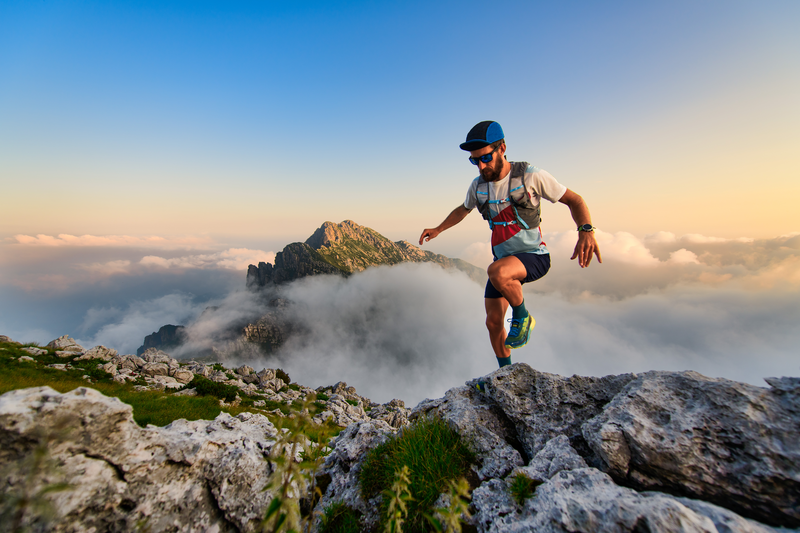
94% of researchers rate our articles as excellent or good
Learn more about the work of our research integrity team to safeguard the quality of each article we publish.
Find out more
ORIGINAL RESEARCH article
Front. Chem. , 20 January 2022
Sec. Chemical Physics and Physical Chemistry
Volume 9 - 2021 | https://doi.org/10.3389/fchem.2021.823519
This article is part of the Research Topic Efficient Near-Infrared-Emitting Materials: Design, Synthesis, Mechanisms, and Applications View all 5 articles
Aggregation-induced emission (AIE) has attracted much attention in the past 2 decades. To develop novel AIE-active materials, ACQ-to-AIE transformation via regioisomerization is one of the most straightforward method. However, most of the reported ACQ-to-AIE transformations are achieved by migrating bulky units. In this work, a facile conversion was realized by migrating a small pyrrolidinyl group from para- to ortho-position on the rofecoxib scaffold. As a result, a pair of new isomers named MOX2 and MOX4 exhibited AIE behavior and ACQ activity, respectively. Moreover, MOX2 also showed solvatochromic, mechanochromic, and acidochromic properties with reversible multi-stimulus behavior. Single crystal X-ray analysis of MOX2 revealed that the molecular conformation and its packing mode were responsible for the AIE emission behavior. Further investigation indicated that MOX2 showed high lipid droplets staining selectivity. Taken together, the current work not only provides a new design philosophy for achieving ACQ-to-AIE conversion by migrating a small pyrrolidinyl group but also presents a promising candidate MOX2 for potential applications such as in security ink, optical recording and biological applications.
Aggregation Induced Emission (AIE) materials have been successfully developed in different research fields since it was discovered by Tang’s group back in 2001 (Luo et al., 2001; Hong et al., 2009; Hong et al., 2011; Mei et al., 2015; Arathi et al., 2016; Mallick et al., 2017; Lou and Yang, 2020). To date, a variety of AIE luminogens (AIEgens) have been reported (Lim et al., 2016; Choi et al., 2020; Li J. et al., 2020) for their inherent characteristics of high brightness in solid states by suppressing extensive π-π stackings in their packing mode (Yuan et al., 2014; Mei et al., 2015), which would otherwise result in aggregation-caused quenching (ACQ) of the fluorophores (Hong et al., 2009; Yuan et al., 2010). These AIEgens varied greatly in terms of their chemical structures, including tetraphenylethylene (TPE) (Feng H. T. et al., 2018; La et al., 2018; Wu et al., 2018), triphenylamine (TPA) (Zhang et al., 2013; Cao et al., 2014; Zhang Y. et al., 2014), cyano-substituted diarylethene (Shen et al., 2013; Zhang X. et al., 2014; Lu et al., 2015), 2,3,4,5-tetraphenylsiloles (Chen et al., 2014), silole (Chang et al., 2015), distyrylanthrancene (Niu et al., 2015; Xiong et al., 2015), and anthracene derivatives (Lu et al., 2010; Zhang et al., 2011; Li A. et al., 2019), conjugated polymers (Ravindran and Somanathan, 2017; Wang et al., 2018), and so on. Modification of the existing AIE scaffolds is the most popular strategy for constructing new AIEgens (Mei et al., 2015). In addition to this strategy, transforming ACQ molecules into AIE-active materials also provides a direct way to design highly bright AIEgens due to the rich source of ACQ (Yuan et al., 2010; Zong et al., 2016; Liu et al., 2017; Lu et al., 2019; Li Y. et al., 2020; Pratihar et al., 2020; Wang et al., 2020; Huang et al., 2021; Long et al., 2021; Zhang et al., 2021).
Traditionally, ACQ-to-AIE transformation can be achieved by integrating bulky substituents into ACQ molecules to avoid strong π-π stackings or by introducing twisted AIEgens (Dhokale et al., 2015; Gomez-Duran et al., 2015; Feng X. et al., 2018) and propeller-shaped molecules into ACQ fluorophores (Ma et al., 2016; Wang et al., 2020). However, integration of twisted AIEgens prolongs the π system thus it would probably change the already satisfactory fluorescent properties of the original ACQ molecules. Therefore, it will be highly desirable to achieve ACQ-to-AIE conversion without extending its conjugated system too much. Some progresses have been made along this line for the past decade (Sasaki et al., 2017; Li Y. et al., 2020). Among them, ACQ-to-AIE conversion was realized by Tang’s group recently through a regioisomerization strategy via shifting a molecular rotor in the end position of a planar core of dithieno [2,3-a:3′,2′-c] benzo [i] phenazine (TBP) to the bay position (Li Y. et al., 2020). The same strategy was utilized by Xu’s group to achieve ACQ-to-AIE conversion through changing the substituted position of a benzanthrone moiety from the meta-position to the ortho-position on the perylenetetracarboxylic diimide core (Long et al., 2021). Similarly, Prasad’s group also realized ACQ-to-AIE transformation by changing a cyano group from the para-position to the meta-position on the same phenyl group (Pratihar et al., 2020). Despite the great examples above, the successful cases of the regioisomerization of small units are still rare (Pratihar et al., 2020). Therefore, it is still necessary to develop more examples of ACQ-to-AIE conversion to guide the design of novel AIEgen materials.
Herein, we report our recent work on the realization of ACQ-to-AIE transformation by migrating a small pyrrolidine group from para-to ortho-position based on the rofecoxib scaffold. The resulting compounds MOX4 with para-substitution showed ACQ effect while compound MOX2 wih ortho-substition exhibited AIE acitivity (Figure 1). Moreover, MOX2 also showed multi-stimulus fluorescent responsive properties, such as mechanochromic, acidochromic properties. In addition, its potential applications have been presented. This work is expected to provide a new guidance on designing AIE-type materials.
All commercial reagents and solvents were used as received. Compounds p-tolylacetic acid and 2-bromo-4′-methanesulfonyl acetophenone were purchased from Shanghai Macklin Biochemical Co., Ltd. and Shanghai Bidepharm Co., Ltd., respectively. Various benzaldehydes with different substituted groups were purchased from Shanghai Shaoyuan Co., Ltd. Reactions were magnetically stirred and monitored on a TLC Silica gel 60G F254 plate from Millipore Sigma (United States). All other reagents and solvents were purchased from Sigma-Aldrich (United States) and used without further purification, unless otherwise stated. Photoluminescence (PL) spectra were recorded on a Varioskan LUX 3020-80110. Differential scanning calorimetry (DSC) were obtained on a DSC STAR system at a heating rate of 15°C/min from 40°C to 500°C under a high purity nitrogen atmosphere. Powder X-ray diffraction (PXRD) patterns were recorded on a Rigaku (D/MaX-3B) diffractometer. Single-crystal X-ray diffraction was conducted with Bruker D8 Quesr/Venture diffraction with λ = 0.77Å (MoKα). Lipid droplets (LDs) imaging was performed with a Nikon Ti-E&C2 scanning unit. 1H NMR and 13C NMR spectra were measured on a Bruker AV 600 spectrometer in appropriated deuterated chloroform solution at room temperature with the solvent residual proton signal as a standard. High resolution mass spectra (HRMS) were recorded on a GCT premier CAB048 mass spectrometer operating in MALDI-TOF mode.
The synthetic route of compounds is outlined in Scheme 1. The key intermediate MOX was synthesized in a one-step reaction involving cyclization of esters via 2-bromo-4′-methanesulfonylacetophenone and p-methyl phenylacetic acid in the presence of triethylamine and DBU. Finally, the target compounds MOX2 and MOX4 were prepared via Knoevenagel condensation reaction. (General procedure). Piperidine, 3 drops, was added to a mixture of 0.140 g (0.0004 mol) of intermediate (MOX) and 0.140 g of 2-(1-Pyrrolidinyl) bezaldehyde in 10 ml of methanol, and the mixture was stirred at room temperature for 12 h in dark atmosphere. The mixture was then cooled, and the precipitate was filtered off and washed with methanol on a filter. The yellow powder (MOX2, 0.1001 g) was obtained with the yield 72%. The DSC thermogram of powder MOX2 showed only one endothermic peak that corresponds to the melting point (Tm = 203.6°C) (Supplementary Figure S12). 1H NMR (600 MHz, DMSO-d6) δ 8.09 (d, J = 8.3 Hz, 2H), 7.89 (d, J = 8.8 Hz, 1H), 7.72 (d, J = 8.3 Hz, 2H), 7.26 (d, J = 23.8 Hz, 3H), 7.17 (d, J = 8.1 Hz, 2H), 7.05–6.89 (m, 2H), 6.04 (s, 4H), 3.06 (s, 3H), 2.28 (s, 1H), 1.76 (s, 4H). 13C NMR (151 MHz, DMSO-d6) δ 168.3, 150.3, 148.8, 146.6, 142.1, 139.2, 136.1, 131.5, 130.6, 130.5, 129.5, 129.3, 128.0, 126.5, 124.8, 123.2, 120.6, 116.8, 111.9, 52.6, 43.7, 24.9, 21.4.HR-MS (ESI): calced for C29H27NO4S: 486.1734 [(M + H)+]), found:486.1813. The red powder (MOX4, 0.1230 g) was synthesized following the general procedure with the yield 88%. The DSC thermogram of powder MOX4 showed only one endothermic peak that corresponds to the melting point (Tm = 231.3°C) (Supplementary Figure S12). 1H NMR (600 MHz, DMSO-d6) δ 8.05 (d, J = 8.1 Hz, 2H), 7.67 (dd, J = 8.2, 3.9 Hz, 4H), 7.20 (d, J = 8.0 Hz, 2H), 7.13 (d, J = 8.0 Hz, 2H), 6.61 (d, J = 8.7 Hz, 1H), 5.94 (s, 1H), 3.33 (s, 3H), 2.27 (s, 4H), 1.97 (s, 4H). 13C NMR (151 MHz, DMSO-d6) δ 168.4, 148.7, 148.6, 144.2, 141.9, 138.5, 136.3, 133.0, 130.8, 129.5, 129.2, 128.0, 127.0, 122.4, 120.5, 115.2, 112.5, 47.7, 43.8, 25.4, 21.3. HR-MS (ESI): calced for C29H27NO4S:486.1734 [(M + H)+], found:486.1771. The final products were characterized by 1H NMR, 13C NMR, high-resolution mass spectrometry and single-crystal X-ray diffraction. The relevant data are collected from the original spectra and listed in the Support Information (Supplementary Figures S1–S6).
As displayed in Figure 2A, MOX2 had two absorption bands at 350 and 430 nm, respectively. The high energy band was attributed to the π-π transition while the low energy band was probably caused by the internal charge transfer (ICT) (Pati et al., 2013; Pati et al., 2015). In contrast, MOX4 has only one maximum absorption band at 484 nm. In terms of emission, MOX2 had a maximum PL intensity at 674 nm, slightly red-shifted compared to 652 nm of MOX4 (Figure 2B). Another attractive feature of MOX2 was its large Stokes shifts (13,675 cm−1) in dimethyl sulfoxide (DMSO) as displayed in Supplementary Table S1, which may be beneficial for overcoming the self-quenching of traditional fluorophores (Li P. et al., 2019). We carried out an experiment of 50 μM concentration, this intensity was consistent with the Beer-Lambert’s plot (Supplementary Figure S7). To provide insight into regioisomerization of the pyrrolidine group in compounds MOX2 and MOX4 on their photophysical properties, density functional theory (DFT) calculations at the B3LYP/6-311++G (2 d,p) level were carried out (Lee et al., 1988). The ICT was evident in comparison between the highest occupied molecular orbital (HOMO) and the lowest unoccupied molecular orbital (LUMO). The energy band gap was determined to be 3.05 and 2.75 eV for MOX2 and MOX4, respectively, which was consistent with our experimental absorption data (Figure 2C).
FIGURE 2. Normalized absorption (A) and PL (B) spectra of MOX2 and MOX4 dyes in DMSO (50 μM). (C) The optimized structure of MOX4, MOX2, and their HOMO and LUMO at the B3LYP/6-31++G (2 d, p) level.
The UV-vis absorption and PL spectra of MOX2 and MOX4 were measured in different solvents, such as DMSO, dimethylformamide (DMF), chloroform, dichloromethane (DCM), ethanol (EtOH), tetrahydrofuran (THF). The photophysical data were summarized in Supplementary Table S1. As the solvent varied from chloroform to DMSO, the λabs of MOX2, and MOX4 displayed minor changes, indicating that their ground states are hardly affected by the solvent polarity (Supplementary Figure S8). In contrast, the PL spectra of MOX2 and MOX4 exhibited different maximum wavelengths in different solvents (Figure 3). With the solvent polarity changed from chloroform to DMSO, the λem of MOX2, and MOX4 are red-shifted of 40 and 58 nm, respectively. Furthermore, the PL intensity of MOX2 was gradually weakened with increased polarity of the solvent, indicating that the PL intensity of MOX2 was highly dependent on the polarity of solvents.
FIGURE 3. PL spectra of compounds MOX2 (A) and MOX4 (B) in various solvents (50 µM), such as DMSO, DMF, chloroform, DCM, EtOH, THF, respectively. Inset: photograph of MOX2 (A) and MOX4 (B) in various solvents with UV irradiation (λ = 365 nm).
To demonstrate the feasibility of the present strategy, the photophysical properties of the two isomers were performed to monitor the PL intensity fluctuation of the molecules in DMSO/water mixtures with various water volume fractions (fw) as shown in Figure 4. The absorption spectra as a function of the water fraction of MOX2 and MOX4 in DMSO as displayed in Supplementary Figure S9. As shown in Figure 4C, molecule MOX4 displayed strong emission in DMSO due to the presence of a large π-conjugated planar structure. As fw was increased from 0 to 100 vol%, the PL intensity was decreased gradually, and the quantum yield (QY) of MOX4 decreased significantly from DMSO (3.96%) to water (0.30%) (Table 1). This result demonstrated that compound MOX4 has typical ACQ effect. On the contrary, MOX2 exhibited week emission in DMSO (Figure 4B). In the water fraction range (fw = 0–60 vol%), the PL intensity was still weak. However, when fw reached 80 vol%, the PL intensity was increased significantly, which was ascribed to the aggregation of the compound (Figure 4D) (Liu et al., 2016; Sun et al., 2018; Gao et al., 2019). Moreover, MOX2 gave a low QY in pure DMSO (0.13%) but a high QY in pure water (2.62%) (Table 1). Therefore, MOX2 was AIE-active. The distinct difference in solid-state QY suggested a significant effect of the pyrrolidinyl position as regioisomers on the fluorescent properties.
FIGURE 4. (A) The photos in different solvent states of MOX2 and MOX4 at 365 nm (10 µM). (B) The MOX2 PL spectra as a function of the water fraction in DMSO. (C) The MOX4 PL spectra as a function of the water fraction in DMSO. (D) Variation in PL intensity (I/I0) of MOX2 with fw (%), where I0 is the luminescence intensity in pure H2O. (E) Variation in PL intensity (I/I0) of MOX4 with fw (%), where I0 is the luminescence intensity in pure DMSO.
To better understand the origin of the AIE property, the single crystal of MOX2 was obtained (CCDC 2107729) and its molecular packing was analyzed as follows. The crystal data and structure refinement for MOX2 as displayed in Supplementary Table S2. As shown in Figure 5A, the dihedral angles between A-B, A-C, and B-C planes are 80.76°, 29.94°, and 65.82°, respectively. In addition, MOX2 adopted a loose packing mode with weaker intermolecular C–H∙∙∙O (2.484 Å, 2.696 Å, 2.633 Å, 2.659 Å) interactions as shown in Figure 5B; Supplementary Figure S10. The existence of these intermolecular interactions helped rigidify the molecular conformation, thus restricting the intramolecular rotation of the phenyl group. Moreover, the non-radiative relaxation process could be largely prohibited and significant emission enhancement was observed in its solid form, yielding the remarkable AIE phenomenon. It is worth noting that such relatively weak intermolecular interactions coculd be easily destroyed upon exposure to external mechanical force, giving rise to the mechanochromic properties as studied below. The single crystal data of MOX2 was displayed in the Supporting Information (Supplementary Table S2).
FIGURE 5. Crystal structure of MOX2. (A) a single molecule in the crystal strucure and relevant dihedral angles; (B) a tetramer in the crystal structure.
Due to the AIE characteristics of MOX2, its mechanochromic luminescence (MCL) behaviors was investigated. As shown in Figure 6, the pristine MOX2 powder emitted strong yellow fluorescence with a maximum at 592 nm. The emission peak red-shifted to 660 nm after grinding with red emission under 365 nm light. The spectral shift value was 68 nm (Table 2). The emission of the ground sample was restored to its original yellow color by immersing of acetone for 2 s or heated at 50°C within 10 min (Figure 6A). Moreover, fluorescence color conversion could be repeated numerous times (Figures 6B–E) without fatigue (Chan et al., 2014). To gain insight into the MCL phenomenon, PXRD and DSC experiments were conducted on the pristine, grinding, immersing, and heating solids of MOX2 and MOX4 (Figure 7). It showed that the intensity of the diffraction peaks observed for powdered crystalline MOX2 significantly decreased upon grinding, which indicated the loss of crystallinity. The diffraction peaks of MOX4 still existed after heavily grinding. Furthermore, MOX2 exhibited greater redshift than MOX4 after grinding (Supplementary Figure S11). The transition from a crystalline structure to an amorphous state upon grinding was further confirmed by DSC experiments. In a DSC measurement of grinding MOX2 and MOX4 (Supplementary Figure S12), exothermic peaks that corresponded to the cold-crystallization transition MOX2 (T = 107.7°C) and MOX4 (T = 155.1°C) were observed followed by endothermic peaks that corresponded to the melting point of powder MOX2 (T = 203.6°C) and MOX4 (T = 231.3°C), respectively. These experimental results suggested that the transformation between crystalline structure and the amorphous state was responsible for the observed MCL behavior upon external stimuli.
FIGURE 6. (A) PL spectra of MOX2 powder under alternating grinding, immersing and heating; Images were taken under a 365 nm hand-held UV lamp. Change in the PL spectrum of MOX2 in grinding-immersing (B) and grinding-heating (D) circles. Repeated switching of the emission wavelength in grinding-immersing (C) and grinding-heating (E) circles.
FIGURE 7. (A) PXRD patterns of compound MOX2 in different states: pristine (black line), grinding (red line). (B) PXRD patterns of compound MOX4 in different states: pristine (black line), grinding (red line). (C) A reversible data recording device of MOX2 based on its mechanochromic phosphorescence.
By exploiting the excellent MCL behavior and good reversibility of MOX2, a simple rewritable phosphorescence data recording device was fabricated (Figure 7C) (Gundu et al., 2017). The specific reversible procedure is as follows. First, the original sample was put into a mortar and ground as the grinding sample. Next, it was spread on a filter paper to make a thin film, showing orange emission under UV excitation. Then, the characters “AIE” were written on the thin film with a writing brush with the acetone solvent as the “ink”. Due to the change of the emission color, the yellow-emitting letters could be clearly seen. Next, the “AIE” was erased by using a cotton swab to make it completely disappear, resulting in the recovery of the original orange thin film. New letters “MCL” could be written again with the “writing brush” of the acetone solvent, which could still be removed by using a cotton swab according to the above method. This writing-erasing process can be repeated for several cycles. Based on this, MOX2 could be a potential fluorescent material for applications as repeated writing.
Next, the sensing properties of MOX2 was exploited because the pyrrolidine unit can be protonated. The solution of MOX2 was prepared in DCM at a concentration of 1 mM. This solution was added dropwise to a filter paper. At this point, the filter paper showed a dark red color under 365 nm illumination. Then after fumigation with trifluoroacetic acid (TFA) for 1 min, at the very beginning, the filter paper exhibited light blue under 365 nm illumination. With the passage of time, the light blue faded, and then the orange began to appear (Supplementary Figure S13). Until 480 min, the blue was completely gone. The color of the filter paper sheet returned to orange (Supplementary Figure S13) (Chen et al., 2018). Meanwhile, as shown in Figure 8A, to investigate the possible acidochromic properties of MOX2, the spectral response ability of MOX2 towards TFA in methanol solution (1.0 × 10−4 M) was also measured. When TFA was gradually added to the methanol solution of MOX2, the yellow solution by degrees converted into a light blue one (Figure 8A inset). Upon addition of TFA, the color of MOX2 in methanol solvent changed from orange to blue under 365 nm illumination, and a new blue-shifted peak formed in the PL spectrum (Figure 8A) (Cao et al., 2019; Cao et al., 2021). With the concentration of TFA increased from 0 to 21.32 mM, pronounced changes in emission suggested that MOX2 had been protonated by TFA. The ratio of PL intensity had a quadratic relationship with TFA concentration in a certain range of TFA concentrations (Figure 8B) (Cao et al., 2020). As displayed in Figure 8C, we further explored the acidochromic behavior of MOX2 for making a rewritable media by coating the solution on a filter paper. The letters were written on filter paper were stable, which could be erased only on fuming with TFA. Fumigation with ammonia could quickly return the sample to the initial color. Fumigation with TFA again can be quickly wiped off. The color would gradually return to its initial appearance after 2 h. This demonstrated that MOX2 has the potential to be used as security link.
FIGURE 8. (A) PL spectra of MOX2 (100 μM) in methanol solvent with different concentration (0.67-21.32 mM) of TFA. Photographs pf MOX2 (100 μM) in methanol solvent before and after acidification at 365 nm UV light showed in the inset. (B) The plot and linear fitting of PL intensity at 484 nm versus TFA (0.67-21.32 mM). (C) Photographs of filter papers coated with MOX2 (10 mM) in DCM solvent under different conditions.
Lipid droplets (LDs) are key organelles which are closely related to living status and death process of living cells (Kuerschner et al., 2008; Walther and Farese, 2009). Before cell imaging experiments, the potential cytotoxicity of MOX2 and MOX4 was evaluated using the MTT assay and low cytotoxicity of them was observed (Supplementary Figure S14). We first conducted co-staining experiments of LDs in living HeLa cells (Figure 9). The cells were stained with BODIPY 503 (1 × 10−5 M) and MOX2 (1 × 10–5 M) for 0.5 h, and were imaged in green channel (λex = 488 nm, λem = 500–540 nm) and red channel (λex = 488 nm; λem = 600–640 nm), respectively. The two channels are well overlapped with each other. This result indicated the good LDs selectivity of MOX2. When HeLa cells were incubated with MOX2 for 30 min at 37°C, strong red fluorescence signals were observed from inside cells shown in Supplementary Figure S15. This was not surprising for the far-red emitting compound MOX2, which showed a bright fluorescence image in vitro.
FIGURE 9. Colocalization confocal imaging of living HeLa cells stained with MOX2 and BODIPY 503: (A) bright field image; (B) imaging channel of BODIPY 503 (λex = 488 nm; λem = 500–540 nm); (C) imaging channel of MOX2 (λex = 488 nm; λem = 600–640 nm); (D) overlay image. Scale bar: 10 µm. (E) Red and green lines represent the fluorescence of MOX2 and BODIPY, respectively.
SCHEME 1. Synthetic route of MOX2 and MOX4. Reaction conditions: (A) Et3N, 25°C, 1 h, DBU −5°C, 1 h; (B) piperidine and 2-(1-pyrrolidinyl)benzaldehyde or 4-(1-Pyrrolidinyl)benzaldehyde.
In this work, ACQ-to-AIE transformation was achieved by migrating a small pyrrolidinyl group from para- to ortho-position in a new molecular system, in which the para-isomer MOX4 showed the ACQ behavior and the ortho-isomer MOX2 is AIE-active. Moreover, compound MOX2 exhibited mechanochromic properties and pressure sensing ability. PXRD and DSC revealed that the MCL mechanism was ascribed to the transformation among crystalline state and the amorphous state by grinding. Furthermore, investigation of the acidochromic behavior of MOX2 revealed that it could be further used as security link. In further biological experimental study, MOX2 could be utilized as a fluorescent probe for LDs imaging in living cells. This work provides a novel strategy to achieve the ACQ-to-AIE transformation, which will provide a new guideline for future design of new AIEgens from ACQ molecules.
The original contributions presented in the study are included in the article/Supplementary Material, further inquiries can be directed to the corresponding authors.
ZW, RL, LC performed the fluorescence and mass spectrometry experiments, and wrote the manuscript. XZ, WL, XL, and LWC performed data collation. NC, SS, ZL, and JH reviewed manuscript drafts. ZW, RL, and LC contributed equally in this work. XC, BL, and LX conceived the entire project.
Financial support from the Natural Science Foundation of Fujian Province (No. 2020J06028) to LX and the NSFC (NO. 51872048) to RL, the CAS/SAFEA International Partnership Program for Creative Research Teams to XC and National Natural Science Foundation of China (Nos. 81872751 and 82104003). Liao Ning Revitalization Talents Program (No. XLYC2002115) and Key R&D Plan of Liaoning Province in 2020 (No. 2020020215-JH2/103).
The authors declare that the research was conducted in the absence of any commercial or financial relationships that could be construed as a potential conflict of interest.
All claims expressed in this article are solely those of the authors and do not necessarily represent those of their affiliated organizations, or those of the publisher, the editors and the reviewers. Any product that may be evaluated in this article, or claim that may be made by its manufacturer, is not guaranteed or endorsed by the publisher.
The Supplementary Material for this article can be found online at: https://www.frontiersin.org/articles/10.3389/fchem.2021.823519/full#supplementary-material
Arathi, A. S., Mallick, S., and Koner, A. L. (2016). Tuning Aggregation-Induced Emission of 2,3-Napthalimide by Employing Cyclodextrin Nanocavities. ChemistrySelect 1, 3535–3540. doi:10.1002/slct.201600840
Cao, Y., Xi, W., Wang, L., Wang, H., Kong, L., Zhou, H., et al. (2014). Reversible Piezofluorochromic Nature and Mechanism of Aggregation-Induced Emission-Active Compounds Based on Simple Modification. RSC Adv. 4 (47), 24649–24652. doi:10.1039/C4RA03042G
Cao, X., Ding, Q., Li, Y., Gao, A., and Chang, X. (2019). Continuous Multi-Channel Sensing of Volatile Acid and Organic Amine Gases Using a Fluorescent Self-Assembly System. J. Mater. Chem. C 7, 133–142. doi:10.1039/c8tc04964e
Cao, X., Li, Y., Han, Q., Gao, A., Wang, B., Chang, X., et al. (2020). Design of Large π-conjugated α-cyanostilbene Derivatives as Colorimetric Sensors for Volatile Acids and Organic Amine Gases. J. Mater. Chem. C 8, 4058–4064. doi:10.1039/c9tc06148g
Cao, X., Gao, A., Hou, J.-t., and Yi, T. (2021). Fluorescent Supramolecular Self-Assembly Gels and Their Application as Sensors: A Review. Coord. Chem. Rev. 434, 213792. doi:10.1016/j.ccr.2021.213792
Chan, C. Y. K., Lam, J. W. Y., Zhao, Z., Chen, S., Lu, P., Sung, H. H. Y., et al. (2014). Aggregation-Induced Emission, Mechanochromism and Blue Electroluminescence of Carbazole and Triphenylamine-Substituted Ethenes. J. Mater. Chem. C 2 (21), 4320–4327. doi:10.1039/C4TC00097H
Chang, Z.-F., Jing, L.-M., Wei, C., Dong, Y.-P., Ye, Y.-C., Zhao, Y. S., et al. (2015). Hexaphenylbenzene-Based, π-Conjugated Snowflake-Shaped Luminophores: Tunable Aggregation-Induced Emission Effect and Piezofluorochromism. Chem. Eur. J. 21 (23), 8504–8510. doi:10.1002/chem.201406311
Chen, L., Jiang, Y., Nie, H., Lu, P., Sung, H. H. Y., Williams, I. D., et al. (2014). Creation of Bifunctional Materials: Improve Electron-Transporting Ability of Light Emitters Based on AIE-Active 2,3,4,5-Tetraphenylsiloles. Adv. Funct. Mater. 24 (23), 3621–3630. doi:10.1002/adfm.201303867
Chen, S., Liu, W., Ge, Z., Zhang, W., Wang, K.-P., and Hu, Z.-Q. (2018). Dimethylamine Substituted Bisbenzocoumarins: Solvatochromic, Mechanochromic and Acidochromic Properties. CrystEngComm 20 (36), 5432–5441. doi:10.1039/C8CE01034J
Choi, S.-K., Rho, J., Yoon, S. E., Seok, J.-H., Kim, H., Min, J., et al. (2020). Full Color Tunable Aggregation-Induced Emission Luminogen for Bioimaging Based on an Indolizine Molecular Framework. Bioconjug. Chem. 31 (11), 2522–2532. doi:10.1021/acs.bioconjchem.0c00467
Dhokale, B., Jadhav, T., Mobin, S. M., and Misra, R. (2015). Meso Alkynylated Tetraphenylethylene (TPE) and 2,3,3-Triphenylacrylonitrile (TPAN) Substituted BODIPYs. J. Org. Chem. 80 (16), 8018–8025. doi:10.1021/acs.joc.5b01117
Feng HT, H.-T., Yuan, Y.-X., Xiong, J.-B., Zheng, Y.-S., and Tang, B. Z. (2018). Macrocycles and Cages Based on Tetraphenylethylene with Aggregation-Induced Emission Effect. Chem. Soc. Rev. 47 (19), 7452–7476. doi:10.1039/C8CS00444G
Feng X, X., Qi, C., Feng, H.-T., Zhao, Z., Sung, H. H. Y., Williams, I. D., et al. (2018). Dual Fluorescence of Tetraphenylethylene-Substituted Pyrenes with Aggregation-Induced Emission Characteristics for White-Light Emission. Chem. Sci. 9 (25), 5679–5687. doi:10.1039/C8SC01709C
Gao, C., Hossain, M. K., Wahab, M. A., Xiong, J., Qiu, B.-M., Luo, H., et al. (2019). Understanding the Details of Aggregation-Induced Emission (AIE) Effect in D-π-A Type Imidazolium-Based Compounds through the Stepwise Change of Rotatable Moieties. Dyes Pigm. 160, 909–914. doi:10.1016/j.dyepig.2018.08.032
Gomez-Duran, C. F. A., Hu, R., Feng, G., Li, T., Bu, F., Arseneault, M., et al. (2015). Effect of AIE Substituents on the Fluorescence of Tetraphenylethene-Containing BODIPY Derivatives. ACS Appl. Mater. Inter. 7 (28), 15168–15176. doi:10.1021/acsami.5b05033
Gundu, S., Kim, M., Mergu, N., and Son, Y.-A. (2017). AIE-active and Reversible Mechanochromic Tetraphenylethene-Tetradiphenylacrylonitrile Hybrid Luminogens with Re-writable Optical Data Storage Application. Dyes Pigm. 146, 7–13. doi:10.1016/j.dyepig.2017.06.043
Hong, Y., Lam, J. W. Y., and Tang, B. Z. (2009). Aggregation-Induced Emission: Phenomenon, Mechanism and Applications. Chem. Commun. 29, 4332–4353. doi:10.1039/b904665h
Hong, Y., Lam, J. W. Y., and Tang, B. Z. (2011). Aggregation-Induced Emission. Chem. Soc. Rev. 40 (11), 5361–5388. doi:10.1039/c1cs15113d
Huang, M., Lu, H., Wang, K., Liu, B., Wang, M., Qiao, X., et al. (2021). A Facile Design of Azaanthracene Derivatives: ACQ-AIE Conversion and Blue-Shifted Mechanofluorochromic Emission. Dyes Pigm. 186, 108992. doi:10.1016/j.dyepig.2020.108992
Kuerschner, L., Moessinger, C., and Thiele, C. (2008). Imaging of Lipid Biosynthesis: How a Neutral Lipid Enters Lipid Droplets. Traffic 9 (3), 338–352. doi:10.1111/j.1600-0854.2007.00689.x
La, D. D., Bhosale, S. V., Jones, L. A., and Bhosale, S. V. (2018). Tetraphenylethylene-Based AIE-Active Probes for Sensing Applications. ACS Appl. Mater. Inter. 10 (15), 12189–12216. doi:10.1021/acsami.7b12320
Lee, C., Yang, W., and Parr, R. G. (1988). Development of the Colle-Salvetti Correlation-Energy Formula into a Functional of the Electron Density. Phys. Rev. B 37 (2), 785–789. doi:10.1103/PhysRevB.37.785
Li A, A., Liu, Y., Han, L., Xu, S., Song, C., Geng, Y., et al. (2019). Pressure-Induced Remarkable Luminescence-Changing Behaviours of 9, 10-Distyrylanthracene and its Derivatives with Distinct Substituents. Dyes Pigm. 161, 182–187. doi:10.1016/j.dyepig.2018.09.041
Li J, J., Wang, J., Li, H., Song, N., Wang, D., and Tang, B. Z. (2020). Supramolecular Materials Based on AIE Luminogens (AIEgens): Construction and Applications. Chem. Soc. Rev. 49, 1144–1172. doi:10.1039/c9cs00495e
Li P, P., Zhang, D., Zhang, Y., Lu, W., Zhang, J., Wang, W., et al. (2019). Aggregation-Caused Quenching-type Naphthalimide Fluorophores Grafted and Ionized in a 3D Polymeric Hydrogel Network for Highly Fluorescent and Locally Tunable Emission. ACS Macro Lett. 8 (8), 937–942. doi:10.1021/acsmacrolett.9b00337
Li Y, Y., Liu, S., Ni, H., Zhang, H., Zhang, H., Chuah, C., et al. (2020). ACQ‐to‐AIE Transformation: Tuning Molecular Packing by Regioisomerization for Two‐Photon NIR Bioimaging. Angew. Chem. Int. Ed. 59 (31), 12822–12826. doi:10.1002/anie.202005785
Lim, S., Tang, B. Z., and Hong, Y. (2016). “AIE Luminogens for Visualizing Cell Structures and Functions,” in Aggregation-Induced Emission: Materials and Applications. Editors M. Fujiki, B. Liu, and B. Z. Tang (Washington, DC: American Chemical Society), 1227, 199–216. doi:10.1021/bk-2016-1227.ch008
Liu, X., Qiao, Q., Tian, W., Liu, W., Chen, J., Lang, M. J., et al. (2016). Aziridinyl Fluorophores Demonstrate Bright Fluorescence and Superior Photostability by Effectively Inhibiting Twisted Intramolecular Charge Transfer. J. Am. Chem. Soc. 138 (22), 6960–6963. doi:10.1021/jacs.6b03924
Liu, J., Zhang, C., Dong, J., Zhu, J., Shen, C., Yang, G., et al. (2017). Endowing a Triarylboron Compound Showing ACQ with AIE Characteristics by Transforming its Emissive TICT State to Be Dark. RSC Adv. 7 (24), 14511–14515. doi:10.1039/C7RA00426E
Long, X., Wu, J., Yang, S., Deng, Z., Zheng, Y., Zhang, W., et al. (2021). Discovery of and Insights into One-Photon and Two-Photon Excited ACQ-To-AIE Conversion via Positional Isomerization. J. Mater. Chem. C 9 (35), 11679–11689. doi:10.1039/D1TC01963E
Lou, X. Y., and Yang, Y. W. (2020). Aggregation‐induced Emission Systems Involving Supramolecular Assembly. Aggregate 1, 19–30. doi:10.1002/agt2.1
Lu, H., Xu, B., Dong, Y., Chen, F., Li, Y., Li, Z., et al. (2010). Novel Fluorescent PH Sensors and a Biological Probe Based on Anthracene Derivatives with Aggregation-Induced Emission Characteristics. Langmuir 26 (9), 6838–6844. doi:10.1021/la904727t
Lu, Q., Li, X., Li, J., Yang, Z., Xu, B., Chi, Z., et al. (2015). Influence of Cyano Groups on the Properties of Piezofluorochromic Aggregation-Induced Emission Enhancement Compounds Derived from Tetraphenylvinyl-Capped Ethane. J. Mater. Chem. C 3 (6), 1225–1234. doi:10.1039/C4TC02165G
Lu, H., Wang, K., Liu, B., Wang, M., Huang, M., Zhang, Y., et al. (2019). Systematic Oligoaniline-Based Derivatives: ACQ-AIE Conversion with a Tunable Insertion Effect and Quantitative Fluorescence "Turn-On" Detection of BSA. Mater. Chem. Front. 3 (2), 331–338. doi:10.1039/C8QM00543E
Luo, J., Xie, Z., Lam, J. W. Y., Cheng, L., Tang, B. Z., Chen, H., et al. (2001). Aggregation-Induced Emission of 1-Methyl-1,2,3,4,5-Pentaphenylsilole. Chem. Commun. 18, 1740–1741. doi:10.1039/b105159h
Ma, X., Sun, R., Cheng, J., Liu, J., Gou, F., Xiang, H., et al. (2016). Fluorescence Aggregation-Caused Quenching versus Aggregation-Induced Emission: A Visual Teaching Technology for Undergraduate Chemistry Students. J. Chem. Educ. 93 (2), 345–350. doi:10.1021/acs.jchemed.5b00483
Mallick, S., Arathi, A. S., and Koner, A. L. (2017). Customized Tuning of Aggregation-Induced Emission of a Napthalimide Dye by Surfactants and Cyclodextrin. J. Colloid Interf. Sci. 499, 46–53. doi:10.1016/j.jcis.2017.03.097
Mei, J., Leung, N. L. C., Kwok, R. T. K., Lam, J. W. Y., and Tang, B. Z. (2015). Aggregation-Induced Emission: Together We Shine, United We Soar!. Chem. Rev. 115 (21), 11718–11940. doi:10.1021/acs.chemrev.5b00263
Niu, C., You, Y., Zhao, L., He, D., Na, N., and Ouyang, J. (2015). Solvatochromism, Reversible Chromism and Self‐Assembly Effects of Heteroatom‐Assisted Aggregation‐Induced Enhanced Emission (AIEE) Compounds. Chem. Eur. J. 21 (40), 13983–13990. doi:10.1002/chem.201501902
Pati, A. K., Mohapatra, M., Ghosh, P., Gharpure, S. J., and Mishra, A. K. (2013). Deciphering the Photophysical Role of Conjugated Diyne in Butadiynyl Fluorophores: Synthesis, Photophysical and Theoretical Study. J. Phys. Chem. A. 117 (30), 6548–6560. doi:10.1021/jp404809g
Pati, A. K., Gharpure, S. J., and Mishra, A. K. (2015). On the Photophysics of Butadiyne Bridged Pyrene-Phenyl Molecular Conjugates: Multiple Emissive Pathways through Locally Excited, Intramolecular Charge Transfer and Excimer States. Faraday Discuss. 177, 213–235. doi:10.1039/C4FD00170B
Pratihar, S., Bhattacharyya, A., and Prasad, E. (2020). Achieving ACQ-AIE Modulation Using Isostructural Organic Fluorophores. J. Photochem. Photobiol. A Chem. 396, 112458. doi:10.1016/j.jphotochem.2020.112458
Ravindran, E., and Somanathan, N. (2017). Efficient white-light Emission from a Single Polymer System with "spring-like" Self-Assemblies Induced Emission Enhancement and Intramolecular Charge Transfer Characteristics. J. Mater. Chem. C 5 (19), 4763–4774. doi:10.1039/C7TC01036B
Sasaki, S., Suzuki, S., Igawa, K., Morokuma, K., and Konishi, G.-i. (2017). The K-Region in Pyrenes as a Key Position to Activate Aggregation-Induced Emission: Effects of Introducing Highly Twisted N,N-Dimethylamines. J. Org. Chem. 82 (13), 6865–6873. doi:10.1021/acs.joc.7b00996
Shen, X. Y., Wang, Y. J., Zhao, E., Yuan, W. Z., Liu, Y., Lu, P., et al. (2013). Effects of Substitution with Donor-Acceptor Groups on the Properties of Tetraphenylethene Trimer: Aggregation-Induced Emission, Solvatochromism, and Mechanochromism. J. Phys. Chem. C 117 (14), 7334–7347. doi:10.1021/jp311360p
Sun, H., Tang, X.-X., Miao, B.-X., Yang, Y., and Ni, Z. (2018). A New AIE and TICT-Active Tetraphenylethene-Based Thiazole Compound: Synthesis, Structure, Photophysical Properties and Application for Water Detection in Organic Solvents. Sensors Actuators B Chem. 267, 448–456. doi:10.1016/j.snb.2018.04.022
Walther, T. C., and Farese, R. V. (2009). The Life of Lipid Droplets. Biochim. Biophys. Acta Mol. Cel Biol. Lipids 1791 (6), 459–466. doi:10.1016/j.bbalip.2008.10.009
Wang, Z., Wang, C., Fang, Y., Yuan, H., Quan, Y., and Cheng, Y. (2018). Color-Tunable AIE-Active Conjugated Polymer Nanoparticles as Drug Carriers for Self-Indicating Cancer Therapy via Intramolecular FRET Mechanism. Polym. Chem. 9 (23), 3205–3214. doi:10.1039/C8PY00329G
Wang, H., Xing, H., Gong, J., Zhang, H., Zhang, J., Wei, P., et al. (2020). "Living" Luminogens: Light Driven ACQ-To-AIE Transformation Accompanied with Solid-State Actuation. Mater. Horiz. 7 (6), 1566–1572. doi:10.1039/D0MH00447B
Wu, B., Xue, T., Wang, W., Li, S., Shen, J., and He, Y. (2018). Visible Light Triggered Aggregation-Induced Emission Switching with a Donor-Acceptor Stenhouse Adduct. J. Mater. Chem. C 6 (31), 8538–8545. doi:10.1039/C8TC02621A
Xiong, Y., Yan, X., Ma, Y., Li, Y., Yin, G., and Chen, L. (2015). Regulating the Piezofluorochromism of 9,10-Bis(Butoxystyryl)Anthracenes by Isomerization of Butyl Groups. Chem. Commun. 51 (16), 3403–3406. doi:10.1039/C4CC10196K
Yuan, W. Z., Lu, P., Chen, S., Lam, J. W. Y., Wang, Z., Liu, Y., et al. (2010). Changing the Behavior of Chromophores from Aggregation-Caused Quenching to Aggregation-Induced Emission: Development of Highly Efficient Light Emitters in the Solid State. Adv. Mater. 22 (19), 2159–2163. doi:10.1002/adma.200904056
Yuan, M.-S., Wang, D.-E., Xue, P., Wang, W., Wang, J.-C., Tu, Q., et al. (2014). Fluorenone Organic Crystals: Two-Color Luminescence Switching and Reversible Phase Transformations between π-π Stacking-Directed Packing and Hydrogen Bond-Directed Packing. Chem. Mater. 26 (7), 2467–2477. doi:10.1021/cm500441r
Zhang, X., Chi, Z., Zhang, J., Li, H., Xu, B., Li, X., et al. (2011). Piezofluorochromic Properties and Mechanism of an Aggregation-Induced Emission Enhancement Compound Containing N-Hexyl-Phenothiazine and Anthracene Moieties. J. Phys. Chem. B 115 (23), 7606–7611. doi:10.1021/jp202112e
Zhang, Y., Sun, J., Lv, X., Ouyang, M., Cao, F., Pan, G., et al. (2013). Heating and Mechanical Force-Induced "turn on" Fluorescence of Cyanostilbene Derivative with H-type Stacking. CrystEngComm 15 (44), 8998–9002. doi:10.1039/c3ce41221k
Zhang, H., Zhang, H., Huang, G., and Li, B. S. (2021). Two Tetraphenylethene-Pyrene Isomers: Distinct Fluorescence and Mechanochromic Properties. Dyes Pigm. 185, 108947. doi:10.1016/j.dyepig.2020.108947
Zhang, X., Ma, Z., Yang, Y., Zhang, X., Jia, X., and Wei, Y. (2014). Fine-tuning the Mechanofluorochromic Properties of Benzothiadiazole-Cored Cyano-Substituted Diphenylethene Derivatives through D-A Effect. J. Mater. Chem. C 2 (42), 8932–8938. doi:10.1039/C4TC01457J
Zhang, Y., Sun, J., Zhuang, G., Ouyang, M., Yu, Z., Cao, F., et al. (2014). Heating and Mechanical Force-Induced Luminescence On-Off Switching of Arylamine Derivatives with Highly Distorted Structures. J. Mater. Chem. C 2 (1), 195–200. doi:10.1039/C3TC31416B
Keywords: aggregation-induced emission, aggregation-caused quenching, reigoisomerization, multi-stimuli responsive, LDs imaging
Citation: Wang Z, Li R, Chen L, Zhai X, Liu W, Lin X, Chen L, Chen N, Sun S, Li Z, Hao J, Chen X, Lin B and Xie L (2022) Precise Molecular Design of a Pair of New Regioisomerized Fluorophores With Opposite Fluorescent Properties. Front. Chem. 9:823519. doi: 10.3389/fchem.2021.823519
Received: 27 November 2021; Accepted: 30 December 2021;
Published: 20 January 2022.
Edited by:
Lucas Rodrigues, University of São Paulo, BrazilReviewed by:
Xinhua Cao, Xinyang Normal University, ChinaCopyright © 2022 Wang, Li, Chen, Zhai, Liu, Lin, Chen, Chen, Sun, Li, Hao, Chen, Lin and Xie. This is an open-access article distributed under the terms of the Creative Commons Attribution License (CC BY). The use, distribution or reproduction in other forums is permitted, provided the original author(s) and the copyright owner(s) are credited and that the original publication in this journal is cited, in accordance with accepted academic practice. No use, distribution or reproduction is permitted which does not comply with these terms.
*Correspondence: Xueyuan Chen, eGNoZW5AZmppcnNtLmFjLmNu; Bin Lin, cmFuZHliaW5saW5AZ21haWwuY29t; Lijun Xie, bGlqdW54aWU4MjI0QG91dGxvb2suY29t
†These authors have contributed equally to this work and share first authorship
Disclaimer: All claims expressed in this article are solely those of the authors and do not necessarily represent those of their affiliated organizations, or those of the publisher, the editors and the reviewers. Any product that may be evaluated in this article or claim that may be made by its manufacturer is not guaranteed or endorsed by the publisher.
Research integrity at Frontiers
Learn more about the work of our research integrity team to safeguard the quality of each article we publish.