- Weifang Medical University, Weifang, China
A novel and efficient rearrangement of N-tosylhydrazones bearing allyl ethers into trans-olefin-substituted sulfonylhydrazones is proposed. The reaction involves breakage of the C-O bond and formation of the C-N bond. The reaction can be extended to a wide range of substrates, and the target products can be synthesized smoothly, regardless of the presence of electron-donating and electron-withdrawing groups. The proposed strategy is a new direction in the field of rearrangement reactions.
Introduction
Hydrazones are a class of Schiff bases with a special molecular structure containing a substructure (-NHN = C-). Many studies have shown that hydrazones possess a wide range of physiological activities, including antioxidant, anti-inflammatory, antibacterial, insecticidal, antiviral, and antitumor activities. In recent years, hydrazones have been highly valued in the fields of medicine, pesticides, materials science, and testing reagents, and have broad development prospects (Yang et al., 1996; Khan, 2008; Özbek et al., 2009; Özdemir et al., 2009; Belkheiri et al., 2010; Özdemir et al., 2010; Özkay et al., 2010).
QuinShimizu’s group developed a method for the oxidation of N-sulfonyl hydrazide catalyzed by lead tetraacetate (Scheme 1A) (Shimizu et al., 1980). Subsequently, Ashok et al. established a new scheme for the synthesis of sulfinates (Scheme 1B) through the K2CO3-catalyzed rapid conversion of N-sulfonyl hydrazide (Korawat and Basak, 2020). Hossain et al. reported a synthetic route to 1,3-disubstituted allenes through the CuI-catalyzed cross-coupling of N-tosylhydrazones with terminal alkynes (Scheme 1C) (Hossain et al., 2013). Furthermore, palladium-catalyzed allylation is a reliable and widely used method (Trost et al., 2006; Lu and Ma, 2007; Mohr and Stoltz, 2007; Weaver et al., 2011)and has been extensively used in total synthesis (Trost and Crawley, 2003; Enquist and Stoltz, 2008; Huters et al., 2012). Therefore, metal-catalyzed cleavage of C-O bonds of ethers remains an intriguing topic. Herein, we report the Pd-catalyzed rearrangement of N-tosylhydrazones bearing allyl ethers to produce trans-olefin-substituted sulfonylhydrazones (Scheme 1D).
Results and Discussion
When the reaction was carried out with N-tosylhydrazones 1a in the presence of Pd(PPh3)4 in THF and K2CO3 as the base, the desired product (E)-N-allyl-N'-(2-hydroxybenzylidene)-4-methylbenzene sulfonohydrazide 2a was obtained. Different catalysts were screened for the reaction, such as Pd(OAc)2, Pd(PPh3)Cl2, PdCl2, Pd2 (dba)3, and Pd(PPh3)4. Among these, Pd(PPh3)4 proved to be the best catalyst, which led to 55% yield of the final compound (Table 1, entries 2–6). When the reaction was carried out in the absence of a catalyst, the target compound was not obtained (Table 1, entry 7).
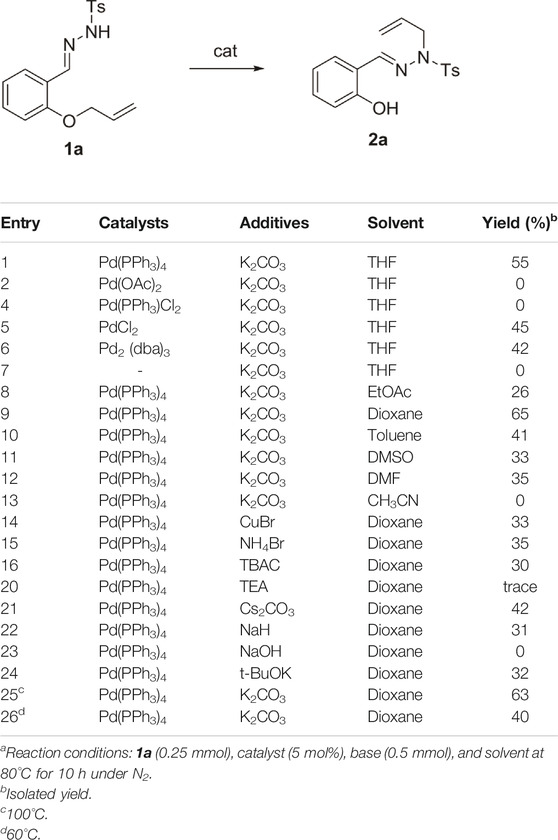
TABLE 1. Screening of reaction conditionsa.
Next, the reaction was carried out in different solvents such as toluene, EtOAc, dioxane, DMSO, DMF, and CH3CN to determine the optimal solvent (Table 1, entries 8–13). Subsequently, the effects of different additives such as CuBr, NH4Br, and TBAC, on the product yield were investigated. The product yield did not increase significantly in the presence of these additives (Table 1, entries 14 and 15). K2CO3 was the most effective in facilitating the reaction, while other bases such as TEA, Cs2CO3, NaH, NaOH, and t-BuOK led to significantly lower product yields. Increasing or decreasing the temperature had no significantly improve the reaction yield. (Table 1, entries 25, 26). Therefore, the optimal reaction conditions were 1a (0.25 mmol) as the substrate, Pd(PPh3)4 as the catalyst (5 mol%), and K2CO3 (0.5 mmol) as the base in dioxane (0.1 M) for 10 h at 80°C under N2 conditions.
With the optimal conditions in hand, we explored the scope of the reaction. First, we investigated the effect of various substituted N-sulfonylhydrazones as substrates (Scheme 2) on the reaction. The results revealed that the reaction conditions showed good tolerance for the functional groups on these substrates. Not only halogen groups (3-Br, 4-Br, 5-Br, 3-Cl, 4-Cl, 5-Cl, 4-F, 5-F, 3,5-2F, and 3,5-2Cl) and electron-donating substituents (3-CH3, 4-CH3, and 5-CH3) but also strongly electron-withdrawing (4-NO2, 4-CF3) groups could be tolerated under the optimized conditions, so that the reaction proceeded smoothly.
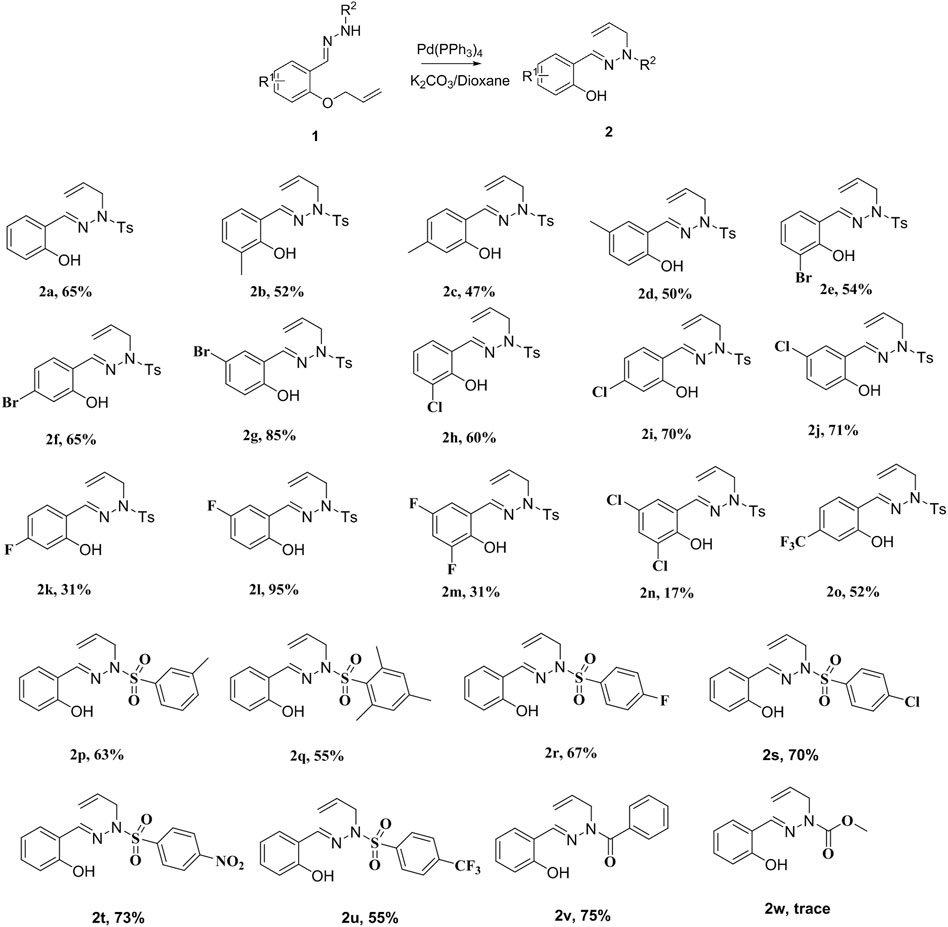
SCHEME 2. Scope of the reaction of N-sulfonylhydrazone. Reaction conditions: 1a (0.25 mmol), catalyst (5 mol%), base (0.50 mmol), and solvent at 80°C for 10 h under N2.
Halogen groups substituted at various positions on the benzene ring had different effects on the reaction. For example, halogen substitution at the 5-position of the benzene ring gave a higher yield (2k–2l) than did substitution at the 3- and 4-positions. In particular, 5-F substitution in the benzene ring generated the target compound in 95% isolated yield (2l). However, the reaction yields were significantly lower when double halogen substitution was present on the phenyl ring (2m, 2n). Moreover, the target compound (2o) was obtained smoothly when the substrate was charged with strong electron-withdrawing group (4-CF3), with yields of 52%.
Subsequently, we focused our attention on the effect of different substituted sulfonylhydrazones on the reaction yields (Scheme 2). The results showed that this method has wide applicability (2p–2w). Electron-withdrawing groups increased the yield of the reaction, while electron-donating groups decreased the yield. For example, the yields obtained with halogen substitution were higher than those observed with methyl substitution (2p and 2q vs. 2r and 2s). Encouragingly, even with strong electron-withdrawing group substitution, the corresponding target compounds were furnished smoothly (2t, 2u). The reaction also proceeded smoothly when the p-toluenesulfonyl group was replaced by the benzoyl group (2v), giving the target product in 75% yield. Unfortunately, the reaction did not proceed smoothly when the p-toluenesulfonate group was displaced by the methyl formate group (2w).
The reaction catalyzed by Pd(0) afforded sulfonylhydrazones, mainly the trans-isomer. The structure of 2a was confirmed by X-ray single-crystal diffraction analysis, and the chemical structures of other examples were obtained by analogy (Figure 1, see Supplementary Material for details). Based on the above results, we performed a scale-up experiment to extend the adaptability of the reaction. When 7.0 mmol of 1a was reacted under palladium catalysis, the corresponding product 2a was obtained in 60% yield (Scheme 3).
Subsequently, the reaction mechanism was investigated. Upon introducing free radical inhibitors (TEMPO or BHT) into the system, the reaction proceeded smoothly to afford the corresponding products (Scheme 4, Eqs. 1 and 2). This result suggested that the reaction did not involve a free radical mechanism. Unfortunately, the reaction did not proceed smoothly when the allyl group was replaced by a 2-methylallyl group (Scheme 4, Eq. 3). When 1a was substituted by substrate 1b′, 2b′ was not obtained under standard conditions, but the compound 3 was afforded, indicating that the terminal double bond with substituent was easily removed in the reaction (Scheme 4, Eq. 4). When 1a was replaced by substrate 2a′, the target compound 2a could not be obtained under the standard conditions (Scheme 4, Eq. 5). In contrast, if N′-benzylidene-4-methylbenzenesulfonyl hydrazide was added to the reaction system, 2a and 3a were produced (Scheme 4, Eq. 6).
Based on these results and the literature reports, we propose a plausible reaction mechanism (Tang et al., 2021; He et al., 2019; Nakamura et al., 2007; Butt and Zhang, 2015; Huo et al., 2014; Nakamura et al., 2008; Liu et al., 2018; Ma and Jiao, 2002; Yamamoto and Radhak-rishnan, 1999; Sieber and Morken, 2006; Bates and Satcharoen, 2002; Hashmi et al., 2013; Kolundzič et al., 2014) (Scheme 5). Initially, 1 is added to Pd(0) via oxidation, followed by exchange with the ligand of 1 to give π-allylpalladium species B. Then, B undergoes reductive elimination to afford intermediate C, which reacts with Pd(0) to form intermediate D. Since there is no β-H atom, D is exchanged with molecule 1 to produce B and simultaneously generates the final product 2. In addition, we also propose a possible reaction mechanism when the reaction substrate is 1b′. Oxidative addition of 1b′ to Pd(0), isomerization and subsequent β-H elimination generate Pd-H species F. Reductive elimination of intermediate F to afford product 3 and release Pd(0) for the next cycle (Scheme 5).
Conclusion
In conclusion, we report the palladium-catalyzed rearrangement of N-tosylhydrazones bearing allyl ethers to generate trans-olefin-substituted sulfonylhydrazones. We also investigated the applicability of the reaction to furnish the corresponding products, regardless of the presence of strongly electron-donating or electron-withdrawing substituents. The reaction involves the breakage of C-O bonds and the formation of C-N bonds, which forms the basis for the study of rearrangement reactions. Further investigation into the application of this reaction is ongoing in our laboratory.
Data Availability Statement
The datasets presented in this study can be found in online repositories. The names of the repository/repositories and accession number(s) can be found in the article/Supplementary Material.
Author Contributions
YiL and JH were responsible for designing the experiments. YC, JF, and RD performed the experimentations. YuL and JH analyzed the results and wrote the publication.
Funding
The authors thank the National Natural Science Foundation of China (No. 81903469) and Science and Technology Development Project of Weifang (No. 2019GX029) and which was supported by the Public Domestic Visiting Program of Weifang Medical University.
Conflict of Interest
The authors declare that the research was conducted in the absence of any commercial or financial relationships that could be construed as a potential conflict of interest.
Publisher’s Note
All claims expressed in this article are solely those of the authors and do not necessarily represent those of their affiliated organizations, or those of the publisher, the editors and the reviewers. Any product that may be evaluated in this article, or claim that may be made by its manufacturer, is not guaranteed or endorsed by the publisher.
Supplementary Material
The Supplementary Material for this article can be found online at: https://www.frontiersin.org/articles/10.3389/fchem.2021.782641/full#supplementary-material
References
Bates, R. W., and Satcharoen, V. (2002). Nucleophilic transition metal based cyclization of allenes. Chem. Soc. Rev. 31, 12–21. doi:10.1039/B103904K
Belkheiri, N., Bouguerne, B., Bedos-Belval, F., Duran, H., Bernis, C., Salvayre, R., et al. (2010). Synthesis and antioxidant activity evaluation of a syringic hydrazones family. Eur. J. Med. Chem. 45, 3019–3026. doi:10.1016/j.ejmech.2010.03.031
Butt, N. A., and Zhang, W. (2015). Transition Metal-Catalyzed Allylic Substitution Reactions with Unactivated Allylic Substrates. Chem. Soc. Rev. 44, 7929–7967. doi:10.1039/c5cs00144g
Enquist Jr, J. A., and Stoltz, B. M. (2008). The total synthesis of (-)-cyanthiwigin F by means of double catalytic enantioselective alkylation. Nature 453, 1228–1231. doi:10.1038/nature07046
Hashmi, A. S. K., Graf, K., Ackermann, M., and Rominger, F. (2013). Gold(I)-Catalyzed Domino Reaction of Allyl 2-en-4-ynyl Ethers to 1,3,6-Trien-4-yl Ketones. ChemCatChem 5, 1200–1204. doi:10.1002/cctc.201200787
He, Y., Yuan, C., Jiang, Z., Shuai, L., and Xiao, Q. (2019). Expeditious Synthesis of Isoquinolone Derivatives by Rhodium(I)-Catalyzed Annulation Reaction through C-C Bond Cleavage. Org. Lett. 21, 185–189. doi:10.1021/acs.orglett.8b03653
Hossain, M. L., Ye, F., Zhang, Y., Wang, J., et al. (2013). CuI-Catalyzed Cross-Coupling of N-Tosylhydrazones with Terminal Alkynes: Synthesis of 1,3-Disubstituted Allenes. J. Org. Chem. 78, 1236–1241. doi:10.1021/jo3024686
Huo, X., Quan, M., Yang, G., Zhao, X., Liu, D., Liu, Y., et al. (2014). Hydrogen-Bond-Activated Palladium-Catalyzed Allylic Alkylation via Allylic Alkyl Ethers: Challenging Leaving Groups. Org. Lett. 16, 1570–1573. doi:10.1021/ol5000988
Huters, A. D., Styduhar, E. D., and Garg, N. K. (2012). Total Syntheses of the Elusive Welwitindolinones with Bicyclo[4.3.1] Cores. Angew. Chem. Int. Ed. 51, 3758–3765. doi:10.1002/anie.201107567
Khan, S. A. (2008). Synthesis, characterization and in vitro antibacterial activity of new steroidal 5-en-3-oxazolo and thiazoloquinoxaline. Eur. J. Med. Chem. 43, 2040–2044. doi:10.1016/j.ejmech.2007.12.008
Kolundzič, F., Murali, A., Galan, P. P., Bauer, J. O., Strohmann, C., Kumar, K., et al. (2014). A Cyclization–Rearrangement Cascade for the Synthesis of Structurally Complex Chiral Gold(I)–Aminocarbene Complexes. Angew. Chem. Int. Ed. 53, 8122–8126. doi:10.1002/anie.201403651
Korawat, H. S., and Basak, A. K. (2020). K2CO3-Catalyzed Rapid Conversion of N-Sulfonylhydrazones to Sulfinates. ACS Omega 5 (28), 17818–17827. doi:10.1021/acsomega.0c02616
Liu, T., Sun, X., and Wu, L. (2018). Palladium-Catalyzed Cascade C−O Cleavage and C−H Alkenylation of Phosphinyl Allenes: An Expeditious Approach to 3-Alkenyl Benzo[b ]phosphole Oxides. Adv. Synth. Catal. 360, 2005–2012. doi:10.1002/adsc.201800103
Lu, Z., and Ma, S. (2008). Metal-Catalyzed Enantioselective Allylation in Asymmetric Synthesis. Angew. Chem. Int. Ed. 47, 258–297. doi:10.1002/anie.200605113
Ma, S., and Jiao, N. (2002). Pd0-Catalyzed Three-Component Tandem Double-Addition-Cyclization Reaction: Stereoselective Synthesis ofcis-Pyrrolidine Derivatives. Angew. Chem. Int. Ed. 41, 4737–4740. doi:10.1002/anie.200290033
Mohr, J. T., and Stoltz, B. M. (2007). Enantioselective Tsuji Allylations. Chem. Asian J. 2, 1476–1491. doi:10.1002/asia.200700183
Nakamura, I., Sato, T., Terada, M., and Yamamoto, Y. (2008). Chirality Transfer in Gold-Catalyzed Carbothiolation of o-Alkynylphenyl 1-Arylethyl Sulfides. Org. Lett. 10, 2649–2651. doi:10.1021/ol8007556
Nakamura, I., Yamagishi, U., Song, D., Konta, S., and Yamamoto, Y. (2007). Gold- and Indium-Catalyzed Synthesis of 3- and 6-Sulfonylindoles fromortho-Alkynyl-N-sulfonylanilines. Angew. Chem. Int. Ed. 46, 2284–2287. doi:10.1002/anie.200604038
Özbek, N., Kavak, G., Özcan, Y., İde, S., and Karacan, N. (2009). Structure, antibacterial activity and theoretical study of 2-hydroxy-1-naphthaldehyde-N-methylethanesulfonylhydrazone. J. Mol. Struct. 919, 154–159. doi:10.1016/j.molstruc.2008.09.010
Özdemir, Ü. Ö., Arslan, F., and Hamurcu, F. (2010). Synthesis, characterization, antibacterial activities and carbonic anhydrase enzyme inhibitor effects of new arylsulfonylhydrazone and their Ni(II), Co(II) complexes. Spectrochimica Acta A: Mol. Biomol. Spectrosc. 75, 121–126. doi:10.1016/j.saa.2009.09.052
Özdemir, Ü. Ö., Güvenç, P., Şahin, E., and Hamurcu, F. (2009). Synthesis, characterization and antibacterial activity of new sulfonamide derivatives and their nickel(II), cobalt(II) complexes(II), cobalt(II) complexes. Inorg. Chim. Acta 362, 2613–2618. doi:10.1016/j.ica.2008.11.029
Özkay, Y., Tunalı, Y., Karaca, H., and Işıkdağ, İ. (2010). Antimicrobial activity and a SAR study of some novel benzimidazole derivatives bearing hydrazone moiety. Eur. J. Med. Chem. 45, 3293–3298. doi:10.1016/j.ejmech.2010.04.012
Shimizu, T., Hayashi, Y., Nagano, Y., and Teramura, K. (1980). Intramolecular Cycloaddition Reactions ofN-Sulfonyl Nitrile Imides Bearing Alkenyl Groups. Bcsj 53 (2), 429–434. doi:10.1246/bcsj.53.429
Sieber, J. D., and Morken, J. P. (2006). Sequential Pd-Catalyzed Asymmetric Allene Diboration/α-Aminoallylation. J. Am. Chem. Soc. 128, 74–75. doi:10.1021/ja057020r
Tang, T.-M., Liu, M., Wu, H., Gou, T., Hu, X., Wang, B.-Q., et al. (2021). Pd-Catalyzed tandem C-C/C-O/C-H single bond cleavage of 3-allyloxybenzocyclobutenols. Org. Chem. Front. 8, 3867–3875. doi:10.1039/d0qo01619e
Trost, B. M., and Crawley, M. L. (2003). Asymmetric Transition-Metal-Catalyzed Allylic Alkylations: Applications in Total Synthesis. Chem. Rev. 103, 2921–2944. doi:10.1021/cr020027w
Trost, B. M., Machacek, M. R., and Aponick, A. (2006). Predicting the Stereochemistry of Diphenylphosphino Benzoic Acid (DPPBA)-Based Palladium-Catalyzed Asymmetric Allylic Alkylation Reactions: A Working Model. Acc. Chem. Res. 39 (10), 747–760. doi:10.1021/ar040063c
Weaver, J. D., Recio, A., Grenning, A. J., and Tunge, J. A. (2011). Transition Metal-Catalyzed Decarboxylative Allylation and Benzylation Reactions. Chem. Rev. 111 (3), 1846–1913. doi:10.1021/cr1002744
Yamamoto, Y., and Radhakrishnan, U. (1999). Palladium catalysed pronucleophile addition to unactivated carbon-carbon multiple bonds. Chem. Soc. Rev. 28, 199–207. doi:10.1039/a806581k
Keywords: trans-structure, allyl ethers, sulfonylhydrazones, rearrangement, palladium
Citation: Chang Y, Fu J, Li Y, Ding R, Liu Y and Hu J (2021) Pd-Catalyzed Rearrangement Reaction of N-Tosylhydrazones Bearing Allyl Ethers Into Trans-Olefin-Substituted Sulfonylhydrazones. Front. Chem. 9:782641. doi: 10.3389/fchem.2021.782641
Received: 24 September 2021; Accepted: 11 October 2021;
Published: 25 October 2021.
Edited by:
Guigen Li, Texas Tech University, United StatesReviewed by:
Ying Xia, Sichuan University, ChinaJiaxi Xu, Beijing University of Chemical Technology, China
Copyright © 2021 Chang, Fu, Li, Ding, Liu and Hu. This is an open-access article distributed under the terms of the Creative Commons Attribution License (CC BY). The use, distribution or reproduction in other forums is permitted, provided the original author(s) and the copyright owner(s) are credited and that the original publication in this journal is cited, in accordance with accepted academic practice. No use, distribution or reproduction is permitted which does not comply with these terms.
*Correspondence: Yue Liu, bGl1eXVlQHdmbWMuZWR1LmNu; Jinxing Hu, amlueGluZ2h1MjAxM0B3Zm1jLmVkdS5jbg==