugarcane bagasse, and perennial grasses such as miscanthus) and dicot (e.g., poplar hard wood, agave, and alfaalfa) feed stocks using AFEX have also shown higher sugar conversion like AFEX-treated corn stover when subjected to commercial biomass-degrading enzyme hydrolysis and are expected to be excellent animal feed (Balan et al., 2011; Balan et al., 2012) (Table 1).
The majority of the ester linkages in lignocellulosic biomass are cleaved by ammonia catalyst during AFEX pretreatment (Balan et al., 2009b). Two competing reactions take place: 1) hydroxide ion (generated from water) attacks ester linkages to produce hydrolysis products such as acetic acid, ferulic acid, and coumaric acid and several di-ferulate di-acids and 2) ammonia attacks ester linkages to produce ammonolysis products, for example, monoamides such as acetamide, feruloylamide, coumaroyl amide, and several di-ferulate mono- and di-amides (Chundawat et al., 2010; Vismeh et al., 2018; Chundawat et al., 2020).
R-CO-OR’ + OH− R’OH + R-CO-O− (hydrolysis reaction)
R-CO-OR’ + NH3 R’OH + R-CO-NH2 (ammonolysis reaction)
Similar cleavages of ester linkages are reported when lignocellulosic biomass is pretreated using dilute acid or dilute alkali (Chen et al., 2012; Saulnier et al., 2020). The carboxamides produced during ammonia pretreatment of forages are considered as potential feed carcinogens when consumed beyond a certain concentration, and there is widespread fear of these compounds entering into the human food chain (Chundawat et al., 2010; Vismeh et al., 2018Mokomele et al., 2018; Bals et al., 2019; Moore et al., 2019). For example, acetamide (CAS60-35-5) is classified as one of the possible neurotoxins (IARC Casa Grop-2B) following chronic exposures at high dosage. In vitro lab studies have shown that the amides are reported to inhibit microorganisms such as yeast (Tang et al., 2015; Xue et al., 2018). Literature reports show that acetamide is generally of low acute oral toxicity in rodents. However, high doses of acetamide affect the central nervous system, liver, kidneys, spleen, and lungs (Moore et al., 2019; Nault et al., 2020).
To overcome the formation of carboxamides during AFEX pretreatment, we have developed a two-step pretreatment process for the first time that will help overcome the bottleneck of using them as animal feed (Balan and Sousa, 2019). Three different industrial lignocellulosic biomass byproducts, namely, BSG, HFM, and DDGS have been used in this study. We, first, calculated the amount of alkali such as NaOH and Ca(OH)2 that are needed to de-esterify ester linkages in lignocellulosic biomass. The right quantity of alkali is mixed with water, evenly added to lignocellulosic biomass, and heated up to 100°C for a fixed residence time. The presence of alkali solution on biomass facilitated the cleavage of ester linkages to produce corresponding organic acids. Subsequently, ammonia was loaded to the de-esterified biomass with appropriate moisture in a high-pressure reactor to carry out the AFEX process. Ammonia helps catalyze the cleavage of LCCs and solubilize larger portions of lignin and hemicelluloses, which are displaced to the surface of biomass when ammonia is released from the high-pressure reactor (Balan et al., 2009b; Chundawat et al., 2011). The two-step pretreatment process for BSG, HFM, and DDGS yielded no significant increase in carboxamide content relative to the untreated materials and showed a comparable digestibility to that of the one-step AFEX pretreated biomass when subjected to commercial biomass-degrading enzymes. This modified pretreatment procedure will help produce highly digestible, safe, and sustainable animal feed, benefiting several farmers around the world.
The DDGS was collected from Big River Resources, LLC (West Burlington, IA), and stored at ambient temperature until it was used. The composition of DDGS biomass on a dry weight basis was determined using the NREL protocol (Sluiter et al., 2010). It contains glucan (∼21%), xylan (∼8.2%), and protein (∼25.6%). The BSG was obtained from Anheuser-Busch Inc. Brewery Plant St. Louis, MO, with the help of our industrial partner Trucent, Dexter, MI. BSC was oven-dried at 60°C until the moisture content was less than 8% and stored at room temperature. The composition of BSG on a dry weight basis was found to contain glucan (14.7%), xylan (16.8%), arabinan (7%), and protein (34.3%) using the NREL protocol. High-fiber meal (HFM) was separated from BSG using the reported protocol (He et al., 2019). Briefly, the method entailed using BSG at 5% (w/w) solids was mixed with pure water and incubated at 60°C for 4 h to solubilize protein. Then, the slurry was transferred to a sieve shaker to separate the fiber from soluble protein. The retentate, the fibrous portion of BSG left behind on the sieve, is called HFM and dried at 60°C in a convection oven for 24 h until the moisture content was less than 8% and stored at room temperature. The composition of HFM on a dry weight basis was found to contain glucan (18.1%), xylan (23.8%), arabinan (9.6%), and protein (18.3%).
AFEX pretreatment was carried out using previously reported protocol without any extraction (Dale et al., 1985; Balan et al., 2009a). The moisture content of BSG/HFM/DDGS was increased to 40% by spraying water, followed by loading into a high-pressure tubular reactor. The moisture content of the samples was measured using an A&D moisture analyzer fitted with an IR lamp. The reactor was first closed and degassed to remove any air from the reactor, and a known amount of liquid ammonia was loaded to BSG or DDGS at 1:1 ratio and headed by an external heater until the temperature in the reactor reached 100°C. Once the desired temperature was reached, the pretreatment was continued for another 30 min, before the ammonia was vented from the top. AFEX treated samples were transferred to aluminum trays and dried in a fume hood overnight to remove residual ammonia and moisture. The samples were then stored in zip-lock bags in a refrigerator. The total nitrogen content of the biomass was measured by the Kjeldahl method using the NREL protocol (Hames et al., 2008).
BSG, HFM, and DDGS was pretreated using 3% aqueous ammonium hydroxide at 9% solids loading for 6 h at 60°C (Dien et al., 2013; Le et al., 2021). Then, the pretreated biomass was filtered using the Buchner funnel, and the sample was dried in the fume hood overnight to remove residual ammonia.
To simulate biomass digestibility experiments by rumen in the gut, we carried out enzymatic hydrolysis at 3% solids loading. The total reaction volume was 50 ml, and experiments were carried out in a 125 ml flask (Mokomele et al., 2018). Experiments were carried out in an orbital shaker incubator set at 200 rpm at 50°C for 24 h. About 3 mg of commercial enzyme cocktail was added in the ratios 80:15:5 (Ctec2: Htec2: Pectinex). All the commercial enzymes used in this experiment were provided by Novozymes. About 1 ml of hydrolyzed samples was heated at 100°C for 10 min, cooled down on ice, and passed through a 0.2 micro syringe nylon filter before analyzing the sample using HPLC using Biorad Aminex 80P column to estimate the glucose and xylose conversion (Kim et al., 2008). Mixed sugar comprising glucose and xylose at varying concentration was used to construct the standard curve (Ruiz and Ehrman 1996).
In this study, de-esterification of BSG or DDGS was carried out using mild alkali such as Ca(OH)2 and strong alkali such as sodium hydroxide (NaOH). The alkali concentration was varied between 2 and 4%, while the DDGS or BSG solid loadings were maintained as 10 or 20%. The samples were heated at 80°C for 15 min and filtered using a mirror cloth. The alkali-treated BSG, HFM, and BSG samples were dried in the hood at room temperature to reduce the moisture content to 60%. The de-esterified biomass was subjected to AFEX pretreatment using 1:1 ammonia to biomass loading, 100°C for 15 min residence time. The pretreated biomass was collected in the tray and kept in the hood overnight until most of residual ammonia is removed.
Acetamide was quantified using an established protocol using GC-MS without any derivatization (Vismeh et al., 2018). Briefly, acetamide was extracted using water as a solvent in an ASE 200 Accelerated Solvent Extractor (Dionex) at approximately 11 ml of water per gram sample for two cycles. About 1.0 µL of extracts of untreated and pretreated lignocellulose biomass (HFM, BSG, DDGS) was injected into a DB-WAX column (Agilent, Santa Clara, CA) with dimension 30 × 0.25 mm i.d., 0.25 μm using helium as carrier gas at 1.5 ml/min and injector temperature of 240°C. Mass spectra (70 eV electron ionization) were collected in full scan mode (m/z 30–400). The column temperature was programmed as follows: initial column temperature at 50°C and holding for 1 min; and increasing to 250°C at 15°C/min and holding for 2 min. The total run time for each sample was 16.3 min/sample. The analytes were quantified using extracted ion chromatograms of molecular ions m/z 59 and 62 for acetamide and acetamide-d3 (internal standard), respectively.
Most industrial lignocellulosic byproducts are comprised of cellulose, hemicellulose, lignin, and protein. The composition of these materials depends on how the feedstocks are processed. Naturally, these lignocellulosic biomasses are recalcitrant due to the complex network of different components and crystallinity of cellulose (Figure 1). BSG is a byproduct stream of the beer brewing process and comprises the extracted residue of barley malt alone or mixed with other cereal grains (Westendorf and Wohlt, 2002). Modern large-scale breweries generate approximately 20 kg of wet BSG per hectoliter of beer. A large-scale North American brewery will produce over 100,000 tons of wet BSG per year, and more than three million tons of BSG/year are available in the US alone. Wet BSG coming out of the brewery has a short shelf life and is therefore immediately sold to nearby dairy or cattle farms. Simply drying the BSG will increase the shelf life and make it suitable for sale in more distant markets, but the drying process is expensive (Aliyu and Bala, 2011). Therefore, the brewing industry seeks means to enhance the value of BSG beyond just a dried product. It is posited that enhanced value can be achieved by creating nutritionally rich fractions from the BSG, which are then sold into targeted species and life cycle-specific markets.
FIGURE 1. Ultrastructure of plant cell wall comprising cellulose, hemicellulose, lignin, protein, and ash in different ratios. Detailed structure of lignin monomers. Three different types of lignocellulosic biomass, namely, dry distillers grains with soluble (DDGS), high-fiber meal (HFM), and Brewer’s spent grains (BSG), used to produce de-esterified biomass without carboxamides are given.
BSG can be mechanically separated into a high protein meal (HPM) and a high-fiber meal (HFM), representing approximately 35 and 65% of BSG dry matter, respectively (Kishi et al., 1991). Preliminary economic analysis shows that dry HPM and HFM can give incremental profit improvement relative to wet BSG (Buffington 2014). However, the economics can be made even more compelling by further upgrading BSG or its fractions for specific animal nutrition markets.
Several past research and review articles provide a wealth of knowledge about BSG composition, feeding trials and nutrition value to ruminant and non-ruminant animals, and new methods of upgrading BSG into higher-value products, including human food and protein concentrate (Mussatto et al., 2006; Celus et al., 2009; Lynch et al., 2016; Chetrariu and Dabija 2020; Kavalopoulos et al., 2021; Marcus and Fox 2021). BSG also have sizable lignin (cross-linked phenolic polymers) and tannins (with basic units like gallic acid and flavone). The energy content of BSG varied from different sources and types of grain used in a particular brewery. Some of the animal nutrition parameters for BSG include total digestible nutrients (TDN), 71–76%; net energy for lactation (NEL), 0.73–0.82 mcal/lb; net energy for maintenance (NEM), 0.79–0.88 mcal/lb; and net energy for gain (NEG) 0.51–0.58 mcal/lb (Santosa et al., 2003). Feeding wet BSG to animals increased the risk of mycotoxins in their diet, while heat processing was proven to degrade mycotoxin (Schwarz et al., 2018). BSG amino acid profile analysis showed that lysine and threonine are the first two limiting amino acids. High temperature (>190°C) used during dilute acid or alkali-catalyzed steam explosion producing undesirable inhibitory products that inhibit microbes during the digestion process also affects the solubility and stability of proteins (Kemppainen et al., 2016). Adding feed enzymes such as xylanase increased the nutrient availability to animals (Beauchemin et al., 2003; Forssell et al., 2008; Treimo et al., 2008; Denstadli et al., 2010).
DDGS is another co-product produced in the corn ethanol plant 22 million tons in 2019 in the United States and is widely used around the world as animal feed. Prior studies have shown that DDGS digestibility could be improved with AFEX and hot water treatment (Kim et al., 2008). Digestibility of pretreated DDGS could be further improved when supplemented with hemicellulase enzymes such as pectinase and xylanase (Dien et al., 2008; 2013). Pretreating DDGS using dilute acid followed by enzyme hydrolysis and digested the fibers, thereby enriching the protein content, enhanced digestibility and metabolizable energy (Fries-Craft and Bobeck, 2019; Buenavista et al., 2021).
Traditionally, farmers have performed an ammoniation process in the field by pumping anhydrous ammonia under a tarp containing bales of hay at 3–4g/100 g dry biomass, at ambient temperature for 6–8 weeks, to improve the digestibility by 10–20% of late harvest grasses or straw (Solaiman et al., 1979). By doing this, the crude protein of the hay increases by 5–10% due to ammonia reacting with biomass. The in-field ammoniation process provides a modest increase in digestibility but is a safety concern and is expensive as the ammonia is not recoverable. It was not until 1982 that a contained reactor process involving pressurized, high-temperature, concentrated ammonia (>30%) and known as ammonia fiber expansion or AFEX was developed to increase the enzymatic digestibility of biomass (Balan et al., 2009a) by opening the cell wall. DuPont also developed a low-concentration aqueous ammonia (<15% NH4OH) process to pretreat biomass for the production of bio-ethanol in their commercial plant in Nevada, Iowa (Dunson et. al., 2007). Since ammonia is a volatile alkali, it offers the unique advantage of evaporation-based separation and recovery (>98%) after treating the biomass. Conventional AFEX processing uses liquid ammonia (0.3–2 g NH3/g dry biomass) with moist biomass (0.1–2 g water/g dry biomass) and heating (40–120°C) for a period of 5–180 min. Several variations to the conventional AFEX process have been discovered, such as using gaseous ammonia (Campbell et al., 2013; Balan et al., 2017), extractive ammonia (EA) which improves ammonia’s contact with the biomass, and recovery of ammonia after treatment (Sousa et al., 2009; Chundawat et al., 2013). The advantages and disadvantages of each of the different ammonia pretreatment processes are given in Table 2.
In the new two-step pretreatment process reported in this manuscript, DDGS, HFM, and BSG were first de-esterified using alkali [Ca(OH)2 and NaOH] and filtered and dried until the moisture of the sample was 60%. Subsequently, the de-esterified biomass was subjected to AFEX pretreatment as described in materials and methods section. The two-step pretreatment DDGS, HFM and BSG, looks like the respective AFEX pretreated biomass samples. The following events take place during the two-step pretreatment process: 1) esters cleaved during alkali [Ca(OH)2 and NaOH] treatment, 2) most of the lignin-carbohydrate complex (LCC) cleavage and degradation products formation, 3) lignin/hemicellulose got solubilized in ammonia redistribution to the surface when pressure released, and 4) cellulose de-crystallization. Solubilized lignin and hemicellulose are relocated to the surface of the plant cell wall when ammonia is released from the reactor, similar to a previous report (Chundawat et al., 2011). This makes the biomass porous (>10 nm in diameter) and enhances the accessibility of cellulase enzymes.
Most of the lignocellulosic biomass produced by monocot plants have ester linkages such as acetyl, feruloyl, and coumaroyl functional groups (Balan et al., 2009b) (Figure 2). De-esterifying the lignocellulosic biomass is known to improve enzyme digestibility (Chen et al., 2012; Kim et al., 2016). These ester linkages are cleaved when treated with alkali, such as NaOH, KOH, Ca(OH)2, and NH4OH. The cleavage kinetics is influenced by the strength of alkali and the hydrolyzed product dependent on the type of alkali used. For example, NaOH is one of the strongest alkali cleave ester linkages faster than Ca(OH)2. Treating ester linkages using ammonium hydroxide produces corresponding acids and amides (ammonolysis and hydrolysis), while all other alkali produces corresponding organic acids (hydrolysis). One can measure the ester linkages in lignocellulosic biomass by quantifying the organic acids after alkali treatment such as acetic, ferulic, and coumaric acids or their corresponding carboxamides. Based on our previous studies, possible de-esterified products produced after AFEX process are summarized in Figure 3 (Vismeh et al., 2013). Six different di-ferulate di-acids (8-O-4, 8-5C, 5-5, 8-8 NC, 8-5 NC, 8-8C) are produced when corresponding di-ferulate ester linkages are cleaved by hydroxyl ions. On the contrary, when ammonium ion cleaves the di-ferulate ester linkages, 18 different di-ferulates with mono- or di-amides are produced. Other degradation products produced during AFEX pretreatment include p-coumaric acid, acetic acid, and ferulic acids when hydroxide ion cleaves the corresponding ester linkages and p-coumaroyl amide, acetamide, and feruloylamide (Chundawat et al., 2010; Vismeh et al., 2013).
FIGURE 2. Arabinoxylan lignin linkages in lignocellulosic biomass. During the AFEX process, the targeted ester linkages sites are prone to attach to hydroxide and ammonia, producing the corresponding acids or carboxamide functional groups.
FIGURE 3. Some of the major degradation products produced during AFEX pretreatment. Here, six different di-ferulate di-acids (8-O-4, 8-5C, 5-5, 8-8 NC, 8-5 NC, 8-8C) are produced when corresponding di-ferulate ester linkages are cleaved by hydroxyl ions (shown in blue color). On the contrary, when ammonium ion cleaves the di-ferulate ester linkages, corresponding di-ferulates with mono- or di-amides are produced (shown in red color). Other degradation products produced during AFEX pretreatment include p-coumaric acid, acetic acid, and ferulic acids (shown in blue) when hydroxyl ion cleaves the corresponding ester linkages. Similarly, p-coumaroyl amide, acetamide and feruloyl amide (shown in red) are produced when ammonia cleaves the corresponding ester linkages.
In this work, we evaluated three different scenarios: 1) AFEX pretreatment of BSG/HFM/DDGS (using anhydrous liquid ammonia), 2) 3% dilute ammonium hydroxide pretreatment of BSG/HFM/DDGS, and 3) alkali such as NaOH or Ca(OH)2 pretreatment at varying concentrations (2 or 4%) followed by AFEX pretreatment. To monitor the cleavage of ester linkages, we analyzed the concentration of acetic acid and acetamide in the pretreated biomass extracts using the established GC-MS method against the respective standard (acetic acids and acetamides). As expected, Scenarios 1 and 2 produced acetamide and acetic acid in pretreated biomass, while scenario 3 produced only acetic acids (Figure 4).
FIGURE 4. Three different scenarios of alkali treatment of BSG/HFM/BSG. Here, 1, AFEX pretreatment process; 2, dilute ammonium hydroxide pretreatment and 3, either 2 or 4% NaOH, Ca(OH)2 pretreatment followed by anhydrous ammonia pretreatment. Scenarios 1 and 2 produce carboxamides in pretreated biomass, while scenario 3 produces pretreated biomass without carboxamides.
Pretreatment of BSG using AFEX alone produced acetamide at about 3 mg/g DW BSG. We compared these results with BSG treated using mild alkali (Ca(OH)2) at two different concentrations (2 and 4%) and at two different solid loadings (10 and 20%) followed by carrying out AFEX. At 2% Ca(OH)2 treatment followed by AFEX, the concentration of acetamide significantly increased when compared to untreated BSG. We also observed that 10% solids loading produced lesser acetamide (2.5 mg/g DW BSG) when compared to 20% solids loading (2.8 mg/g DW BSG). On the contrary, when the concentration of Ca(OH)2 was increased from 2 to 4% followed by AFEX, we observed a significant drop (down to 0.3–0.4 mg/g DW BSG) in acetamide concentration both at 10 and 20% solids loading (Figure 5).
FIGURE 5. Amount of acetamide (mg/g dry weight) present in untreated (UT) BSG, AFEX pretreated BSG, and two different alkalies [NaOH and Ca(OH)2] treated de-esterified BSG followed by AFEX pretreatment. Here, two different solids loading (10 and 20%) and two different alkali concentrations (2 and 4%) were used during de-esterification of BSG prior to AFEX pretreatment. Error bars represent the standard deviation of two replicated experimental points.
Treatment of BSG with strong alkali (NaOH) followed by AFEX produced a similar concentration to acetamide as mild alkali (Ca(OH)2) treatment followed by AFEX. We only saw a slight drop in the concentration of acetamide (2–2.5 mg/g DW BSG) at 2% NaOH treatment followed by AFEX, when compared to the concentration of acetamide (2.5–2.8 mg/g DW BSG) following treatment with 2% [Ca(OH)2]. Further increasing alkali concentration of NaOH from 4 to 8% at 10% BSG loading gave acetamide concentration (0.02–0.04 mg/g DW biomass) like untreated biomass (data not shown). However, considering the de-esterification efficiency and subsequent feed quality, high alkali dosage (4% alkali) and 10% TS are considered as optimum conditions while treating DDGS.
Pretreatment of DDGS using AFEX alone produced about 5.3 mg/g DW of acetamide, which was much higher when compared to BSG, indicating that DDGS had high acetylated xylan. We compared these results with untreated DDGS and pretreated DDGS using mild alkali (Ca(OH)2) and strong alkali (NaOH) at 2 and 4% concentrations and at 10 and 20% solids loading followed by carrying out AFEX. When compared to 2% alkali loading, 4% alkali loading at both solids loading followed by heating at 80°C for 15 min resulted in better cleavage of ester linkages. These results show a critical concentration of alkali needs to efficiently de-esterify the BSG. Subjecting the de-esterified biomass at 4% alkali followed by AFEX produced acetamide concentrations like untreated DDGS (Figure 6). By comparing de-acetylation reactions of DDGS and BGC, we conclude that alkali treatment was more effective in the case of DDGS when compared to BSG, indicating the complexity of two different substrates for use as animal feed.
FIGURE 6. Amount of acetamide (mg/g dry weight) produced in untreated (UT) DDGS, AFEX pretreated DDGS, and two different alkalies [NaOH and Ca(OH)2] treated de-esterified DDGS followed by AFEX pretreatment. About 10% solids loading and 4% alkali concentration were used during de-esterification of DDGS prior to AFEX pretreatment. Error bars represent the standard deviation of two replicated experimental points.
We also evaluated the total nitrogen contents of the biomass in BSG and DDGS after AFEX treatment. We found 55–57 mg/g for AFEX pretreated BSG and 72 mg/g for AFEX treated DDGS (results not shown). The more nitrogen content implies more ammoniation and de-acetylation during the AFEX treatment process that led to acetamide formation in DDGS as explained before. We also did not find a significant change in acetamide content (as total nitrogen) when varying temperature or ammonia to biomass ratio. It is important to note that AFEX pretreatment at low temperature and low ammonia to biomass ratio will be more cost-effective.
We evaluated the digestibility of untreated and two different ammonias treated (3% ammonium hydroxide and 1:1 ammonia to biomass ratio in AFEX) BSG, HFM, and DDGS using commercial enzymes (Ctec2: Htec2: Pectinex at 80:15:5 ratio). The results show that untreated BSG, HFM, and DDGS were recalcitrant and produced lower sugar yields when compared to ammonia-treated BSG, HFM, and DDGS, respectively. De-esterification of BSG, HFM, and DDG using NaOH (using 4% alkali) followed by AFEX resulted in higher sugar yield (Figure 7). Higher concentration of ammonia used during AFEX pretreatment (1:1 ammonia to biomass loading) helped to solubilize lignin and sugar oligomers and re-deposit on the surface when ammonia is released during the de-pressurizing step, thereby creating pores in biomass. The pores created in the biomass facilitate easy access of enzymes during hydrolysis by producing higher sugar conversions, as reported earlier (Chundawat et al., 2011). For BSG, we observed that 3% ammonium hydroxide treatment produced twofold higher sugar yield, and AFEX treatment produced threefold sugar yield when compared to control. These results show that the concentration of ammonia and pressure exerted during AFEX pretreatment plays an important role in increasing the digestibility of cellulose and hemicellulose. A similar increase in cellulose and hemicellulose conversation has been reported for different AFEX pretreated monocot and dicot lignocellulosic biomasses (corn stover, agave bagasse, agave leaf matter, switchgrass, reed canary grass, elephant grass, alfaalfa, miscanthus, rice straw, sorghum, sugarcane bagasse, sugarcane leaf matter, poplar) (Balan et al., 2011, 2012; Flores-Gómez et al., 2018; Mokomele et al., 2018) (Table 1).
FIGURE 7. Enzyme hydrolysis of untreated (UT), 3% dilute ammonium hydroxide, and AFEX pretreated BSG, HFM, and DDGS. Green bars represent glucose, and orange bars represent xylose yield (g/L). Error bars represent the standard deviation of two replicated experimental points.
In the case of DDGS, we observed that 3% ammonium hydroxide gave a moderate increase in glucose yield, while AFEX treatment resulted in a threefold higher glucose yield. Though we found higher glucose yield after AFEX treatment when compared to untreated DDGS, the xylose yield was still low. It has been reported that fibers in DDGS have complex xylan linkages and require specialized enzymes, such as pectinase and esterase in the cocktail, to get a higher sugar yield (Dien et al., 2008). In this work, we have demonstrated a two-step process of producing highly digestible BSG, HFM, and DDGS without any acetamide formation for the first time. We hypothesize that similar results could be obtained when two-step processing is applied to other agricultural residues. However, the processing conditions must be optimized.
As the demand for animal feed increases, technologies need to be developed to efficiently use existing feedstocks or find additional feed resources and upgrade them to meet the nutritional requirement of animals. BSG is a sustainable feedstock available throughout the year that can be fractionated and upgraded for sale into animal feed markets. The average price of BSG and DDGS is $100–$200 and $178/dry ton, respectively (Table 3). Other comparable animal feed products in the market include corn gluten meal and wheat middling, whose prices are $125 and $168, respectively. Producing highly digestible pretreated BSG or HFM is expected to fetch a high market price. More importantly, the highly digestible animal feed will result in efficient feed utilization and less animal waste, benefiting the environment. This allows farmers to comply with the ever-tightening regulatory requirements set by the environment protection agencies. Extension of the technology to other agricultural byproducts will enhance animal feed safety and sustainability. Eventually, developing such technology will bring sustainable business opportunities for feed manufacturing companies and animal feed distributors and benefit several ruminant animal farmers around the world. The potential US market for highly digestible BSG, HFM, and DDGS is given in Table 4.
TABLE 3. BSG, HFM, and competitive fiber energy feed. Compositional analysis is provided based on dry matter basis.
For the first time, we demonstrated a two-step pretreatment process (alkaline treatment followed by AFEX) that was an effective method for reducing or eliminating acetamide formation in lignocellulosic biomass, such as BSG, HFM, and DDGS. As detailed above, in several cases, the final acetamide content was 0.04 mg/g equal to that of untreated biomass and, therefore, safe to be used as animal feed. In addition, the pretreatment improved the digestibility by twofold to threefold when compared to untreated BSG, HFM, and DDGS. Compared to the conventional AFEX process, the amount of ammonia required to treat the biomass was lowered following calculated alkali pretreatment. This is another major advantage of the integrated process that could reduce the overall pretreatment cost of biomass without compromising the quality of the product. While only BSG, HFM, and DDGS were experimented during this study, these techniques could easily be applied to other lignocellulosic products such as corn stover, switchgrass, sugarcane bagasse, wheat straw, sorghum, energy cane, miscanthus, soybean meal, and many other widely available lignocellulosic biomasses. From here, research should focus on the optimization for each of these substrates, as well as further improvements in digestibility by adding feed enzymes commonly used in animal feed industry.
The raw data supporting the conclusion of this article will be made available by the authors without undue reservation.
VB and LS conceptualized the idea. VB wrote the original draft. HD did the experimental work. BU prepared the figures. AJ analyzed acetamide concentration in the pretreated samples. AJ and LS reviewed and edited the paper and supervised the work. VB obtained the resources.
Funding for this project was provided by the Michigan Translational Research and Commercialization (MTRAC) AgBio Innovation Hub located at Michigan State University. AJ is supported in part by Michigan AgBioResearch through the USDA National Institute of Food and Agriculture, Hatch project numbers MICL02474. The research was also supported by the Office of Biological and Environmental Research, Office of Science, United States, Department of Energy through DOE Great Lakes Bioenergy Research Center (GLBRC) Grant DE-FC02-07ER64494.
The authors declare that the research was conducted in the absence of any commercial or financial relationships that could be construed as a potential conflict of interest.
All claims expressed in this article are solely those of the authors and do not necessarily represent those of their affiliated organizations or those of the publisher, editors, and reviewers. Any product that may be evaluated in this article, or claim that may be made by its manufacturer, is not guaranteed or endorsed by the publisher.
Balan thanks the University of Houston and the State of Texas for his start-up funds. We thank Mr. Raymond Roach and Mr. James Blyer from Trucent company, MI for providing BSG, HPM, and DDGS for this research. We also thanks Prof. Bruce Dale for using his lab resources.
AFEX, ammonia fiber expansion; AraF, arabino-furanosyl; BSG,b rewer’s spent grains; CS, corn stover; DDGS, dry distillers grains with soluble; DFA, dehydro di-ferulic acids; EA, extractive ammonia; FA, ferulic acid; GAX, glucuronoarabinoxylan; HFM, high-fiber meal produced from BSG; LCC, lignin-carbohydrate complex; pCA, p-coumaric acid; UT, untreated.
Alabi, O. O., Atteh, J. O., Adejumo, I. O., and Ogundele, O. O. (2014). Effects of Dietary Levels of Brewers’ Dried Grain Supplemented with Commercial Enzymes on Performance, Nutrient Retention, and Gastro-Intestinal Tract Characteristics of Arbor Acres Broilers. Int. J. Agri. Innov. Res. 2 (5), 2319–1473. doi:10.9734/AJEA/2014/7254
Aliyu, S., and Bala, M. (2011). Brewer’s Spent Grain: A Review of its Potentials and Applications. Afr. J. Biotechnol. 10 (3), 324–331. doi:10.5897/ajb11.2741
Ashour, E. A., Abd El-Hack, M. E., El-Hindawy, M. M., Attia, A. I., Osman, A. O., Swelum, A. A., et al. (2019). Impacts of Dietary Inclusion of Dried Brewers' Grains on Growth, Carcass Traits, Meat Quality, Nutrient Digestibility and Blood Biochemical Indices of Broilers. SA J. Sci. 49 (3), 573–584. doi:10.4314/sajas.v49i3.18
Balakshin, M., Capanema, E., Gracz, H., Chang, H.-m., and Jameel, H. (2011). Quantification of Lignin-Carbohydrate Linkages with High-Resolution NMR Spectroscopy. Planta 233 (6), 1097–1110. doi:10.1007/s00425-011-1359-2
Balan, V., Bals, B., Chundawat, S. P. S., Marshall, D., and Dale, B. E. (2009a). Lignocellulosic Biomass Pretreatment Using AFEX. Methods Mol. Biol. (Clifton, N.J.) 581, 61–77. doi:10.1007/978-1-60761-214-8_5
Balan, V., Bals, B., da Costa Sousa, L., Garlock, R., and Dale, B. E. (2011). “Chapter 5. A Short Review on Ammonia-Based Lignocellulosic Biomass Pretreatment,” in RSC Energy and Environment Series No. 4 Chemical and Biochemical Catalysis for Next Generation Biofuel. Editor Blake. Simmons (London, UK: Published by the Royal Society of Chemistry), 89–114. doi:10.1039/9781849732857-00089
Balan, V., Dale, B. E., Chundawat, S. P. S., and Sousa, L. D. (2017). Methods for Pretreating Biomass. US 9,644,222 B2. Available at: https://patents.google.com/patent/US9644222B2.
Balan, V., Sousa, L. d. C., Chundawat, S. P. S., Marshall, D., Sharma, L. N., Chambliss, C. K., et al. (2009b). Enzymatic Digestibility and Pretreatment Degradation Products of AFEX-Treated Hardwoods (Populus Nigra). Biotechnol. Prog. 25 (2), 365–375. doi:10.1002/btpr.160
Balan, V., Sousa, L. D., Chundawat, S. P. S., Humpula, J. F., and Dale, B. E. (2012). Overview to Ammonia Pretreatments for Lignocellulosic Biorefineries. Dynamic Biochem. Process Biotechnol. Mol. Biol. ©2012 Glob. Sci. Books 6 (Special Issue 2), 1–11. doi:10.7282/T3GM89JK
Balan, V., and Sousa, L. D. (2019). De - Esterification of Biomass Prior to Ammonia Pretreatment and Systems and Products Related Thereto. Available at: https://patentimages.storage.googleapis.com/48/9f/a6/4bd9306646345c/US20190010295A1.pdf (US 2019/0010295 A1.
Bals, B., Teymouri, F., Haddad, D., Julian, W. A., Vismeh, R., Jones, A. D., et al. (2019). Presence of Acetamide in Milk and Beef from Cattle Consuming AFEX-Treated Crop Residues. J. Agric. Food Chem. 67 (38), 10756–10763. doi:10.1021/acs.jafc.9b04030
Baruah, J., Nath, B. K., Sharma, R., Kumar, S., Deka, R. C., Baruah, D. C., et al. (2018). Recent Trends in the Pretreatment of Lignocellulosic Biomass for Value-Added Products. Front. Energ. Res. 6, 141. doi:10.3389/fenrg.2018.00141
Beauchemin, K. A., Colombatto, D., Morgavi, D. P., and Yang, W. Z. (2003). Use of Exogenous Fibrolytic Enzymes to Improve Feed Utilization by Ruminants. J. Anim. Sci. 81 (14-2), E37–E47. doi:10.2527/2003.8114_suppl_2E37x
Beauchemin, K. A., Ribeiro, G. O., Ran, T., Marami Milani, M. R., Yang, W., Khanaki, H., et al. (2019). Recombinant Fibrolytic Feed Enzymes and Ammonia Fibre Expansion (AFEX) Pretreatment of Crop Residues to Improve Fibre Degradability in Cattle. Anim. Feed Sci. Tech. 256, 114260. doi:10.1016/j.anifeedsci.2019.114260
Buenavista, R. M. E., Siliveru, K., and Zheng, Y. (2021). Utilization of Distiller's Dried Grains with Solubles: A Review. J. Agric. Food Res. 5, 100195. doi:10.1016/j.jafr.2021.100195
Buffington, J. (2014). The Economic Potential of Brewer's Spent Grain (BSG) as a Biomass Feedstock. Aces 04, 308–318. doi:10.4236/aces.2014.43034
Bunzel, M., Ralph, J., Lu, F., Hatfield, R. D., and Steinhart, H. (2004). Lignins and Ferulate−Coniferyl Alcohol Cross-Coupling Products in Cereal Grains. J. Agric. Food Chem. 52 (21), 6496–6502. doi:10.1021/jf040204p
Campbell, T. J., Teymouri, F., Bals, B., Glassbrook, J., Nielson, C. D., and Videto, J. (2013). A Packed Bed Ammonia Fiber Expansion Reactor System for Pretreatment of Agricultural Residues at Regional Depots. Biofuels 4 (1), 23–34. doi:10.4155/bfs.12.71
Celus, I., Brijs, K., and Delcour, J. A. (2009). Fractionation and Characterization of Brewers' Spent Grain Protein Hydrolysates. J. Agric. Food Chem. 57 (12), 5563–5570. doi:10.1021/jf900626j
Chen, X., Shekiro, J., Franden, M. A., Wang, W., Wang, W., Zhang, M., et al. (2012). The Impacts of Deacetylation Prior to Dilute Acid Pretreatment on the Bioethanol Process. Biotechnol. Biofuels 5, 8. doi:10.1186/1754-6834-5-8
Chetrariu, A., and Dabija, A. (2020). Brewer's Spent Grains: Possibilities of Valorization, a Review. Appl. Sci. 10, 5619. doi:10.3390/app10165619
Choct, M., Dersjant-Li, Y., McLeish, J., and Peisker, M. (2010). Soy Oligosaccharides and Soluble Non-starch Polysaccharides: a Review of Digestion, Nutritive and Anti-nutritive Effects in Pigs and Poultry. Asian Australas. J. Anim. Sci. 23, 1386–1398. doi:10.5713/ajas.2010.90222
Chundawat, S. P. S., Bals, B., Campbell, T., Sousa, L., Gao, D., Jin, M., et al. (2013). “Primer on Ammonia Fiber Expansion Pretreatment,” in Chapter 9 for the Book Titled “Aqueous Pretreatment of Plant Biomass for Biological and Chemical Conversion to Fuels and Chemicals”. Editor Charles. Wyman. First Edition (Wiley Series in Renewable Resources), 169–200. doi:10.1002/9780470975831.ch9
Chundawat, S. P. S., Donohoe, B. S., da Costa Sousa, L., Elder, T., Agarwal, U. P., Lu, F., et al. (2011). Multi-scale Visualization and Characterization of Lignocellulosic Plant Cell wall Deconstruction during Thermochemical Pretreatment. Energy Environ. Sci. 4, 973–984. doi:10.1039/C0EE00574F
Chundawat, S. P. S., Pal, R. K., Zhao, C., Campbell, T., Teymouri, F., Videto, J., et al. (2020). Ammonia Fiber Expansion (AFEX) Pretreatment of Lignocellulosic Biomass. JoVE 158, e57488. doi:10.3791/57488
Chundawat, S. P. S., Vismeh, R., Sharma, L. N., Humpula, J. F., da Costa Sousa, L., Chambliss, C. K., et al. (2010). Multifaceted Characterization of Cell wall Decomposition Products Formed during Ammonia Fiber Expansion (AFEX) and Dilute Acid Based Pretreatments. Bioresour. Tech. 101 (21), 8429–8438. doi:10.1016/j.biortech.2010.06.027
da Costa Sousa, L., Chundawat, S. P., Balan, V., and Dale, B. E. (2009). 'Cradle-to-grave' Assessment of Existing Lignocellulose Pretreatment Technologies. Curr. Opin. Biotechnol. 20, 339–347. doi:10.1016/j.copbio.2009.05.003
da Costa Sousa, L., Jin, M., Chundawat, S., Bokade, V., Tang, X., Azarpira, A., et al. (2016). Next-generation Ammonia Pretreatment Enhances Biofuel Production From Biomass via Simultaneous Cellulose De-crystallization and Lignin Extraction. Energy Env. Sci. 9, 1215–1223. doi:10.1039/x0xx00000x
Dale, B. E., Henk, L. L., and Shiang, M. (1985). Fermentation of Lignocellulosic Materials Treated by Ammonia Freeze-Explosion. Developments in Industrial Microbiology, 26. Delhi, India: DIMCAL, 2230070–2334563.
Denstadli, V., Westereng, B., Biniyam, H. G., Ballance, S., Knutsen, S. H., and Svihus, B. (2010). Effects of Structure and Xylanase Treatment of Brewers' Spent Grain on Performance and Nutrient Availability in Broiler Chickens. Br. Poult. Sci. 51 (3), 419–426. doi:10.1080/00071668.2010.495745
Dien, B. S., O'Bryan, P. J., Hector, R. E., Iten, L. B., Mitchell, R. B., Qureshi, N., et al. (2013). Conversion of Switchgrass to Ethanol Using Dilute Ammonium Hydroxide Pretreatment: Influence of Ecotype and Harvest Maturity. Environ. Tech. 34 (13–14), 1837–1848. doi:10.1080/09593330.2013.833640
Dien, B. S., Ximenes, E. A., O’Bryan, P. J., Moniruzzaman, M., Li, X.-L., Balan, V., et al. (2008). Enzyme Characterization for Hydrolysis of AFEX and Liquid Hot-Water Pretreated Distillers' Grains and Their Conversion to Ethanol. Bioresour. Tech. 99, 5216–5225. doi:10.1016/j.biortech.2007.09.030
Dunson, J. R., Tucker, M., Elander, R. T., and Hennessey, S. M. (2007). Treatment of Biomass to Obtain Fermentable Sugars. WO2006110901A2. Available at: https://patents.google.com/patent/WO2006110901A2/en.
Falls, M., Meysing, D., Lonkar, S., Liang, C., Karim, M. N., Carstens, G., et al. (2017). Development of Highly Digestible Animal Feed from Lignocellulosic Biomass Part 1: Oxidative Lime Pretreatment (OLP) and ball Milling of Forage Sorghum1. Translational Anim. Sci. 1 (2), 208–214. doi:10.2527/tas2017.0024
Flores-Gómez, C. A., Escamilla Silva, E. M., Zhong, C., Dale, B. E., da Costa Sousa, L., and Balan, V. (2018). Conversion of Lignocellulosic Agave Residues into Liquid Biofuels Using an AFEX-Based Biorefinery. Biotechnol. Biofuels 11, 7. doi:10.1186/s13068-017-0995-6
Forssell, P., Kontkanen, H., Schols, H. A., Hinz, S., Eijsink, V. G. H., Treimo, J., et al. (2008). Hydrolysis of Brewers' Spent Grain by Carbohydrate Degrading Enzymes. J. Inst. Brewing 114, 306–314. doi:10.1002/j.2050-0416.2008.tb00774.x
Fries-Craft, K., and Bobeck, E. A. (2019). Evaluation of a High-Protein DDGS Product in Broiler Chickens: Performance, Nitrogen-Corrected Apparent Metabolisable Energy, and Standardised Ileal Amino Acid Digestibility. Br. Poult. Sci. 60, 749–756. doi:10.1080/00071668.2019.1652884
Hames, B., Scarlata, C., and Sluiter, A. (2008). Determination of Protein Content in Biomass Laboratory Analytical Procedure (LAP). Technical Report NREL/TP-510-42625. Available at: https://www.nrel.gov/docs/gen/fy08/42625.pdf.
Hatfield, R. D., Marita, J. M., and Frost, K. (2008). Characterization of P -coumarate Accumulation, P -coumaroyl Transferase, and Cell wall Changes during the Development of Corn Stems. J. Sci. Food Agric. 88 (14), 2529–2537. doi:10.1002/jsfa.3376
Hatfield, R. D., Ralph, J., and Grabber, J. H. (1999). Cell wall Cross-Linking by Ferulates and Diferulates in Grasses. J. Sci. Food Agric. 79 (3), 403–407. doi:10.1002/(SICI)1097-0010(19990301)79:3<403:AID-JSFA263>3.0.CO;2-0
He, Y., Kuhn, D. D., Ogejo, J. A., O’Keefe, S. F., Fraguas, C. F., Wiersema, B. D., et al. (2019). Wet Fractionation Process to Produce High Protein and High Fiber Products from brewer's Spent Grain. Food Bioproducts Process. 117, 266–274. doi:10.1016/j.fbp.2019.07.011
Hoover, A. N., Tumuluru, J. S., Teymouri, F., Moore, J., and Gresham, G. (2014). Effect of Pelleting Process Variables on Physical Properties and Sugar Yields of Ammonia Fiber Expansion Pretreated Corn stover. Bioresour. Tech. 164, 128–135. doi:10.1016/j.biortech.2014.02.005
Kavalopoulos, M., Stoumpou, V., Christofi, A., Mai, S., Barampouti, E. M., Moustakas, K., et al. (2021). Sustainable Valorisation Pathways Mitigating Environmental Pollution from Brewers' Spent Grains. Environ. Pollut. 270, 116069. doi:10.1016/j.envpol.2020.116069
Kemppainen, K., Rommi, K., Holopainen, U., and Kruus, K. (2016). Steam Explosion of Brewer's Spent Grain Improves Enzymatic Digestibility of Carbohydrates and Affects Solubility and Stability of Proteins. Appl. Biochem. Biotechnol. 180, 94–108. doi:10.1007/s12010-016-2085-9
Kim, J. S., Lee, Y. Y., and Kim, T. H. (2016). A Review on Alkaline Pretreatment Technology for Bioconversion of Lignocellulosic Biomass. Bioresour. Tech. 199, 42–48. doi:10.1016/j.biortech.2015.08.085
Kim, Y., Hendrickson, R., Mosier, N. S., Ladisch, M. R., Bals, B., Balan, V., et al. (2008). Enzyme Hydrolysis and Ethanol Fermentation of Liquid Hot Water and AFEX Pretreated Distillers' Grains at High-Solids Loadings. Bioresour. Tech. 99 (12), 5206–5215. doi:10.1016/j.biortech.2007.09.031
Kishi, S., Kimura, T., Minami, T., and Kobayashi, H. (1991). Process for Producing Protein-Rich Product, Fibrous Product And/or Vegetable Oil from Brewers Spent Grain. US Patent # 5135765A. Available at: https://patents.google.com/patent/EP0369818A2/en.
Lacassagne, L., Francesch, M., Caire, B., and Mekion, J. P. (1998). Utilization of Tannin Containing and Tannin Free Faba Beans (Vicia Faba) by Young Chicks. Effects of Pelleting Feed on Energy, Protein, and Starch Digestibility. Anim. Feed Sci. Technol. 20, 59–68.
Le, T. D. T., Nguyen Truong, V. P., Ngo, M. T. T., Kim, T. H., and Oh, K. K. (2021). Pretreatment of Corn Stover Using an Extremely Low-Liquid Ammonia (ELLA) Method for the Effective Utilization of Sugars in Simultaneous Saccharification and Fermentation (SSF) of Ethanol. Fermentation 7, 191. doi:10.3390/fermentation7030191
Liang, J., Nabi, M., Zhang, P., Zhang, G., Cai, Y., Wang, Q., et al. (2020). Promising Biological Conversion of Lignocellulosic Biomass to Renewable Energy with Rumen Microorganisms: A Comprehensive Review. Renew. Sust. Energ. Rev. 134, 110335. doi:10.1016/j.rser.2020.110335
Liu, K. (2011). Chemical Composition of Distillers Grains, a Review. J. Agric. Food Chem. 59 (5), 1508–1526. doi:10.1021/jf103512z
Lynch, K. M., Steffen, E. J., and Arendt, E. K. (2016). Brewers' Spent Grain: a Review with an Emphasis on Food and Health. J. Inst. Brew. 122 (4), 553–568. doi:10.1002/jib.363
Marcus, A., and Fox, G. (2021). Fungal Biovalorization of a Brewing Industry Byproduct, Brewer's Spent Grain: A Review. Foods 10, 2159. doi:10.3390/foods10092159
Marriott, P. E., Gómez, L. D., and McQueen‐Mason, S. J. (2016). Unlocking the Potential of Lignocellulosic Biomass through Plant Science. New Phytol. 209, 1366–1381. doi:10.1111/nph.13684
Mnich, E., Bjarnholt, N., Eudes, A., Harholt, J., Holland, C., Jørgensen, B., et al. (2020). Phenolic Cross-Links: Building and De-constructing the Plant Cell wall. Nat. Prod. Rep. 37, 919–961. doi:10.1039/c9np00028c
Mokomele, T., da Costa Sousa, L., Bals, B., Balan, V., Goosen, N., Dale, B. E., et al. (2018). Using Steam Explosion or AFEX to Produce Animal Feeds and Biofuel Feedstocks in a Biorefinery Based on Sugarcane Residues. Biofuels, Bioprod. Bioref. 12 (6), 978–996. doi:10.1002/bbb.1927
Moore, M. M., Gollapudi, B., Nagane, R., Khan, N., Patel, M., Khanvilkar, T., et al. (2019). The Food Contaminant Acetamide Is Not an In Vivo Clastogen, Aneugen, or Mutagen in Rodent Hematopoietic Tissue. Regul. Toxicol. Pharmacol. 108, 104451. doi:10.1016/j.yrtph.2019.104451
Mor, P., Bals, B., Kumar, S., Tyagi, N., Reen, J. K., Tyagi, B., et al. (2019). Influence of Replacing Concentrate Mixture with AFEX Pellets on Rumen Fermentation, Blood Profile and Acetamide Content in the Rumen of Crossbred (Alpine × Beetle) Female Goats. Small Ruminant Res. 170, 109–115. doi:10.1016/j.smallrumres.2018.10.016
Mor, P., Bals, B., Tyagi, A. K., Teymouri, F., Tyagi, N., Kumar, S., et al. (2018). Effect of Ammonia Fiber Expansion on the Available Energy Content of Wheat Straw Fed to Lactating Cattle and buffalo in India. J. Dairy Sci. 101 (9), 7990–8003. doi:10.3168/jds.2018-14584
Mussatto, S. I., Dragone, G., and Roberto, I. C. (2006). Brewers' Spent Grain: Generation, Characteristics and Potential Applications. J. Cereal Sci. 43, 1–14. doi:10.1016/j.jcs.2005.06.001
Nault, R., Bals, B., Teymouri, F., Black, M. B., Andersen, M. E., McMullen, P. D., et al. (2020). A Toxicogenomic Approach for the Risk Assessment of the Food Contaminant Acetamide. Toxicol. Appl. Pharmacol. 388, 114872. doi:10.1016/j.taap.2019.114872
Oryschak, M., Korver, D., Zuidhof, M., and Beltranena, E. (2010a). Nutritive Value of Single-Screw Extruded and Nonextruded Triticale Distillers Dried Grains with Solubles, with and without an Enzyme Complex, for Broilers. Poult. Sci. 89, 1411–1423. doi:10.3382/ps.2009-00619
Oryschak, M., Korver, D., Zuidhof, M., Meng, X., and Beltranena, E. (2010b). Comparative Feeding Value of Extruded and Nonextruded Wheat and Corn Distillers Dried Grains with Solubles for Broilers. Poult. Sci. 89 (10), 2183–2196. doi:10.3382/ps.2010-00758
Ribeiro, G. O., Gruninger, R. J., Jones, D. R., Beauchemin, K. A., Yang, W. Z., Wang, Y., et al. (2020). Effect of Ammonia Fiber Expansion-Treated Wheat Straw and a Recombinant Fibrolytic Enzyme on Rumen Microbiota and Fermentation Parameters, Total Tract Digestibility, and Performance of Lambs. J. Anim. Sci. 98 (5), skaa116. doi:10.1093/jas/skaa116
Ruiz, R., and Ehrman, T. (1996). “Determination of Carbohydrates in Biomass by High Performance Liquid Chromatography,” in Laboratory Analytical Procedure No. 002 (Golden, CO: National Renewable Energy Laboratory). Available at: http://citeseerx.ist.psu.edu/viewdoc/download?doi=10.1.1.475.72&rep=rep1&type=pdf.
Salami, S. A., Luciano, G., O'Grady, M. N., Biondi, L., Newbold, C. J., Kerry, J. P., et al. (2019). Sustainability of Feeding Plant By-Products: A Review of the Implications for Ruminant Meat Production. Anim. Feed Sci. Tech. 251, 37–55. doi:10.1016/j.anifeedsci.2019.02.006
Santos, M., Jiménez, J. J., Bartolomé, B., Gómez-Cordovés, C., and del Nozal, M. J. (2003). Variability of brewer's Spent Grain within a Brewery. Food Chem. 80, 17–21. doi:10.1016/S0308-8146(02)00229-7
Saulnier, B. K., Phongpreecha, T., Singh, S. K., and Hodge, D. B. (2020). Impact of Dilute Acid Pretreatment Conditions on P-Coumarate Removal in Diverse maize Lines. Bioresour. Tech. 314, 123750. doi:10.1016/j.biortech.2020.123750
Schwarz, P. B., Casper, H. H., and Beattie, S. (2018). Fate and Development of Naturally Occurring Fusarium Mycotoxins during Malting and Brewing1. J. Am. Soc. Brewing Chemists 53 (3), 121–127. doi:10.1094/ASBCJ-53-0121
Sluiter, J. B., Ruiz, R. O., Scarlata, C. J., Sluiter, A. D., and Templeton, D. W. (2010). Compositional Analysis of Lignocellulosic Feedstocks. 1. Review and Description of Methods. J. Agric. Food Chem. 58 (16), 9043–9053. doi:10.1021/jf1008023
Solaiman, S. G., Horn, G. W., and Owens, F. N. (1979). Ammonium Hydroxide Treatment on Wheat Straw. J. Anim. Sci. 49 (3), 802–808. doi:10.2527/jas1979.493802x
Tang, X., da Costa Sousa, L., Jin, M., Chundawat, S., Chambliss, C., La, M. W., et al. (2015). Designer Synthetic media for Studying Microbial-Catalyzed Biofuel Production. Biotechnol. Biofuels 8 (1), 1. doi:10.1186/s13068-014-0179-6
Treimo, J., Aspmo, S. I., Eijsink, V. G. H., and Horn, S. J. (2008). Enzymatic Solubilization of Proteins in Brewer's Spent Grain. J. Agric. Food Chem. 56, 5359–5365. doi:10.1021/jf073317s
Vismeh, R., Haddad, D., Moore, J., Nielson, C., Bals, B., Campbell, T., et al. (2018). Exposure Assessment of Acetamide in Milk, Beef, and Coffee Using Xanthydrol Derivatization and Gas Chromatography/Mass Spectrometry. J. Agric. Food Chem. 66 (1), 298–305. doi:10.1021/acs.jafc.7b02229
Vismeh, R., Lu, F., Chundawat, S. P. S., Humpula, J. F., Azarpira, A., Balan, V., et al. (2013). Profiling of Diferulates (Plant Cell wall Cross-Linkers) Using Ultrahigh-Performance Liquid Chromatography-Tandem Mass Spectrometry. Analyst 138 (21), 6683–6692. doi:10.1039/C3AN36709F
Westendorf, M. L., and Wohlt, J. E. (2002). Brewing By-Products: Their Use as Animal Feeds. Vet. Clin. North America: Food Anim. Pract. 18 (2), 233–252. doi:10.1016/S0749-0720(02)00016-6
Westendorf, M. L., Wohlt, J. E., Sniffen, C. J., and Ward, R. T. (2014). Nutrient Content of Brewers Grains Produced at a Commercial Brewery: Variation in Protein/nitrogen, Fiber, Carbohydrate, Fat, and Minerals. The Prof. Anim. Scientist 30 (4), 400–406. doi:10.15232/pas.2013-01272
Xu, L., Zhang, S.-J., Zhong, C., Li, B.-Z., and Yuan, Y.-J. (2020). Alkali-Based Pretreatment-Facilitated Lignin Valorization: A Review. Ind. Eng. Chem. Res. 59, 16923–16938. doi:10.1021/acs.iecr.0c01456
Xue, S., Jones, A. D., Sousa, L., Piotrowski, J., Jin, M., Sarks, C., et al. (2018). Water-soluble Phenolic Compounds Produced from Extractive Ammonia Pretreatment Exerted Binary Inhibitory Effects on Yeast Fermentation Using Synthetic Hydrolysate. PLoS One 13 (3), e0194012. doi:10.1371/journal.pone.0194012
Keywords: lignocellulosic biomass, alkaline pretreatment, AFEX process, acetamide, animal feed, ester linkages
Citation: Dong H, Sousa LdC, Ubanwa B, Jones AD and Balan V (2022) A New Method to Overcome Carboxyamide Formation During AFEX Pretreatment of Lignocellulosic Biomass. Front. Chem. 9:826625. doi: 10.3389/fchem.2021.826625
Received: 01 December 2021; Accepted: 28 December 2021;
Published: 21 January 2022.
Edited by:
Kai Yan, Sun Yat-sen University, ChinaReviewed by:
Aimin Wu, South China Agricultural University, ChinaCopyright © 2022 Dong, Sousa, Ubanwa, Jones and Balan. This is an open-access article distributed under the terms of the Creative Commons Attribution License (CC BY). The use, distribution or reproduction in other forums is permitted, provided the original author(s) and the copyright owner(s) are credited and that the original publication in this journal is cited, in accordance with accepted academic practice. No use, distribution or reproduction is permitted which does not comply with these terms.
*Correspondence: Venkatesh Balan, dmJhbGFuQHVoLmVkdQ==
Disclaimer: All claims expressed in this article are solely those of the authors and do not necessarily represent those of their affiliated organizations, or those of the publisher, the editors and the reviewers. Any product that may be evaluated in this article or claim that may be made by its manufacturer is not guaranteed or endorsed by the publisher.
Research integrity at Frontiers
Learn more about the work of our research integrity team to safeguard the quality of each article we publish.