- 1Hubei Key Laboratory of Wudang Local Chinese Medicine, School of Pharmaceutical Sciences, Hubei University of Medicine, Shiyan, China
- 2Institute of Biomedicine, Hubei University of Medicine, Shiyan, China
- 3The First Clinical College, Hubei University of Medicine, Shiyan, China
- 4Department of Chemical Biology and Pharmaceutical Engineering, School of Chemistry and Chemical Engineering, Anhui University of Technology, Shiyan, China
A simplefuro [2,3-d]pyrimidinone-based Schiff base FPS was synthesized via aza-Wittig reaction and structure elucidation was carried out by spectroscopic studies FT-IR, 1H NMR, and 13C NMR and mass spectrometry. FPS showed weak fluorescence emission in methanol and the selectivity of FPS to different metal ions (Mn2+, Ca2+, Fe2+, Fe3+, Mg2+, Al3+, Ba2+, Ag+, Co2+, Na+, K+, Cu2+, Zn2+, Pb2+, Bi3+) were studied by absorption and fluorescence titration. The results show that FPS has selective fluorescence sensing behavior for Zn2+ ions and the limit of detection (LOD) was calculated to be 1.19 × 10–8 mol/L. Moreover, FPS-Zn2+ acts as a metal based highly selective and sensitive new chemosensor for Cu2+ ions and the LOD was calculated to be 2.25 × 10–7 mol/L. In accordance with the results and theoretical calculations, we suspected that the binding mechanisms of FPS to Zn2+ and Cu2+ were assigned to be the cooperative interaction of Zn2+(Cu2+)-N.
Introduction
Metal ions have pivotal functions for the growth and development process of organisms, and it is of great significance to identify and monitor metal ions in the environment and in organisms (Domingo 1994; Schmidt et al., 2009; Garza-Lombo et al., 2018). Fluorescence analysis technology has received increased attention in view of its utility for selective recognition of metal ion owing to its high selectivity, low toxicity, real-time detection, convenient and simple operation, and relatively friendly environment (Ma et al., 2015; Chae et al., 2020; Nsanzamahoro et al., 2020). In the past few years, many researchers have been devoted to finding and developing of some site-specific small-molecule fluorescence probes for highly selective recognition of metal ions (Liang et al., 2017; Bae et al., 2018; Yang et al., 2019) and for analyzing different metal ions, which are widely distributed in organisms and environments worldwide (Wang et al., 2019; Kim et al., 2021; Klenner et al., 2021; Sannigrahi et al., 2021). Many diseases, such as Alzheimer’s disease (Isaev et al., 2020), neuron disease (Marchetti 2014), Parkinson’s disease (Park et al., 2015), ischemia (Yin et al., 2019), epilepsy, and certain types of cancer and so on (Hildebrand et al., 2015; Hershfinkel 2018; Wang et al., 2020), are caused by the excessive or insufficient intake of Zn2+ and Cu2+ ions.
Recently, we have focused on the synthesis of nitrogenous heterocyclic compounds via aza-Wittig reaction, attempting to apply and evaluate their biological activities (Hu et al., 2010; Hu et al., 2014; Wang et al., 2016a; Wang et al., 2016b; Li et al., 2016; Liu M.-G. et al., 2019; Liu N. et al., 2019; Gao et al., 2019; Liu et al., 2021). Herein, we designed a Schiff base bearing furopyrimidinone scaffold (FPS) synthesized from 2-hydroxy-benzaldehyde with furo [2,3-d]-pyrimidine-5-carbohydrazide (Scheme 1), and fluorescence analysis showed that FPS displayed highly selective recognition Zn2+ with no apparent interference from other metal ions in MeOH solution and its applications in cell imaging and detection Cu2+ ions.
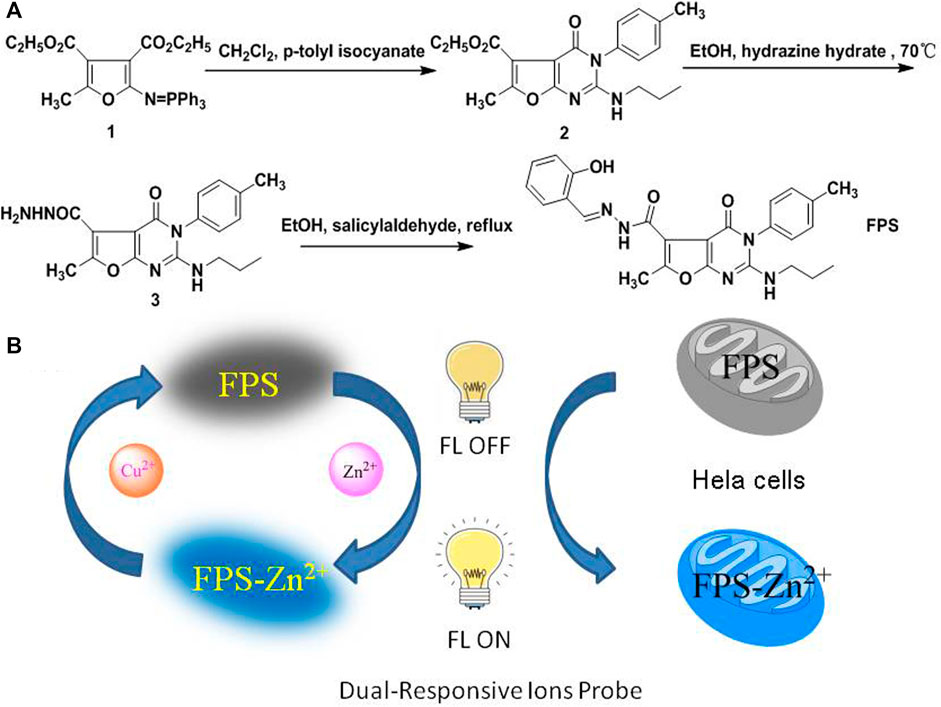
SCHEME 1. (A) The route of synthesis for FPS, (B) Dual-Response of FPS to Zn2+ and Cu2+, schematic of FPS applicated in cell imaging and detection Cu2+ ions.
Experimental Sections
Materials and General Methods
Unless otherwise stated, starting materials were commercially available and analytically pure, and the solvent was dried before use. The water used was redistilled water. The UV absorption and fluorescence emission spectra were recorded on a U-2550 Double-beam UV-Vis spectrophotometer (Japan) and a F-7000 fluorescence spectrometer (Japan), respectively. Melting points were recorded using an uncorrected X-4digital melting point apparatus. NMR were recorded on a Bruker Avance 400 MHz spectrometer (CDCl3 and DMSO-d6) with resonances relative to tetramethyl-silane (TMS) as an internal standard. Mass spectra (ESI) were recorded on a Waters XEVO G2-XSmass spectrometer. Fluorescence images of cells were analyzed by Dual photon confocal microscope (Olympus FV1000 MPE).
Synthesis
Synthesis of 2-Ethyl-3,4-dihydro-6-methyl-4-oxo-2-(propylamino)-3-p-tolyl-furo[2,3-d]pyrimidine-5-carboxylate 3
Preparation of ethyl-3,4-dihydro-6-methyl-4-oxo-2-(propylamino)-3-p-tolyl-furo-[2,3-d]pyrimidine-5-carboxylate 2. As described in methods previously (Hu et al., 2012). A mixture of 1 (2.5 g, 5 mmol) and p-tolyl isocyanate (5 mmol) in anhydrous methylene dichloride 24 h at 0–5°C under N2, and then n-propylamine (5.2 mmol) was added, after the mixture was stirred for 1 h at room temperature. The solution was removed under reduced pressure and anhydrous EtOH (10 ml) with five drops of EtONa (10%) in EtOH was added. The mixture was stirred for 4 h at room temperature. The precipitated solid was collected and washed with ethanol to give ethyl 3,4-dihydro-6-methyl-4-oxo-2-(propyl-amino)-3-p-tolyl-furo [2,3-d]pyrimidine-5-carboxylate 2, which was used directly without further purification. A solution of 2 (5 mmol) and hydrazine hydrate (1 ml, 80%) in EtOH were stirred at 60–65°C for 15 h, after the solution was concentrated under reduced pressure and the residue recrystallized from CH2Cl2/EtOH (v:v = 4:1, 20 ml) to give 3,4-dihydro-6-methyl-4-oxo-2-(propylamino)-3-p-tolyl-furo [2,3-d]pyrimidine-5-carbohydrazide 3, white solid, m. p.: 260–262°C; 1H NMR (400 MHz, DMSO-d6) δ: 0.8 (t, J = 8.0 Hz, 3H, CH3), 1.46–1.51 (m, 2H, CH2), 2.43 (s, 3H, CH3), 2.65 (s, 3H, CH3), 3.16–3.2 (m, 2H, NCH2), 4.44 (s, 2H, NH2), 6.45 (s, 1H, NH), 7.23–7.42 (m, 4H, ArH), 10.88 (s, 1H, NH); 13C NMR (100 MHz, DMSO-d6) δ: 165.45, 161.63, 160.70, 153.22, 153.00, 139.52, 132.11, 131.25, 129.24, 110.52, 93.38, 43.52, 22.11, 21.36, 13.48, 11.58; MS (70 eV) m/z (100%): Anal. calcd for C18H21N5O3 (M, 355.16), found [M + H+, 356.17].
Synthesis of N'-(2-hydroxybenzylidene)-3,4-dihydro-6-methyl-4-Oxo-2-(propylamino)-3-P-tolylfuro[2,3-d]pyrimidine-5-carbohydrazide FPS
A mixture of 3 (1.1 g, 3 mmol) and salicylaldehyde (3 mmol) in 25 ml ethanol was stirred for 10 h at 70–75°C, after the solution was concentrated under reduced pressure and the solid was collected and recrystallized from CH2Cl2/EtOH to give FPS (1.1 g, 84%). m. p.: 216–218°C. 1H NMR (400 MHz, DMSO-d6): δ = 13.55 (bs, 1H, ArOH), 8.35 (s, 1H, N = CH), 7.58–6.89 (m, 8H, Ar-H), 6.51 (bs, 1H, NH), 3.23 (q, J = 8.0, 2H, CH2), 2.72 (s, 3H, CH3), 2.45 (s, 3H, CH3), 0.82 (t, J = 8.0, 3H, CH3). 13C NMR (100 MHz, DMSO-d6): δ = 11.1, 13.3, 20.9, 21.6, 43.1, 92.6, 109.8, 116.3, 118.6, 119.3, 128.8, 129.7, 131.0, 131.4, 131.5, 139.3, 147.4, 152.9, 155.2, 157.4, 158.0, 160.7, 165.1. HRESI-MS m/z anal. calcd for C25H25N5O4 (M, 459.1985), found [M + H+, 460.1982].
Spectroscopic Study
FPS was formulated into 1.0 mmol/L solution in DMSO and then diluted to definite concentration with methanol before the spectral experiment. The salts used in standard stock solutions of metal ions were MnSO4, Ca(NO3)2, CuSO4, Al(NO3)3, Ba(NO3)2, AgNO3, CoCl2, ZnSO4, FeSO4, NaCl, KCl, Pb(NO3)2, MgSO4, Fe(NH4) (SO4)2 in distilled water to prepare 0.050 mol/L. The spectral changes of the mixed solutions of FPS with various metal ions were studied by UV-Vis absorption and fluorescence spectroscopy at room temperature. The fluorescence emission of FPS were recorded with excitation at 480 nm.
CCK8 Assay
The cytotoxicity of FPS was researched by CCK8 assay according to reported methods (Hou et al., 2019). Hela cells were cultured in DMEM medium containing 10% fetal bovine serum cell culture medium with 5% CO2 atmosphere at 37°C. The cells were transferred into 24-well plates and incubated for 24 h at 37°C. FPS, diluted to the desired concentrations (50–1,000 μmol/L) in culture medium, was added to the well. Then the original medium was removed after 24 h, and 10 μl of CCK-8 solution (5 mg/ml stock) was added to the Well and incubated for 2 h at 37°C. Absorbance at 450 nm was recorded with an enzyme-linked immunosorbent assay (ELISA) reader (Bio-Tek). The results showed that FPS exhibited low cytotoxicity against Hela cell lines with IC50 more than 500 μmol/L.
Result and Discussion
Fluorescence and UV-Vis Properties of FPS
FPS showed low fluorescence emission could be because of the photoinduced electron transfer (PET) process between the imine group and the benzene ring. To research the diverse solvent effect on FPS and FPS-Zn2+, the emission and excitation spectra of FPS and FPS-Zn2+ were recorded in different solvents [MeOH, EtOH, DMSO, and DMF (Figure 1)], respectively. These results showed that FPS and FPS-Zn2+have higher fluorescence enhancement in MeOH than the other solvents.
Selectivity of FPS
The fluorescence properties of FPS with various metal ions (10.0 equiv. of Na+, K+, Ba2+, Zn2+, Mn2+, Mg2+, Ca2+, Ag+, Co2+, Ni+, Fe3+, Cd2+, Pb2+, Cu2+, Al3+) were investigated, respectively. Results showed that Zn2+ caused significant fluorescent enhancement at 480 nm with the color change from colorless to the light blue. These phenomena indicated that FPS could be used as fluorescent sensors for Zn2+ recognition (Figure 2A). The ability was also explored of FPS to detect Zn2+ in the presence of other metal ions. A competitive test was carried out, in which 10 equivalent other metal ions were added to the solution of FPS and Zn2+ ion (Figure 2B), respectively. Figure 2B shows that the fluorescence quenching of FPS-Zn2+ undergoes a significant change with the addition of Cu2+ and Fe2+, and there is a little interference with the addition of Al3+, Fe3+, Co2+ and Ni2+ ions.
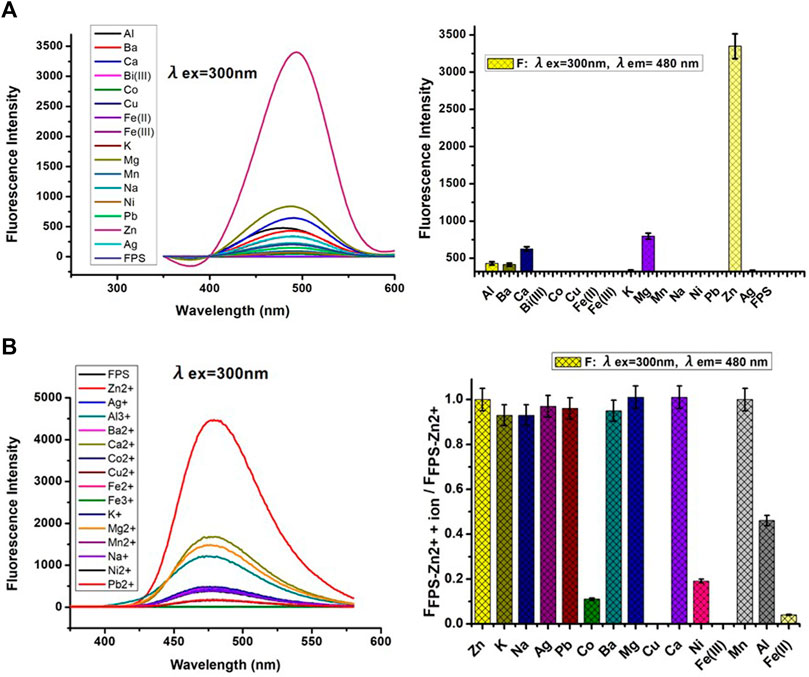
FIGURE 2. (A) Left: Fluorescent spectra of FPS (5 μmol/L) with various metal ions (10.0 equiv.) in methanol; Right: Dense bars indicate the fluorescence intensity (λex = 300nm, λem = 480 nm) (B) Left: Fluorescent spectra of FPS (5 μmol/L) + 10.0 equiv. Zn2+ with various metal ions (10.0 equiv.) in methanol; Right: Dense bar portion indicates the fluorescence intensity (λex = 300 nm, λem = 480 nm) in methanol solution, respectively.
Job’s Plot Measurements
Figure 3A is the Job’s plot of the fluorescence signal for FPS and Zn2+ solutions. The binding stoichiometry can be obtained from the plot. It revealed that a 1:1 binding was obtained between FPS and Zn2+ in methanol solution. Then the fluorescence characteristics of FPS to Zn2+ were further studied by fluorescence titration experiments (Figure 3B). The stability constant of FPS and Zn2+ was calculated to be 4.45×104 (r2 = 0.9626) from the nonlinear least squares fitting of the data, according to the Benesi-Hildebrand equation (Scheme 2). As shown in Figure 3B, with gradual addition of 0–20 μmol/L Zn2+ into the methanol solution of FPS (20.0 μmol/L), the fluorescence emission at 480 nm was increased gradually. Moreover, the detection limit of FPS to Zn2+ was calculated (LOD = 3σ/slope) to be 1.19 × 10–8 mol/L (r2 = 0.9959).
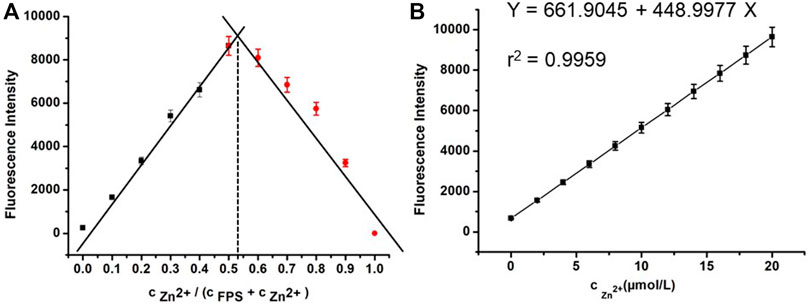
FIGURE 3. (A) Job’s plot for the stoichiometry determination of FPS and Zn2+ in the complexation and the fluorescence was performed as a function of the molar ratio [Zn2+]/([Zn2+] + [FPS]) (B) The fluorescence characteristics of FPS (20.0 μmol/L) with gradual addition of 0–20 μmol/L Zn2+ (λex = 300 nm, λem = 480 nm).
Concentration Effect of Cu2+and Fe2+ on Complex FPS-Zn2+
Based on the results in Figure 2B, to evaluate further the effect of Cu2+ and Fe2+ concentration on the probe FPS for recognition Zn2+, respectively, the fluorescence properties of complex FPS-Zn2+ were studied in methanol solution (Figure 4). For Cu2+ ion, at low concentrations (cCu2+ ≤ 5 μmol/L), the fluorescence emission was continuously quenched with the increase of Cu2+ and there was a good linear relationship. However, for Fe2+, there were no similar phenomena, the fluorescence emission at 480 nm was quenched completely, at that moment, with the addition of Fe2+ even in small amounts. Then to investigate the time-dependent of fluorescence quenching for Cu2+, as shown in Figure 4, the florescence intensity tended to be stable after adding the Cu2+ ion 15 seconds. In addition, the detection limit of complex FPS-Zn2+ to Cu2+ was calculated (LOD = 3σ/slope) to be 2.25 × 10−7 mol/L (r2 = 0.9987). These results show that a fluorescent probe composed of complex FPS-Zn2+ could be used as fluorescent sensors for Cu2+ detection real-time and for Fe2+ qualitative determination.
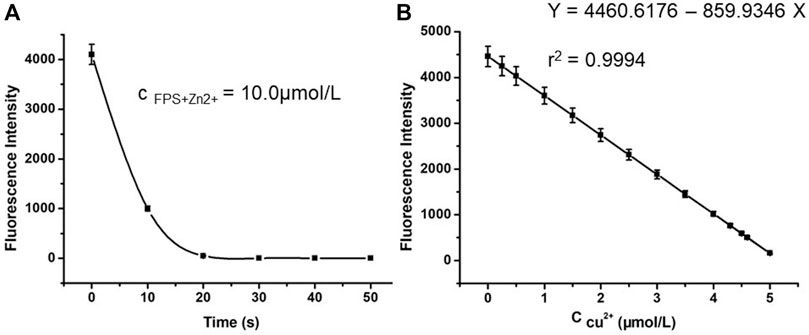
FIGURE 4. (A) The relationship between fluorescence intensity of 10.0 μmol/L FPS and the time (B) Fluorescence spectra (λex = 300 nm) of 10.0 μmol/L FPS and 10 μmol/L Zn2+ in the presence of Cu2+ ion with various concentrations (from 0 to 5.0 μmol/L) in methanol solution.
pH Tolerance of Complex FPS-Zn2+
As shown in Figure 5, the fluorescent spectra of FPS (5 μmol/L) + 1.0 equiv. Zn2+ with pH (1–12) in DMSO/H2O be studied, in the neutral solution, the fluorescence intensity of FPS + Zn2+ complex is strongest. At the same time, when the pH lower than five or higher than 10, the fluorescent of FPS + Zn2+ complex is quenching.
1H NMR Experiment
The binding ability of FPS with Zn2+ was evaluated using 1H NMR. As shown in Figure 6, when Zn2+ was added to FPS, the protons on the phenolic hydroxyl and amide of the FPS Ha and Hb were nearly despaired, respectively. This indicated that phenolic hydroxyl and amide coordinated to Zn2+ and the FPS-Zn2+ complex was formed.
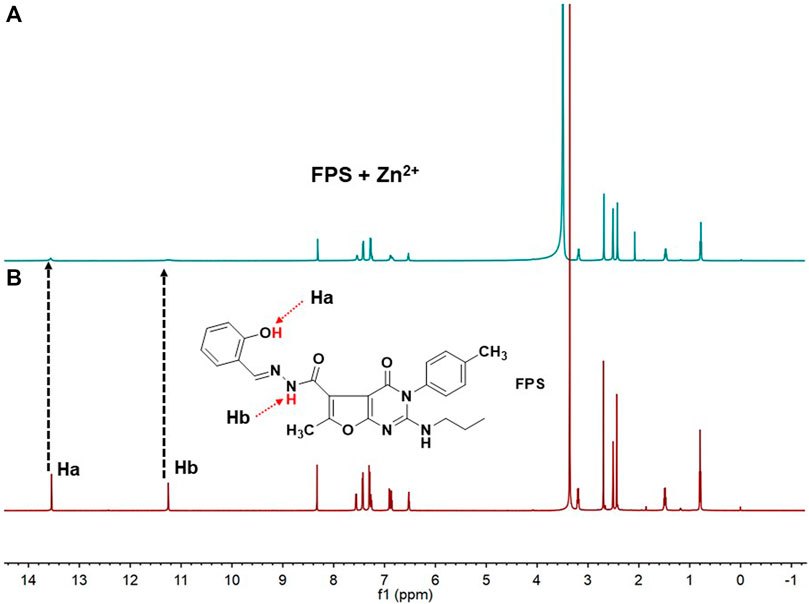
FIGURE 6. 1H NMR spectra (400 MHz, DMSO-d6, 298 K) of (A) FPS (6 mm) + Zn2+ (6 mm) (B) free FPS (6 mm). This indicated that phenolic hydroxyl and amide play important roles of FPS-Zn2+ complex.
Theoretical Calculations
Based on the experimental data and Job’s plot, to further elucidate the influence of the structure on the electronic properties, DFT calculations are performed for FPS, FPS-X (X = Zn2+, Fe2+, Cu2+). As shown in Figure 7, for complex FPS-X (X = Zn2+, Fe2+, Cu2+), the deprotonated O atom of phenolic hydroxyl, O atoms of carbonyl, imine and the O atom of methanol were coordinated with metal ions. The calculated distributions of molecular orbitals (HOMO, highest occupied molecular orbital; SOMO, single electron occupied molecular orbital; and LUMO, lowest unoccupied molecular orbital) are shown in Figure 7. The HOMO and LUMO of FPS are predominately determined by the phenol moiety, the bride including acyl hydrazine moiety, furan moiety, and sectionally listed in pyridine moiety, respectively. Once FPS coordinated to metal ions (Zn2+, Fe2+, Cu2+), the HOMO/SOMO and LUMO in FPS-X (X = Zn2+, Fe2+, Cu2+) were conversely localized on the pyridine moiety and metal ions one, respectively. Apparently, this phenomenon was attributed to the enlargement of the conjugated system due to the complexation of the FPS and metal ions. The lower energy gap between the HOMO and LUMO level of FPS-X (X = Zn2+, Fe2+, Cu2+) compared with 4.21 eV of FPS was in good agreement with the red shift of the experimental fluorescence spectra (Figure 2B). Furthermore, the calculational binding energy Ebind (Supplementary Table S1) of FPS-X (X = Zn2+,Fe2+, Cu2+) show that the minimal values of FPS-Zn2+ and the maximum values of FPS-Cu2+ which cause the greater complexation of Cu2+compared with Zn2+ and Fe2+, probably further leading to the fluorescence quenching of FPS-Cu2+.
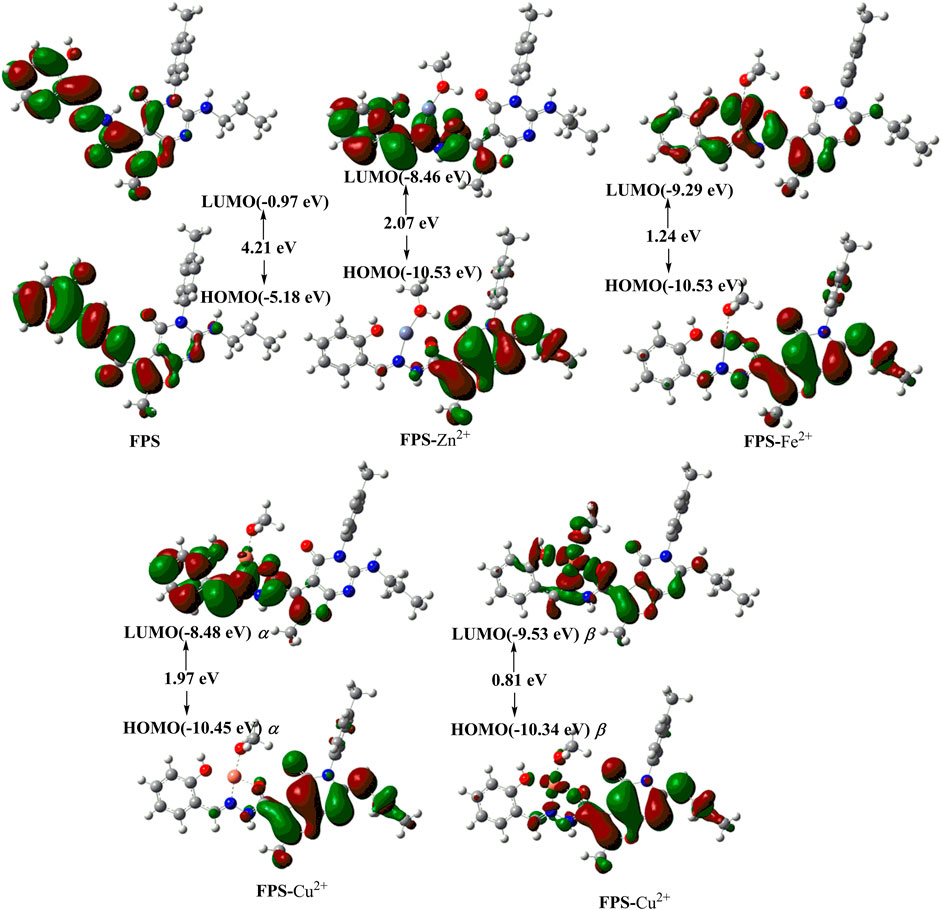
FIGURE 7. The optimized structures and Frontier molecular orbitals of FPS and FPS-X (X = Zn2+, Fe2+, Cu2+).
Fluorescence calculations of FPS-X (X = Zn2+, Fe2+, Cu2+) (Supplementary Tables S2–S4) was in good agreement with the experimental fluorescence data.
Fluorescence Imaging in Living Cells
Hela cells were cultured in DMEM medium containing 10% fetal bovine serum cell culture medium with 5% CO2 atmosphere at 37°C. The cells were transferred into 24-well plates and incubated for 24 h at 37°C. The first group Hela cells treated in a culture medium (DMSO: DMEM = 1: 99, v/v) alone were used as a control (Figure 8A). In group 2 and 3, Hela cells were cultured with probe FPS solution (5.0 μmol/L) for 25 min (Figure 8B). In groups 4 and 5, Hela cells were cultivated successively with probe FPS (5.0 μmol/L and 10.0 μmol/L) for 25 min, after being washed with PBS, and further incubated with Zn2+ (10.0 μmol/L and 20.0 μmol/L) for 5 min, respectively (Figure 8C).
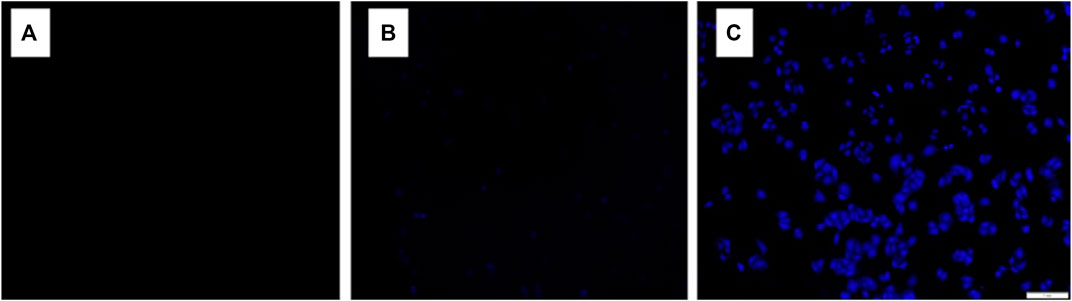
FIGURE 8. CLSM images of Hela cells treated under various conditions: (A) Hela cells were incubated with culture medium as a control; (B) Hela cells were cultured with probe FPS solution (5.0 μmol/L) for 25 min; (C) Hela cells were cultured with probe FPS solution (5.0 μmol/L) for 25 min, and after being washed with PBS and further incubated with Zn2+ (10.0 μmol/L) were then incubated for 5 min.

SCHEME 2. Benesi-Hildebrand equation. Where Fmax and Fmin are the fluorescence intensity of FPS in the presence and absence of zinc ions, respectively. F represents fluorescent intensities (at 480 nm) of FPS as a function of Zn2+ concentration. [FPS] = 20.0 μmol/L and [Zn2+] = 0–20 μmol/L.
Conclusion
This work was to synthesize and discover a specific “Dual-Response” to Zn2+ and Cu2+ probe based on a Schiff base bearing furopyrimidinone scaffold. The probe FPS with excellent linear relationship for the Zn2+ detection showed good and weak potential in imaging the exogenous and endogenous Zn2+, respectively, which can lead to the exploitation of growingly specific probes, particularly fluorescent for detection of Zn2+, Cu2+ and diagnosis of Zn2+, Cu2+ related diseases. In addition, the DFT calculations results showed how the structure affects the fluorescent behavior of FPS, which may help us to understand the essence of metal ions regulating effect in nature, and even give valuable reference to extend the real application of cell imaging (Li et al., 2021), imaging-guided (Sun et al., 2019), stimuli-responsive bioimaging (Min et al., 2021).
Data Availability Statement
The original contributions presented in the study are included in the article/Supplementary Material, further inquiries can be directed to the corresponding authors.
Author Contributions
YH: Conceptualization, Writing—original draft. CL, JL and JF: Living cells experiments. LG: Formal analysis, Investigation. XH: Project administration. JM: Conceptualization, Resources, Writing—review and editing. LL: Software, Methodology, Writing—review and editing.
Funding
This work was financially supported by the National Natural Science Foundation of China (No. 81773746), the Natural Science Foundation of Hubei Provincial Department of Education (No. D20192102), the Open Project of Hubei Key Laboratory of Wudang Local Chinese Medicine Research (Hubei University of Medicine) (No. WDCM2018001, WDCM2020006), the Hubei Provincial Innovation and Entrepreneurship Training Program for College Students (No. S201910929016).
Conflict of Interest
The authors declare that the research was conducted in the absence of any commercial or financial relationships that could be construed as a potential conflict of interest.
Publisher’s Note
All claims expressed in this article are solely those of the authors and do not necessarily represent those of their affiliated organizations, or those of the publisher, the editors and the reviewers. Any product that may be evaluated in this article, or claim that may be made by its manufacturer, is not guaranteed or endorsed by the publisher.
Supplementary Material
The Supplementary Material for this article can be found online at: https://www.frontiersin.org/articles/10.3389/fchem.2021.774090/full#supplementary-material
References
Bae, J.-E., Kim, I. J., and Nam, K. H. (2018). Spectroscopic Analysis of the Cu2+-Induced Fluorescence Quenching of Fluorescent Proteins Amcyan and Morange2. Mol. Biotechnol. 60, 485–491. doi:10.1007/s12033-018-0088-1
Chae, J. B., Lee, H., and Kim, C. (2020). Determination of Zinc Ion by a Quinoline-Based Fluorescence Chemosensor. J. Fluoresc. 30, 347–356. doi:10.1007/s10895-020-02501-6
Domingo, J. L. (1994). Metal‐induced Developmental Toxicity in Mammals: A Review. J. Toxicol. Environ. Health 42, 123–141. doi:10.1080/15287399409531868
Gao, H.-T., Wang, H.-M., Hou, N., Guo, X.-R., Zeng, X.-H., and Hu, Y.-G. (2019). Synthesis, Crystal Structure and Antitumor Activities of 2-Acyl-Beta-Lactam-2-Carboxamides. Chin. J. Struct. Chem. 38, 416–421.
Garza-Lombó, C., Posadas, Y., Quintanar, L., Gonsebatt, M. E., and Franco, R. (2018). Neurotoxicity Linked to Dysfunctional Metal Ion Homeostasis and Xenobiotic Metal Exposure: Redox Signaling and Oxidative Stress. Antioxid. Redox Signaling 28, 1669–1703. doi:10.1089/ars.2017.7272
Hershfinkel, M. (2018). The Zinc Sensing Receptor, Znr/Gpr39, in Health and Disease. Ijms 19, 439. doi:10.3390/ijms19020439
Hildebrand, M. S., Phillips, A. M., Mullen, S. A., Adlard, P. A., Hardies, K., Damiano, J. A., et al. (2015). Loss of Synaptic Zn2+ Transporter Function Increases Risk of Febrile Seizures. Sci. Rep. 5, 17816. doi:10.1038/srep17816
Hou, N., Man, J. H., Wang, X. Y., He, S. J., Li, Q., and Hu, Y. G. (2019). Efficient Synthesis and Biological Evaluation of 2,4‐Diaminothieno[2,3‐ D ]pyrimidine Derivative. ChemistrySelect 4, 4901–4904. doi:10.1002/slct.201900123
Hu, Y.-G., Wang, Y., Du, S.-M., Chen, X.-B., and Ding, M.-W. (2010). Efficient Synthesis and Biological Evaluation of Some 2,4-Diamino-Furo[2,3-D]pyrimidine Derivatives. Bioorg. Med. Chem. Lett. 20, 6188–6190. doi:10.1016/j.bmcl.2010.08.122
Hu, Y.-G., Zheng, A.-H., Li, G.-J., Dong, M.-Z., Ye, F., Sun, F., et al. (2014). Efficient Synthesis of New Thieno 2,3-D Pyrimidin-4(3h)-One Derivatives for Evaluation as Anticancer Agents. J. Heterocycl. Chem. 51, 84–88. doi:10.1002/jhet.1823
Hu, Y., Gao, H., Wang, G., Wang, Y., Qu, Y., and Xu, J. (2012). Synthesis and Antitumor Activity of Some 2-Amino-Furo[2,3-D]- Yrimidin-4(3h)-One Derivatives. Chin. J. Org. Chem. 32, 1468–1472. doi:10.6023/cjoc201203003
Isaev, N. K., Stelmashook, E. V., and Genrikhs, E. E. (2020). Role of Zinc and Copper Ions in the Pathogenetic Mechanisms of Traumatic Brain Injury and Alzheimer's Disease. Rev. Neurosci. 31, 233–243. doi:10.1515/revneuro-2019-0052
Kim, H., Sarkar, S., Nandy, M., and Ahn, K. H. (2021). Imidazolyl-Benzocoumarins as Ratiometric Fluorescence Probes for Biologically Extreme Acidity. Spectrochimica Acta A: Mol. Biomol. Spectrosc. 248, 119088. doi:10.1016/j.saa.2020.119088
Klenner, M. A., Pascali, G., Massi, M., and Fraser, B. H. (2021). Fluorine‐18 Radiolabelling and Photophysical Characteristics of Multimodal PET-Fluorescence Molecular Probes. Chem. Eur. J. 27, 861–876. doi:10.1002/chem.202001402
Li, Q., Chen, Y.-M., Hu, Y.-G., Luo, X., Ko, J. K. S., and Cheung, C. W. (2016). Synthesis and Biological Activity of Fused Furo[2,3-D]pyrimidinone Derivatives as Analgesic and Antitumor Agents. Res. Chem. Intermed. 42, 939–949. doi:10.1007/s11164-015-2064-8
Li, R.-H., Feng, X.-Y., Zhou, J., Yi, F., Zhou, Z.-Q., Men, D., et al. (2021). Rhomboidal Pt(Ii) Metallacycle-Based Hybrid Viral Nanoparticles for Cell Imaging. Inorg. Chem. 60, 431–437. doi:10.1021/acs.inorgchem.0c03095
Liang, L., Lan, F., Ge, S., Yu, J., Ren, N., and Yan, M. (2017). Metal-Enhanced Ratiometric Fluorescence/Naked Eye Bimodal Biosensor for Lead Ions Analysis with Bifunctional Nanocomposite Probes. Anal. Chem. 89, 3597–3605. doi:10.1021/acs.analchem.6b04978
Liu, J., Xie, Y., Yang, Q., Huang, N., and Wang, L. (2021). Ugi Four-Component Reaction Based on the In Situ Capture of Amines and Subsequent Modification Tandem Cyclization Reaction: "One-Pot" Synthesis of Six- and Seven-Membered Heterocycles. Chin. J. Org. Chem. 41, 2374–2383. doi:10.6023/cjoc202012040
Liu, M.-G., Liu, N., Xu, W.-H., and Wang, L. (2019a). Tandem Reaction Strategy of the Passerini/Wittig Reaction Based on the In Situ Capture of Isocyanides: One-Pot Synthesis of Heterocycles. Tetrahedron 75, 2748–2754. doi:10.1016/j.tet.2019.03.057
Liu, N., Chao, F., Liu, M.-G., Huang, N.-Y., Zou, K., and Wang, L. (2019b). Odorless Isocyanide Chemistry: One-Pot Synthesis of Heterocycles via the Passerini and Postmodification Tandem Reaction Based on the In Situ Capture of Isocyanides. J. Org. Chem. 84, 2366–2371. doi:10.1021/acs.joc.8b03242
Ma, L., Wu, G., Li, Y., Qin, P., Meng, L., Liu, H., et al. (2015). A Reversible Metal Ion Fueled DNA Three-Way Junction Molecular Device for "Turn-On and -Off" Fluorescence Detection of Mercury Ions (Ii) and Biothiols Respectively with High Selectivity and Sensitivity. Nanoscale 7, 18044–18048. doi:10.1039/c5nr04688b
Marchetti, C. (2014). Interaction of Metal Ions with Neurotransmitter Receptors and Potential Role in Neurodiseases. Biometals 27, 1097–1113. doi:10.1007/s10534-014-9791-y
Min, X., Zhang, J., Li, R.-H., Xia, F., Cheng, S.-Q., Li, M., et al. (2021). Encapsulation of Nir-Ii Aiegens in Virus-like Particles for Bioimaging. ACS Appl. Mater. Inter. 13, 17372–17379. doi:10.1021/acsami.1c02691
Nsanzamahoro, S., Cheng, W., Mutuyimana, F. P., Li, L., Wang, W., Ren, C., et al. (2020). Target Triggered Fluorescence "Turn-Off" of Silicon Nanoparticles for Cobalt Detection and Cell Imaging with High Sensitivity and Selectivity. Talanta 210, 120636. doi:10.1016/j.talanta.2019.120636
Park, J.-S., Blair, N. F., and Sue, C. M. (2015). The Role of Atp13a2 in Parkinson's Disease: Clinical Phenotypes and Molecular Mechanisms. Mov Disord. 30, 770–779. doi:10.1002/mds.26243
Sannigrahi, A., Chowdhury, S., Das, B., Banerjee, A., Halder, A., Kumar, A., et al. (2021). The Metal Cofactor Zinc and Interacting Membranes Modulate Sod1 Conformation-Aggregation Landscape in an In Vitro Als Model. Elife 10, 61453. doi:10.7554/elife.61453
Schmidt, K., Wolfe, D. M., Stiller, B., and Pearce, D. A. (2009). Cd2+, Mn2+, Ni2+ and Se2+ Toxicity to Saccharomyces cerevisiae Lacking YPK9p the Orthologue of Human ATP13A2. Biochem. Biophysical Res. Commun. 383, 198–202. doi:10.1016/j.bbrc.2009.03.151
Sun, Y., Ding, F., Chen, Z., Zhang, R., Li, C., Xu, Y., et al. (2019). Melanin-Dot-Mediated Delivery of Metallacycle for Nir-Ii/Photoacoustic Dual-Modal Imaging-Guided Chemo-Photothermal Synergistic Therapy. Proc. Natl. Acad. Sci. USA. 116, 16729–16735. doi:10.1073/pnas.1908761116
Wang, C., Zhang, R., Wei, X., Lv, M., and Jiang, Z. (2020). “Metalloimmunology: The Metal Ion-Controlled Immunity,” in Advances in Immunology in China, Pt B. Editors C. Dong, and Z. Jiang, 187–241. doi:10.1016/bs.ai.2019.11.007
Wang, L., Frei, M. S., Salim, A., and Johnsson, K. (2019). Small-Molecule Fluorescent Probes for Live-Cell Super-resolution Microscopy. J. Am. Chem. Soc. 141, 2770–2781. doi:10.1021/jacs.8b11134
Wang, L., Guan, Z.-R., and Ding, M.-W. (2016a). One-Pot Synthesis of 1h-Isochromenes and 1,2-Dihydroisoquinolines by a Sequential Isocyanide-Based Multicomponent/Wittig Reaction. Org. Biomol. Chem. 14, 2413–2420. doi:10.1039/c5ob02405f
Wang, L., Xie, Y.-B., Huang, N.-Y., Yan, J.-Y., Hu, W.-M., Liu, M.-G., et al. (2016b). Catalytic Aza-Wittig Reaction of Acid Anhydride for the Synthesis of 4H-Benzo[d][1,3]oxazin-4-Ones and 4-Benzylidene-2-Aryloxazol-5(4h)-Ones. ACS Catal. 6, 4010–4016. doi:10.1021/acscatal.6b00165
Yang, H., Wu, Y., and Tian, F. (2019). A Fluorescent Sensor for Cu2+ Ion with High Selectivity and Sensitivity Based on Ict and Pet. J. Fluoresc. 29, 1153–1159. doi:10.1007/s10895-019-02406-z
Yin, H. Z., Wang, H.-L., Ji, S. G., Medvedeva, Y. V., Tian, G., Bazrafkan, A. K., et al. (2019). Rapid Intramitochondrial Zn2+ Accumulation in Ca1 Hippocampal Pyramidal Neurons after Transient Global Ischemia: A Possible Contributor to Mitochondrial Disruption and Cell Death. J. Neuropathol. Exp. Neurol. 78, 655–664. doi:10.1093/jnen/nlz042
Keywords: furo[2, 3-d]pyrimidinone, schiff base, fluorescence, Zn2+ recognition, Cu2+/Fe2+ detection, live cells imaging
Citation: Hu Y, Luo C, Gui L, Lu J, Fu J, Han X, Ma J and Luo L (2021) Synthesis and Discovery of Schiff Base Bearing Furopyrimidinone for Selective Recognition of Zn2+ and its Applications in Cell Imaging and Detection of Cu2+. Front. Chem. 9:774090. doi: 10.3389/fchem.2021.774090
Received: 10 September 2021; Accepted: 25 October 2021;
Published: 29 November 2021.
Edited by:
Yue Sun, South-Central University for Nationalities, ChinaReviewed by:
Long Wang, China Three Gorges University, ChinaDi Wu, Wuhan University of Technology, China
Sun Zhongyue, Hubei University of Chinese Medicine, China
Copyright © 2021 Hu, Luo, Gui, Lu, Fu, Han, Ma and Luo. This is an open-access article distributed under the terms of the Creative Commons Attribution License (CC BY). The use, distribution or reproduction in other forums is permitted, provided the original author(s) and the copyright owner(s) are credited and that the original publication in this journal is cited, in accordance with accepted academic practice. No use, distribution or reproduction is permitted which does not comply with these terms.
*Correspondence: Xinya Han, eGlueWFoYW5AYWh1dC5lZHUuY24=; Junkai Ma, bWFqdW5rYWkxN0BoYm11LmVkdS5jbg==; Lun Luo, bHVvbHVuQGhibXUuZWR1LmNu