- 1College of Materials Science and Engineering, Zhejiang University of Technology, Hangzhou, China
- 2Key Laboratory of Bioorganic Phosphorus Chemistry and Chemical Biology, Department of Chemistry, Tsinghua University, Beijing, China
- 3Key Laboratory and Engineering Laboratory of Lymphatic Surgery Jilin Province, China-Japan Union Hospital of Jilin University, Changchun, China
A hydrophilic TPE-based tetracationic cyclophane TPE-cyc was synthesized, which could capture intracellular Nicotinamide adenine dinucleotide phosphate and fuel the antioxidative ability of tumor cells to detoxify reactive oxygen species (ROS). Meanwhile, upon the reduction by cellular GSH, TPE-cyc could light up tumor cells, acting as a GSH-responsive fluorescent switch to image cells with high resolution.
Introduction
Understanding the significance of molecular recognition in complex biological processes where various nucleic acids, enzymes and nucleotides are involved, has helped chemists to develop intelligent structures with fascinating properties (Meyer et al., 2003; Wu et al., 2017; Wu D et al., 2021). Artificial molecular receptors specific to biomolecules have attracted increasing attentions in recent years owing to their potential applications in medical and biological fields (Jisha et al., 2006; Hariharan et al., 2007; Pluth and Raymond, 2007; Kuruvilla et al., 2008; Ojida et al., 2008; Jisha et al., 2010; Alfonso and Sola, 2020; Escobar and Pablo, 2021). Nicotinamide adenine dinucleotide phosphate (NADPH), a main cellular reductant, plays an important role in maintaining glutathione in its reduced modality (GSH), which eliminates intracellular reactive oxygen species (ROS), thus preventing cells from oxidative damage (Dröge, 2002; Ying, 2008; Celton et al., 2012; Schulze and Harris, 2012; Fernandez-Marcos et al., 2016). Although the probable mechanisms of NADPH-involved physiological processes have been proposed, deeper exploration is still needed due to the complexity and uncertainty of the existing mechanisms. Therefore, a molecular recognition system which can selectively recognize NADPH is urgently needed.
The discovery of crown ethers by Pedersen opened the way for supramolecular chemists to design macrocyclic molecules that act as molecular receptors based on non-covalent interactions or weak coordination (Pedersen, 1967). Since then, chemists have constructed various macrocyclic hosts such as cyclodextrins (Liao et al., 2010; Crini, 2014; Prochowicz et al., 2017; Chen et al., 2021), cucurbit [n]turils (Ong et al., 2002; Jeon et al., 2004; Lagona et al., 2005; Das and Scherman, 2011; Francisco et al., 2019), calix [n]arenes (Ikeda and Shinkai, 1997; Philip kaifer 2002; Sameni et al., 2009; Perret and Coleman, 2011; Li et al., 2020), cyclophanes (Ariga et al., 2005; Si et al., 2014; Strutt et al., 2014; Liu et al., 2017; Sapotta et al., 2019; Neira et al., 2020) and pillararenes (Cao et al., 2014; Li et al., 2014; Ogoshi et al., 2016; Sathiyajith et al., 2017; Guo H et al., 2020; .Cai et al., 2021). The discovery of “blue box” by Stoddart et al. opened the new era of cationic cyclophanes (Gong et al., 2010; Gong et al., 2011; Dale et al., 2016). Cationic cyclophanes are good candidates for molecular recognition because they not only possess multi-cationic states but also display self-assembly behavior by incorporating π-electron-rich guests (Trabolsi et al., 2010a; Barnes et al., 2015; Cheng et al., 2015; Chen et al., 2016; Sun et al., 2017). For example, tetracationic cyclophanes which are constructed on the basis of π-electron-deficient 4,4’-bipyridinium units, can selectively complex with π-electron-rich guests to form 1:1 or 1:2 complexes (Bühner et al., 1988; Philp et al., 1991; Trabolsi et al., 2010b). Recently, tetraphenylethene (TPE) derivatives which are a classic aggregation-induced emission (AIE) luminophores (Zhou et al., 2018; Ding et al., 2021; Wu H et al., 2021), have been utilized as building blocks to construct macrocyclic compounds (Mei et al., 2015). Attributing to the AIE effect and propeller structure, the TPE-based cyclophanes not only display excellent photophysical properties but also possess flexible and diversified cavity structures which can be explored to capture biomolecules (Luo et al., 2012; Zhao et al., 2012; Kwok et al., 2015). Although a number of TPE-based cationic cyclophanes have been utilized for host–guest recognition, the example of biomolecules recognition in aqueous media by hydrophilic TPE-based cationic cyclophanes is rare.
Here, we synthesized a hydrophilic TPE-based tetracationic cyclophane (TPE-cyc), in which TPE and 4.4’-bipyridinium units acted as building blocks. Attributing to the electrostatic interactions and π-π stacking, TPE-cyc could specifically recognize NADPH and complex it in a 1:1 manner. After TPE-cyc being internalized by tumor cells, TPE-cyc could capture cellular NADPH to partially break the equilibrium of NADPH-generating reaction (NADH + NADP+→NADPH + NAD+) and eventually fuel the NADPH-dependent antioxidative ability to detoxify ROS. Meanwhile, the high concentration of GSH in tumor cells could reduce the 4,4’-bipyridinium (MV) units of TPE-cyc into radical cation state and disrupt the photo-induced electron transfer (PET) effect between electron-rich TPE and electron-deficient bipyridinium units, thus recovering the fluorescence of TPE units and lighting up tumor cells (Scheme 1). Hence, TPE-cyc acts as a GSH-responsive fluorescent switch to image cells with high resolution.
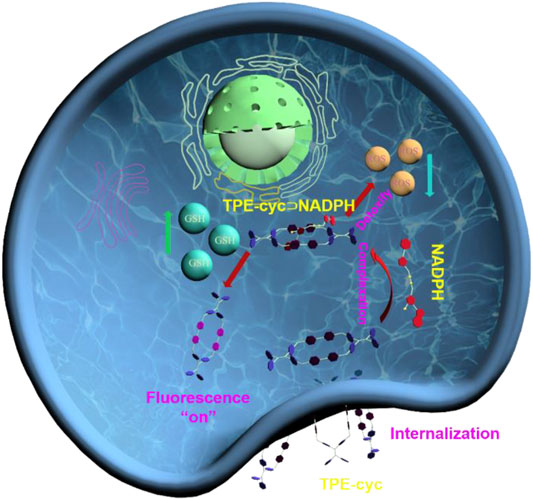
SCHEME 1. Schematic illustration of the changes of cellular metabolic function regulated by TPE-cyc⊃NADPH.
Materials and Methods
Materials
NADPH was purchased from Sigma. TPE-cyc was synthesized according to literature procedures (Cheng et al., 2019). 1H NMR and 13C NMR spectra were recorded on a Bruker AvanceⅢ-400 spectrometry. The 2D NOESY NMR spectra were recorded on a Bruker Avance DMX 600 spectrophotometer with TMS as the internal reference. UV-vis-NIR spectra were taken on a Shimadzu UV-3150 spectrophotometer. The fluorescence experiments were measured on an RF-5301 spectrofluorophotometer (Shimadzu Corporation, Japan). The isothermal titration calorimetry (ITC) experiments were performed on a VP-ITC micro-calorimeter (Microcal, United States). The cell images were taken by a confocal laser scanning microscopy (CLSM, Radiance2100, Bio-Rad) with a 100 × oil immersion lens. Flow cytometry measurements were conducted using a FACSCalibur flow cytometer (BD FACSCalibur).
Methods
Synthesis of Compound 3
37.1 ml n-butyllithium (1.6 M) was added into a solution of 1 (10 g, 59.4 mmol) in dry THF (100 ml) at 0°C under N2 atmosphere. The orange-red solution was stirred for 0.5 h at 0°C. Then, 2 (6.3 g, 29.7 mmol) was dissolved in dry THF and dropwise added to the above mixture. The resulting solution was allowed to warm to room temperature and still stirred for 8 h. At last, saturated ammonium chloride solution was added to quench the reaction. The mixture was extracted with DCM three times. The organic phase were combined and dried over anhydrous Na2SO4. The solvent was removed by rotary evaporation and the acquired crude product was dissolved in toluene with the 4Å molecular sieve dehydration unit. After addition of catalytic p-toluenesulphonic acid (342 mg, 1.8 mmol), the toluene solution was refluxed for 5 h and the generated H2O was separated. The organic layer was washed with 10% NaHCO3 aqueous solution and dried over anhydrous Na2SO4. After removal of toluene, the obtained mixture was purified by column chromatography (silica gel; petroleum ether) to obtain 3 as a white solid (9.0 g, 85%).
Synthesis of Compound 4
Under a N2 atmosphere, 3 (1.1 g, 2.83 mmol) was first dissolved in CCl4 (20 ml), then dibenzoyl peroxide (50 mg, 0.2 mmol) and NBS (1.5 g, 8.49 mmol) were added. The mixture was heated to reflux for 12 h. After reaction, the solution was filtered to remove solid impurity and the CCl4 solution was washed with brine three times. The organic phase were collected and dried by anhydrous Na2SO4. The CCl4 was evaporated and the crude product was purified by a silica gel column chromatography (silica gel; petroleum ether) to obtain 4 as a white solid (0.9 g, 60%).
Synthesis of Compound 5
4,4’-bipyridine (2.0 g, 12.8 mmol) was dissolved in CH3CN (20 ml)and was heated to reflux. Next, compound 4 (1.2 mg, 2.3 mmol) was dissolved in CH3CN (5 ml) and dropwise added to the bipyridine solution. The resulting mixture was refluxed for 3 days. The formed precipitate was filtered and washed with CH3CN three times, and compound 5 was obtained after dry under high vacuum (1.7 g, 90%).
Synthesis of Compound 6
Tetrabutylammonium iodide (TBAI, 35 mg, 0.095 mmol), 4 (220 mg, 0.42 mmol) and 5 (400 mg, 0.42 mmol) were dissolved in dry CH3CN (100 ml) and was heated at 85°C for 72 h. After reaction, the orange product was acquired by centrifuge and dried under high vacuum. After NH4PF6 anion conversion in water, six was obtained as a pale yellow solid (250 mg, 37%).
Synthesis of Compound TPE-Cyc
Tetrabutylammonium chloride (TBACl, 205.66 mg, 0.74 mmol) and 6 (100 mg, 0.074 mmol) were dissolved in CH3CN and stirred overnight. After reaction, the orange terreous product was acquired by centrifuge and washed with CH3CN three times. TPE-cyc was obtained after dry in high vacuum (61 mg, 90%).
Results and Discussion
Investigation of Host–Guest Complexation Between TPE-Cyc and NADPH
TPE-cyc was synthesized via a two-step SN2 reaction as shown in Scheme 1. To investigate the host–guest interaction between TPE-cyc and NADPH, 1H NMR spectroscopy was conducted in D2O. As shown in Figures 1A,B, when an equimolar amount of NADPH and TPE-cyc were mixed in D2O, apparent chemical shift changes of the protons on TPE-cyc were observed. For instance, the signals of protons H1, H2 and H3 on TPE-cyc were divided into multiple sets of sharp signals with obvious chemical shift changes, which may be induced by the electrostatic interaction between the pyridinium unit and tetraphosphate unit of NADPH. Meanwhile, the signals of protons H4-8 disappeared completely after complexation. All these results provided compelling evidence for the host–guest interactions between TPE-cyc and NADPH (Cheng et al., 2019). In addition, the signals of protons on NADPH also displayed obvious peak broadening effect, further indicating the occurrence of host–guest complexation. On the other hand, there were H1-Hd-k and H2-Hd-k inter-correlation between TPE-cyc and NADPH in nuclear overhauser effect spectroscopy (NOESY) spectrum (Figure 2A), confirming the formation of TPE-cyc⊃NADPH.
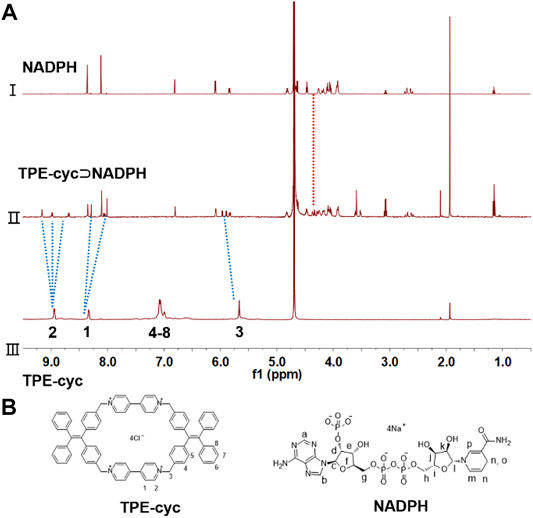
FIGURE 1. (A) Partial 1H NMR spectra (D2O, room temperature, 400 MHz): (Ⅰ) NADPH (1.00 mM). (Ⅱ) TPE-cyc⊃NADPH [TPE-cyc (1.00 mM) and NADPH (1.00 mM)]. (Ⅲ) TPE-cyc (1.00 mM). (B) Chemical structures of TPE-cyc and NADPH.
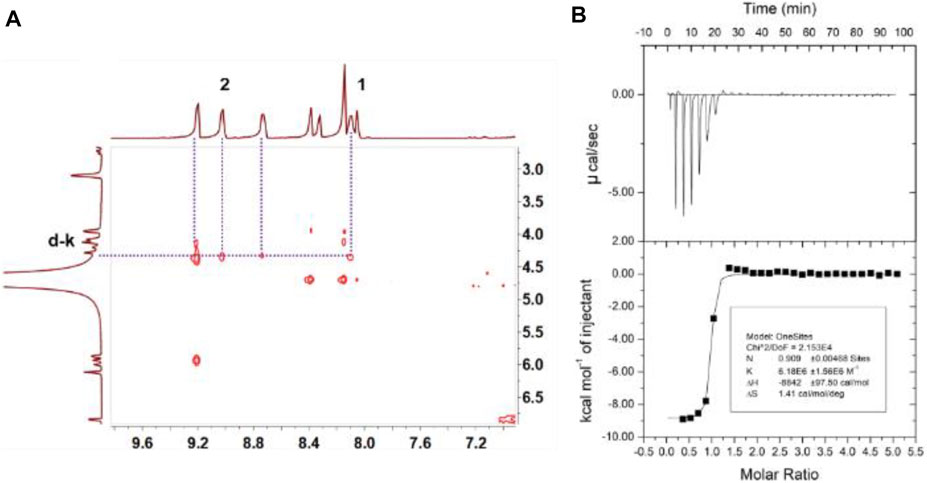
FIGURE 2. (A) Partial NOESY NMR spectra (600 MHz, D2O, room temperature) of TPE-cyc (1.0 mM) and NADPH (1.0 mM). (B) Microcalorimetric titration of TPE-cyc (2.00 mM, 10 µl per injection) with NADPH (0.100 mM) in water at 298.15 K.
Isothermal titration calorimetry (ITC) experiment was carried to provide the thermodynamic energy information for the complexation. As shown in Figure 2B, the Ka value of TPE-cyc⊃NADPH was calculated to be (6.18 ± 1.56) × 106 M−1 and the stoichiometry was 1:1. The acquired information of entropy and enthalpy changes (ΔH° < 0; TΔS° > 0) from ITC data demonstrated that this supramolecular complexation was promoted by a beneficial entropy-assisted enthalpy change. The driving forces of the molecular recognition were the synergistic effect of hydrophobic interaction, electrostatic interaction and π-π stacking interaction. Furthermore, there was a fragment peak m/z = 948.3 [corresponding to (TPE-cyc‧NADPH-3Cl-H)2+] in electrospray ionization mass spectrometry (Supplementary Figure S9), which further demonstrated the formation of a 1:1 TPE-cyc⊃NADPH complex. The UV-vis absorption was also conducted to investigate the complexation between TPE-cyc and NADPH. As shown in Figure 3A, by the continual addition of NADPH into TPE-cyc solution, the maximum absorbance of TPE-cyc at 250 nm gradually decreased and moved to 258 nm, and the maximum decrease occurred when 1.0 equiv. of NADPH was added (Figure 3B), supporting a 1:1 stoichiometry of TPE-cyc⊃NADPH.
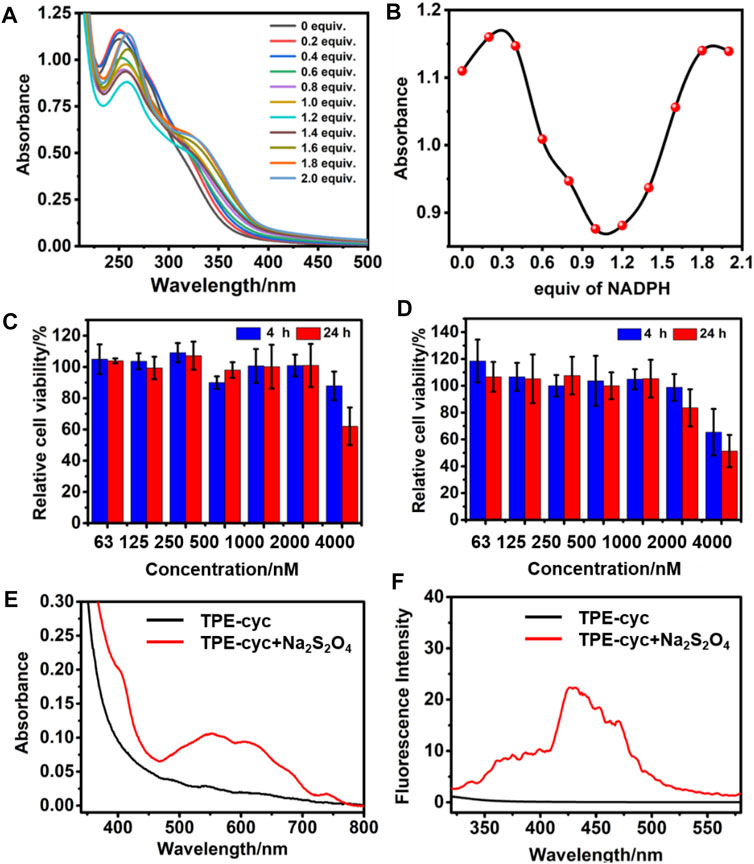
FIGURE 3. (A) UV/vis spectra of TPE-cyc in the presence of different amounts of NADPH. (B) The plots of UV absorption maximum of TPE-cyc in the presence of different amounts of NADPH. Cytotoxicity of (C) HeLa and (D) U87 cells incubated with different concentrations of TPE-cyc for 4 and 24 h (E) UV/vis spectra of TPE-cyc with or without Na2S2O4. (F) Fluorescence spectra of TPE-cyc with or without Na2S2O4.
Investigation of Cell Viability Regulated by TPE-Cyc
NADPH is known as a crucial co-enzyme in the event of cellular electron transfer which drives the biosynthesis of amino acids, DNA, phospholipids, fatty acids and steroids. Another important function of NADPH originating from the powerful reducibility of NADPH is to fuel the activities of various enzymes, such as glutathione peroxidase (GSHPx), catalase and superoxide dismutase, which play an important role in permitting microorganisms to survive in aerobic environment. Hence, breaking the balance of NADPH in living system can induce severe damage for cells, even death. Considering the strong complexing ability of TPE-cyc for NADPH, we investigate the impact of TPE-cyc on biological functions of cells.
The cell viability was firstly assessed by a 3-(4′,5′-dimethylthiazol-2′-yl)-2,5-diphenyl tetrazolium bromide (MTT) assay, wherein U87 and HeLa cells were incubated with different concentrations of TPE-cyc for 4 or 24 h. As shown in Figures 3C,D, cell viability maintained nearly 100% survival rate in the range of 0.063–1.0 μM, suggesting this concentration range of TPE-cyc could not disrupt the NADPH balance. However, the survival rate decreased with the further increase of concentration. For example, when the concentration reached 4 μM, the survival rate fell by half (Figures 3C,D), indicating this concentration of TPE-cyc could capture enough intracellular NADPH and eventually induce cell death. In addition, the cytotoxicity of six was similar to TPE-cyc, suggesting tetracationic macrocyclic structure was the key of cytotoxicity against cells (Supplementary Figure S11).
Investigation of Antioxidative Ability of Tumor Cells Regulated by TPE-Cyc
NADPH has an important ability to keep glutathione in its reduction form GSH, which eliminates ROS and transforms harmful hydrogen peroxide into water under the help of GSHPx (Margis et al., 2008). Therefore, NADPH plays a vital role in resisting cellular oxidative stress. We utilized 2′,7′-dichlorofluorescin diacetate (DCF-DA) as a fluorescence probe to monitor the intracellular ROS level (Li et al., 2018; Ren et al., 2020). DCFH-DA itself does not emit fluorescence, but the intracellular ROS can oxidize the non-emissive DCF-DA into emissive DCF. As shown in Supplementary Figure S12, with the increase of incubation time, the level of ROS in TPE-cyc group decreased, suggesting the antioxidative ability of cells was enhanced. Flow cytometry (FCM) was also applied to quantitatively analyze the level of intracellular ROS. Similar to the result of fluorescence imaging, ROS production significantly decreased with the extension of time (Figure 4A). The reason may be ascribed that the capture of intracellular NADPH by TPE-cyc broke the equilibrium of NADPH-involving redox process and triggered the generation of reducing substances which can detoxify ROS.
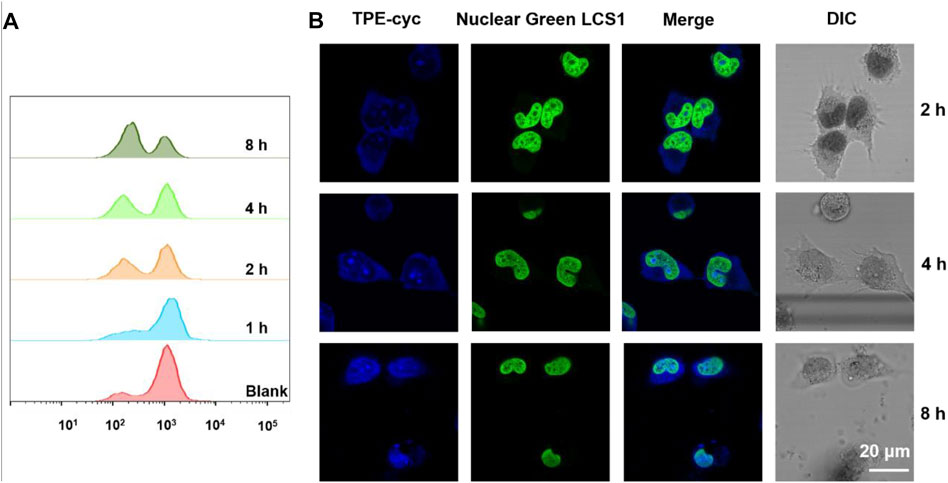
FIGURE 4. (A) Flow cytometry results of ROS fluorescence signals in HeLa cells incubated with TPE-cyc for different time periods. (B) Confocal images of HeLa cells incubated with TPE-cyc for different time periods (Nuclear Green LCS1 was used to stain nuclei of living HeLa cells).
Investigation of Cell Imaging Ability of TPE-Cyc
Owing to the PET effect between TPE and bipyridinium units, water-soluble TPE-cyc is a quencher-type host and it has an intense UV absorption but no fluorescence (Hayashida and Kaku, 2013), which severely limits its expansion and application. In the reductive condition, MV2+ can be reduced into MV⋅+ and the PET effect between TPE and bipyridinium units is disrupted (Jiao et al., 2019; Guo Q. H et al., 2020; Zhang et al., 2020), which may be used to initiate the fluorescence of TPE-cyc. As shown in Figure 3E, there was a characteristic peak of MV⋅+ in the range of 450–750 nm after addition of Na2S2O4, indicating MV2+ was reduced into MV⋅+ with the help of reducing agent (Lomoth et al., 2002). As expected, there was a new fluorescence emission within the range of 300–550 nm in the TPE-cyc + Na2S2O4 group, suggesting the fluorescence of TPE-cyc was initiated. In tumor cells, the concentration of GSH is high, which can be utilized to light up TPE-cyc. Confocal laser scanning microscopy (CLSM) was utilized to study the internalization behavior of TPE-cyc. As shown in Figure 4B, after 2 h incubation, apparent blue fluorescence arising from TPE-cyc was observed in the cytoplasm, indicating that TPE-cyc was easily internalized by HeLa cells. With the incubation time increased to 8 h, the intensity of blue fluorescence significantly increased, suggesting the endocytosis of TPE-cyc occurred in a time-dependent mode and intracellular reducing environment ensured continuous luminous of TPE-cyc, which provided a crucial advantage for TPE-cyc to be applied in fluorescence imaging.
Conclusion
In summary, a hydrophilic tetracationic cyclophane was constructed based on TPE and 4,4’-bipyridinium units. Attributing to the large and flat rectangle-like cavity, TPE-cyc could specifically recognize NADPH and form a 1:1 host–guest complex. TPE-cyc was not only easily phagocytized by tumor cells but also able to selectively capture cellular NADPH, thus breaking the equilibrium of NADPH-involving redox process and improving the antioxidative ability to eliminate ROS. Meanwhile, the sufficient intracellular reducing environment such as the high concentration of GSH reduced MV2+ unit of TPE-cyc into MV⋅+ to forbid the PET effect between TPE and MV units, realizing the fluorescence recovery of TPE-cyc and eventually fluorescence imaging of tumor cells with high resolution. This current study opens a door for cationic cyclophanes to broaden their biological applications in recognizing biomacromolecules and imaging tumor cells, which has a great potential to diagnose and treat difficult miscellaneous diseases of humans in the future.
Data Availability Statement
The original contributions presented in the study are included in the article/Supplementary Material, further inquiries can be directed to the corresponding authors.
Author Contributions
DW and SQ conceived and designed the research DW, XY, and BB prepared TPE-cyc and measured the photophysical properties. ZZ performed the in vitro experiments and analysed the data. DW and SQ co-wrote the paper.
Funding
This work was supported by the Zhejiang Provincial Natural Science Foundation of China (LQ20B040001).
Conflict of Interest
The authors declare that the research was conducted in the absence of any commercial or financial relationships that could be construed as a potential conflict of interest.
Publisher’s Note
All claims expressed in this article are solely those of the authors and do not necessarily represent those of their affiliated organizations, or those of the publisher, the editors and the reviewers. Any product that may be evaluated in this article, or claim that may be made by its manufacturer, is not guaranteed or endorsed by the publisher.
Supplementary Material
The Supplementary Material for this article can be found online at: https://www.frontiersin.org/articles/10.3389/fchem.2021.817720/full#supplementary-material
References
Alfonso, I., and Solà, J. (2020). Molecular Recognition of Zwitterions with Artificial Receptors. Chem. Asian J. 15 (7), 986–994. doi:10.1002/asia.201901789
Ariga, K., Nakanishi, T., Terasaka, Y., Tsuji, H., Sakai, D., and Kikuchi, J. I. (2005). Piezoluminescence at the Air−Water Interface through Dynamic Molecular Recognition Driven by Lateral Pressure Application. Langmuir 21 (3), 976–981. doi:10.1021/la0477845
Barnes, J. C., Dale, E. J., Prokofjevs, A., Narayanan, A., Gibbs-Hall, I. C., Juríček, M., et al. (2015). Semiconducting Single Crystals Comprising Segregated Arrays of Complexes of C60. J. Am. Chem. Soc. 137 (6), 2392–2399. doi:10.1021/ja512959g
Bühner, M., Geuder, W., Gries, W. K., Hünig, S., Koch, M., and Poll, T. (1988). A Novel Type of Cationic Host Molecules Withπ-Acceptor Properties. Angew. Chem. Int. Ed. Engl. 27 (11), 1553–1556. doi:10.1002/anie.198815531
Cai, Y., Yan, X., Wang, S., Zhu, Z., Cen, M., Ou, C., et al. (2021). Pillar[5]arene-Based 3D Hybrid Supramolecular Polymer for Green Catalysis in Water. Inorg. Chem. 60 (5), 2883–2887. doi:10.1021/acs.inorgchem.0c03645
Cao, Y., Hu, X. Y., Li, Y., Zou, X., Xiong, S., Lin, C., et al. (2014). Multistimuli-responsive Supramolecular Vesicles Based on Water-Soluble Pillar[6]arene and SAINT Complexation for Controllable Drug Release. J. Am. Chem. Soc. 136 (30), 10762–10769. doi:10.1021/ja505344t
Celton, M., Sanchez, I., Goelzer, A., Fromion, V., Camarasa, C., and Dequin, S. (2012). A Comparative Transcriptomic, Fluxomic and Metabolomic Analysis of the Response of Saccharomyces cerevisiae to Increases in NADPH Oxidation. BMC Genomics 13, 317. doi:10.1186/1471-2164-13-317
Chen, L., Lin, Y., Zhang, Z., Yang, R., Bai, X., Liu, Z., et al. (2021). A Novel Dual-Prodrug Carried by Cyclodextrin Inclusion Complex for the Targeting Treatment of colon Cancer. J. Nanobiotechnol. 19, 329. doi:10.1186/s12951-021-01064-3
Chen, Q., Sun, J., Li, P., Hod, I., Moghadam, P. Z., Kean, Z. S., et al. (2016). A Redox-Active Bistable Molecular Switch Mounted inside a Metal-Organic Framework. J. Am. Chem. Soc. 138 (43), 14242–14245. doi:10.1021/jacs.6b09880
Cheng, C., McGonigal, P. R., Schneebeli, S. T., Li, H., Vermeulen, N. A., Ke, C., et al. (2015). An Artificial Molecular Pump. Nat. Nanotech 10 (6), 547–553. doi:10.1038/nnano.2015.96
Cheng, L., Zhang, H., Dong, Y., Zhao, Y., Yu, Y., and Cao, L. (2019). Tetraphenylethene-based Tetracationic Cyclophanes and Their Selective Recognition for Amino Acids and Adenosine Derivatives in Water. Chem. Commun. 55 (16), 2372–2375. doi:10.1039/c9cc00599d
Crini, G. (2014). Review: a History of Cyclodextrins. Chem. Rev. 114 (21), 10940–10975. doi:10.1021/cr500081p
Dale, E. J., Vermeulen, N. A., Juríček, M., Barnes, J. C., Young, R. M., Wasielewski, M. R., et al. (2016). Supramolecular Explorations: Exhibiting the Extent of Extended Cationic Cyclophanes. Acc. Chem. Res. 49 (2), 262–273. doi:10.1021/acs.accounts.5b00495
Das, D., and Scherman, O. A. (2011). Cucurbituril: At the Interface of Small Molecule Host-Guest Chemistry and Dynamic Aggregates. Isr. J. Chem. 51 (5-6), 537–550. doi:10.1002/ijch.201100045
Ding, Y., Tong, Z., Jin, L., Ye, B., Zhou, J., Sun, Z., et al. (2021). A NIR Discrete Metallacycle Constructed from Perylene Bisimide and Tetraphenylethylene Fluorophores for Imaging‐Guided Cancer Radiochemotherapy. Adv. Mater., 2106388. doi:10.1002/adma.202106388
Dröge, W. (2002). Free Radicals in the Physiological Control of Cell Function. Physiol. Rev. 82 (1), 47–95. doi:10.1152/physrev.00018.2001
Escobar, L., and Ballester, P. (2021). Molecular Recognition in Water Using Macrocyclic Synthetic Receptors. Chem. Rev. 121 (4), 2445–2514. doi:10.1021/acs.chemrev.0c00522
Fernandez-Marcos, P. J., and Nóbrega-Pereira, S. (2016). NADPH: New Oxygen for the ROS Theory of Aging. Oncotarget 7 (32), 50814–50815. doi:10.18632/oncotarget.10744
Francisco, V., Lino, M., and Ferreira, L. (2019). A Near Infrared Light-Triggerable Modular Formulation for the Delivery of Small Biomolecules. J. Nanobiotechnol. 17, 97. doi:10.1186/s12951-019-0530-y
Gong, H. Y., Rambo, B. M., Karnas, E., Lynch, V. M., Keller, K. M., and Sessler, J. L. (2011). Environmentally Responsive Threading, Dethreading, and Fixation of Anion-Induced Pseudorotaxanes. J. Am. Chem. Soc. 133 (5), 1526–1533. doi:10.1021/ja109102k
Gong, H. Y., Rambo, B. M., Karnas, E., Lynch, V. M., and Sessler, J. L. (2010). A 'Texas-sized' Molecular Box that Forms an Anion-Induced Supramolecular Necklace. Nat. Chem 2 (5), 406–409. doi:10.1038/nchem.597
Guo, H., Yan, X., Lu, B., Wang, J., Yuan, X., Han, Y., et al. (2020). Pillar[5]arene-based Supramolecular Assemblies with Two-step Sequential Fluorescence Enhancement for Mitochondria-Targeted Cell Imaging. J. Mater. Chem. C 8 (44), 15622–15625. doi:10.1039/D0TC04343E
Guo, Q. H., Zhou, J., Mao, H., Qiu, Y., Nguyen, M. T., Feng, Y., et al. (2020). TetrazineBox: A Structurally Transformative Toolbox. J. Am. Chem. Soc. 142 (11), 5419–5428. doi:10.1021/jacs.0c01114
Hariharan, M., Karunakaran, S. C., and Ramaiah, D. (2007). Selective Recognition of Tryptophan through Inhibition of Intramolecular Charge-Transfer Interactions in an Aqueous Medium. Org. Lett. 9 (3), 417–420. doi:10.1021/ol062636m
Hayashida, O., and Kaku, Y. (2013). Synthesis of Dabsyl-Appended Cyclophanes and Their Heterodimer Formation with Pyrene-Appended Cyclophanes. J. Org. Chem. 78 (20), 10437–10442. Epub 2013 Sep 30. doi:10.1021/jo4018843
Ikeda, A., and Shinkai, S. (1997). Novel Cavity Design Using Calix[n]arene Skeletons: Toward Molecular Recognition and Metal Binding. Chem. Rev. 97 (5), 1713–1734. doi:10.1021/cr960385x
Jeon, Y. J., Kim, H., Jon, S., Selvapalam, N., Oh, D. H., Seo, I., et al. (2004). Artificial Ion Channel Formed by Cucurbit[n]uril Derivatives with a Carbonyl Group Fringed Portal Reminiscent of the Selectivity Filter of K+ Channels. J. Am. Chem. Soc. 126 (49), 15944–15945. doi:10.1021/ja044748j
Jiao, T., Cai, K., Nelson, J. N., Jiao, Y., Qiu, Y., Wu, G., et al. (2019). Stabilizing the Naphthalenediimide Radical within a Tetracationic Cyclophane. J. Am. Chem. Soc. 141 (42), 16915–16922. doi:10.1021/jacs.9b08926
Jisha, V. S., Arun, K. T., Hariharan, M., and Ramaiah, D. (2006). Site-selective Binding and Dual Mode Recognition of Serum Albumin by a Squaraine Dye. J. Am. Chem. Soc. 128 (18), 6024–6025. doi:10.1021/ja061301x
Jisha, V. S., Arun, K. T., Hariharan, M., and Ramaiah, D. (2010). Site-Selective Interactions: Squaraine Dye−Serum Albumin Complexes with Enhanced Fluorescence and Triplet Yields. J. Phys. Chem. B. 114 (17), 5912–5919. doi:10.1021/jp100369x
Kuruvilla, E., Nandajan, P. C., Schuster, G. B., and Ramaiah, D. (2008). Acridine−Viologen Dyads: Selective Recognition of Single-Strand DNA through Fluorescence Enhancement. Org. Lett. 10 (19), 4295–4298. doi:10.1021/ol801731k
Kwok, R. T. K., Leung, C. W. T., Lam, J. W. Y., and Tang, B. Z. (2015). Biosensing by Luminogens with Aggregation-Induced Emission Characteristics. Chem. Soc. Rev. 44 (13), 4228–4238. doi:10.1039/c4cs00325j
Lagona, J., Mukhopadhyay, P., Chakrabarti, S., and Isaacs, L. (2005). The Cucurbit[n]uril Family. Angew. Chem. Int. Ed. 44 (31), 4844–4870. doi:10.1002/anie.200460675
Li, M., Liu, G., Wang, K., Wang, L., Fu, X., Lim, L. Y., et al. (2020). Metal Ion-Responsive Nanocarrier Derived from Phosphonated Calix[4]arenes for Delivering Dauricine Specifically to Sites of Brain Injury in a Mouse Model of Intracerebral Hemorrhage. J. Nanobiotechnol. 18, 61. doi:10.1186/s12951-020-00616-3
Li, X., Zhao, X., Pardhi, D., Pardhi, D., Wu, Q., Zheng, Y., et al. (2018). Folic Acid Modified Cell Membrane Capsules Encapsulating Doxorubicin and Indocyanine green for Highly Effective Combinational Therapy In Vivo. Acta Biomater. 74, 374–384. doi:10.1016/j.actbio.2018.05.006
Li, Z. Y., Zhang, Y., Zhang, C. W., Chen, L.-J., Wang, C., Tan, H., et al. (2014). Cross-Linked Supramolecular Polymer Gels Constructed from Discrete Multi-Pillar[5]arene Metallacycles and Their Multiple Stimuli-Responsive Behavior. J. Am. Chem. Soc. 136 (24), 8577–8589. doi:10.1021/ja413047r
Liao, X., Chen, G., Liu, X., Chen, W., Chen, F., and Jiang, M. (2010). Photoresponsive Pseudopolyrotaxane Hydrogels Based on Competition of Host-Guest Interactions. Angew. Chem. Int. Edition 49 (26), 4409–4413. doi:10.1002/anie.201000141
Liu, Z., Nalluri, S. K. M., and Stoddart, J. F. (2017). Surveying Macrocyclic Chemistry: from Flexible crown Ethers to Rigid Cyclophanes. Chem. Soc. Rev. 46 (9), 2459–2478. doi:10.1039/c7cs00185a
Lomoth, R., Häupl, T., Johansson, O., and Hammarström, L. (2002). Redox-Switchable Direction of Photoinduced Electron Transfer in an Ru(bpy)3(2+)-Viologen Dyad. Chem. Eur. J. 8 (1), 102–110. doi:10.1002/1521-3765(20020104)8:1<102:aid-chem102>3.0.co;2-s
Luo, X., Zhao, W., Shi, J., Li, C., Liu, Z., Bo, Z., et al. (2012). Reversible Switching Emissions of Tetraphenylethene Derivatives Among Multiple Colors with Solvent Vapor, Mechanical, and Thermal Stimuli. J. Phys. Chem. C 116 (41), 21967–21972. doi:10.1021/jp306908u
Margis, R., Dunand, C., Teixeira, F. K., and Margis-Pinheiro, M. (2008). Glutathione Peroxidase Family - an Evolutionary Overview. FEBS J. 275 (15), 3959–3970. doi:10.1111/j.1742-4658.2008.06542.x
Mei, J., Leung, N. L. C., Kwok, R. T. K., Lam, J. W. Y., and Tang, B. Z. (2015). Aggregation-Induced Emission: Together We Shine, United We Soar. Chem. Rev. 115 (21), 11718–11940. doi:10.1021/acs.chemrev.5b00263
Meyer, E. A., Castellano, R. K., and Diederich, F. (2003). Interactions with Aromatic Rings in Chemical and Biological Recognition. Angew. Chem. Int. Ed. 42 (11), 1210–1250. doi:10.1002/anie.200390319
Neira, I., Blanco-Gómez, A., Quintela, J. M., García, M. D., and Peinador, C. (2020). Dissecting the "Blue Box": Self-Assembly Strategies for the Construction of Multipurpose Polycationic Cyclophanes. Acc. Chem. Res. 53 (10), 2336–2346. doi:10.1021/acs.accounts.0c00445
Ogoshi, T., Yamagishi, T. A., and Nakamoto, Y. (2016). Pillar-Shaped Macrocyclic Hosts Pillar[n]arenes: New Key Players for Supramolecular Chemistry. Chem. Rev. 116 (14), 7937–8002. doi:10.1021/acs.chemrev.5b00765
Ojida, A., Takashima, I., Kohira, T., Nonaka, H., and Hamachi, I. (2008). Turn-on Fluorescence Sensing of Nucleoside Polyphosphates Using a Xanthene-Based Zn(II) Complex Chemosensor. J. Am. Chem. Soc. 130 (36), 12095–12101. doi:10.1021/ja803262w
Ong, W., Gómez-Kaifer, M., and Kaifer, A. E. (2002). Cucurbit[7]uril: a Very Effective Host for Viologens and Their Cation Radicals. Org. Lett. 4 (10), 1791–1794. doi:10.1021/ol025869w
Pedersen, C. J. (1967). Cyclic Polyethers and Their Complexes with Metal Salts. J. Am. Chem. Soc. 89 (26), 7017–7036. doi:10.1021/ja01002a035
Perret, F., and Coleman, A. W. (2011). Biochemistry of Anionic Calix[n]arenes. Chem. Commun. 47 (26), 7303–7319. doi:10.1039/c1cc11541c
Philip, I. E., and Kaifer, A. E. (2002). Electrochemically Driven Formation of a Molecular Capsule Around the Ferrocenium Ion. J. Am. Chem. Soc. 124 (43), 12678–12679. doi:10.1021/ja028202d
Philp, D., Slawin, A. M. Z., Spencer, N., Stoddart, J. F., and Williams, D. J. (1991). The Complexation of Tetrathiafulvalene by cyclobis(Paraquat-P-Phenylene). J. Chem. Soc. Chem. Commun., 1584. doi:10.1039/C39910001584
Pluth, M. D., and Raymond, K. N. (2007). Reversible Guest Exchange Mechanisms in Supramolecular Host-Guest Assemblies. Chem. Soc. Rev. 36 (2), 161–171. doi:10.1039/b603168b
Prochowicz, D., Kornowicz, A., and Lewiński, J. (2017). Interactions of Native Cyclodextrins with Metal Ions and Inorganic Nanoparticles: Fertile Landscape for Chemistry and Materials Science. Chem. Rev. 117 (22), 13461–13501. doi:10.1021/acs.chemrev.7b00231
Ren, Z., Sun, S., Sun, R., Cui, G., Hong, L., Rao, B., et al. (2020). A Metal-Polyphenol‐Coordinated Nanomedicine for Synergistic Cascade Cancer Chemotherapy and Chemodynamic Therapy. Adv. Mater. 32 (6), 1906024. doi:10.1002/adma.201906024
Sameni, S., Jeunesse, C., Matt, D., and Harrowfield, J. (2009). Calix[4]arene Daisychains. Chem. Soc. Rev. 38 (7), 2117–2146. doi:10.1039/b900183b
Sapotta, M., Hofmann, A., Bialas, D., and Würthner, F. (2019). A Water-Soluble Perylene Bisimide Cyclophane as a Molecular Probe for the Recognition of Aromatic Alkaloids. Angew. Chem. Int. Ed. 58 (11), 3516–3520. doi:10.1002/anie.201813559
Sathiyajith, C., Shaikh, R. R., Han, Q., Zhang, Y., Meguellati, K., and Yang, Y. W. (2017). Biological and Related Applications of Pillar[n]arenes. Chem. Commun. 53 (4), 677–696. doi:10.1039/c6cc08967d
Schulze, A., and Harris, A. L. (2012). How Cancer Metabolism Is Tuned for Proliferation and Vulnerable to Disruption. Nature 491 (7424), 364–373. doi:10.1038/nature11706
Si, W., Li, Z. T., and Hou, J. L. (2014). Voltage-driven Reversible Insertion into and Leaving from a Lipid Bilayer: Tuning Transmembrane Transport of Artificial Channels. Angew. Chem. Int. Ed. 53 (18), 4578–4581. doi:10.1002/anie.201311249
Strutt, N. L., Zhang, H., Schneebeli, S. T., and Stoddart, J. F. (2014). Functionalizing Pillar[n]arenes. Acc. Chem. Res. 47 (8), 2631–2642. doi:10.1021/ar500177d
Sun, J., Liu, Z., Liu, W. G., Wu, Y., Wang, Y., Barnes, J. C., et al. (2017). Mechanical-Bond-Protected, Air-Stable Radicals. J. Am. Chem. Soc. 139 (36), 12704–12709. doi:10.1021/jacs.7b06857
Trabolsi, A., Fahrenbach, A. C., Dey, S. K., Share, A. I., Friedman, D. C., Basu, S., et al. (2010a). A Tristable [2]pseudo[2]rotaxane. Chem. Commun. 46 (6), 871–873. doi:10.1039/b918321c
Trabolsi, A., Khashab, N., Fahrenbach, A. C., Friedman, D. C., Colvin, M. T., Cotí, K. K., et al. (2010b). Radically Enhanced Molecular Recognition. Nat. Chem 2 (1), 42–49. doi:10.1038/nchem.479
Wu, D., Li, Y., Yang, J., Shen, J., Zhou, J., Hu, Q., et al. (2017). Supramolecular Nanomedicine Constructed from Cucurbit[8]uril-Based Amphiphilic Brush Copolymer for Cancer Therapy. ACS Appl. Mater. Inter. 9 (51), 44392–44401. doi:10.1021/acsami.7b16734
Wu, D., Wang, S., Yu, G., and Chen, X. (2021). Cell Death Mediated by the Pyroptosis Pathway with the Aid of Nanotechnology: Prospects for Cancer Therapy. Angew. Chem. Int. Ed. 60 (15), 8018–8034. doi:10.1002/anie.202010281
Wu, H., Chen, Z., Qi, S., Bai, B., Ye, J., Wu, D., et al. (2021). Evaluation of the Stability of Cucurbit[8]uril-Based Ternary Host−guest Complexation in Physiological Environment and the Fabrication of a Supramolecular Theranostic Nanomedicine. J. Nanobiotechnol. 19, 330. doi:10.1186/s12951-021-01076-z
Ying, W. (2008). NAD+/NADH and NADP+/NADPH in Cellular Functions and Cell Death: Regulation and Biological Consequences. Antioxid. Redox Signaling 10 (2), 179–206. doi:10.1089/ars.2007.1672
Zhang, Y., Chen, Q., Wang, Y., Zheng, X., Wang, H., Cao, F., et al. (2020). A Bistable [2]catenane Switched by Hetero-Radical Pairing Interactions. Chem. Commun. 56 (79), 11887–11890. doi:10.1039/d0cc02012e
Zhao, N., Yang, Z., Lam, J. W. Y., Sung, H. H. Y., Xie, N., Chen, S., et al. (2012). Benzothiazolium-functionalized Tetraphenylethene: an AIE Luminogen with Tunable Solid-State Emission. Chem. Commun. 48 (69), 8637–8639. doi:10.1039/c2cc33780k
Zhou, S., Zhao, H., Feng, R., Ding, L., Li, Z., Deng, C., et al. (2018). Application of Amphiphilic Fluorophore-Derived Nanoparticles to Provide Contrast to Human Embryonic Stem Cells without Affecting Their Pluripotency and to Monitor Their Differentiation into Neuron-like Cells. Acta Biomater. 78, 274–284. doi:10.1016/j.actbio.2018.07.051
Keywords: tetracationic cyclophanes, tetraphenylethene, NADPH recognition, host-guest complexation, fluorescent switch
Citation: Wu D, Zhang Z, Yu X, Bai B and Qi S (2021) Hydrophilic Tetraphenylethene-Based Tetracationic Cyclophanes: NADPH Recognition and Cell Imaging With Fluorescent Switch. Front. Chem. 9:817720. doi: 10.3389/fchem.2021.817720
Received: 18 November 2021; Accepted: 02 December 2021;
Published: 22 December 2021.
Edited by:
Yong Yao, Nantong University, ChinaCopyright © 2021 Wu, Zhang, Yu, Bai and Qi. This is an open-access article distributed under the terms of the Creative Commons Attribution License (CC BY). The use, distribution or reproduction in other forums is permitted, provided the original author(s) and the copyright owner(s) are credited and that the original publication in this journal is cited, in accordance with accepted academic practice. No use, distribution or reproduction is permitted which does not comply with these terms.
*Correspondence: Dan Wu, danwu@zjut.edu.cn; Shaolong Qi, 15584187168@163.com