- 1State Key Laboratory of Reliability and Intelligence Electrical Equipment, Hebei University of Technology, Tianjin, China
- 2National Engineering Research Center for Technological Innovation Method and Tool, School of Mechanical Engineering, Hebei University of Technology, Tianjin, China
- 3School of Electronics and Information Engineering, Hebei University of Technology, Tianjin, China
In recent years, gas sensing electronic devices have always attracted wide attention in the field of environment, industry, aviation and others. In order to improve the gas sensing properties, many micro- and nano-fabrication technologies have been proposed and investigated to develop high-performance gas sensing devices. It is worth noting that light irradiation is an effective strategy to enhance gas sensitivity, shorten the response and recovery time, reduce operating temperature. In this review, firstly, the latest research advances of gas sensors based on different micro-nanostructure materials under UV light and visible light activation is introduced. Then, the gas sensing mechanism of light-assisted gas sensor is discussed in detail. Finally, this review describes the present application of gas sensors with improved properties under light activation assisted conditions and the perspective of their applications.
Introduction
With the rapid improvement of economy and the quality of human life, people have realized that environmental pollution has caused irreparable damage to the Earth. Harmful gases from coal-fired power stations, garbage incineration, automobile exhaust and industrial waste gas not only pose a threat to the environment but also endanger human health (Cheng et al., 2021a). Therefore, the development of harmful gas detection technology is of great significance.
The metal oxide semiconductor (MOS) sensors have many advantages of small size, high response, fast response and so on, which attract great interest in real-time detection of different gases. However, the high operating temperature can reduce the life of the device and sensitivity. Consequently, researchers began to explore gas sensing devices by putting forward a large number of theoretical methods and experimental schemes, which can work at low temperature or even room temperature (RT). In recent years, with the continuous development of nanotechnology and nanomaterials, the research of RT gas sensor has also got significant progress include morphological control (Wang et al., 2021, noble metal surface modification (Wang et al., 2019a) or doping and the formation of heterostructures (Wang et al., 2019b). Furthermore, light activation is an effective method to improve the performance of MOS sensors. The optical irradiation of MOS sensor can change the surface electronic properties by adjusting the concentration of optical carriers in MOS, so as to promote the interaction between molecule and sensor layer (Kumar et al., 2020). Herein, we will summarize the latest progress of photoactivated RT MOS sensors in the past few years.
Recently, many reports have confirmed that ultraviolet (UV) irradiation can indeed improve the performance of MOS sensors, including higher sensitivity, shorter recovery time and lower power consumption. UV excitation can increase the density of free electron-hole pairs and lead to photodissociation of the target gas and chemical surface adsorbents (Zhai et al., 2018; Park et al., 2014; Hyodo et al., 2017). However, the harmful effects of UV light on human skin and eyes remain an acute problem, and UV light accounts for only 5–7% of the total energy from sunlight. Therefore, additional UV-LED light sources are required, which presents a new challenge to the size design of the sensor. By contrast, visible light is superior to UV light in terms of energy acquisition and energy utilization. More importantly, it does no harm to human health. Similarly, visible light activation of narrow band gap metal oxides for RT gas sensing has been explored by numerous researchers in the past few years (Hasani et al., 2015; Li et al., 2018; Song et al., 2020). While visible light activation is an effective way to improve the performance of MOS sensors, it still faces great challenges to widely apply it in real environment.
In this paper, we discuss RT gas sensors under light assisted conditions. This review is divided into three parts: firstly, we focus on the latest progress of gas sensors based on MOS, noble metal doped MOS and MOS with heterojunction structure at room temperature by different wavelength light sources (UV light and visible light). Secondly, we describe the gas sensing mechanism under light-assisted condition. Finally, we introduce the application status and future prospects of gas sensors under light-assisted conditions.
Light Activation Gas Sensors Based on Different Micro-Nanostructure Materials
Many studies have shown that light irradiation is one of the effective ways to improve the gas sensitivity of pure MOS, noble metal doping MOS nanostructure and MOS with heterostructure can be improved under the condition of light activation, which will be described one by one below.
Gas Sensors Based on Pure Metal Oxide Nanostructure
In 2008, Costello and his colleagues firstly confirmed that UV light can improve the RT sensitivity of ZnO sensor. The ZnO sensor could detect 1 ppb of acetaldehyde and acetone and obtained adjustable sensitivity though manipulating the intensity of UV light (De Lacy Costello et al., 2008). Chen et al. demonstrated the gas sensor based 3D cone-shaped MoS2 bilayer showed high response (∼470%) and short response time (∼25 s) after exposure to 1 ppm of NO under UV light (Chen et al., 2019). As shown in Figure 1A, it is explained that the excellent NO sensing properties is due to the three-dimensional light scattering effect attracted by UV light, which further enhances the light absorption.
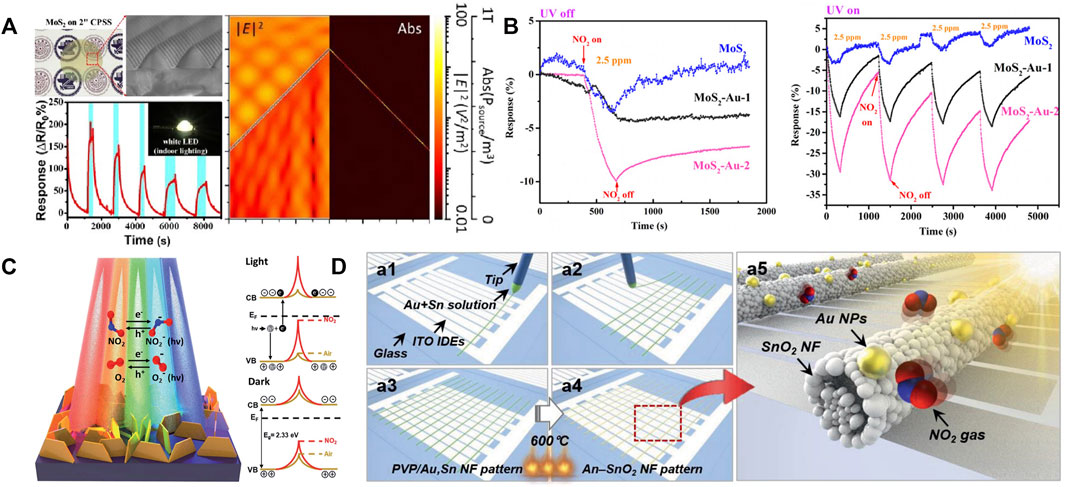
FIGURE 1. (A) Microscopic characterization of MoS2 and gas sensing performance under white light, Simulation results for the Flat-MoS2 and Cone-Shaped-MoS2 gas sensors by finite difference time domain (FDTD) illuminated by the light with the wavelength of 550 nm. Reproduced with permission from ref. 13. Copyright 2019, Royal Society of Chemistry. (B) Schematic illustrations of edge-enriched SnS2 NFs for visible light activated NO2 gas sensor. Reproduced with permission from ref. 14. Copyright 2021, Royal Society of Chemistry. (C) Sensing performance of MoS2 and MoS2-Au sensors toward NO2 gas with no UV illumination. Reproduced with permission from ref. 17. Copyright 2018, American Institute of Physics. (D) Schematic illustration for (left) the experimental procedures to fabricate nanofiber pattern (NF-P) sensors via near-field electrospinning (NFES) and (right) NO2 detection of nanofiber pattern (NF-P) sensors under visible light illumination. Reproduced with permission from ref. 18. Copyright 2021, WILEY-VCH.
Because of the health hazards and low utilization of UV light, researchers began to explore the replacement of UV light with visible light. Li et al. prepared a highly ordered CdS nanoflakes array by using Chemical Vapor Deposition (CVD) technology and studied its gas sensing characteristics (Li et al., 2018). It is found that the sensor has good working potential under natural solar lamps and can be used for outdoor environment monitoring. The excellent gas performances are attributed to the low band gap energy (2.4 eV) and the unique morphology of CdS. These inherent properties of CdS can enhance light absorption and conductivity. Wang et al. successfully prepared SnS2 nanoflowers by solvothermal synthesis (Eom et al., 2021). In Figure 1B, it is confirmed that the high absorbance of SnS2 in the visible region triggered the generation of carriers, which could decrease the resistance and enhance the gas sensing characteristics. Friedman et al. fabricated MoS2 by mechanical stripping and tested the sensitivity of the sensor under visible light (Friedman et al., 2014). They observed a 10-fold difference in the sensitivity of the sensors to trimethylamine before and after light exposure. The improved gas sensitivity of MoS2 sensors comes from photoexcitation.
Gas Sensors Based on Noble Metal Doping Nanostructure
Many studies have demonstrated that the modification of noble metal and light irradiation have synergistic effect on improving the gas sensing performance of pure MOS. On one hand, Li et al. fabricated a sensor based on Au/ZnO porous octahedron (POHs). Compared with the sensor based pure ZnO POH, the response of the sensor based on Au/ZnO POHs to formaldehyde is significantly improved under UV light (Tsai et al., 2018). Zhou et al. reported a UV-assisted, recoverable, highly sensitive and selective NO2 gas sensor based on Au-MoS2 nanocomposite (Zhou et al., 2018a). In Figure 1C, the Au-MoS2 sensor has a three-fold enhanced response to NO2, full recovery property and good repeatability under UV light. The detection of NO2 under UV light provides an alternative strategy for the design of single multifunctional optoelectronic devices.
On the other hand, Kim et al. reported the detection of NO2 gas by a one-dimensional Au-SnO2 nanofiber sensor under visible light at RT (Lim et al., 2021). The sensor shows a high degree of selectivity, sensitivity and repeatability in response to NO2 at sub ppm levels. As shown in Figure 1D, the excellent RT NO2 properties are in connection with the effects of Au nanoparticles from local surface plasmon resonance (LSPR) in visible light. This work makes the new transparent design possible for oxide gas sensors without external heaters or light sources. Additionally, Chen et al. developed a high-performance visible light activated NO2 gas sensor based on LSPR and increased surface oxygen vacancy (Chen et al., 2020). The results show that Au NPs modification can significantly improve the visible photosensitivity of ZnO films compared with pure ZnO films. It provides an effective way to construct high-performance photoactivated gas sensor.
Gas Sensors Based on Heterojunction Nanostructure Composite
Both the construction of heterostructure and light irradiation can increase active sites and charge transfer of pure metal oxide semiconductor. The heterostructure of MOS can enhance charge transduction and adjust grain boundary potential barrier, which is beneficial to improve the gas sensitivity. For one thing, Chang et al. prepared hollow ZnO/MoS2 nanosheets with core/shell heterostructure (Chang et al., 2020). The high acetone response and fast response/recovery rate are caused by the fast gas transport channel and the n-p heterojunction of MoS2 nanosheets. Moreover, UV light is introduced to further improve the acetone reaction and greatly reduce the operating temperature. The optical diffraction and reflection caused by the decoration of layered MoS2 nanosheets can significantly improve the light capture. Yang et al. successfully synthesized hollow ZnO-SnO2 heterostructure for triethylamine (TEA) (Yang et al., 2018). Particularly, the sensor based ZnO-SnO2 showed short response time (1.8 s) and recovery time (18 s) under UV light.
For another, Liu et al. reported hollow SnO2@SnS2 nanostructures prepared by one-step hydrothermal method (Liu et al., 2020). The sensor based on SnO2@SnS2 has a fast response rate and good recovery ability to ppb-level NO2 under visible light at RT. The excellent sensing performance of SnO2@SnS2 sensor is due to the hollow and porous heterojunction structure, which is conducive to gas diffusion, especially visible light assisted to promote charge transfer and gas desorption. Chen et al. prepared gas-sensitive ZnO nanorods with sea urchin-level mesoporous structure modified by PbS quantum dots (QDs) (Chen et al., 2018). The PbS QDs have a narrow band gap shown in the Near Infrared Spectroscopy Analysis (NIR) spectrum. Compared with the original ZnO nanorods, the sensor based ZnO/PbS nanocomposites have a higher response and faster response/recovery speed to ppm level NO2 under visible light.
Sensing Mechanism of Light-Assisted Gas Sensor
Light activation has been proved to be an effective way to improve the performance of gas sensors, but the relationship between the types of light source, the structure of materials and the improvement of gas sensing properties are not clear. The explanations for the improved gas sensitivity of UV light are as follows:
Firstly, Gong et al. proposed the gas sensor based on a novel ZnO hybrid with nanowire/optical fiber (Gong et al., 2017). The results show that UV irradiation can improve the sensitivity and shorten the response time, and the sensor also has good long-term stability. UV irradiation can respond to gas with ppb-level concentration at low temperature. The electrons in the ZnO hybrid excited by UV irradiation promote the reduction of ethanol gas, leading to the higher gas performance. Therefore, the irradiation of UV will generate more conductive electrons, improve the conductivity of the sensing materials and promote the electron transfer under UV irradiation, thus improving the response of the gas sensor. Secondly, Zhou et al. have proposed that the increased sensitivity is ascribed to the increase in the number of active adsorption sites and the introduction of active catalysts such as Au, Ag, Pd and other noble metals (Zhou et al., 2018a). For example, Au nanoparticles can accelerate the capture of more photons, resulting in additional photoexcited carriers to promote the interaction between gas and sensing materials. The efficient separation of photoexcited carriers at the interface of MoS2/Au also contributes to rapid and complete recovery under UV light. Finally, Zhou et al. explained that UV light can excite electron-hole pairs in ZnO (n-type) and MoS2 (p-type) (Zhou et al., 2018b). The photoexcited electrons in the conduction band can be easily transferred to ZnO by the built-in field, which is similar to the excited hole in the valence band of ZnO to the excited hole of MoS2. Therefore, light carriers can be effectively separated at the interface of heterojunction in order to improve the gas sensing properties.
The explanations for the improved gas sensitivity of visible light are as follows: Primarily, the explanations given by Li et al. are as follows: The photon energy of visible light greater than the band gap of semiconductor can produce electron-hole pairs. Electrons and holes cannot recombine efficiently, and the electrons of light source move to the conduction band and then are accumulated in the conduction band. This results in the high concentration of electrons in the conduction band and the high conductivity of the semiconductor. Therefore, the sensitivity of the gas sensor is significantly increased under visible light (Li et al., 2018). Posteriorly, Chen et al. developed a high-performance visible light activated NO2 sensor based on LSPR and increased surface oxygen vacancy (Chen et al., 2020). The mechanism of visible light activated by LSPR absorption are illustrated. At the interface of metal and semiconductor, the Fermi level of ZnO is transferred to a positive charge when electrons transfer from ZnO nanorods to Au nanorods, resulting in an upward-bending band interface and a Schottky barrier. The photoexcited electrons generated in Au can overcome the blocking and injection of conduction band in ZnO, making more free electrons can participate in the chemical reaction with the surface adsorbed O2 and NO2 molecules. Niu et al. prepared vertically stacked MoS2/GaSe heterojunction by all-dry transfer method (Niu et al., 2021). When the p-n junction between MoS2 and GaSe is exposed to visible light, electron-hole pairs in both MoS2 and GaSe layers are generated by light and tend to migrate at the interface. Due to the built-in electric field, electrons transfer to n-type MoS2 and holes transfer to p-type GaSe, resulting in carrier separation. NO2 has a strong electron affinity and can easily capture electrons from the conduction band of the material. In general, the separation of the light source carrier and the built-in electric field acts as a driving force to drive the gas sensing behavior.
The possible mechanism and reasons for the superior gas performance of light irradiation can be explained as follows:
1) For pure metal oxide nanostructure, the gas sensing performance depends on the amount of oxygen active substance adsorbed on the nanostructure surface. Under dark conditions, the concentration of free carriers inherent in the nanostructure is low, resulting in less utilization of the active site to produce absorbed oxygen. In contrast, with the increase of the number of free carriers under light irradiation, the surface absorption site improves the chemical activity of the surface and thus enhances the gas sensitivity.
2) For noble metal doping nanostructure, the LSPR effect not only enhances the absorption of light, but also inhibits the reorganization of electron-hole pairs produced by light. The intermediate-thermal electrons generated by LSPR absorption can overcome the Schottky barrier at the noble metal/oxide junction and inject into the oxide conduction band. Therefore, more surface adsorbed oxygen is formed on the oxide surface, leading to a stronger sensing reaction.
3) For heterojunction nanostructure composite, the formation of heterojunction can promote the separation of electron-hole pairs, effectively accelerate the electron conversion between different particles, and improve the response rate. When the heterojunction is exposed to light irradiation, electron-hole pairs in the semiconductor are generated by light and tend to migrate at the interface. Due to the built-in electric field, the electron transfer rate is enhanced, resulting in carrier separation and improving the gas sensitivity.
The Present Application of Gas Sensors Under Light Activation
As essential for environmental monitoring, process control and early diagnosis of human disease, gas sensors under light activation are used to sense volatile organic compounds (VOCs). Wu et al. demonstrated a specific and highly sensitive detection of ketones using two-dimensional (2D) MoTe2 (Wu et al., 2018). They also investigated the effect of UV activation on the sensing performance of VOCs. Activation of UV light results in high sensitivity, low detection limit (∼0.2 ppm) to acetone and high stability even in high humidity, which is crucial for early diagnosis of diabetes. Zhao et al. demonstrated a flexible, transparent and high-performance gas sensor based In2Se3 using a simple pulsed laser deposition (PLD) method (Zheng et al., 2017). The gas sensor can work under the activation of visible light and shows excellent performance in detecting acetylene gas. No significant degradation of sensing performance was observed even after 100 bending cycles. The In2Se3 sensor has an average transmittance of 64% in the visible light range (400–800 nm). In addition, they found that the sensitivity, response and recovery rate of the sensor based In2Se3 depend on the light intensity. The excellent performance of In2Se3 sensors on flexible substrates provides a brilliant future for practical applications in wearable optoelectronic systems. Cheng et al. also prepared CuO flower-like materials (FMs) by hydrothermal method, and then obtained Au@CuO FMs by in-situ reduction reaction (Cheng et al., 2021b). The performance of the sensor based Au@CuO FMs was evaluated by detecting volatile and toxic gases such as ethanol, isopropanol, methanol and formaldehyde under UV light. It was found that the sensor based Au@CuO FMs had a higher response (95.3) than the sensor based CuO FMs at a concentration of 1,000 ppm, while the sensor based Au@CuO FMs had a response of 174 under UV light. Therefore, Au@CuO FMs is a promising ethanol detection method. In summary, light irradiation as an effective means enables gas sensors to maintain better performance in environmental monitoring and human health protection.
Conclusion
In this paper, we first introduce the latest research progress of gas sensors with different microstructures (pure metal oxide nanostructure, noble metal doping nanostructure, heterojunction composite nanostructure) activated by UV and visible light. In the next place, we introduce the light activation mechanism of gas sensors with different structures from the perspective of microscopic mechanism. Finally, several applications of photoactivated gas sensors in environmental monitoring, process control and early diagnosis of human diseases are listed.
Author Contributions
All authors listed have made a substantial, direct and intellectual contribution to the work, and approved it for publication.
Funding
This research work was financially supported by Natural Science Foundation of Hebei Province (F2020202050) and the National Natural Science Foundation of China (Grant No. 52104189).
Conflict of Interest
The authors declare that the research was conducted in the absence of any commercial or financial relationships that could be construed as a potential conflict of interest.
Publisher’s Note
All claims expressed in this article are solely those of the authors and do not necessarily represent those of their affiliated organizations, or those of the publisher, the editors and the reviewers. Any product that may be evaluated in this article, or claim that may be made by its manufacturer, is not guaranteed or endorsed by the publisher.
References
Chang, X., Qiao, X., Li, K., Wang, P., Xiong, Y., Li, X., et al. (2020). UV Assisted Ppb-Level Acetone Detection Based on Hollow ZnO/MoS2 Nanosheets Core/shell Heterostructures at Low Temperature. Sensors Actuators B: Chem. 317, 128208. doi:10.1016/j.snb.2020.128208
Chen, C., Zhang, Q., Xie, G., Yao, M., Pan, H., Du, H., et al. (2020). Enhancing Visible Light-Activated NO2 Sensing Properties of Au NPs Decorated ZnO Nanorods by Localized Surface Plasmon Resonance and Oxygen Vacancies. Mater. Res. Express 7, 015924. doi:10.1088/2053-1591/ab6b64
Chen, R., Wang, J., Xia, Y., and Xiang, L. (2018). Near Infrared Light Enhanced Room-Temperature NO2 Gas Sensing by Hierarchical ZnO Nanorods Functionalized with PbS Quantum Dots. Sensors Actuators B: Chem. 255, 2538–2545. doi:10.1016/j.snb.2017.09.059
Chen, Y.-Z., Wang, S.-W., Yang, C.-C., Chung, C.-H., Wang, Y.-C., Huang Chen, S.-W., et al. (2019). An Indoor Light-Activated 3D Cone-Shaped MoS2 Bilayer-Based NO Gas Sensor with PPb-Level Detection at Room-Temperature. Nanoscale 11, 10410–10419. doi:10.1039/c8nr10157d
Cheng, M., Li, W., Li, C., Wang, Q., Tan, Q., Yang, W., et al. (2021a). Photochemical Sensitive Study of Au@CuO Flower-like Materials. Sensors Actuators B: Chem. 348, 130644. doi:10.1016/j.snb.2021.130644
Cheng, Y., Ren, B., Xu, K., Jeerapan, I., Chen, H., Li, Z., et al. (2021b). Recent Progress in Intrinsic and Stimulated Room-Temperature Gas Sensors Enabled by Low-Dimensional Materials. J. Mater. Chem. C 9, 3026–3051. doi:10.1039/d0tc04196c
De Lacy Costello, B. P. J., Ewen, R. J., Ratcliffe, N. M., and Richards, M. (2008). Highly Sensitive Room Temperature Sensors Based on the UV-LED Activation of Zinc Oxide Nanoparticles. Sensors Actuators B: Chem. 134, 945–952. doi:10.1016/j.snb.2008.06.055
Eom, T. H., Cho, S. H., Suh, J. M., Kim, T., Lee, T. H., Jun, S. E., et al. (2021). Substantially Improved Room Temperature NO2 Sensing in 2-dimensional SnS2 Nanoflowers Enabled by Visible Light Illumination. J. Mater. Chem. A. 9, 11168–11178. doi:10.1039/d1ta00953b
Friedman, A. L., Keith Perkins, F., Cobas, E., Jernigan, G. G., Campbell, P. M., Hanbicki, A. T., et al. (2014). Chemical Vapor Sensing of Two-Dimensional MoS2 Field Effect Transistor Devices. Solid-State Electro. 101, 2–7. doi:10.1016/j.sse.2014.06.013
Gong, B., Shi, T., Zhu, W., Liao, G., Li, X., Huang, J., et al. (2017). UV Irradiation-Assisted Ethanol Detection Operated by the Gas Sensor Based on ZnONanowires/optical Fiber Hybrid Structure. Sensors Actuators B: Chem 245, 821–827. doi:10.1016/j.snb.2017.01.187
Hasani, A., Sharifi Dehsari, H., Amiri Zarandi, A., Salehi, A., Taromi, F. A., and Kazeroni, H. (2015). Visible Light-Assisted Photoreduction of Graphene Oxide Using CdS Nanoparticles and Gas Sensing Properties. J. Nanomater. 2015, 1–11. doi:10.1155/2015/930306
Hyodo, T., Urata, K., Kamada, K., Ueda, T., and Shimizu, Y. (2017). Semiconductor-type SnO2-Based NO2 Sensors Operated at Room Temperature under UV-Light Irradiation. Sensors Actuators B: Chem. 253, 630–640. doi:10.1016/j.snb.2017.06.155
Kumar, R., Liu, X., Zhang, J., and Kumar, M. (2020). Room-temperature Gas Sensors under Photoactivation: from Metal Oxides to 2D Materials. Nano-micro Lett. 12, 164. doi:10.1007/s40820-020-00503-4
Li, H.-Y., Yoon, J.-W., Lee, C.-S., Lim, K., Yoon, J.-W., and Lee, J.-H. (2018). Visible Light Assisted NO2 Sensing at Room Temperature by CdS Nanoflake Array. Sensors Actuators B: Chem. 255, 2963–2970. doi:10.1016/j.snb.2017.09.118
Lim, K., Jo, Y. M., Yoon, J. W., Kim, J. S., Lee, D. J., Moon, Y. K., et al. (2021). A Transparent Nanopatterned Chemiresistor: Visible‐Light Plasmonic Sensor for Trace‐Level NO 2 Detection at Room Temperature. Small 17, 2100438. doi:10.1002/smll.202100438
Liu, D., Tang, Z., and Zhang, Z. (2020). Visible Light Assisted Room-Temperature NO2 Gas Sensor Based on Hollow SnO2@SnS2 Nanostructures. Sensors Actuators B: Chem. 324, 128754. doi:10.1016/j.snb.2020.128754
Niu, Y., Zeng, J., Liu, X., Li, J., Wang, Q., Li, H., et al. (2021). A Photovoltaic Self‐Powered Gas Sensor Based on All‐Dry Transferred MoS 2 /GaSe Heterojunction for ppb‐Level NO 2 Sensing at Room Temperature. Adv. Sci. 8, 2100472. doi:10.1002/advs.202100472
Park, S., Hong, T., Jung, J., and Lee, C. (2014). Room Temperature Hydrogen Sensing of Multiple Networked ZnO/WO3 Core-Shell Nanowire Sensors under UV Illumination. Curr. Appl. Phys. 14, 1171–1175. doi:10.1016/j.cap.2014.06.019
Song, Y., Zhang, Y., Ma, M., Ren, J., Liu, C., and Tan, J. (2020). Visible Light-Assisted Formaldehyde Sensor Based on HoFeO3 Nanoparticles with Sub-ppm Detection Limit. Ceramics Int. 46, 16337–16344. doi:10.1016/j.ceramint.2020.03.191
Tsai, Y.-T., Chang, S.-J., Ji, L.-W., Hsiao, Y.-J., Tang, I.-T., Lu, H.-Y., et al. (2018). High Sensitivity of NO Gas Sensors Based on Novel Ag-Doped ZnO Nanoflowers Enhanced with a UV Light-Emitting Diode. ACS Omega 3, 13798–13807. doi:10.1021/acsomega.8b01882
Wang, Z., Gao, S., Fei, T., Liu, S., and Zhang, T. (2019b). Construction of ZnO/SnO2 Heterostructure on Reduced Graphene Oxide for Enhanced Nitrogen Dioxide Sensitive Performances at Room Temperature. ACS Sens. 4, 2048–2057. doi:10.1021/acssensors.9b00648
Wang, Z., Sackmann, A., Gao, S., Weimar, U., Lu, G., Liu, S., et al. (2019a). Study on Highly Selective Sensing Behavior of Ppb-Level Oxidizing Gas Sensors Based on Zn2SnO4 Nanoparticles Immobilized on Reduced Graphene Oxide under Humidity Conditions. Sensors Actuators B: Chem. 285, 590–600. doi:10.1016/j.snb.2019.01.109
Wang, Z., Sun, J., Huo, Y., Yan, Y., Ma, Z., Bu, M., et al. (2021). Porous Co3O4 Nanocrystals Derived by Metal-Organic Frameworks on Reduced Graphene Oxide for Efficient Room-Temperature NO2 Sensing Properties. J. Alloys Comp. 856, 158199. doi:10.1016/j.jallcom.2020.158199
Wu, E., Xie, Y., Yuan, B., Hao, D., An, C., Zhang, H., et al. (2018). Specific and Highly Sensitive Detection of Ketone Compounds Based on P-type MoTe2 under Ultraviolet Illumination. ACS Appl. Mater. Inter. 10, 35664–35669. doi:10.1021/acsami.8b14142
Yang, T., Gu, K., Zhu, M., Lu, Q., Zhai, C., Zhao, Q., et al. (2019). ZnO-SnO2 Heterojunction Nanobelts: Synthesis and Ultraviolet Light Irradiation to Improve the Triethylamine Sensing Properties. Sensors Actuators B: Chem. 279, 410–417. doi:10.1016/j.snb.2018.10.031
Zhai, J., Wang, T., Wang, C., and Liu, D. (2018). UV-light-assisted Ethanol Sensing Characteristics of G-C3N4/ZnO Composites at Room Temperature. Appl. Surf. Sci. 441026, 317–323. doi:10.1016/j.apsusc.2018.02.026
Zheng, Z., Yao, J., Wang, B., and Yang, G. (2017). A Flexible, Transparent and High-Performance Gas Sensor Based on Layer-Materials for Wearable Technology. Nanotechnology 28, 415501. doi:10.1088/1361-6528/aa8317
Zhou, Y., Gao, C., and Guo, Y. (2018b). UV Assisted Ultrasensitive Trace NO2gas Sensing Based on Few-Layer MoS2nanosheet-ZnO Nanowire Heterojunctions at Room Temperature. J. Mater. Chem. A. 6, 10286–10296. doi:10.1039/C8TA02679C
Keywords: light irradiation, micro-nanostructure, gas sensing devices, sensor, metal oxide semiconductor
Citation: Ma Z, Wang Z and Gao L (2021) Light-Assisted Enhancement of Gas Sensing Property for Micro-Nanostructure Electronic Device: A Mini Review. Front. Chem. 9:811074. doi: 10.3389/fchem.2021.811074
Received: 08 November 2021; Accepted: 24 November 2021;
Published: 24 December 2021.
Edited by:
Yue-Feng Liu, Jilin University, ChinaReviewed by:
Jianxun Dai, Dalian University of Technology, ChinaJing Cao, Tianjin Polytechnic University, China
Copyright © 2021 Ma, Wang and Gao. This is an open-access article distributed under the terms of the Creative Commons Attribution License (CC BY). The use, distribution or reproduction in other forums is permitted, provided the original author(s) and the copyright owner(s) are credited and that the original publication in this journal is cited, in accordance with accepted academic practice. No use, distribution or reproduction is permitted which does not comply with these terms.
*Correspondence: Ziying Wang, d2FuZ3p5QGhlYnV0LmVkdS5jbg==; Lingxiao Gao, bGluZ3hpYW8uZ2FvQGhlYnV0LmNvbQ==