- 1School of Chemistry and Chemical Engineering, Yancheng Institute of Technology, Yancheng, China
- 2Key Laboratory for Advanced Technology in Environmental Protection of Jiangsu Province, Yancheng Institute of Technology, Yancheng, China
- 3School of Mechanical Engineering, Yancheng Institute of Technology, Yancheng, China
Co3O4 is an environmental catalyst that can effectively decompose ozone, but is strongly affected by water vapor. In this study, Co3O4@SiO2 catalysts with a core-shell-like structure were synthesized following the hydrothermal method. At 60% relative humidity and a space velocity of 720,000 h−1, the prepared Co3O4@SiO2 obtained 95% ozone decomposition for 40 ppm ozone after 6 h, which far outperformed that of the 25wt% Co3O4/SiO2 catalysts. The superiority of Co3O4@SiO2 is ascribed to its core@shell structure, in which Co3O4 is wrapped inside the SiO2 shell structure to avoid air exposure. This research provides important guidance for the high humidity resistance of catalysts for ozone decomposition.
Introduction
Ozone is widely used in food, medicine, and waste treatment owing to its excellent oxidizing ability (Alameddine et al., 2021; Kim et al., 2022). However, even low concentrations of ozone are harmful to human health, especially to the eyes, nose, and throat (Ferrara et al., 2020; Ferrara et al., 2021). The maximum eight-hour average concentration of ozone allowed by the World Health Organization is 100 μg/m3. Ozone concentrations in the atmosphere near ground level have considerably increased in recent years due to increased levels of volatile organic compounds and nitrogen oxides (Ou et al., 2016). Ozone in the outdoor air can infiltrate into indoor environments. Indoor ozone is considered more harmful than outdoor ozone because modern humans spend most of their time indoors (Abbass et al., 2017; Namdari et al., 2021; Nazaroff and Weschler 2021). The development of environmental technologies to effectively eliminate ozone is therefore necessary.
There are four common treatment methods to eliminate ozone: activated carbon (Yu et al., 2020); absorption (Yang et al., 2017); thermal decomposition and catalytic decomposition (Gopi et al., 2017; Ma et al., 2017; Gong et al., 2018). Catalytic decomposition is considered to be one of the most feasible and effective methods for ozone removal (Li et al., 2020). Noble metals and transitional metal oxides are common catalysts for heterogeneous reactions including decomposition of ozone (Nikolov et al., 2010; Gong et al., 2017; Deng et al., 2019; Tao et al., 2021a; Tao et al., 2021b). Among the transition metal oxides, CoxOy catalysts with higher oxidation states have exhibited higher ozone decomposition performance than other cobalt oxide catalysts (Tang et al., 2014a). Abdedayem (Abdedayem et al., 2017) demonstrated that the ozone decomposition abilities of Co3O4 support on loaded olivine is proportional to its dispersion degree. However, numerous metal oxide catalysts suffer from interactions with water vapor, and including cobalt oxides (Zhu et al., 2021). It is generally believed that water vapor affects ozone decomposition via competitive adsorption with the transition metal oxides on the active sites (Jia et al., 2016).
In this study, core@shell structure catalysts were synthesized with mesoporous silica as the shell and Co3O4 nanoparticles as the core (Co3O4@SiO2) following the solvothermal route using polyvinylpyrrolidone (PVP) as the capping agent. For comparison, spherical silica supported different additions of Co3O4 and labeled xCo3O4/SiO2, where x = 10, 15, and 20, or 25%. The ozone decomposition performance of xCo3O4/SiO2 increased with increasing Co3O4. The 25Co3O4/SiO2 and Co3O4@SiO2 catalysts yielded high ozone decomposition activity at 20% relative humidity. The ozone elimination activity of 20Co3O4/SiO2 sharply decreased upon increasing the relative humidity to 60%, and the Co3O4@SiO2 catalyst exhibited a better moisture resistance performance for ozone decomposition. This study provides important insights for the further development of coated catalysts for gaseous ozone decomposition.
Experimental Methods
Catalyst Preparation
Co3O4@SiO2 was synthesized in accordance with previously published studies (Khan et al., 2015). First, 0.70 g PVP and 0.35 g Co(NO3)2·6H2O were dissolved in 40 ml ethanol. The solutions were transferred to stainless steel lined with Poly tetra fluoroethylene (PTFE) in an autoclave and heated at 453 K for 4 h. The obtained black powder was dispersed in 103.8 ml ethanol, to which 82.8 ml distilled water, 7.2 ml 25% aqueous ammonia solution, 0.3 g cetyltrimethylammonium bromide, and 1.0 ml tetraethoxysilane were added. The solution was stirred for 48 h at room temperature. The product was collected via filtration, washed three times with distilled water, dried at 333 K, and then calcined at 773 K for 6 h. The finished samples were denoted as Co3O4@SiO2 (wt% = 30%). SiO2 was impregnated with 10, 15, 20, or 25% cobalt loading in an ethanol solution of cobalt nitrate, and the resulting product was calcined at 773 K for 6 h. The prepared samples were labeled as 10Co3O4/SiO2, 15Co3O4/SiO2, 20Co3O4/SiO2, and 25Co3O4/SiO2, respectively.
Catalyst Characterization
The samples were characterized by X-ray diffraction (XRD) using a D/max-RB diffractometer. X-ray photoelectron spectroscopy (XPS) was performed using a Thermo Fisher ESCALAB 250Xi. Morphological and microstructural characterizations were carried out using a Hitachi EM-3010 transmission electron microscope (TEM). The surface areas were calculated by the Brunauer-Emmett-Teller (BET) method. The pore diameters were estimated from the desorption branchers of the isotherms based on the Barrett-Joyner-Halenda (BJH) model.
Catalyst Test
The ozone decomposition activity of the prepared catalysts was evaluated using a flow-through quartz tube reactor (inner diameter = 10 mm) with 0.10 g of catalyst separated by quartz sand at different temperatures and relative humidity (20, 40, and 60%) under atmospheric pressure conditions. Ozone was generated by flowing 20% O2/N2 compressed gas through an ozone generator. The relative humidity of the gas stream was measured using a humidity probe (Benetech, GM1361+). The total gas flow rate passing through the quartz reactor was controlled at 1,500 ml/min and contained 40 ppm O3. The ozone concentrations at the inlet and outlet were detected using a 106-L ozone online analyzer (2B Technologies, Boulder, Co, United States). The ozone conversion was calculated according to:
where
Results and Discussion
Catalyst Characteristics
The morphology and nanostructure of the catalysts were observed by TEM. Figure 1A–C show that the Co3O4@SiO2 nanoparticles were relatively dispersible with an average size of 40 nm. This indicates that PVP can prevent Co3O4 nanoparticle agglomeration under hydrothermal conditions. Figure 1D–F show that the spherical Co3O4/SiO2 composites prepared via incipient wetness impregnation were highly dispersed with a relatively smooth external surface. This indicates that a majority of the Co3O4 nanoparticles were incorporated into the mesopores (Xie et al., 2011). With regard to the spent Co3O4/SiO2 catalyst, the large aggregates were clearly located on the external surface of the spherical SiO2 support, and indicating that small Co3O4 nanoparticles outside of the mesopores easily agglomerated into large Co3O4 aggregates during the reaction.
X-ray photoelectron spectroscopy (XPS) tests were performed to detect the chemical state and composition of the element catalyst surface. According to the previously reported Co3O4 spectrum (Gao et al., 2021), the Co 2p spectrum of Co3O4 (Figure 2A) consists of two peaks, Co 2p3/2 and Co 2p1/2, located at 779.9 and 794.8 eV, respectively. However, the Co 2p3/2 and Co 2p1/2 peaks in the Co3O4@SiO2 and Co3O4/SiO2 catalysts shifted to approximately 781.0 and 796.0 eV, respectively, both of which occur at higher energies than those of pure Co3O4. This shift is mainly due to the interaction between the silica and Co3O4 species, which results in a charge transfer from the Co3O4 to the SiO2 support and has a positive impact on the cobalt catalytic performance.
The atomic surface contents of cobalt were 0.8 and 7.6% for the Co3O4@SiO2 and Co3O4/SiO2 catalysts, respectively. This significant difference further confirms that the preparation of Co3O4@SiO2 successfully encapsulated Co3O4 into the SiO2 matrix. The O 1 s spectra of the catalysts are shown in Figure 2B. The main O 1 s peak centered at 533.0 eV represents the lattice oxygen of Co3O4 and SiO2, but is difficult to be accurately distinguished. The oxygen in the unreducible silica has no notable effect on the catalysis of ozone.
Figure 3 shows the nitrogen isothermal adsorption-desorption curves and pore size distributions of the Co3O4@SiO2 and 25Co3O4/SiO2 catalysts. The nitrogen adsorption-desorption isotherms clearly show that both samples have typical hysteresis loops and are classified as type-IV isotherms, thus indicating that the samples have a mesoporous structure. The average pore diameter of the two samples ranges between 6 and 9 nm. The pore volume of Co3O4@SiO2 (0.15 cm3/g) is larger than that of Co3O4/SiO2 (0.11 cm3/g). The specific surface area of Co3O4/SiO2 is 94.8 m2/g, which is 1.5 times greater than that of Co3O4@SiO2 (68.8 m2/g). The specific surface area of a catalyst is generally believed to have a substantial impact on the catalytic activity, in which catalysts with larger specific surface areas usually have higher catalytic activities. Effect of Co3O4@SiO2 and Co3O4/SiO2 on ozone decomposition.
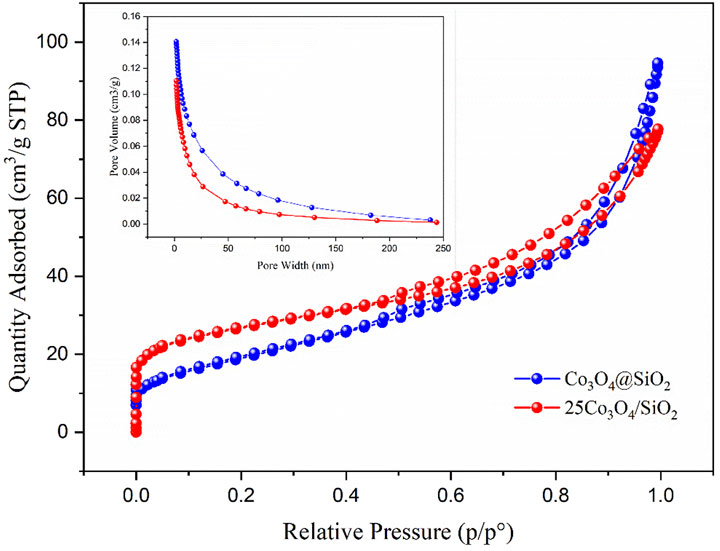
FIGURE 3. Nitrogen adsorption-desorption isotherms and BJH pore-size distribution curves of Co3O4@SiO2 and 25Co3O4/SiO2.
The ozone decomposition rates of Co3O4@SiO2 and Co3O4/SiO2 with different Co3O4 loadings were evaluated in a gas flow with 40 ppm ozone at 20% relative humidity. The activity of the 10Co3O4/SiO2 catalyst dropped sharply within 1 h, and the 15Co3O4/SiO2 and 20Co3O4/SiO2 catalysts dropped to 94% ozone conversion after 4 h. The time to achieve 100% ozone removal rate increased to 9 h for a Co3O4 load of 25%. However, the Co3O4@SiO2 catalyst with 30
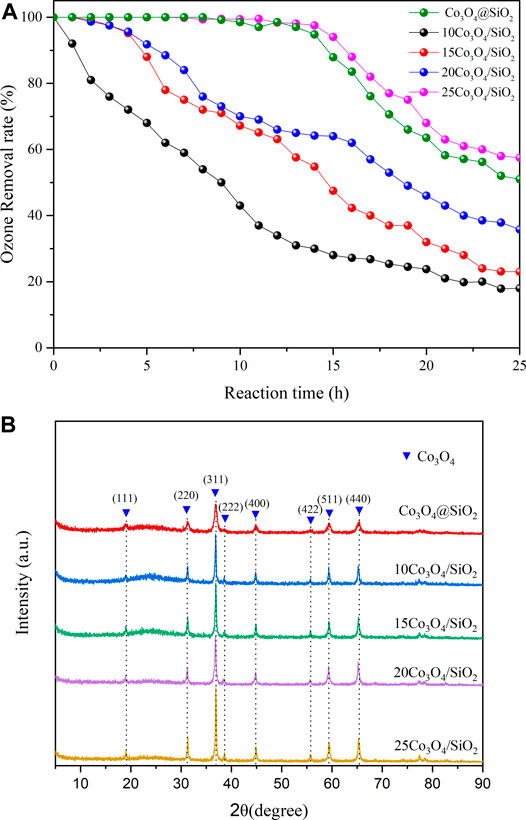
FIGURE 4. (A) Ozone removal rates and (B) XRD patterns of Co3O4@SiO2 and xCo3O4 (x = 10, 15, 20, and 25). Temperature = 25°C; relative humidity = 20%.
Figure 5A,B show the ozone removal rates of 25Co3O4@SiO2 and Co3O4/SiO2, respectively, at relative humidity conditions of 20, 40, and 60%. The 25Co3O4@SiO2 and Co3O4/SiO2 catalysts exhibit similar ozone removal rates at 20% relative humidity. The 25Co3O4/SiO2 catalyst shows 99% ozone conversion for 11 h at 20% relative humidity. The removal rate then sharply drops and ultimately stabilizes at 60%. It is noted that the ozone removal rate sharply decreases with increasing relative humidity, especially when the relative humidity is increased from 40 to 60%. For Co3O4@SiO2, the ozone removal rate begins to decrease during the first 12 h of the reaction runs with a gas flow of 40 ppm ozone, and then decreases to 60% when the reaction has been maintained for 24 h. The ozone removal rate of Co3O4@SiO2 shows a different trend from that of 25Co3O4/SiO2 at 40% relative humidity. When the relative humidity is increased to 60%, the ozone removal rate sharply decreases and remains at 30%, which is approximately 10% higher than that of 25Co3O4/SiO2. This indicates that the main reason for the different performance of the two catalysts is their differing structures. The Co3O4 loaded on the surface of SiO2 is directly exposed to the reaction environment. The accumulation of oxygen atoms and adsorption of water vapor thus lead to catalyst deactivation. In contrast, in the Co3O4@SiO2 catalyst, the Co3O4 is wrapped by SiO2, and which isolates water vapor and prevents it from directly contacting with the Co3O4. The deactivation can thus be attributed to the accumulation of oxygen atoms.
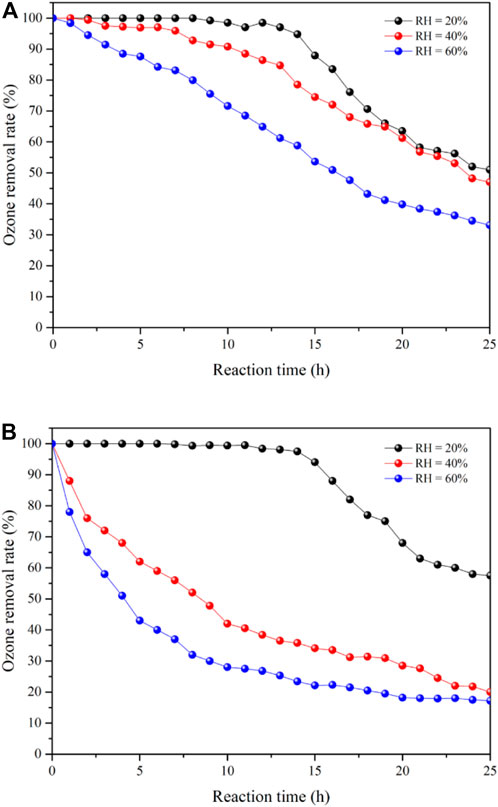
FIGURE 5. Ozone removal rate of 25Co3O4@SiO2 (A) and Co3O4/SiO2 (B). Temperature = 25°C; relative humidity (RH) = 20, 40, and 60%.
Proposed Mechanism
According to the experimental results, we proposed a possible mechanism involving oxygen vacancies (Ov) as depicted below. Initially, the ozone molecule is adsorbed on the oxygen vacancy of the surface of Co3O4 and the ozone decompose into oxygen, while another oxygen atom is left on the surface of Co3O4 and form lattice oxygen (O2−). Subsequently, the ozone molecule reacts with lattice oxygen and form oxygen and O22−. Finally, the O22− breaks off the Co3O4 surface in form of oxygen.
Conclusion
In this work, Co3O4@SiO2 and xCo3O4/SiO2 (x = 10, 15, 20, and 25) catalysts were successfully synthesized using the hydrothermal method. Under similar loading conditions, the ozone removal rates of Co3O4@SiO2 and 25Co3O4/SiO2 were nearly the same under flow conditions of 40 ppm ozone and 20% relative humidity. When the relative humidity increased to 60%, the ozone removal rate of Co3O4@SiO2 was higher than that of 25Co3O4@SiO2. XRD, XPS, and BET characterizations indicate that the high Co3O4@SiO2 performance is related to the core@shell structure. This study thus provides insight for developing catalysts to effectively remove gaseous ozone.
Data Availability Statement
The original contributions presented in the study are included in the article/Supplementary Material, further inquiries can be directed to the corresponding authors.
Author Contributions
FC and QX conceived the idea. JD, YC, and FH designed and fabricated the sample, ZM, DC, MC, GZ, JK, and SX conducted the the expriment. All the authors contrubuted to analysis of the data and draft of the manuscript.
Funding
National Key Research and Development Program of China 2016YFC0209203 Funding for school-level research projects of Yancheng Institute of Technology xjr2019055.
Conflict of Interest
The authors declare that the research was conducted in the absence of any commercial or financial relationships that could be construed as a potential conflict of interest.
Publisher’s Note
All claims expressed in this article are solely those of the authors and do not necessarily represent those of their affiliated organizations, or those of the publisher, the editors and the reviewers. Any product that may be evaluated in this article, or claim that may be made by its manufacturer, is not guaranteed or endorsed by the publisher.
Supplementary Material
The Supplementary Material for this article can be found online at: https://www.frontiersin.org/articles/10.3389/fchem.2021.803464/full#supplementary-material
References
Abbass, O. A., Sailor, D. J., and Gall, E. T. (2017). Effectiveness of Indoor Plants for Passive Removal of Indoor Ozone. Building Environ. 119, 62–70. doi:10.1016/j.buildenv.2017.04.007
Abdedayem, A., Guiza, M., Rivas Toledo, F. J., and Ouederni, A. (2017). Ozone Decomposition over Cobalt Supported on Olive Stones Activated Carbon: Effect of Preparation Method on Catalyst Activity. Ozone: Sci. Eng. 39, 435–446. doi:10.1080/01919512.2017.1331729
Agilandeswari, K., and Rubankumar, A. (2016). Synthesis, Characterization, Optical, and Magnetic Properties of Co3O4 Nanoparticles by Quick Precipitation. Synth. Reactivity Inorg. Metal-Organic, Nano-Metal Chem. 46, 502–506. doi:10.1080/15533174.2014.988807
Alameddine, M., Siraki, A., Tonoyan, L., and Gamal El-Din, M. (2021). Treatment of a Mixture of Pharmaceuticals, Herbicides and Perfluorinated Compounds by Powdered Activated Carbon and Ozone: Synergy, Catalysis and Insights into Non-free OH Contingent Mechanisms. Sci. Total Environ. 777, 146138. doi:10.1016/j.scitotenv.2021.146138
Deng, H., Kang, S., Ma, J., Wang, L., Zhang, C., and He, H. (2019). Role of Structural Defects in MnOx Promoted by Ag Doping in the Catalytic Combustion of Volatile Organic Compounds and Ambient Decomposition of O3. Environ. Sci. Technol. 53, 10871–10879. doi:10.1021/acs.est.9b01822
Ferrara, F., Pambianchi, E., Pecorelli, A., Woodby, B., Messano, N., Therrien, J.-P., et al. (2020). Redox Regulation of Cutaneous Inflammasome by Ozone Exposure. Free Radic. Biol. Med. 152, 561–570. doi:10.1016/j.freeradbiomed.2019.11.031
Ferrara, F., Pambianchi, E., Woodby, B., Messano, N., Therrien, J.-P., Pecorelli, A., et al. (2021). Evaluating the Effect of Ozone in UV Induced Skin Damage. Toxicol. Lett. 338, 40–50. doi:10.1016/j.toxlet.2020.11.023
Gao, Z., Zhao, D., Cheng, Q., Zhao, D., Yang, Y., Tian, Y., et al. (2021). Mesoporous SiO 2 ‐Encapsulated Nano‐Co 3 O 4 Catalyst for Efficient CO Oxidation. Chemcatchem 13, 4010–4018. doi:10.1002/cctc.202100602
Gong, S., Chen, J., Wu, X., Han, N., and Chen, Y. (2018). In-situ Synthesis of Cu2O/reduced Graphene Oxide Composite as Effective Catalyst for Ozone Decomposition. Catal. Commun. 106, 25–29. doi:10.1016/j.catcom.2017.12.003
Gong, S., Li, W., Xie, Z., Ma, X., Liu, H., Han, N., et al. (2017). Low Temperature Decomposition of Ozone by Facilely Synthesized Cuprous Oxide Catalyst. New J. Chem. 41, 4828–4834. doi:10.1039/c7nj00253j
Gopi, T., Swetha, G., Chandra Shekar, S., Ramakrishna, C., Saini, B., Krishna, R., et al. (2017). Catalytic Decomposition of Ozone on Nanostructured Potassium and Proton Containing δ-MnO2 Catalysts. Catal. Commun. 92, 51–55. doi:10.1016/j.catcom.2017.01.002
Jia, J., Zhang, P., and Chen, L. (2016). Catalytic Decomposition of Gaseous Ozone over Manganese Dioxides with Different Crystal Structures. Appl. Catal. B: Environ. 189, 210–218. doi:10.1016/j.apcatb.2016.02.055
Khan, S. A., Khan, S. B., and Asiri, A. M. (2015). Core-shell Cobalt Oxide Mesoporous Silica Based Efficient Electro-Catalyst for Oxygen Evolution. New J. Chem. 39, 5561–5569. doi:10.1039/c5nj00521c
Kim, S. H., An, H.-R., Lee, M., Hong, Y., Shin, Y., Kim, H., et al. (2022). High Removal Efficiency of Industrial Toxic Compounds through Stable Catalytic Reactivity in Water Treatment System. Chemosphere 287, 132204. doi:10.1016/j.chemosphere.2021.132204
Li, X., Ma, J., and He, H. (2020). Recent Advances in Catalytic Decomposition of Ozone. J. Environ. Sci. 94, 14–31. doi:10.1016/j.jes.2020.03.058
Ma, J., Wang, C., and He, H. (2017). Transition Metal Doped Cryptomelane-type Manganese Oxide Catalysts for Ozone Decomposition. Appl. Catal. B: Environ. 201, 503–510. doi:10.1016/j.apcatb.2016.08.050
Namdari, M., Lee, C.-S., and Haghighat, F. (2021). Active Ozone Removal Technologies for A Safe Indoor Environment: A Comprehensive Review. Building Environ. 187, 107370. doi:10.1016/j.buildenv.2020.107370
Nazaroff, W. W., and Weschler, C. J. (2021). Indoor Ozone: Concentrations and Influencing Factors. Indoor Air 00, 1–21. doi:10.1111/ina.12942
Nikolov, P., Genov, K., Konova, P., Milenova, K., Batakliev, T., Georgiev, V., et al. (2010). Ozone Decomposition on Ag/SiO2 and Ag/clinoptilolite Catalysts at Ambient Temperature. J. Hazard. Mater. 184, 16–19. doi:10.1016/j.jhazmat.2010.07.056
Ou, J., Yuan, Z., Zheng, J., Huang, Z., Shao, M., Li, Z., et al. (2016). Ambient Ozone Control in a Photochemically Active Region: Short-Term Despiking or Long-Term Attainment? Environ. Sci. Technol. 50, 5720–5728. doi:10.1021/acs.est.6b00345
Tang, W.-X., Liu, H.-D., Wu, X.-F., and Chen, Y.-F. (2014a). Higher Oxidation State Responsible for Ozone Decomposition at Room Temperature over Manganese and Cobalt Oxides: Effect of Calcination Temperature. Ozone: Sci. Eng. 36, 502–512. doi:10.1080/01919512.2014.894454
Tao, Z., Fu, M., and Liu, Y. (2021b). A Mini-Review of Carbon-Resistant Anode Materials for Solid Oxide Fuel Cells. Sust. Energ. Fuels 5, 5420–5430. doi:10.1039/d1se01300a7
Tao, Z., Fu, M., Liu, Y., Gao, Y., Tong, H., Hu, W., et al. (2021a). High-performing Proton-Conducting Solid Oxide Fuel Cells with Triple-Conducting Cathode of Pr0.5Ba0.5(Co0.7Fe0.3)O3-δ Tailored with W. Int. J. Hydrogen Energ. doi:10.1016/j.ijhydene.2021.10.145
Xie, R., Li, D., Hou, B., Wang, J., Jia, L., and Sun, Y. (2011). Solvothermally Derived Co3O4@m-SiO2 Nanocomposites for Fischer-Tropsch Synthesis. Catal. Commun. 12, 380–383. doi:10.1016/j.catcom.2010.10.010
Yang, S., Zhu, Z., Wei, F., and Yang, X. (2017). Carbon Nanotubes/Activated Carbon Fiber Based Air Filter media for Simultaneous Removal of Particulate Matter and Ozone. Building Environ. 125, 60–66. doi:10.1016/j.buildenv.2017.08.040
Yu, Y., Ji, J., Li, K., Huang, H., Shrestha, R. P., Kim Oanh, N. T., et al. (2020). Activated Carbon Supported MnO Nanoparticles for Efficient Ozone Decomposition at Room Temperature. Catal. Today 355, 573–579. doi:10.1016/j.cattod.2019.05.063
Keywords: ozone decomposition, Co3O4, SiO2, Core@Shell constructure, relative humidity 1. introduction
Citation: Ding J, Cheng F, Meng Z, Cao Y, Han F, Chen D, Cao M, Zhang G, Kang J, Xu S and Xu Q (2021) Core-Shell-Like Structured Co3O4@SiO2 Catalyst for Highly Efficient Catalytic Elimination of Ozone. Front. Chem. 9:803464. doi: 10.3389/fchem.2021.803464
Received: 28 October 2021; Accepted: 17 November 2021;
Published: 09 December 2021.
Edited by:
Lin Sun, Yancheng Institute of Technology, ChinaReviewed by:
Zetian Tao, University of South China, ChinaJunjian Miao, Shanghai Ocean University, China
Copyright © 2021 Ding, Cheng, Meng, Cao, Han, Chen, Cao, Zhang, Kang, Xu and Xu. This is an open-access article distributed under the terms of the Creative Commons Attribution License (CC BY). The use, distribution or reproduction in other forums is permitted, provided the original author(s) and the copyright owner(s) are credited and that the original publication in this journal is cited, in accordance with accepted academic practice. No use, distribution or reproduction is permitted which does not comply with these terms.
*Correspondence: Feng Cheng, Y2hmQHljaXQuZWR1LmNu; Qi Xu, eWN4cXN0ZXZlQDE2My5jb20=