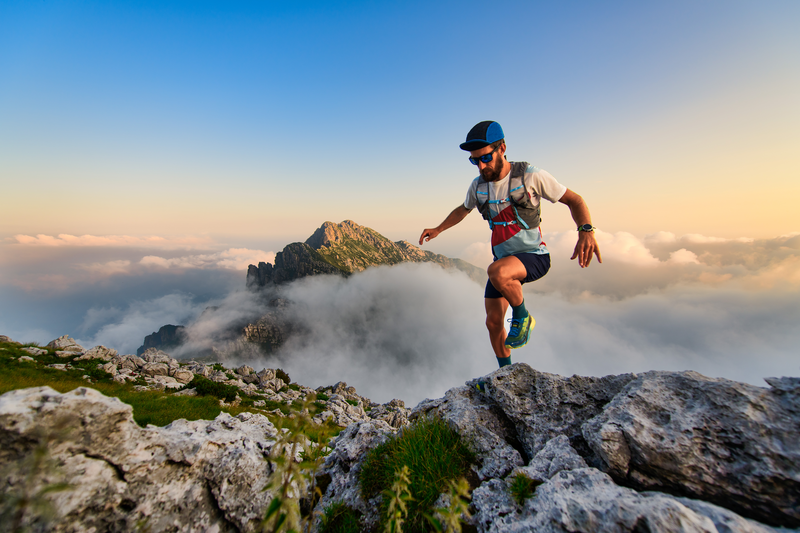
95% of researchers rate our articles as excellent or good
Learn more about the work of our research integrity team to safeguard the quality of each article we publish.
Find out more
ORIGINAL RESEARCH article
Front. Chem. , 17 January 2022
Sec. Analytical Chemistry
Volume 9 - 2021 | https://doi.org/10.3389/fchem.2021.782481
This article is part of the Research Topic Women in Analytical Chemistry View all 19 articles
Reported herein is a fluorometric and colorimetric sensor for the presence of trace amounts of water in organic solvents, using syn-bimane based boronate ester 1. This sensor responds to the presence of water with a highly sensitive turn-off fluorescence response, with detection limits as low as 0.018% water (v/v). Moreover, analogously high performance was observed when compound 1 was adsorbed on filter paper, with the paper-based sensor responding both to the presence of liquid water and to humid atmospheres. Reusability of the paper-based sensor up to 11 cycles was demonstrated, albeit with progressive decreases in the performance, and 1H NMR and mass spectrometry analyses were used to explain the observed, hydrolysis-based sensor response.
The detection of water in organic solvents is of significant interest from a variety of industrial, pharmaceutical, and chemical safety perspectives (Mishra and Singh 2021). From an industrial perspective, even minute quantities of water in organic solvents can rapidly contaminate chemical reactions, leading to deactivation of catalysts, a reduction in reaction efficiencies, and the generation of undesired side products (Lin et al., 2012). From a pharmaceutical perspective, the presence of water in organic solvents can dramatically affect the optimized syntheses of pharmaceutically active agents, with significant financial consequences particularly for large-scale syntheses (Thenrajan et al., 2021). From a chemical safety perspective, the incompatibility of a variety of molecules with water is well-known (Zor et al., 2021), and inadvertent exposure to water found as a contaminant in organic solvents can lead to the violent decomposition of water-incompatible reagents, resulting in chemical explosions and/or other undesired highly exothermic reactions (Yang et al., 2019).
Current methods for the detection of water in organic solvents tend to rely on spectral monitoring of the solvent to detect signals that correspond to the presence of water, and include the use of FTIR (Saleh and Tripp 2021), Raman (Yeung and Chan 2010), UV-visible (Mohar 2019), and fluorescence spectroscopy (Zhou et al., 2019; Kumar et al., 2016). Other methods, including gas chromatography (Kay et al., 2021) and electrochemical methods (Marecek and Samec 2020), have also been reported. These methods, which rely either on the detection of water directly or on the detection of signal changes corresponding to other molecules that interact with water, generally lead to extremely high sensitivity and selectivity (Wang et al., 2021). Nonetheless, ongoing challenges in using such methods include the need for costly and non-portable laboratory-grade instruments to monitor such spectral changes, as well as the fact that many of these methods are not reversible. Moreover, toxicity concerns remain around the use of water-detection materials that contain heavy metals, such as mercury and cadmium (Othong et al., 2020); around the use and responsible disposal of sensors composed of graphene oxide (Chi et al., 2021), carbon dots (Lee et al., 2019), and other carbon-based materials (Liu et al., 2020); and around sensors that rely on relatively weak noncovalent interactions in the sensor construction (Wu et al., 2020), and the potential for the degradation of such sensors to lead to environmental contamination (Gao et al., 2010).
Recent reports from one of our research groups have demonstrated the development of high-performance fluorometric (Haynes and Levine 2020) and colorimetric (Haynes et al., 2019) sensors for a variety of organic and inorganic analytes. Moreover, recent successes in novel sensor development have been reported in joint publications from our research groups, and demonstrate that bimane-based supramolecular constructs act as highly effective sensors for cobalt (II) ions (Pramanik et al., 2020) and for molecular iodine (Pramanik et al., 2021). These sensors, which operate in both solution-state and on filter papers to provide fluorometric and colorimetric analyte detection, have notable practical advantages, including their high sensitivity, ease of access, and non-toxicity of both the bimane transducing element and supramolecular cyclodextrin scaffold. The lack of toxicity of both cyclodextrin (Kfoury et al., 2019) and bimane (MSDS 38369) has been well-established in the literature, and has led to the use of both of these components in a variety of biologically-relevant applications (Barbosa et al., 2019; Lapidot et al., 2016; Lavis and Raines 2008). Moreover, an additional report from one of our research groups has demonstrated a streamlined approach to access bimane derivatives, which facilitates practically attainable access to a variety of these highly fluorescent structures (Szumski et al., 2021). This straightforward access, combined with our already demonstrated ability to use bimanes as components of effective sensors (Roy et al., 2018), prompted us to investigate the use of bimane derivatives as high impact fluorescent sensors for water contamination. Moreover, a recent theoretical report indicated that bimane is expected to demonstrate significant water-induced fluorescence quenching, although experimental evidence in support of these calculations was not provided (Maillard et al., 2021).
Reported herein are the results of our investigations, which demonstrate that bimane 1 responds to the presence of liquid water in organic solvents and water vapor in high humidity environments, and that such a response occurs both in solution of bimane 1 and on filter papers to which bimane 1 had been adsorbed, leading to colorimetric and fluorimetric changes. Although water sensing via a similar boronate hydrolysis mechanism has been demonstrated using other fluorophores (Selvaraj et al., 2019), it has not yet been demonstrated using the bimane scaffold and represents a significant step towards the establishment of bimanes as a viable option in the tool-box of available fluorophores (Kosower et al., 1979). Determining the linear relationship between the sensor response (either colorimetric or fluorometric) and the amount of water provides a method for its straightforward quantitation. Finally, good reversibility of the paper-based sensors was also demonstrated, as were detailed mechanistic investigations that explain the basis of the sensor response.
Compound 1 was synthesized from compounds 3 and 4. Bis(triphenylphosphine) palladium (II) chloride (20 mg, 0.028 mmol, 0.10 equiv) and cuprous iodide (2.7 mg, 0.014 mmol, 0.05 equiv) were added to a solution of 4-ethynylphenylboronic acid pinacol ester 4 (140 mg, 0.61 mmol, 2.2 equiv), diisopropylethylamine (0.48 ml, 2.8 mmol, 10 equiv), and compound 3 (150 mg, 0.28 mmol, 1.0 equiv) in CH3CN (200 ml). The mixture was stirred at 80 °C for 1 hour under a nitrogen atmosphere. After 1 hour, the solvent was evaporated under reduced pressure, and the resulting crude product was purified via flash chromatography over silica gel eluting with 5% ethyl acetate: 95% dichloromethane. The product was isolated as a reddish-yellow colored solid in 67% yield (138 mg). 1H NMR (CDCl3): 7.72−7.70 (d, J = 8 Hz, 2H, Ar-H), 7.36−7.34 (d, J = 8 Hz, 2H, Ar-H), 7.32-7.28 (m, 2H, Ar-H), 7.24-7.23 (d, J = 4 Hz, 1H, Ar-H), 7.17−7.13 (m, 2H, Ar-H), 1.33 [s, 12 H, 2(-Me)2] ppm; 13C NMR (CDCl3): 134.64, 131.24, 130.89, 129.31, 128.29, 84.15, 25.01 ppm; DEPTQ: 134.96, 131.20, 129.63, 128.61, 126.12, 25.32 ppm; HRMS m/z [M + H]+ calculated: 741.3322, found: 741.3332.
UV-Visible absorption spectroscopy was used in two different situations: 1) to study the solvent dependent properties of the bimane-based boronate compound 1; and 2) to investigate the detection of water using bimane-based boronate compound 1. Procedures for each of these situations are detailed below.
The effects of 13 different solvent systems were investigated by measuring the UV-visible absorption spectra of bimane 1 (from 200 to 700 nm) at a concentration of 10 µM in each of the solvent systems (see Supplementary Table S1).
The changes of the UV-visible absorbance spectrum of compound 1 upon the addition of varying concentrations of water was measured in a variety of water-miscible solvents. These experiments were conducted in HPLC grade solvents, with the concentration of compound 1 held constant at 10 μM, and with increasing concentrations of Milli-Q purified water added to the solution.
Fluorescence spectroscopy was used to investigate two different situations: 1) to study the solvent dependent fluorescence properties of compound 1; and 2) to investigate the detection of water using compound 1 in a variety of solvents. In all cases, the excitation of bimane 1 occurred at 450 nm, and the excitation and emission slit widths were both 5.0 nm. The procedures used in each of these situations are discussed in detail below:
The concentration of compound 1 was held constant at 10 µM in acetonitrile, acetonitrile-water (1:1 vol: vol), methanol, ethanol, tetrahydrofuran, ethyl acetate, dichloromethane, chloroform, acetone, diethyl ether, N,N-dimethylformamide (DMF), dimethylsulfoxide (DMSO), and water. The fluorescence emission of compound 1 in each solvent was recorded via excitation at 450 nm. Changes in the fluorescence emission of bimane 1 were quantified by integrating the fluorescence emission vs. wavenumber on the X-axis (using OriginPro 2020).
Increasing concentrations of water were added to solutions of compound 1 in organic, water-miscible solvents, with the concentration of bimane 1 held constant at 10 µM. The concentration of water in these experiments ranged from 0 to 43.16 µM. In all cases, changes in the fluorescence emission of compound 1 under these conditions were quantified by integrating the fluorescence emission vs. wavenumber on the X-axis using OriginPro 2020.
Whatman #1 filter papers with dimensions of 3.5 cm × 0.9 cm were coated with a solution of compound 1 by submerging the filter papers in an acetonitrile solution of compound 1 [(1) = 10 µM] for 60 min at room temperature. After 60 min, the papers were carefully removed from the solution using tweezers and were then placed on a Petri dish and allowed to dry for 3 hours in an open-air environment. After that, varying amounts of water (100, 150, 200, 250, 300, or 350 µL) were added via pipette to the top of the paper, and the paper was allowed to dry for 4 h at room temperature on a benchtop. The dried papers were then visualized under a long-wave, hand-held TLC lamp (365 nm excitation) and the results of these studies are reported herein.
The fluorescent sensor for water reported herein relies on bimane 1, which was synthesized via the Sonogashira coupling of diiodobimane 3 with two equivalents of acetylene 4 (Figure 1). This diidobimane was in turn accessed from 5-phenyl-4,4-dihydropyrazol-3-one 2 in a one-pot, three-step sequence in 68% overall yield, representing a marked improvement over multi-step, literature-reported methods that require the use of toxic chlorine gas and provide low overall yields (Kosower et al., 1982). The relative quantum yield of bimane 1 (0.17) and its extinction coefficient (1.23 × 104 L M−1 cm−1) are somewhat lower than values reported for other bimane structures (i.e., 0.83 for an unsymmetrically substituted bimane containing one chlorine substituent) (Szumski et al., 2021), but are in line with Kosower’s observation that electron-donating alkyl and aryl substituents on the syn-bimane structure lead to noticeable reductions in the quantum yield (Kosower et al., 1983).
Interestingly, compound 1 displayed mild solvatochromism in its steady-state photophysical measurements, with slight solvent-induced differences in the absorbance (Figure 2A) and emission (Figure 2B) spectra observed. These changes were markedly more pronounced in a fully aqueous solvent, with water leading to a strong decrease in the visible absorbance band of compound 1, and an even more pronounced decrease in the fluorescence emission. Interestingly, compound 1 in the solid-state also displayed a strong fluorescence emission, which decreased markedly upon the addition of water.
FIGURE 2. The (A) UV-visible and (B) fluorescence emission spectra of compound 1 in a variety of solvents, highlighting the marked difference in spectra observed in water (orange trace) compared to the organic solvents [(1) = 10 μM; λex = 450 nm].
This water-induced quenching displayed a strong solvent-dependent response (Figure 3), with the addition of water to acetonitrile displaying the greatest degree of water-induced fluorescence quenching (Figure 3A, 53% decrease in the integrated fluorescence emission compared to the emission in the absence of water). Extremely high sensitivity for low concentrations of water was also demonstrated (Table 1), with the lowest detection limit for water of 0.018% (v/v) calculated in DMSO. This detection limit is in line with the limits of the most sensitive sensors for water in organic solvents reported to date (Huang et al., 2021), and indicates that the sensor reported herein represents a highly sensitive detection method (Kumar et al., 2021). Of note, all aqueous studies were conducted in double deionized water, so that no interference and/or fluorescence quenching occurred due to residual metal ions. Such ions, in particular palladium (Das et al., 2016), sodium (Roy et al., 2018), and cobalt (Pramanik et al., 2020), have been shown to complex effectively to the bimane core, leading to marked changes in the bimane’s photophysical properties. Also of note, the existence of trace amounts of water even in the “0%” water is likely, based on literature precedent (Son et al., 2001), but this trace amount of water is accounted for as part of the baseline for our fluorescence quenching experiments, in accordance with literature precedent (Porter and Markham 1970).
FIGURE 3. An illustration of the changes in the fluorescence emission of compound 1 that occurs in the absence (black line) or presence (red line) of water in water-miscible organic solvents: (A) Acetonitrile; (B) THF (C) DMF; and (D) DMSO. [(1) = 10 μM; λex = 450 nm].
TABLE 1. Limits of detection and quantification of water in organic, water-miscible solvents, calculated as the percentage of water by volume.a
Moreover, this highly sensitive fluorescence-based quenching response was accompanied by noticeable changes in the color of bimane 1 solutions in varying solvents with increasing percentages of water, which were particularly visible using 365 nm excitation of the bimane 1 solutions. Examples of such dramatic color changes are shown for water added to acetonitrile, THF, and DMSO (Figure 4, top). The quantitative blue values changed as a result of the addition of increasing percentages of water in a manner that was linear for a broad range of water percentages (Figure 4, bottom, Table 2); such linearity, in turn, directly enables the ability to detect unknown water concentrations within such solvent systems. Finally, general applicability for the system was also demonstrated, with both purified milli-Q water and aqueous buffer solutions between pH 5 and pH 7.5 inducing identical colorimetric responses (see ESI for more details).
FIGURE 4. Top: Naked eye color changes induced by the addition of increasing percentages of water to water-miscible solvents. Bottom: The linear relationship between the added water percentage and the quantitative blue value of the solution, measured from photographs taken under 365 nm excitation. (A) Acetonitrile solutions; (B) DMF solutions; and (C) DMSO solutions (other solvents are shown in the ESI; blue bars represent the calculated standard deviations for each data point).
TABLE 2. The linear relationship between the percentage of water added to solutions of bimane 1 in water-miscible solvents and the quantitative blue value of the resulting solution when imaged under 365 nm excitation.a
The mechanism by which the addition of water induces the fluorescence quenching of bimane 1 is likely via the water-induced hydrolysis of the boronate ester moieties on compound 1 to form the hydrolyzed boronic acid 5 (Figure 5). The formation of compound 5 was confirmed by 1H NMR titrations and high-resolution mass spectrometry (see ESI for more details). Moreover, an analogous hydrolysis reaction occurred in methanol, resulting in the formation of methyl ester-substituted boronate moieties in lieu of the pinacol boronates. The fact that changing the hybridization of boron-containing moieties from tetrahedral to trigonal planar induces such marked fluorescence decreases has been previously reported in the literature (Samaniego Lopez et al., 2015; Williams et al., 2021), and is fully consistent with the results reported herein. Notably, this fluorescence quenching occurs rapidly (within seconds), and remains stable (with less than 5% additional quenching) up to 20 min after the initial contact between compound 1 and water.
FIGURE 5. The hydrolysis of boronate ester 1 to boronic acid 5, which likely is responsible for water-induced fluorescence decreases.
Gratifyingly, moving from solution-state water sensing to sensing on a solid-support resulted in analogous water-induced photophysical changes, with the addition of water to filter paper onto which bimane 1 had been adsorbed causing a marked change in the color and fluorescence of the system (Figure 6A). Notably, the procedure by which water is added to these paper sensors (dropping via pipette onto the functionalized paper) is done by design to ensure that we control the amount of water that the paper contacts, but we recognize that such a procedure will require further optimization before real-world device development and deployment. These changes were quantified by RGB color analysis (Figure 6B), which shows that the system becomes rapidly saturated with water, resulting in minimal changes in the RGB values after 100 µL of water were added. Even more significantly, bimane 1-functionalized filter papers responded to the presence of water vapor with an analogously strong sensor response, with exposure of the functionalized paper to a high humidity chamber (99% relative humidity) resulting in analogous changes in the sensor’s photophysical (colorimetric and fluorometric) profile (Figure 6C).
FIGURE 6. (A) Photograph of bimane 1-functionalized filter paper after the addition of water via micropipette (left-to-right: 0, 100, 150, 200, 250, 300, and 350 µL) (B) Quantitative RGB values of the bimane 1-functionalized filter paper as a function of the addition of increasing amounts of water (calculated via ImageJ analysis); (C) Photographs of bimane 1-functionalized filter paper under low humidity and high humidity conditions, imaged under 365 nm excitation.
Finally, the bimane 1-functionalized filter papers demonstrated strong reversibility in the sensing of water in both the liquid and vapor phase. Liquid water, which induced noticeable photophysical changes of the bimane 1-functionalized paper (see Figure 6A, above), was effectively removed by drying the papers under ambient conditions followed by brief heating (80 °C for 10 min). This drying allowed for the introduction of water again to the paper, followed by re-drying of the paper under the aforementioned conditions. Overall, 11 cycles of sensing followed by drying were demonstrated, albeit with progressive decreases in the performance observed. This reusability was visible by naked eye detection (Figure 7A), and could also be quantified by measuring the saturation values for each of the papers (Figure 7B). Similarly, the reusability of bimane 1-functionalized papers as humidity sensors was also demonstrated, with 7 cycles of exposure to 99% relative humidity, followed by drying and reuse of the same sensor with comparable performance results (Figure 7C). Of note, at this stage we cannot say with full certainty that in the dry state the original bimane 1 is regenerated. Reaction of bimane 5 with free OH groups in the surface of the paper would also lead to fluorescent bimane based boronate esters, which means that the observed partial reversibility may be a result of the generation of new fluorescent bimane-based boronate esters on the paper support.
FIGURE 7. An illustration of the regeneration and reusability of bimane 1-functionalized filter papers: (A) The detection of liquid water droplets over 11 cycles of exposure followed by drying; (B) Quantitative green values of the papers plotted as a function of cycle number, with each cycle representing an exposure to water followed by drying; and (C) Reusability of the paper sensors for the detection of humidity through exposing the papers to 99% relative humidity, followed by drying under ambient humidity conditions and re-exposure.
The development of methods for the rapid, sensitive, and generally applicable detection of water represents a high priority research area due to its significant applications in chemical safety and industry. The work reported herein represents an important step towards addressing this issue, by reporting that a boronate ester-functionalized bimane, compound 1, undergoes rapid and sensitive fluorescence quenching in the presence of trace quantities of water, both in solution and in the vapor phase. The successful access to this structure due to recent advances in synthetic methodology for syn-bimanes represents an additional important advance, and the reusability of paper-based sensors with compound 1 highlights the strong practical applicability of this system. Future work will be directed towards demonstrating the broad applicability of this sensor in real-world samples, and results of these and other investigations will be reported in due course.
The original contributions presented in the study are included in the article/Supplementary Material and further inquiries can be directed to the corresponding authors.
JK optimized the synthesis of the bimane boronate ester, conducted detailed analyses of the intermediates and final products along the synthetic pathway, and did fluorescence analytical work. AP optimized the sensing component of the work reported herein, including the work of the sensor in solution and on paper, using both fluorescence and colorimetric analysis. ML and FG assisted in the design of experiments and the interpretation of results. All authors contributed to the writing of the manuscript, with ML and FG providing final edits as needed. All authors give approval to the submission of this work, and all authors agree to be accountable for the work reported herein.
Ariel University is acknowledged for providing start-up funding to ML, FG and a postdoctoral research fellowship for AP. FG is the incumbent of the Cosman endowment for organic chemistry research.
The authors declare that the research was conducted in the absence of any commercial or financial relationships that could be construed as a potential conflict of interest.
All claims expressed in this article are solely those of the authors and do not necessarily represent those of their affiliated organizations, or those of the publisher, the editors, and the reviewers. Any product that may be evaluated in this article, or claim that may be made by its manufacturer, is not guaranteed or endorsed by the publisher.
The authors gratefully acknowledge Dr. Vered Marks for her assistance with 1H NMR titration experiments and Dr. Itay Pitussi for his assistance with high resolution mass spectrometry. Dr. Rami Krieger is thanked for his role in maintaining all departmental instruments used for these experiments.
The Supplementary Material for this article can be found online at: https://www.frontiersin.org/articles/10.3389/fchem.2021.782481/full#supplementary-material
Barbosa, P. F. P., Cumba, L. R., Andrade, R. D. A., and do Carmo, D. R. (2019). Chemical Modifications of Cyclodextrin and Chitosan for Biological and Environmental Applications: Metals and Organic Pollutants Adsorption and Removal. J. Polym. Environ. 27, 1352–1366. doi:10.1007/s10924-019-01434-x
Chi, H., Ze, L. J., Zhou, X., and Wang, F. (2021). GO Film on Flexible Substrate: An Approach to Wearable Colorimetric Humidity Sensor. Dyes Pigm. 185, 108916. doi:10.1016/j.dyepig.2020.108916
Das, P. J., Diskin-Posner, Y., Firer, M., Montag, M., and Grynszpan, F. (2016). syn-Bimane as a Chelating O-Donor Ligand for Palladium(II). Dalton Trans. 45, 17123–17131. doi:10.1039/C6DT02141G
Gao, Q., Xiu, Y., Li, G.-D., and Chen, J.-S. (2010). Sensor Material Based on Occluded Trisulfur Anionic Radicals for Convenient Detection of Trace Amounts of Water Molecules. J. Mater. Chem. 20, 3307–3312. doi:10.1039/b925233a
Haynes, A., Halpert, P., and Levine, M. (2019). Colorimetric Detection of Aliphatic Alcohols in β-Cyclodextrin Solutions. ACS Omega 4, 18361–18369. doi:10.1021/acsomega.9b02612
Haynes, A. Z., and Levine, M. (2020). Detection of Anabolic Steroids via cyclodextrin-Promoted Fluorescence Modulation. RSC Adv. 10, 25108–25115. doi:10.1039/d0ra03485a
Huang, J., Liang, Y., Liu, H.-B., Zhang, X., and Wang, J. (2021). N-Hydroxypropyl Substituted 4-Hydroxynaphthalimide: Differentiation of Solvents and Discriminative Determination of Water in Organic Solvents. Spectrochimica Acta A: Mol. Biomol. Spectrosc. 253, 119559. doi:10.1016/j.saa.2021.119559
Kay, J., Thomas, R., Gruenhagen, J., and Venkatramani, C. J. (2021). Simultaneous Quantitation of Water and Residual Solvents in Pharmaceuticals by Rapid Headspace Gas Chromatography with thermal Conductivity Detection (GC-TCD). J. Pharm. Biomed. Anal. 194, 113796. doi:10.1016/j.jpba.2020.113796
Kfoury, M., Auezova, L., Greige-Gerges, H., and Fourmentin, S. (2019). Encapsulation in Cyclodextrins to Widen the Applications of Essential Oils. Environ. Chem. Lett. 17, 129–143. doi:10.1007/s10311-018-0783-y
Kosower, E. M., Bernstein, J., Goldberg, I., Pazhenchevsky, B., and Goldstein, E. (1979). Configuration and Planarity of Fluorescent and Nonfluorescent 1,5-diazabicyclo[3.3.0]octadienediones (9,10-dioxabimanes) by X-ray Crystallography. J. Am. Chem. Soc. 101, 1620–1621. doi:10.1021/ja00500a055
Kosower, E. M., Faust, D., Ben-Shoshan, M., and Goldberg, I. (1982). Bimanes. 14. Synthesis and Properties of 4,6-bis(carbalkoxy)-1,5-diazabicyclo[3.3.0]octa-3,6-Diene-2,8-Diones [4,6-Bis(carbalkoxy)-9,10-Dioxa-Syn-Bimanes]. Preparation of the Parent Syn-Bimane, Syn-(hydrogen,hydrogen)bimane. J. Org. Chem. 47, 214–221. doi:10.1021/jo00341a007
Kosower, E. M., Kanety, H., and Dodiuk, H. (1983). Bimanes VIII: Photophysical Properties of Syn- and Anti-1,5-diazabicyclo[3.3.0] Octadienediones (9,10-dioxabimanes). J. Photochem. 21, 171–182. doi:10.1016/0047-2670(83)80020-3
Kumar, P., Ghosh, A., and Jose, D. A. (2021). Chemical Sensors for Water Detection in Organic Solvents and Their Applications. ChemistrySelect 6, 820–842. doi:10.1002/slct.202003920
Kumar, P., Kaushik, R., Ghosh, A., and Jose, D. A. (2016). Detection of Moisture by Fluorescent OFF-ON Sensor in Organic Solvents and Raw Food Products. Anal. Chem. 88, 11314–11318. doi:10.1021/acs.analchem.6b03949
Lapidot, I., Baranes, D., Pinhasov, A., Gellerman, G., Albeck, A., Grynszpan, F., et al. (2016). α¯-Aminoisobutyric Acid Leads a Fluorescent Syn-Bimane LASER Probe across the Blood-Brain Barrier. Mc 12, 48–53. doi:10.2174/1573406411666150518105010
Lavis, L. D., and Raines, R. T. (2008). Bright Ideas for Chemical Biology. ACS Chem. Biol. 3, 142–155. doi:10.1021/cb700248m
Lee, H. J., Jana, J., Thi Ngo, Y.-L., Wang, L. L., Chung, J. S., and Hur, S. H. (2019). The Effect of Solvent Polarity on Emission Properties of Carbon Dots and Their Uses in Colorimetric Sensors for Water and Humidity. Mater. Res. Bull. 119, 110564. doi:10.1016/j.materresbull.2019.110564
Lin, W., Cheng, H., Ming, J., Yu, Y., and Zhao, F. (2012). Deactivation of Ni/TiO2 Catalyst in the Hydrogenation of Nitrobenzene in Water and Improvement in its Stability by Coating a Layer of Hydrophobic Carbon. J. Catal. 291, 149–154. doi:10.1016/j.jcat.2012.04.020
Liu, X., Zhou, Z., Wang, T., Deng, P., and Yan, Y. (2020). Visual Monitoring of Trace Water in Organic Solvents Based on Ecofriendly B/r-CDs Ratiometric Fluorescence Test Paper. Talanta 216, 120958. doi:10.1016/j.talanta.2020.120958
Maillard, J., Klehs, K., Rumble, C., Vauthey, E., Heilemann, M., and Fürstenberg, A. (2021). Universal Quenching of Common Fluorescent Probes by Water and Alcohols. Chem. Sci. 12, 1352–1362. doi:10.1039/d0sc05431c
Mareček, V., and Samec, Z. (2020). Electrochemical Study of the Anomalous Salt Extraction from Water to a Polar Organic Solvent. J. Solid State. Electrochem. 24, 2173–2174. doi:10.1007/s10008-020-04656-5
Mishra, S., and Singh, A. K. (2021). Optical Sensors for Water and Humidity and Their Further Applications. Coord. Chem. Rev. 445, 214063. doi:10.1016/j.ccr.2021.214063
Mohar, M. (2019). A Metallogel Based on a Zwitterionic Spirocyclic Meisenheimer Complex: Sensing of Fluoride Ions in Water and Moisture Content in Organic Solvents. ChemistrySelect 4, 5308–5314. doi:10.1002/slct.201900939
Othong, J., Boonmak, J., Kielar, F., and Youngme, S. (2020). Dual Function Based on Switchable Colorimetric Luminescence for Water and Temperature Sensing in Two-Dimensional Metal-Organic Framework Nanosheets. ACS Appl. Mater. Inter. 12, 41776–41784. doi:10.1021/acsami.0c12014
Porter, L. J., and Markham, K. R. (1970). The Unsuitability of Ethanol as a Solvent for the Spectroscopic Detection of Functional Groups in Hydroxyflavones with Aluminium Chloride. Phytochemistry 9, 1363–1365. doi:10.1016/S0031-9422(00)85333-9
Pramanik, A., Amer, S., Grynszpan, F., and Levine, M. (2020). Highly Sensitive Detection of Cobalt through Fluorescence Changes in β-Cyclodextrin-Bimane Complexes. Chem. Commun. 56, 12126–12129. doi:10.1039/d0cc05812b
Pramanik, A., Karmakar, J., Grynzspan, F., and Levine, M. (2021). Facile Iodine Detection via Fluorescence Quenching of β‐Cyclodextrin:Bimane‐Ditriazole Inclusion Complexes. Isr. J. Chem. 61, 253–260. doi:10.1002/ijch.202000092
Roy, A., Das, P. J., Diskin-Posner, Y., Firer, M., Grynszpan, F., and Montag, M. (2016). Quenching of syn-Bimane Fluorescence by Na+ Complexation. New J. Chem. 42, 15541–15545. doi:10.1039/c8nj01945b
Saleh, S. H., and Tripp, C. P. (2021). Measurement of Water Concentration in Oils Using CaO Powder and Infrared Spectroscopy. Talanta 228, 122250. doi:10.1016/j.talanta.2021.122250
Samaniego Lopez, C., Lago Huvelle, M. A., Uhrig, M. L., Coluccio Leskow, F., and Spagnuolo, C. C. (2015). Recognition of Saccharides in the NIR Region with a Novel Fluorogenic Boronolectin: In Vitro and Live Cell Labeling. Chem. Commun. 51, 4895–4898. doi:10.1039/C4CC10425K
Sekharan, T. R., Katari, O., Ruhina Rahman, S. N., Pawde, D. M., Goswami, A., Chandira, R. M., et al. (2021). Neoteric Solvents for the Pharmaceutical Industry: An Update. Drug Discov. Today 26, 1702–1711. doi:10.1016/j.drudis.2021.03.005
Selvaraj, M., Rajalakshmi, K., Nam, Y.-S., Lee, Y., Kim, B. C., Pai, S. J., et al. (2019). Rapid-response and Highly Sensitive Boronate Derivative-Based Fluorescence Probe for Detecting H2O2 in Living Cells. J. Anal. Methods Chem. 2019, 5174764. doi:10.1155/2019/5174764
Son, H. S., Roh, J., Shin, S. K., Park, J. W., and Ku, J. K. (2001). Luminescence Spectroscopy of Eu3+ (Bis-Tris) Complexes in Anhydrous DMF [Bis-Tris = 2,2-Bis(hydroxymethyl)-2,2′,2″-Nitrilotriethanol]: Luminescence Quenching Rate Constants for the 5D0 State of Eu3+ by DMF and Polyalcoholic OH Groups. J. Chem. Soc. Dalton Trans., 1524–1528. doi:10.1039/b100566i
Szumski, O., Karmakar, J., and Grynszpan, F. (2021). Re-enter the syn-(Me,I)Bimane: A Gateway to Bimane Derivatives with Extended π-Systems. Synlett 32, 1141–1145. doi:10.1055/s-0040-1706045
Wang, Y., Zhou, Y., Xie, G., Li, J., Wang, Y., Liu, X., et al. (2021). Dual Resistance and Impedance Investigation: Ultrasensitive and Stable Humidity Detection of Molybdenum Disulfide Nanosheet-Polyethylene Oxide Hybrids. ACS Appl. Mater. Inter. 13, 25250–25259. doi:10.1021/acsami.1c02119
Williams, G. T., Kedge, J. L., and Fossey, J. S. (2021). Molecular Boronic Acid-Based Saccharide Sensors. ACS Sens. 6, 1508–1528. doi:10.1021/acssensors.1c00462
Wu, Y., Ji, J., Zhou, Y., Chen, Z., Liu, S., and Zhao, J. (2020). Ratiometric and Colorimetric Sensors for Highly Sensitive Detection of Water in Organic Solvents Based on Hydroxyl-Containing Polyimide-Fluoride Complexes. Analytica Chim. Acta 1108, 37–45. doi:10.1016/j.aca.2020.02.043
Yang, Q., Sheng, M., Henkelis, J. J., Tu, S., Wiensch, E., Zhang, H., et al. (2019). Explosion Hazards of Sodium Hydride in Dimethyl Sulfoxide, N,N-Dimethylformamide, and N,N-Dimethylacetamide. Org. Process. Res. Dev. 23, 2210–2217. doi:10.1021/acs.oprd.9b00276
Yeung, M. C., and Chan, C. K. (2010). Water Content and Phase Transitions in Particles of Inorganic and Organic Species and Their Mixtures Using Micro-Raman Spectroscopy. Aerosol Sci. Technology 44, 269–280. doi:10.1080/02786820903583786
Zhou, L., Liao, J. F., Huang, Z. G., Wei, J. H., Wang, X. D., Li, W. G., et al. (2019). A Highly Red‐Emissive Lead‐Free Indium‐Based Perovskite Single Crystal for Sensitive Water Detection. Angew. Chem. Int. Ed. 58, 5277–5281. doi:10.1002/anie.201814564
Keywords: bimane, fluorescence, water sensor, boronate ester, paper-based sensing
Citation: Pramanik A, Karmakar J, Grynszpan F and Levine M (2022) Highly Sensitive Water Detection Through Reversible Fluorescence Changes in a syn-Bimane Based Boronic Acid Derivative. Front. Chem. 9:782481. doi: 10.3389/fchem.2021.782481
Received: 24 September 2021; Accepted: 09 December 2021;
Published: 17 January 2022.
Edited by:
Ottavia Giuffrè, University of Messina, ItalyReviewed by:
Jinsong Han, China Pharmaceutical University, ChinaCopyright © 2022 Pramanik, Karmakar, Grynszpan and Levine. This is an open-access article distributed under the terms of the Creative Commons Attribution License (CC BY). The use, distribution or reproduction in other forums is permitted, provided the original author(s) and the copyright owner(s) are credited and that the original publication in this journal is cited, in accordance with accepted academic practice. No use, distribution or reproduction is permitted which does not comply with these terms.
*Correspondence: Flavio Grynszpan, ZmxhdmlvZ0BhcmllbC5hYy5pbA==; Mindy Levine, bWluZHlsQGFyaWVsLmFjLmls
†These authors have contributed equally to this work
Disclaimer: All claims expressed in this article are solely those of the authors and do not necessarily represent those of their affiliated organizations, or those of the publisher, the editors and the reviewers. Any product that may be evaluated in this article or claim that may be made by its manufacturer is not guaranteed or endorsed by the publisher.
Research integrity at Frontiers
Learn more about the work of our research integrity team to safeguard the quality of each article we publish.