- 1School of Mechanical and Electronic Engineering, Nanjing Forestry University, Nanjing, China
- 2School of Information Science and Engineering, Jiaxing University, Jiaxing, China
- 3School of Materials Science and Engineering, Southeast University, Nanjing, China
- 4School of Automotive and Transportation Engineering, Shenzhen Polytechnic, Shenzhen, China
Recently, expanding the applications of two-dimensional (2D) materials by constructing van der Waals (vdW) heterostructures has become very popular. In this work, the structural, electronic and optical absorption performances of the heterostructure based on AlN and Zr2CO2 monolayers are studied by first-principles simulation. It is found that AlN/Zr2CO2 heterostructure is a semiconductor with a band gap of 1.790 eV. In the meanwhile, a type-I band structure is constructed in AlN/Zr2CO2 heterostructure, which can provide a potential application of light emitting devices. The electron transfer between AlN and Zr2CO2 monolayer is calculated as 0.1603 |e| in the heterostructure, and the potential of AlN/Zr2CO2 heterostructure decreased by 0.663 eV from AlN layer to Zr2CO2 layer. Beisdes, the AlN/Zr2CO2 vdW heterostructure possesses excellent light absorption ability of in visible light region. Our research provides a theoretical guidance for the designing of advanced functional heterostructures.
Introduction
In 2004, the graphene was prepared and discovered to possess abundant interesting performances (Geim and Novoselov, 2007), which has also encouraged researchers to explore other two-dimensional (2D) materials (Pumera and Sofer, 2017; Sun et al., 2020a; Wang et al., 2020a; Sun and Schwingenschlögl, 2020; Zhang et al., 2021a; Tan et al., 2021) different with bulk materials (Chen et al., 2021). These 2D materials have attracted much attentions because of their unique electronic (Qi et al., 2020), magnetic (Wang et al., 2020b), thermal (Xie et al., 2014; Qin et al., 2019a), mechanical (Qin and Liu, 2017) and optical properties (Wang et al., 2020c). For example, at room temperature, black phosphorus with a thickness of less than 7.5 nm can display transistor performance, and the leakage current modulation order is 105 (Li et al., 2014). Arsenene can adjust its band gap by applying external strain on the surface (Kamal and Ezawa, 2015). Based on transition-metal dichalcogenides (TMDs), PtS2, the mobility of field effect transistors (FETs) has been proved to be at least 200 cm2/V·s (Pi et al., 2019). All those desirable characteristics promise 2D materials in future advanced applications, such as, photocatalyst (Wang et al., 2019; Zhang et al., 2020a), metal-ion batteries (Sun and Schwingenschlögl, 2021a), and photoelectric devices (Zhang et al., 2020b; Lou et al., 2021).
Recently, in order to further extend the performance and application range of these 2D materials, the prediction of new 2D materials (Sun et al., 2020b; Ding et al., 2020; Sun and Schwingenschlögl, 2021b; Zhang et al., 2021b; Sun et al., 2021) and the modification of known 2D materials have become more and more exciting (Liu et al., 2019; Sun et al., 2019; Zhang et al., 2020c; Wang et al., 2021; Zheng et al., 2021). In many modification methods, two different materials are usually combined as a heterostructure by horizontal (Qin et al., 2019b; Ren et al., 2020a) or vertical direction [(Wang et al., 2020d), (Wang et al., 2020c)]. In particular, the vertical heterostructure is constructed by weak van der Waals (vdW) force at the interface instead of covalent bond, which can result tremendous and novel performances. For example, the type-II heterostructure possesses staggered band alignment, which has ability to separate the photogenerated electrons and holes, revealing a promising application as photocatalyst. It also has been proved by some theoretical and experimental investigations, such as TMDs/BP (Ren et al., 2019a), h-BN/C2N (Wang et al., 2020e), TMDs/Mg(OH)2 (Luo et al., 2019) etc. The type-I band structure in heterostructure can make the charge transfer from wide band gap materials to narrow band gap materials, which can be pretty reflected in light-emitting devices such as LEDs (Bellus et al., 2017; Ren et al., 2021b). Interestingly, the band structure of black/red phosphorus heterostructure can be transformed from type-I to Z-scheme system by quantum confinement effect (Shi et al., 2019). TMDs based heterostructure, such as MoTe2/WSe2, has excellent photoluminescence (about 1.1 eV from MoTe2), which provides promising optoelectronic applications (Yamaoka et al., 2018). Furthermore, type-I heterostructure also can be used as a photocatalyst for water splitting because of remarkable light absorption characteristics (Do et al., 2020; Zhu et al., 2021a). More recently, 2D aluminum nitride (AlN) has attracted significant focus because of novel electronic (Zhang, 2012) and magnetic (Zhang and Zheng, 2011) performances, which also can be tuned by doping (Bai et al., 2015). Besides, some heterostructure constructed by AlN also have been studied, such as BiSb/AlN (Singh and Romero, 2017) and AlN/BP (Yang et al., 2017) etc. Importantly, it has been reported that the AlN films can be prepared on 6H–SiC substrates by various sputtering pressures by RF reactive magnetron sputtering (Kuang et al., 2012) and the AlN nanowires was also has been synthetized (Xu et al., 2003), which demonstrated the preparation method for AlN monolayer. At the same time, the Zr2CO2 as a MXene materials has been studied extensively to form vdW heterostructure (Zhu et al., 2021b). InSe/Zr2CO2 heterostructure possesses unique electron mobility (about 104 cm2/V·s) as a photocatalyst (He et al., 2019). MoS2/Zr2CO2 heterostructure also has decent band edge positions for the redox reaction of the water splitting (Xu et al., 2020). Interestingly, Zr2CO2/blue phosphorene heterostructure has a transformable band structure between type-I and type-II under external strain (Guo et al., 2017). Moreover, the MXene also can be prepared by suitable means (Lei et al., 2015). Therefore, both AlN and MXene possess possibility of preparation, which also show the future synthetic work on AlN/MXene heterostructure. And the investigations about the heterostructure based on AlN and Zr2CO2 monolayer are rare, it is excited to explore the novel properties and the potential application of the AlN/Zr2CO2 heterostructure.
In this work, the AlN and Zr2CO2 are selected to build a heterostructure. Using first-principle theoretical calculation methods, the structural and electronic natures of the AlN/Zr2CO2 heterostructure are addressed, which shows that the type-I band alignment in AlN/Zr2CO2 heterostructure gives a potential usage of light-emitting devices. Then, the interfacial performances of the heterostructure are calculated by charge density and potential drop. Finally, the light absorption capacity of the AlN/Zr2CO2 heterostructure is explored.
Computing Method
In this simulations work, the calculations were performed by first-principles method using density functional theory by the circumstances of Vienna ab initio simulation package (Kresse and Furthmüller, 1996a; Kresse and Furthmüller, 1996b; Capelle, 2006). The generalized gradient approximation and the projector augmented wave potentials were considered to explain the exchange correlation functional (Kresse and Joubert, 1999; Grimme, 2006). Besides, the DFT-D3 method was conducted using Grimme to demonstrate the weak disperson forces (Grimme et al., 2010). Furthermore, the Heyd–Scuseria–Ernzerhof hybrid method was used for decent electronic and optical results of the studied system (Heyd et al., 2005). Moreover, the energy cut-off was 500 eV. The Monkhorst–Pack k-point grids was 15 × 15 × 1 and the vacuum space was set as 25 Å, which can efficiently prevent the interaction of nearby layers. The convergence standard for force and energy were limited in 0.01 eV·Å−1 and smaller than 0.01 meV, respectively.
Results and Discussion
First, the AlN/Zr2CO2 is optimized by a decent lattice constant of 3.365 Å, which is comparable with of the AlN (3.127 Å) (Ren et al., 2020b) and Zr2CO2 (3.294 Å) (Guo et al., 2017) monolayers. When monolayered AlN and Zr2CO2 are combined to form the heterostructure, considering that there are various combination modes of AlN and Zr2CO2 monolayers, we only select the most representative highly symmetrical combination configurations among them. These six combination styles of AlN/Zr2CO2 heterostructure are shown as Figures 1A–F, named AO-1 to AO-6, respectively. For AO-1, the N and Al atoms are located on the upper O and upper Zr atoms, respectively. The AO-2 is obtained by putting the N and Al atoms on the C and lower O atoms, respectively. The AO-3 is built by locating the N and Al atoms on the C and lower Zr atoms, respectively. Then, fixing the N and Al atoms on the lower O and lower Zr atoms, respectively, can construct the AlN/Zr2CO2 heterostructure by AO-4 configuration. Differently, locating the N and Al atoms on the lower O and C atoms, respectively, can build the AO-5 configuration. Furthermore, AO-6 configuration is constructed by fixing the N and Al atoms on upper O and C atoms, respectively. Besides, the most stable stacking configuration of the AlN/Zr2CO2 heterostructure is decided by the binding energy, represented by Eb as follow:
where EAlN/Zr2CO2, EAlN and EZr2CO2 are showing the total energy of the AlN/Zr2CO2 system, original AlN and Zr2CO2 monolayers, respectively. Furthermore, the calculation demonstrations that the stacked structure in Figure 1A is the most stable heterostructure with binding energy of –36.05 meV/Å2, which also proves that the single-layer AlN and Zr2CO2 are constructed by vdW force (Chen et al., 2013). In addition, the distance of interface and the bond length of these different stacking configurations of the optimized AlN/Zr2CO2 heterostructure are calculated in Table 1. Moreover, the following discussion in this work is based on the most stable stacking structure of AO-1.
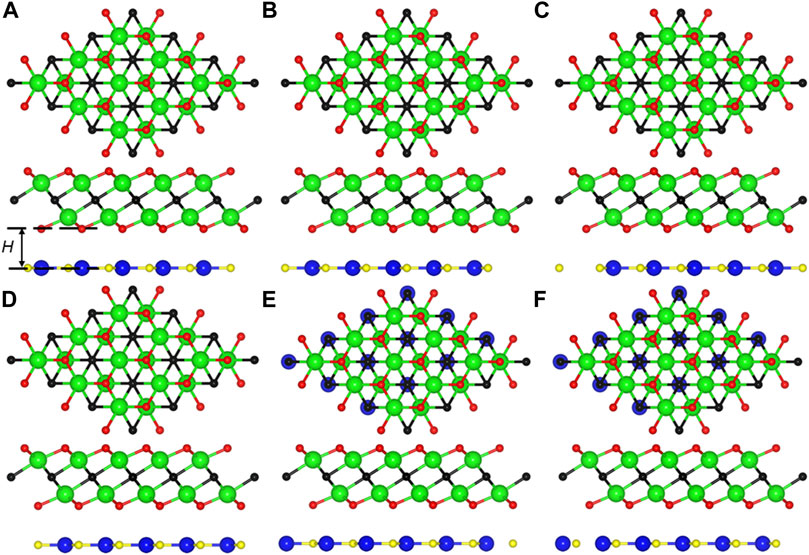
FIGURE 1. The top and side views of the (A) AO-1, (B) AO-2, (C) AO-3, (D) AO-4, (E) AO-5, (F) AO-6 stacking configurations for the AlN/Zr2CO2 heterostructure, the blue, yellow, cyan, black and red balls represent the Al, N, Zr, C, and O atoms, respectively.
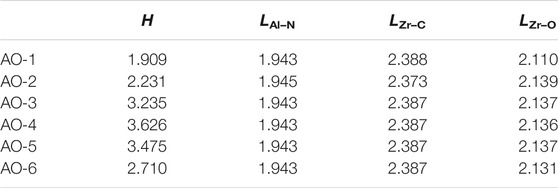
TABLE 1. The optimized distance of interface (H, Å) and the bond length (L, Å) of the AlN/Zr2CO2 heterostructure with different stacking styles.
The projected band energy of AlN/Zr2CO2 vdW heterostructure is obtained by HSE06 calculation, as shown in Figure 2A. One can clearly find that AlN/Zr2CO2 has a semiconductor nature with indirect band gap of 1.790 eV. In addition, the red and black marks are contributed from AlN and Zr2CO2 monolayers, respectively, suggesting that the (conduction band minimum) CBM and (the valence band maximum) VBM of AlN/Zr2CO2 vdW heterostructure are mainly resulted by Zr2CO2 monolayer. Thus, a type-I band structure is constructed in AlN/Zr2CO2 vdW heterostructure. Besides, the partial density of AlN/Zr2CO2 vdW heterostructure, as shown in Figures 2B, 2is also obtained to further prove the characteristics of intrinsic type-I band structure. It is obvious that the CBM and the VBM of the AlN/Zr2CO2 vdW heterostructure are mainly donated by Zr and O atoms, respectively.
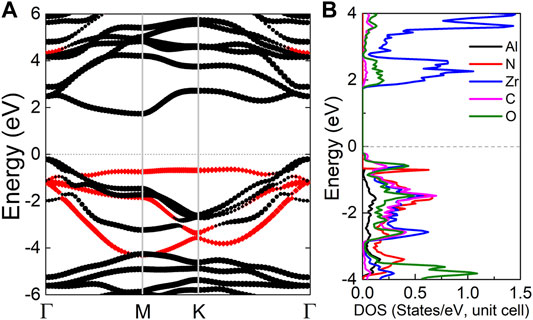
FIGURE 2. (A) The projected band structure and (B) the partial density of states of the AlN/Zr2CO2 vdW heterostructure, the Fermi level is expressed by 0 shown as dash line.
Such type-I band structure in the AlN/Zr2CO2 vdW heterostructure provides some important advanced applications in nano-devices. In AlN/Zr2CO2 vdW heterostructure, as shown in Figure 3A, CBM and VBM of AlN/Zr2CO2 vdW heterostructure are contributed by single-layer Zr2CO2, and the band gap of single-layer Zr2CO2 is less than that of single-layer AlN. When AlN/Zr2CO2 vdW heterostructure is excited by some external conditions, the electrons in the broad-band gap AlN monolayer are inspired and transferred to the CBM, generating holes at the VBM. It is worth noting that under the action of conduction band offset, CBO (valence band offset, VBO), electrons (holes) at the CBM (VBM) of the AlN layer can be transferred to the CBM (VBM) of the Zr2CO2 layer. Besides, the obtained CBO and VBO in AlN/Zr2CO2 vdW heterostructure are 2.432 and 0.471 eV respectively. While the electrons and holes excited in the relatively narrow-band gap of Zr2CO2 layer cannot be transferred to AlN layer due to low energy, in Figure 3B, which explains the AlN/Zr2CO2 vdW heterostructure can be considered as a potential light-emitting device material.
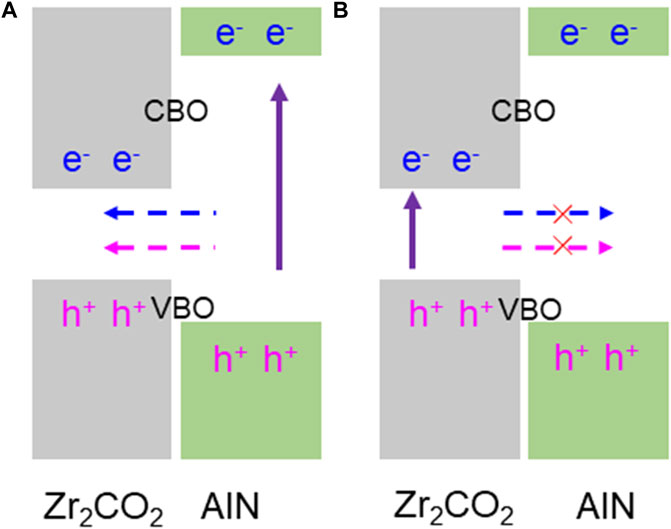
FIGURE 3. Band alignment schematic of the excited charge transfer mode of the AlN/Zr2CO2 vdW heterostructure: (A) feasible and (B) limited charge migration mode.
Then, we discussed the interface properties of AlN/Zr2CO2 vdW heterostructure by the charge density difference (Δρ) and the potential drop (ΔV) in the interface. The charge density difference across the interface of the AlN/Zr2CO2 vdW heterostructure is calculated by:
where ρAlN/Zr2CO2, ρAlN and ρZr2CO2 represent the charge density of the AlN/Zr2CO2 vdW heterostructure, monolayered AlN and Zr2CO2, respectively. Demonstrated by Figure 4A, the Zr2CO2 layer acts as an electron acceptor and AlN is an electron donor layer. Through Bader charge analysis (Tang et al., 2009), the obtained charge transfer from AlN layer to Zr2CO2 layer is 0.1603 |e| in AlN/Zr2CO2 vdW heterostructure. Importantly, there is a certain degree of potential drop across the interface of the AlN/Zr2CO2 vdW heterostructure, shown as Figure 4B, and the calculated potential drop of 0.663 eV also play a critical role to assist the migration of the excited electrons and holes between the AlN/Zr2CO2 vdW heterostructure (Wang et al., 2018).
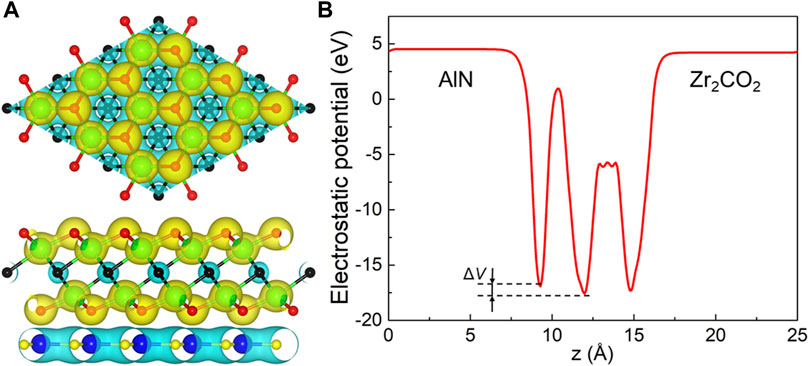
FIGURE 4. (A) The charge density difference and the (B) potential drop of the AlN/Zr2CO2 vdW heterostructure. The yellow and cyan regions contribute the gaining and losing of electrons, respectively.
In order to produce more efficient light-emitting device, active materials should be able to effectively absorb light in the visible and near-infrared regions, especially type-I heterostructure. Therefore, we investigated the light absorption performance of AlN/Zr2CO2 vdW heterostructure by the calculation:
where ω is the angular frequency; α shows absorption coefficient and c is the speed of light. Besides,
Conclusion
In conclusion, the AlN and Zr2CO2 monolayers are constructed by vdW force to form a heterostructure. And the most stable AlN/Zr2CO2 is decided by the lowest binding energy of about –36.05 meV/Å2. The, the HSE06 obtained projected band structure shows the AlN/Zr2CO2 vdW heterostructure possesses semiconductor nature with a band gap of 1.790 eV, and presents a type-I energy band alignment, which is a satisfaction candidate for light-emitting devices. Furthermore, the interface characteristics of AlN/Zr2CO2 vdW heterostructure is investigated by charge density difference (0.1603|e| from AlN layer to Zr2CO2 layer) and potential drop (0.663 eV). Moreover, the AlN/Zr2CO2 vdW heterostructure explains a remarkable light absorption performance, which can further offer excellent technical guidance for nano light-emitting device materials.
Data Availability Statement
The raw data supporting the conclusions of this article will be made available by the authors, without undue reservation.
Author Contributions
All authors listed have made a substantial, direct, and intellectual contribution to the work and approved it for publication.
Funding
This investigation was supported by the Open Fund Project of Maanshan Engineering Technology, Research Center of Advanced Design for Automotive Stamping Dies (Grant number: QMSG202105).
Conflict of Interest
The authors declare that the research was conducted in the absence of any commercial or financial relationships that could be construed as a potential conflict of interest.
Publisher’s Note
All claims expressed in this article are solely those of the authors and do not necessarily represent those of their affiliated organizations, or those of the publisher, the editors and the reviewers. Any product that may be evaluated in this article, or claim that may be made by its manufacturer, is not guaranteed or endorsed by the publisher.
Acknowledgments
KR thanks the potential help by Xiaoqian Xu.
References
Bai, Y., Deng, K., and Kan, E. (2015). Electronic and Magnetic Properties of an AlN Monolayer Doped with First-Row Elements: a First-Principles Study. RSC Adv. 5, 18352–18358. doi:10.1039/c4ra13522a
Bellus, M. Z., Li, M., Lane, S. D., Ceballos, F., Cui, Q., Zeng, X. C., et al. (2017). Type-I van der Waals heterostructure formed by MoS2 and ReS2 monolayers. Nanoscale Horiz. 2, 31–36. doi:10.1039/c6nh00144k
Capelle, K. (2006). A Bird's-Eye View of Density-Functional Theory. Braz. J. Phys. 36, 1318–1343. doi:10.1590/s0103-97332006000700035
Chen, M., Lu, Y., Wang, Z., Lan, H., Sun, G., and Ni, Z. (2021). Melt Pool Evolution on Inclined NV E690 Steel Plates during Laser Direct Metal Deposition. Opt. Laser Techn. 136, 106745. doi:10.1016/j.optlastec.2020.106745
Chen, X., Tian, F., Persson, C., Duan, W., and Chen, N.-x. (2013). Interlayer Interactions in Graphites. Sci. Rep. 3, 3046. doi:10.1038/srep03046
Ding, X.-Y., Zhang, C., Wang, D.-Q., Li, B.-S., Wang, Q., Yu, Z. G., et al. (2020). A New Carbon Allotrope:T5-Carbon. Scripta Materialia 189, 72–77. doi:10.1016/j.scriptamat.2020.08.004
Do, T.-N., Idrees, M., Binh, N. T. T., Phuc, H. V., Hieu, N. N., Hoa, L. T., et al. (2020). Type-I band alignment of BX-ZnO (X = As, P) van der as high-efficiency water splitting photocatalysts: a first-principles study. RSC Adv. 10, 44545–44550. doi:10.1039/d0ra09701b
Geim, A. K., and Novoselov, K. S. (2007). The Rise of Graphene. Nat. Mater 6, 183–191. doi:10.1038/nmat1849
Grimme, S., Antony, J., Ehrlich, S., and Krieg, H. (2010). A Consistent and Accurate Ab Initio Parametrization of Density Functional Dispersion Correction (DFT-D) for the 94 Elements H-Pu. J. Chem. Phys. 132, 154104. doi:10.1063/1.3382344
Grimme, S. (2006). Semiempirical GGA-type Density Functional Constructed with a Long-Range Dispersion Correction. J. Comput. Chem. 27, 1787–1799. doi:10.1002/jcc.20495
Guo, Z., Miao, N., Zhou, J., Sa, B., and Sun, Z. (2017). Strain-mediated type-I/type-II transition in MXene/Blue phosphorene van der Waals heterostructures for flexible optical/electronic devices. J. Mater. Chem. C 5, 978–984. doi:10.1039/c6tc04349f
He, Y., Zhang, M., Shi, J.-j., Cen, Y.-l., and Wu, M. (2019). Improvement of Visible-Light Photocatalytic Efficiency in a Novel InSe/Zr2CO2 Heterostructure for Overall Water Splitting. J. Phys. Chem. C 123, 12781–12790. doi:10.1021/acs.jpcc.9b01175
Heyd, J., Peralta, J. E., Scuseria, G. E., and Martin, R. L. (2005). Energy Band Gaps and Lattice Parameters Evaluated with the Heyd-Scuseria-Ernzerhof Screened Hybrid Functional. J. Chem. Phys. 123, 174101. doi:10.1063/1.2085170
Kamal, C., and Ezawa, M. (2015). Arsenene: Two-Dimensional Buckled and Puckered Honeycomb Arsenic Systems. Phys. Rev. B 91, 085423. doi:10.1103/physrevb.91.085423
Kresse, G., and Furthmüller, J. (1996). Efficiency of Ab-Initio Total Energy Calculations for Metals and Semiconductors Using a Plane-Wave Basis Set. Comput. Mater. Sci. 6, 15–50. doi:10.1016/0927-0256(96)00008-0
Kresse, G., and Furthmüller, J. (1996). Efficient Iterative Schemes Forab Initiototal-Energy Calculations Using a Plane-Wave Basis Set. Phys. Rev. B 54, 11169–11186. doi:10.1103/physrevb.54.11169
Kresse, G., and Joubert, D. (1999). From Ultrasoft Pseudopotentials to the Projector Augmented-Wave Method. Phys. Rev. B 59, 1758–1775. doi:10.1103/physrevb.59.1758
Kuang, X.-P., Zhang, H.-Y., Wang, G.-G., Cui, L., Zhu, C., Jin, L., et al. (2012). AlN Films Prepared on 6H-SiC Substrates under Various Sputtering Pressures by RF Reactive Magnetron Sputtering. Appl. Surf. Sci. 263, 62–68. doi:10.1016/j.apsusc.2012.08.121
Lei, J.-C., Zhang, X., and Zhou, Z. (2015). Recent Advances in MXene: Preparation, Properties, and Applications. Front. Phys. 10, 276–286. doi:10.1007/s11467-015-0493-x
Li, J., Huang, Z., Ke, W., Yu, J., Ren, K., and Dong, Z. (2021). High solar-to-hydrogen efficiency in Arsenene/GaX (X = S, Se) van der Waals heterostructure for photocatalytic water splitting. J. Alloy. Compound. 866. doi:10.1016/j.jallcom.2021.158774
Li, L., Yu, Y., Ye, G. J., Ge, Q., Ou, X., Wu, H., et al. (2014). Black Phosphorus Field-Effect Transistors. Nat. Nanotech 9, 372–377. doi:10.1038/nnano.2014.35
Liu, X., Zhang, Z., Luo, Z., Lv, B., and Ding, Z. (2019). Tunable Electronic Properties of Graphene/g-AlN Heterostructure: The Effect of Vacancy and Strain Engineering. Nanomaterials 9, 1674. doi:10.3390/nano9121674
Lou, J., Ren, K., Huang, Z., Huo, W., Zhu, Z., and Yu, J. (2021). Electronic and Optical Properties of Two-Dimensional Heterostructures Based on Janus XSSe (X = Mo, W) and Mg(OH)2: a First Principles Investigation. RSC Adv. 11, 29576–29584. doi:10.1039/d1ra05521f
Luo, Y., Wang, S., Ren, K., Chou, J.-P., Yu, J., Sun, Z., et al. (2019). Transition-metal dichalcogenides/Mg(OH)2 van der Waals heterostructures as promising water-splitting photocatalysts: a first-principles study. Phys. Chem. Chem. Phys. 21, 1791–1796. doi:10.1039/c8cp06960c
Pi, L., Li, L., Liu, K., Zhang, Q., Li, H., and Zhai, T. (2019). Recent Progress on 2D Noble‐Transition‐Metal Dichalcogenides. Adv. Funct. Mater. 29, 1904932. doi:10.1002/adfm.201904932
Pumera, M., and Sofer, Z. (2017). 2D Monoelemental Arsenene, Antimonene, and Bismuthene: Beyond Black Phosphorus. Adv. Mater. 29, 1605299. doi:10.1002/adma.201605299
Qi, K., Xing, X., Zada, A., Li, M., Wang, Q., Liu, S.-y., et al. (2020). Transition Metal Doped ZnO Nanoparticles with Enhanced Photocatalytic and Antibacterial Performances: Experimental and DFT Studies. Ceramics Int. 46, 1494–1502. doi:10.1016/j.ceramint.2019.09.116
Qin, H., and Liu, Y. (2017). Interlayer Shear Behaviors of Graphene-Carbon Nanotube Network. J. Appl. Phys. 122, 125108. doi:10.1063/1.4992025
Qin, H., Pei, Q.-X., Liu, Y., and Zhang, Y.-W. (2019). The Mechanical and thermal Properties of MoS2-WSe2 Lateral Heterostructures. Phys. Chem. Chem. Phys. 21, 15845–15853. doi:10.1039/c9cp02499a
Qin, H., Pei, Q.-X., Liu, Y., and Zhang, Y.-W. (2019). Thermal Transport in Graphene-Based Layered Materials: An Analytical Model Validated with Extensive Molecular Dynamics Simulations. Carbon 155, 114–121. doi:10.1016/j.carbon.2019.08.062
Ren, K., Liu, X., Chen, S., Cheng, Y., Tang, W., and Zhang, G. (2020). Remarkable Reduction of Interfacial Thermal Resistance in Nanophononic Heterostructures. Adv. Funct. Mater. 30, 2004003. doi:10.1002/adfm.202004003
Ren, K., Luo, Y., Wang, S., Chou, J.-P., Yu, J., Tang, W., et al. (2019). A van der Waals Heterostructure Based on Graphene-like Gallium Nitride and Boron Selenide: A High-Efficiency Photocatalyst for Water Splitting. ACS Omega 4, 21689–21697. doi:10.1021/acsomega.9b02143
Ren, K., Shu, H., Huo, W., Cui, Z., Yu, J., and Xu, Y. (2021a). Mechanical, Electronic and Optical Properties of Novel B2P6 Monolayer: Ultrahigh Carrier Mobility and Strong Optical Absorption. Phys. Chem. Chem. Phys.. doi:10.1039/D1CP03838A
Ren, K., Sun, M., Luo, Y., Wang, S., Yu, J., and Tang, W. (2019). First-principle Study of Electronic and Optical Properties of Two-Dimensional Materials-Based Heterostructures Based on Transition Metal Dichalcogenides and boron Phosphide. Appl. Surf. Sci. 476, 70–75. doi:10.1016/j.apsusc.2019.01.005
Ren, K., Wang, S., Luo, Y., Chou, J.-P., Yu, J., Tang, W., et al. (2020). High-efficiency photocatalyst for water splitting: a Janus MoSSe/XN (X = Ga, Al) van der Waals heterostructure. J. Phys. D: Appl. Phys. 53, 185504. doi:10.1088/1361-6463/ab71ad
Ren, K., Zheng, R., Xu, P., Cheng, D., Huo, W., Yu, J., et al. (2021b). Electronic and Optical Properties of Atomic-Scale Heterostructure Based on MXene and MN (M = Al, Ga): A DFT Investigation. Nanomaterials 11, 2236. doi:10.3390/nano11092236
Shi, R., Liu, F., Wang, Z., Weng, Y., and Chen, Y. (2019). Black/red Phosphorus Quantum Dots for Photocatalytic Water Splitting: from a Type I Heterostructure to a Z-Scheme System. Chem. Commun. 55, 12531–12534. doi:10.1039/c9cc06146k
Singh, S., and Romero, A. H. (2017). Giant Tunable Rashba Spin Splitting in a Two-Dimensional BiSb Monolayer and in BiSb/AlN Heterostructures. Phys. Rev. B 95, 165444. doi:10.1103/physrevb.95.165444
Sun, M., Chou, J.-P., Hu, A., and Schwingenschlögl, U. (2019). Point Defects in Blue Phosphorene. Chem. Mater. 31, 8129–8135. doi:10.1021/acs.chemmater.9b02871
Sun, M., Luo, Y., Yan, Y., and Schwingenschlögl, U. (2021). Ultrahigh Carrier Mobility in the Two-Dimensional Semiconductors B8Si4, B8Ge4, and B8Sn4. Chem. Mater. 33, 6475–6483. doi:10.1021/acs.chemmater.1c01824
Sun, M., and Schwingenschlögl, U. (2020). B2P6: A Two-Dimensional Anisotropic Janus Material with Potential in Photocatalytic Water Splitting and Metal-Ion Batteries. Chem. Mater. 32, 4795–4800. doi:10.1021/acs.chemmater.0c01536
Sun, M., and Schwingenschlögl, U. (2021). Structure Prototype Outperforming MXenes in Stability and Performance in Metal‐Ion Batteries: A High Throughput Study. Adv. Energ. Mater. 11, 2003633. doi:10.1002/aenm.202003633
Sun, M., and Schwingenschlögl, U. (2021). Unique Omnidirectional Negative Poisson's Ratio in δ-Phase Carbon Monochalcogenides. J. Phys. Chem. C 125, 4133–4138. doi:10.1021/acs.jpcc.0c11555
Sun, M., Schwingenschlögl, U., and δ-, C. S. (2020). A Direct-Band-Gap Semiconductor Combining Auxeticity, Ferroelasticity, and Potential for High-Efficiency Solar Cells. Phys. Rev. Appl. 14, 044015. doi:10.1103/physrevapplied.14.044015
Sun, M., Yan, Y., and Schwingenschlögl, U. (2020). Beryllene: A Promising Anode Material for Na- and K-Ion Batteries with Ultrafast Charge/Discharge and High Specific Capacity. J. Phys. Chem. Lett. 11, 9051–9056. doi:10.1021/acs.jpclett.0c02426
Tan, Z.-L., Wei, J.-X., Liu, Y., Rehman, W., Hou, L.-R., and Yuan, C.-Z. (2021). V2CTx MXene and its Derivatives: Synthesis and Recent Progress in Electrochemical Energy Storage Applications. Rare Met., 1–23.
Tang, W., Sanville, E., and Henkelman, G. (2009). A Grid-Based Bader Analysis Algorithm without Lattice Bias. J. Phys. Condens. Matter 21, 084204. doi:10.1088/0953-8984/21/8/084204
Wang, B., Wang, X., Wang, P., Yang, T., Yuan, H., Wang, G., et al. (2019). Bilayer MoSe2/HfS2 Nanocomposite as a Potential Visible-Light-Driven Z-Scheme Photocatalyst. Nanomaterials 9, 1706. doi:10.3390/nano9121706
Wang, G., Gong, L., Li, Z., Wang, B., Zhang, W., Yuan, B., et al. (2020). A Two-Dimensional CdO/CdS Heterostructure Used for Visible Light Photocatalysis. Phys. Chem. Chem. Phys. 22, 9587–9592. doi:10.1039/d0cp00876a
Wang, G., Li, Z., Wu, W., Guo, H., Chen, C., Yuan, H., et al. (2020). A Two-Dimensional h-BN/C2N Heterostructure as a Promising Metal-free Photocatalyst for Overall Water-Splitting. Phys. Chem. Chem. Phys. 22, 24446–24454. doi:10.1039/d0cp03925j
Wang, G., Tang, W., Geng, L., Li, Y., Wang, B., Chang, J., et al. (2020). Rotation Tunable Photocatalytic Properties of ZnO/GaN Heterostructures. Phys. Status Solidi B 257, 1900663. doi:10.1002/pssb.201900663
Wang, G., Zhang, L., Li, Y., Zhao, W., Kuang, A., Li, Y., et al. (2020). Biaxial Strain Tunable Photocatalytic Properties of 2D ZnO/GeC Heterostructure. J. Phys. D: Appl. Phys. 53, 015104. doi:10.1088/1361-6463/ab440e
Wang, K., Ren, K., Cheng, Y., Zhang, M., Wang, H., and Zhang, G. (2020). Effects of Molecular Adsorption on the Spin-Wave Spectrum and Magnon Relaxation in Two-Dimensional Cr2Ge2Te6. Phys. Chem. Chem. Phys. 22, 22047–22054. doi:10.1039/d0cp03884a
Wang, S., Tian, H., Ren, C., Yu, J., and Sun, M. (2018). Electronic and Optical Properties of Heterostructures Based on Transition Metal Dichalcogenides and Graphene-like Zinc Oxide. Sci. Rep. 8, 12009. doi:10.1038/s41598-018-30614-3
Wang, Z., Zhang, Z., Liu, S., Shao, C., Robertson, J., and Guo, Y. (2021). Impact of Carbon-Carbon Defects at the SiO2/4H-SiC (0001) Interface: a First-Principles Calculation. J. Phys. D: Appl. Phys. 55, 025109. doi:10.1088/1361-6463/ac2bc9
Xie, G., Shen, Y., Wei, X., Yang, L., Xiao, H., Zhong, J., et al. (2014). A Bond-Order Theory on the Phonon Scattering by Vacancies in Two-Dimensional Materials. Sci. Rep. 4, 5085. doi:10.1038/srep05085
Xu, C., Xue, L., Yin, C., and Wang, G. (2003). Formation and Photoluminescence Properties of AlN Nanowires. Phys. Stat. Sol. (A) 198, 329–335. doi:10.1002/pssa.200306612
Xu, X., Ge, X., Liu, X., Li, L., Fu, K., Dong, Y., et al. (2020). Two-dimensional M2CO2/MoS2 (M = Ti, Zr and Hf) van der Waals heterostructures for overall water splitting: A density functional theory study. Ceramics Int. 46, 13377–13384. doi:10.1016/j.ceramint.2020.02.119
Yamaoka, T., Lim, H. E., Koirala, S., Wang, X., Shinokita, K., Maruyama, M., et al. (2018). Efficient Photocarrier Transfer and Effective Photoluminescence Enhancement in Type I Monolayer MoTe2/WSe2 Heterostructure. Adv. Funct. Mater. 28, 1801021. doi:10.1002/adfm.201801021
Yang, Q., Tan, C.-J., Meng, R.-S., Jiang, J.-K., Liang, Q.-H., Sun, X., et al. (2017). AlN/BP Heterostructure Photocatalyst for Water Splitting. IEEE Electron. Device Lett. 38, 145–148. doi:10.1109/led.2016.2633487
Zhang, C.-w. (2012). First-principles Study on Electronic Structures and Magnetic Properties of AlN Nanosheets and Nanoribbons. J. Appl. Phys. 111, 043702. doi:10.1063/1.3686144
Zhang, C.-w., and Zheng, F.-b. (2011). First-principles Prediction on Electronic and Magnetic Properties of Hydrogenated AlN Nanosheets. J. Comput. Chem. 32, 3122–3128. doi:10.1002/jcc.21902
Zhang, C., Cao, Y., Liu, Y., Hu, H.-J., Yu, Z. G., and Zhang, Y.-W. (2021). Bct-C5: A New Body-Centered Tetragonal Carbon Allotrope. Diamond Relat. Mater. 119, 108571. doi:10.1016/j.diamond.2021.108571
Zhang, C., Zhou, H., Chen, S., Zhang, G., Yu, Z. G., Chi, D., et al. (2021). Recent Progress on 2D Materials-Based Artificial Synapses. Crit. Rev. Solid State. Mater. Sci., 1–26. doi:10.1080/10408436.2021.1935212
Zhang, Z., Guo, Y., and Robertson, J. (2020). Origin of Weaker Fermi Level Pinning and Localized Interface States at Metal Silicide Schottky Barriers. J. Phys. Chem. C 124. doi:10.1021/acs.jpcc.0c06228
Zhang, Z., Guo, Y., and Robertson, J. (2020). Termination-dependence of Fermi Level Pinning at Rare-Earth arsenide/GaAs Interfaces. Appl. Phys. Lett. 116, 251602. doi:10.1063/5.0007479
Zhang, Z., Huang, B., Qian, Q., Gao, Z., Tang, X., and Li, B. (2020). Strain-tunable III-nitride/ZnO Heterostructures for Photocatalytic Water-Splitting: A Hybrid Functional Calculation. APL Mater. 8, 041114. doi:10.1063/5.0005648
Zheng, Z., Ren, K., Huang, Z., Zhu, Z., Wang, K., Shen, Z., et al. (2021). Remarkably Improved Curie Temperature for Two-Dimensional CrI3 by Gas Molecular Adsorption: a DFT Study. Semicond. Sci. Tech. 36 (7), 075015. doi:10.1088/1361-6641/ac01a2
Zhu, X. T., Xu, Y., Cao, Y., Zhao, Y. Q., Sheng, W., Nie, G.-Z., et al. (2021). Investigation of the Electronic Structure of Two-Dimensional GaN/Zr2CO2 hetero-junction: Type-II Band Alignment with Tunable Bandgap. Appl. Surf. Sci. 542, 148505. doi:10.1016/j.apsusc.2020.148505
Keywords: first-principles calculation, AlN/Zr2CO2, type-I band alignment, applications, heterostructure
Citation: Ren K, Zheng R, Lou J, Yu J, Sun Q and Li J (2021) Ab Initio Calculations for the Electronic, Interfacial and Optical Properties of Two-Dimensional AlN/Zr2CO2 Heterostructure. Front. Chem. 9:796695. doi: 10.3389/fchem.2021.796695
Received: 17 October 2021; Accepted: 25 October 2021;
Published: 12 November 2021.
Edited by:
Zhaofu Zhang, University of Cambridge, United KingdomReviewed by:
Chao Zhang, Anhui University of Science and Technology, ChinaHuasong Qin, Xi’an Jiaotong University, China
Copyright © 2021 Ren, Zheng, Lou, Yu, Sun and Li. This is an open-access article distributed under the terms of the Creative Commons Attribution License (CC BY). The use, distribution or reproduction in other forums is permitted, provided the original author(s) and the copyright owner(s) are credited and that the original publication in this journal is cited, in accordance with accepted academic practice. No use, distribution or reproduction is permitted which does not comply with these terms.
*Correspondence: Jianping Li, szyljp0170@szpt.edu.cn; Qingyun Sun, sunqingyun@njfu.edu.cn
†These authors have contributed equally to this work and share first authorship