- 1Tianjin Key Laboratory of TCM Chemistry and Analysis, Tianjin University of Traditional Chinese Medicine, Tianjin, China
- 2Institute of TCM, Tianjin University of Traditional Chinese Medicine, Tianjin, China
In the process of continuing to investigate ultraviolet b (UVB) irradiation protective constituents from Oplopanax elatus stems, nine new sesquiterpenes, named as eurylosesquiterpenosides A–D (1–4), eurylosesquiterpenols E–I (5–9), and ten known ones (10–19) were gained. Their structures were established by analysis of their NMR spectroscopic data, and electronic circular dichroism calculations were applied to define their absolute configurations. In addition, UVB induced HaCaT cells were used to study their anti-photoaging activities and mechanism. The results consolidated that compounds 7, 11, and 14 could improve the survival rate of HaCaT cells in concentration dependent manner at 10, 25, and 50 μM. Furthermore, western blot assay suggested that all of them could inhibit the expression of matrix metalloproteinase-1 (MMP-1), and increase the level of type I collagen markedly. Compounds 11 and 14 could reduce the phosphorylation of extracellular signal-regulated kinase and p38, respectively. Besides, compounds 7, 11, and 14 could significantly down-regulate the expression of inflammation related protein, such as tumor necrosis factor-α and cyclooxygenase-2, which indicated that they played anti-photoaging activities by reducing MMP-1 expression via down-regulating the production of inflammatory mediators and cytokines in UVB-induced HaCaT cells.
Introduction
Skin aging is categorized into intrinsic and extrinsic aging. Extrinsic aging (photoaging) is considered to be the most direct cause of it and mainly caused by ultraviolet B (UVB) irradiation (Pittayapruek et al., 2016; Cavinato and Jansen-Dürr, 2017). Overexpression of matrix metalloproteinases (MMPs) and degradation of collagens are the characteristics of UVB induced photoaging (Rittié and Fisher, 2002). Type I collagen (COL1A1) is the most abundant of subtype of collagens. MMP-1 plays a crucial role in the process of photoaging in virtue of major collagenase for COL1A1 degradation (Pittayapruek et al., 2016). As one of inflammatory mediators, mitogen-activated protein kinases (MAPKs), comprising extracellular signal-regulated kinase (ERK), c-Jun NH2-terminal kinase (JNK), and p38 are chiefly associated with collagen degradation mediated by MMP-1 (Yang et al., 2020). The activation of MAPKs by increasing the phosphorylation of p38, JNK, and ERK (p-p38, p-JNK, p-ERK) can up-regulate inflammatory cytokines such as nuclear factor kappa B (NF-κB), tumor necrosis factor α (TNF-α), interleukin 6 (IL-6), and cyclooxygenase-2 (COX-2) (Choi et al., 2020). Their overexpression will activate MMP-1 to accelerate the degradation of collagen, thereby promote photoaging (Parrado et al., 2016; Peng et al., 2020). Thus, anti-inflammation, as well as inhibiting collagen degradation are the main strategies for preventing UVB-induced photoaging.
Oplopanax elatus Nakai belongs to Oplopanax genus (Araliaceae family). It was reviewed to contain various constituents such as volatile oil, phenolic acids, lignans, quinic acid esters, steroids, and aliphatic acids, and the stem of it was reported to exhibit anti-aging effect (Yan et al., 2021). Moreover, our previous study demonstrated that phenolic acids obtained from it had anti-photodamage activity, too (Han et al., 2021). We hypothesize there are other components may exhibit benefits for the skin photodamage. Then, the other constituents in the stems of O. elatus, along with their activities and mechanisms against photoaging induced by UVB irradiation in HaCaT cells were continue to be investigated.
Results and Discussion
Structural Elucidation
19 sesquiterpenes, including nine new ones, named as eurylosesquiterpenosides A–D (1–4), eurylosesquiterpenols E–I (5–9) (Figure 1), and ten known ones, oplodiol (10) (Ono et al., 2008), 1(R),4β-dihydroxy-trans-eudesm-7-ene-1-O-β-d-glucopyranoside (11) (Lee et al., 2010), massonside B (12) (Xiao et al., 2016), massonside A (13) (Xiao et al., 2016), (1R,4S,10R)10,11-dimethyl-dicyclohex-5(6)-en-1,4-diol-7-one (14) (Elmasri et al., 2016), cadinane-4β,5α,10β-triol (15) (Kuo et al., 2003; Fang et al., 2006), 7-epi-11-hydroxychabrolidione A (16) (Pereira et al., 2012), (—)-4α,7β-aromaden-dranediol (17) (Beechan et al., 1978), aromadendrane-4α,10α-diol (18) (Moreira et al., 2003), stachytriol (19) (Soliman et al., 2007) (Figure 2) were isolated from the stems of O. elatus. The structures of them were identified by the comprehensive application of UV, IR, NMR, (α)D, MS, as well as electronic circular dichroism (ECD) spectra. Among them, 11–19 were obtained from Oplopanax genus for the first time.
Eurylosesquiterpenoside A (1) was isolated as a white powder and afforded a pseudo-molecular ion peak at m/z 463.25449 (M + COOH)− (calcd for C22H39O10, m/z 463.25377) in the ESI-Q-Orbitrap MS, which was compatible with a molecular formula of C21H38O8. A combined 1H, 13C NMR (Table 1) and HSQC spectra analysis revealed the presence of four methyl [δH 0.91, 1.10, 1.16, 1.18 (3H each, all s, H3-14, 15, 12, 13)], one oxygenated methine [δH 3.41 (1H, dd, J = 4.0, 11.5 Hz, H-1)], two quaternary carbon substituted with oxygen [δC 72.5 (C-4), 73.6 (C-11)], five methene, two methine, and one quaternary carbon. The moieties of “–O–CH–CH2–CH2–” and “–CH–CH2–CH–CH2–CH2–” were deduced from the proton and proton correlations displayed in its 1H 1H COSY spectrum (Figure 3). The HMBC cross-peaks from H3-12 to C-7, C-11, C-13; H3-13 to C-7, C-11, C-12; H3-14 to C-1, C-5, C-9, C-10; H3-15 to C-3–5 (Figure 3) made the above mentioned funtional gruops and moieties connected together, and suggesting it was an eudesmane type sesquiterpene. Meanwhile, the presence of one anomeric carbon signal at δC 102.0, along with other oxygenated carbon signals in the region of δC 63.0–78.3 in its 13C NMR spectrum, indicated there was a monosaccharide moiety. After hydrolyzing 1 with 1 M HCl, the product was analyzed by using HPLC with optical rotation detector (Yoshikawa et al., 2007), and showed positive peak at 10.3 min, which was identical to that of d-glucose standard (10.4 min) (Supplementary Figure S109). Moreover, the monosaccharide was determined to be one β-d-glucopyranose inferring from the large coupling constant of the anomeric proton at δ 4.29 (1H, d, J = 8.0 Hz, H-1′). Furtherly, the relative configuration of its aglycon was elucidated by the NOE correlations between δH 3.41 (H-1) and δH 1.27 (H-5), 1.47 (Hα-3); δH 1.76 (Hβ-3) and δH 1.10 (H3-15); δH 1.93 (Hα-6) and δH 1.27 (H-5), 1.31 (H-7); δH 0.91 (H3-14) and δH 1.10 (H3-15), 1.12 (Hβ-6) (Figure 4). Furthermore, the HMBC correlation from H-1′ to C-1 supported the assumption that the β-d-glucopyranosyl attached to C-1. Both the planar structure and relative configuration were the same as those of the known compounds, boarioside (Munoz et al., 1995) and pterodontoside F (Zhao et al., 1997). But there were great differences in their NMR signals. Lots of research results suggested that 10-methyl and 7-isopropyl was cis configuration when δC-5 and δC-7 were 54 ± 2 and 50 ± 1, respectively; while they would be trans-conformed to each other when δC-5 and δC-7 were 49 ± 1 and 42 ± 1, respectively (Kesselmans et al., 1991; Ando et al., 1994; Shimoma et al., 1998; Zhu et al., 2007). Herein, δC-5 and δC-7 were 54.5 and 50.8 for eurylosesquiterpenoside A (1), respectively, suggesting that the relative configuration of 10-methyl and 7-isopropyl was cis. It was consistent with the NOE analytical result. The δC-5 and δC-7 in boarioside were 48.6 and 43.0 (Munoz et al., 1995), while those of pterodontoside F were 48.4 and 42.6, respectively (Zhao et al., 1997). It further indicated that the structure determination of boarioside and pterodontoside F were mistake. The configuration of pterodontoside F’s aglycon (pterodontriol B) had proved by single crystal diffraction, and its 10-methyl and 7-isopropyl should be trans-, instead of cis-form (Zhu et al., 2007). Therefore, the relative configuration of eurylosesquiterpenoside A (1) was firstly clarified as 1R*,4R*,5R*,7R*,10R* though its planar structure had been reported.
Eurylosesquiterpenoside B (2) was obtained as white powder with negative optical rotation [(α)D25–34.9, MeOH]. It had a molecular formula of C21H36O8 assigned basing on the carboxyl adduct (M + COOH)− at m/z 461.23981 in the ESI-Q-Orbitrap MS. d-glucose was detected from its acid hydrolysate by using the similar method as compound 1 (Yoshikawa et al., 2007). Its 1H and 13C NMR (Table 1) spectra indicated the existence of one β-d-glucopyranosyl [δH 4.32 (1H, d, J = 7.5 Hz, H-1′); δC 63.1, 72.0, 75.1, 77.8, 78.3, 101.9]. There were twenty-one carbon signals in its 13C NMR spectrum. Apart from the above six carbon signals of β-d-glucopyranosyl, fifteen carbon signals were remaining. Most of them were located in the high resonance region, suggesting that compound 2 was a sesquiterpenoid glycoside. Its 1H, 13C NMR spectra showed the signals related to the protons of three methyl [δH 1.01, 1.14 (3H each, both s, H3-14, 15), 1.04 (3H, d, J = 7.0 Hz, H3-13)], one methene bonded to oxygen [δH 3.38 (1H, dd, J = 5.5, 10.5 Hz), 3.57 (1H, dd, J = 6.0, 10.5 Hz), H2-12], one oxygenated methine [δH 3.42 (1H, dd, J = 4.0, 11.5 Hz, H-1)], as well as one olefinic proton signal [δH 5.37 (1H, d, J = 4.5 Hz, H-8)] in its aglycon. Five fragments showed by the bold line in Figure 3 were denoted according to the proton and proton correlations. Moreover, the HMBC cross-peaks were observed from H2-6 to C-7, C-8; H-11 to C-6–8; H2-12 to C-7, C-13; H3-13 to C-7, C-12; H3-14 to C-1, C-5, C-9, C-10; H3-15 to C-3–5; H-1′ to C-1 (Figure 3). Then, the planar structure of two was determined, which was the same as those chrysantiloboside (Shin et al., 2012) and iwayoside C (Ding et al., 2011). The NOE cross-peaks between δH 1.30 (H-5) and δH 1.14 (H3-15), 1.90 (Hα-9), 3.42 (H-1); δH 2.14 (Hβ-9) and δH 1.01 (H3-14) (Figure 4) consolidated that 1-OH, 4-OH, H-5, and 14-CH3 was in β, β, α, and β orientation, respectively. The resonance signals for protons and carbons in C-1–5, 9, 10 were consistent with those of 1(R),4β-dihydroxy-trans-eudesm-7-ene-1-O-β-d-glucopyranoside (11) (Lee et al., 2010), which implied the absolute configurations of C-1, 4, 5, 10 of eurylosesquiterpenoside B (2) were R, S, R, R, respectively. .
Eurylosesquiterpenoside C (3), white powder with (α)D25 –29.3 (in MeOH). The same molecular formula, C21H36O8 [m/z 461.23886 (M + COOH)−; calcd for C22H37O10, 461.23812] as compound 2 was revealed by the ESI-Q-Orbitrap MS analysis. The 1H, 13C NMR (Table 1), and 1H 1H COSY, HSQC, as well as HMBC spectra suggested their planar structure were also same. The 13C NMR data of C-1–5, 10, 14, 15, and 1′–6′, as well as the NOE correlations for H-1, 5, 9, 14, 15 in 3 were very consistent with those of 2 (Figure 4), indicating the absolute configurations of C-1, 4, 5, 10 were identical to 2 (1R,4S,5R,10R). However, the retention times of them were 24.4 min for 2 and 19.5 min [column: Cosmosil 5C18-MS-II (4.6 mm i. d. × 250 mm, 5 µm); mobile phase: CH3CN-1% HAc (16:84, v/v); flow rate: 0.7 ml/min, Supplementary Figure S25] for 2 and 3, respectively. Moreover, the ΔδC of 3 and 2 were −0.3, −1.0, +0.5, +0.4, −0.7 at C-6, 7, 8, 11, 13, respectively, which indicated the difference between them might be caused by the configuration difference of C-11.
It was worth pointing out that the structures of reported compounds iwayoside C (Ding et al., 2011) and chrysantiloboside (Shin et al., 2012) were identical since the consistency of their nuclear magnetic resonance. Moreover, though the planar structures of 2 and 3 were the same as them, there were no relevant reports on the determination of absolute configuration had been found in the literature. Eurylosesquiterpenoside D (4) had the same molecular formula, C21H36O8, as compounds 2 and 3. While, comparing with the 1H and 13C NMR (Table 1) spectra of 2 and 3, it was found that the NMR resonance of C-7 increased significantly. Meanwhile, their coupling and splitting information were also different [2: δH 5.37 (1H, d, J = 4.5 Hz), 3: δH 5.38 (1H, d, J = 5.5 Hz), 4: δH 5.56 (1H, br. s)]. The correlations between δH 1.87 (H-5) and δH 5.56 (H-6); δH 1.93, 1.99 (H2-8) and δH 1.26, 2.05 (H2-9) and correlations from δH 5.56 (H-6) to δC 71.5 (C-4) (Figure 3) suggested the olefinic bond replaced between C-6 and C-7, rather than between C-7 and C-8. According to the NOE correlations between δH 1.87 (H-5) and δH 1.20 (H3-15), 3.44 (H-1); δH 3.44 (H-1) and δH 1.24 (Hα-9); δH 2.05 (Hβ-9) and δH 1.01 (H3-14) (Figure 4), the relative configurations of H-1, H-5, H-9, 14-CH3, and 15-CH3 were revealed. The chemical shifts of protons in C-1–5, 9, and 10 were almost identical to the known compound, 1(R),4β-dihydroxy-trans-eudesm-6-ene-1-O-β-d-glucopyranoside (Lee et al., 2010). Then, the absolute configurations at C-1, 4, 5, and 10 were determined as R, S, R, and R, respectively. Furtherly, the calculated ECD spectrum was identical to that of experimental one (Figure 5) (Nugroho and Morita, 2014; Frisch et al., 2019; Takanawa, 2019). Then, the absolute configuration of 4 was elucidated as 1R,4S,5R,10S.
Eurylosesquiterpenol E (5) was isolated as white powder with negative optical rotation [(α)D25 –71.8, MeOH]. It afforded a pseudo-molecular ion peak at m/z 281.17557 (M + COOH)− (calcd for C16H25O4, m/z 281.17474) in the ESI-Q-Orbitrap MS, which was compatible with a molecular formula of C15H24O2. The 1H, 13C NMR (Table 2) spectra implied the presence of four methyl signals at δH 0.85, 0.98 (3H each, both d, J = 7.0 Hz, H3-12, 13), 1.19 (3H, s, H3-14), 1.78 (3H, br. s, H3-15) and one α,β-unsaturated ketone at δH 6.89 (1H, br. s, H-5) and δC 134.9 (C-4), 147.4 (C-5), 200.7 (C-3). Fifteen carbon signals were displayed in its 13C NMR spectrum, which suggested it was also a sesquiterpene. The proton and proton cross-peaks between H-1 and H2-2; H-6 and H-1, H-5, H-7; H2-8 and H-7, H2-9; H-11 and H-7, H3-12, H3-13 proved the existence of moieties showed with the bold lines in Figure 3. And its planar structure was consolidated by the long-range correlations from H2-2, H-5 to C-3, C-4; H3-14 to C-1, C-9, C-10; H3-15 to C-3–5, which was a cadinane type sesquiterpene. Moreover, the relative configuration was revealed by the NOE correlations between δH 1.72 (H-1) and δH 1.16 (H-7), 1.19 (H3-14); δH 2.25 (H-11) and δH 2.40 (H-6) (Figure 6), and it was identical to that of 3-oxo-α-cadinol (Wu et al., 2005). Furthermore, the ECD experiment was developped to clarify its configuration. As result, its experimental ECD was consistent with that of 1R,6R,7S, 10S calculated (Figure 5) (Nugroho and Morita, 2014; Frisch et al., 2019; Takanawa, 2019). Then, the absolute configuration of eurylosesquiterpenol E (5) was elucidated here firstly.
Eurylosesquiterpenol F (6), a white powder with negative optical rotation [(α)D25 –36.0, MeOH]. The MS [m/z 281.17548 (M + COOH)−; calcd for C16H25O4, m/z 281.17474] and NMR (Table 2; Figure 3) spectra implied the planar structure of 6 was consistent with 5. However, their ΔδC of C-1, 8, 9, 10, 14 were +1.3, +2.2, +1.8, +1.6, and −6.9, respectively, which might be caused by the different configuration of 14-CH3. The cross-peaks between δH 1.84 (H-1) and δH 1.21 (H-7); δH 2.08 (H-6) and δH 1.17 (H3-14), 2.23 (H-11) displaying in its NOSEY spectrum (Figure 6) suggested 14-CH3 was in α orientation. And the relative configuration of 6 was clarified. Finally, the configuration of 1R,6R,7S,10R was determined by the uniformity of its expeimental and calculated ECD spectra (Figure 5).
The molecular formula, C15H26O2, of eurylosesquiterpenol G (7) was assigned basing on the carboxyl adduct (M + COOH)– at m/z 283.19107 (calcd for C16H27O4, m/z 283.19039) in the ESI-Q-Orbitrap MS. Comparing with 5, 6, its molecular weight went up by 2Da. Meanwhile, the 13C NMR (Table 2) spectrum suggested the disappearence of one carbon signal of α,β-unsaturated ketone, and the appearence of one oxygenated methine [δH 4.34 (1H, br. s, H-3)]. Then, 7 was speculated to be formed by the reduction of 3-carbonyl. The correlations displayed in its 1H 1H COSY and HMBC spectra (Figure 3) fully proved the correctness of the speculation. The chemical shift of C-14 (δC 29.0) beling closer to compound 5 (5: δC 28.2; 6: δC 21.4) indicated the configuration of C-10 was S. Moreover, according to the NOE correlations between δH 1.83 (H-1) and δH 1.10 (H-7), 1.45 (H3-14); δH 1.45 (H3-14) and δH 2.49 (Hβ-2); δH 2.06 (Hα-2) and δH 2.46 (H-6), 4.34 (H-3) (Figure 6), the configuration of 1R,3R,6R,7S,10S was clarified. It was confirmed by the consistency of expermental and calculated ECD results (Figure 5) (Nugroho and Morita, 2014; Frisch et al., 2019; Takanawa, 2019).
The molecular formula of eurylosesquiterpenol H (8) was determined as C15H28O4 by ESI-Q-Orbitrap MS spectrometry. The 1H, 13C NMR (Table 2) spectra suggested the presence of four methyl [δH 1.10, 1.17, 1.30 (3H each, all s, H3-14, 12, 13), 0.89 (3H, d, J = 7.0 Hz, H3-15)] and one oxygnated methine [δH 4.70 (1H, br. d, ca. J = 9 Hz, H-2)]. Combining the proton and proton correlations and the long-range cross-peaks from H2-3, H-4, H2-6, H2-9, C-1; H-5 to C-1, C-2, C-10; H2-8 to C-10; H3-12 to C-7, C-11, C-13; H3-13 to C-7, C-11, C-12; H3-14 to C-1, C-9, C-10 (Figure 3), the planar structure of compound 8 was clarified, which was a guaiane type sesquiterpene. The chemical shifts of C-6–10 (Table 2) were consistent with those of stachytriol (19) (Soliman et al., 2007), which indicated that the configurations at C-1, 5, 7, 10 of them were identical. The main difference between 8 and 19 was that C-2 in the former was substituted by the hydroxyl. The NOE correlation between δH 4.70 (H-2) and δH 1.10 (H3-14) implied 2-OH was in β orientation. Meanwhile, the cross-peaks between other protons (Figure 7) were basically consistent with those of stachytriol (19). Consequently, the configuration of eurylosesquiterpenol H (8) was denoted as 1R*,2R*,4S*,5S*,7R*,10S*.
Eurylosesquiterpenol I (9) was isolated as white powder. Its molecular formula was determined as C15H24O3 with m/z 251.16508 (M—H)− (calcd for C15H23O3, m/z 251.16417). 1H and 13C NMR (Table 2) spectra showed three methyl proton signals at δH 1.08, 1.25, 1.45 (3H each, all s, H3-12, 13, 15) and one carboxyl carbon signal at δC 178.8 (C-14). The moieties displayed with the bold lines in Figure 3 were determined by the observation of proton and proton correlations in the 1H 1H COSY spectrum. Moreover, its planar structure was determined by the cross-peaks found from H3-12 to C-6, C-7, C-11, C-13; H3-13 to C-6, C-7, C-11, C-12; H3-15 to C-3–5; H-1 to C-14 (Figure 3). Finally, the NOE correlations between δH 0.56 (H-6) and δH 0.67 (H-7), 1.45 (H3-15); δH 1.45 (H3-15) and δH 1.70 (Hα-3), 2.29 (H-1); δH 1.70 (Hα-3) and δH 2.10 (Hα-2); δH 1.93 (Hβ-2) and δH 2.53 (H-10); δH 2.53 (H-10) and δH 1.83 (H-5) were observed in its NOESY spectrum (Figure 7). Then, the configuration of eurylosesquiterpenol I (9) was elucidated to be 1R*,4S*,5R*,6R*,7R*,10R*.
The structures of known compounds 10–19 were identified by comparing their 1H, 13C NMR spectroscopic data with those reported in literatures.
Biological Activity
In addition, in vitro anti-photoaging activities of the obtained sesquiterpenes were evaluated. MTT assay results showed that most of compounds (2, 3, 6, 7, 10, 11, 13–18) were non-cytotoxic at 50 μM on HaCaT cells (Supplementary Figure S110). Among them, HaCaT cells were pretreated with test samples (50 μM) for 24 h before UVB irradiation individually. Comparing with UVB-induced group (Con), 7, 11, and 14 could significantly improve the survival rate of HaCaT cells after UVB irradiation (Table 3). Moreover, the activities of compounds 7, 11, and 14 against UVB injury were found to be in concentration dependent manner at 10, 25, and 50 μM (Figure 8).
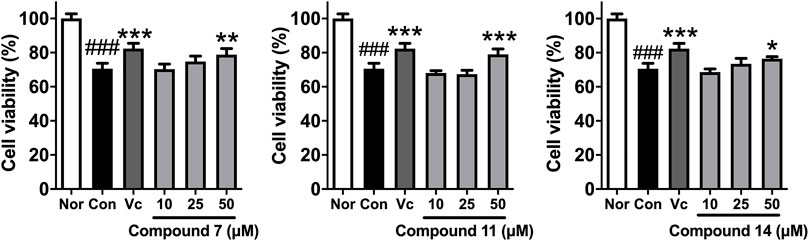
FIGURE 8. The effects of compounds 7, 11, and 14 at concentration of 10, 25, 50 μM on the cell viability of HaCaT cells induced by UVB. Nor: normal group; Con: UVB-induced group; Vitamin C (Vc). Values represent the mean ± SEM of six determinations (###p < 0.001 vs. Nor; ***p < 0.001, **p < 0.01, and *p < 0.05 vs. Con).
As we introduced previously, the level of MMP-1 will be increased after UVB irradiation, and the degradation of COL1A1 will be caused at the same time in HaCaT cells. The process is related to the up-regulation of inflammatory mediator, MAPKs, and inflammatory cytokines such as TNF-α and COX-2. Therefore, the expressions of above proteins were evaluated by using western blot assay to study the anti-photoaging mechanism of compounds 7, 11, and 14.
Comparing with normal group (Nor), the level of MMP-1 was increased and COL1A1 was decreased significantly in Con after UVB irradiation. While, the expression of MMP-1 was significantly decreased by 30, 21, and 16%, and the level of COL1A1 was up-regulated by 24, 36, and 29% in pretreatment of compounds 7, 11, and 14, respectively (Figure 9).
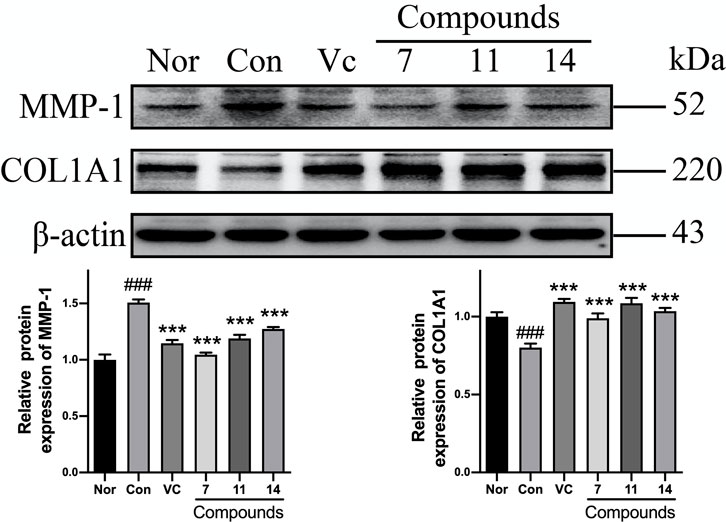
FIGURE 9. The influence of compounds 7, 11, and 14 at concentration of 50 μM on the protein of MMP-1 and COL1A1 in HaCaT cells. Nor: normal group; Con:UVB-induced group; Vitamin C (Vc). Values represent the mean ± SEM of three determinations (###p < 0.001 vs. Nor; ***p < 0.001 vs. Con).
Meanwhile, the phosphorylations of MAPKs were up-regulated in varying levels in Con comparing with Nor. However, the p-ERK was markedly reduced to 0.62-fold by compound 11; and the p-p38 could be inhibited to 0.74-fold by 14. Nevertheless, none of active compounds could prevent up-regulation of p-JNK (Figure 10).
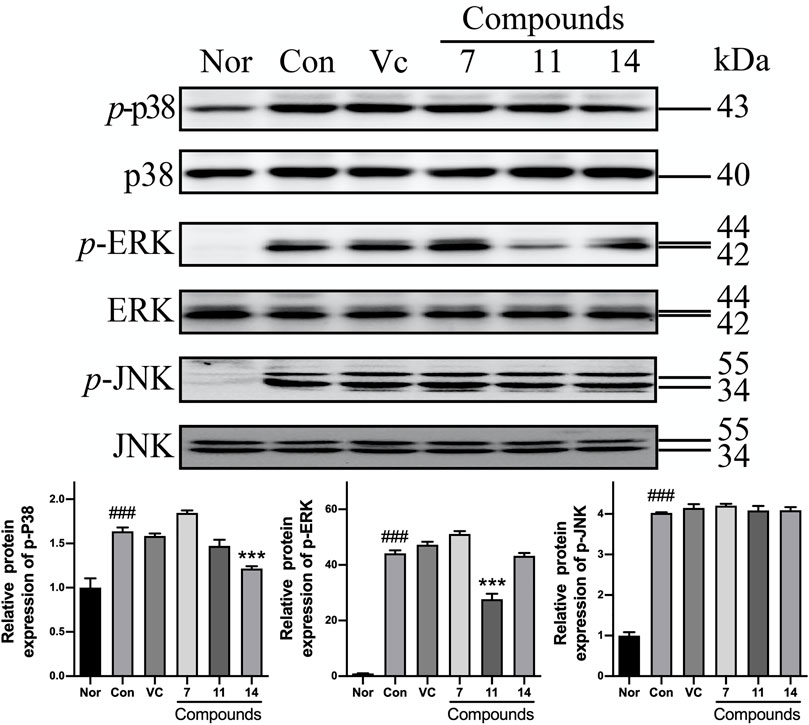
FIGURE10. The influence of compounds 7, 11, and 14 at concentration of 50 μM on the protein of MAPKs in HaCaT cells. Nor: normal group; Con: UVB-induced group; Vitamin C (Vc). Values represent the mean ± SEM of three determinations (###p < 0.001 vs. Nor; ***p < 0.001 vs. Con).
After pretreating with compounds 7, 11, and 14, the TNF-α expression could be significantly reduced by 18, 17, 35%, and the level of COX-2 was decreased by 40, 38, 21% comparing with Con, respectively (Figure 11).
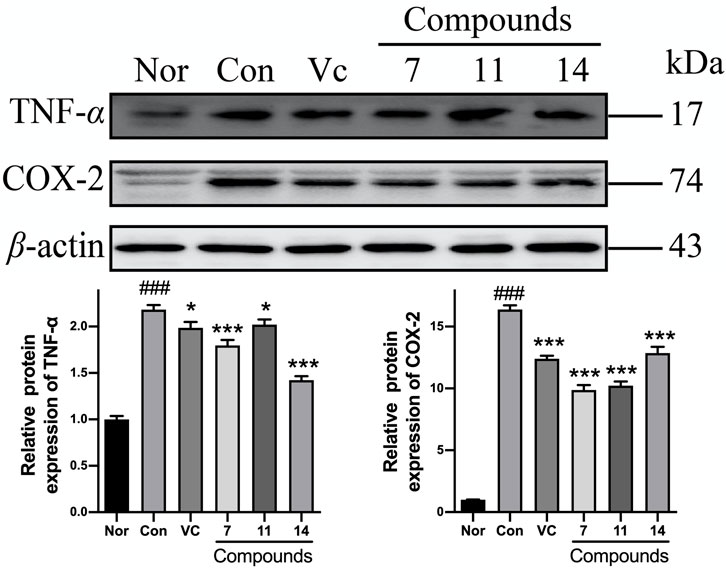
FIGURE 11. The influence of compounds 7, 11, and 14 at concentration of 50 μM on the protein of inflammatory cytokines (TNF-α and COX-2) in HaCaT cells. Nor: normal group; Con: UVB-induced group; Vitamin C (Vc). Values represent the mean ± SEM of three determinations (###p < 0.001 vs. Nor; ***p < 0.001 and *p < 0.05 vs. Con).
Basing on the above results, the anti-photoaging mechanism of compounds 7, 11, and 14 might be related to inhibiting collagen degradation via anti-inflammation.
Materials and Methods
Experimental Procedures for Phytochemistry Study
General Experimental Procedures
NMR spectra were performed on Bruker ascend 600 MHz and/or Bruker ascend 500 MHz NMR spectrometer (Bruker BioSpin AG Industriestrasse 26 CH-8117) with tetramethylsilane as an internal standard. Negative-ion mode ESI-Q-Orbitrap MS were determined on a Thermo ESI-Q-Orbitrap MS mass spectrometer connected to the UltiMate 3000 UHPLC instrument via ESI interface (Thermo Scientific). Optical rotations, UV, IR, and ECD spectra were run on a Rudolph Autopol® IV automatic polarimeter (l = 50 mm) (Rudolph Research Analytical, Hackettstown), Varian Cary 50 UV-Vis (Varian, Inc.), Varian 640-IR FT-IR spectrophotometer (Varian Australia Pty Ltd.), and Circular dichroism spectrum (J-815, JASCO company), respectively.
Column chromatorgtaphies (CC) were accomplished on macroporous resin D101 (Haiguang Chemical Co., Ltd.), silica gel (48–75 μm, Qingdao Haiyang Chemical Co., Ltd.), ODS (50 μm, YMC Co., Ltd.), MCI gel CHP 20P (Mitsubishi Chemical Corporation, CHP20/P120), and Sephadex LH-20 (Ge Healthcare Bio-Sciences). HPLC column: Cosmosil 5C18-MS-II (4.6 mm i. d. × 250 and 20 mm i. d. × 250 mm) (5 μm, Nakalai Tesque, Inc.) were used to analysis and separate the constituents, respectively.
Dichloromethane (CH2Cl2), methanol (MeOH), acetonitrile (CH3CN), acetic acid (HAc), and other reagents (chromatographically pure or analytical pure) were purchased from Tianjin Concord Technology Co., Ltd.
Plant Material
The stems of Oplopanax elatus Nakai were collected from Tonghua city, Jilin province, China, identified by Professor Junyi Zhu (Tonghua Normal University). The voucher specimen (2018121001) was deposited at the Academy of Traditional Chinese Medicine of Tianjin University of TCM.
Extraction and Isolation
The 95% EtOH eluate (70.0 g) obtained previously (Han et al., 2021) was fractionated over silica gel using a gradient elution of CH2Cl2-MeOH (100:0 → 100:1 → 100:3 → 100:7 → 10:1 → 20:3 → 5:1 → 3:1 → 1:1 → 0:100, v/v) to give Fr. 1–Fr. 12. Fraction 5 (289.2 mg) was prepared by pHPLC [MeOH-1% HAc (80:20, v/v)] to yield (1R,4S,10R)10,11-dimethyl-dicyclohex-5(6)-en-1,4-diol-7-one (14, 8.4 mg). Fraction 9 (4.5 g) was separated by pHPLC [CH3CN-1% HAc (16:84, v/v)], and eurylosesquiterpenoside B (2, 42.5 mg), eurylosesquiterpenoside C (3, 20.3 mg), eurylosesquiterpenoside D (4, 7.0 mg), massonside B (12, 31.6 mg), massonside A (13, 15.8 mg) were obtained. Fraction 10 (11.0 g) was subjected to ODS CC [MeOH-H2O (10:90 → 20:80 → 30:70 → 40:60 → 50:50 → 60:40 → 100:0, v/v)] to gain Fr. 10-1–Fr. 10-9. Fraction 10-6 (581.1 mg) was purified by pHPLC [MeOH-1% HAc (25:75, v/v)] and [CH3CN-1% HAc (11:89, v/v)], successively, and eurylosesquiterpenoside A (1, 11.6 mg) was produced.
EtOAc layer extract (150.0 g) was separated by silica gel CC [PE-CH2Cl2 (8:1 → 3:1 → 0:100, v/v) → CH2Cl2-MeOH (100:1 → 100:3 → 100:7 → 10:1 → 0:100, v/v)] to give Fr. E-1–Fr. E-15. Fraction E-8 (9.8 g) was purified by Sephadex LH-20 CC (MeOH) to yield Fr. E-8-1–Fr. E-8-4. Fraction E-8-3 (5.3 g) was fractionated over ODS CC [MeOH-H2O (50:50 →60:40 → 70:30 → 80:20 → 90:10 → 100:0, v/v)], and Fr. E-8-3-1-Fr. E-8-3-16 were given. Fraction E-8-3-12 (286.7 mg) was separated by pHPLC [CH3CN-1% HAc (50:50, v/v)] to produce eurylosesquiterpenol E (5, 66.1 mg) and stachytriol (19, 58.0 mg). Fraction E-9 (15.5 g) was subjected to Sephadex LH-20 CC (MeOH) to yield Fr. E-9-1–Fr. E-9-4. Fraction E-9-3 (3.3 g) was further prepared by ODS CC [MeOH-H2O (50:50 →60:40 → 70:30 → 80:20 → 90:10 → 100:0, v/v)], then, Fr. E-9-3-1–Fr. E-9-3-12 were provided. Fraction E-9-3-4 (359.0 mg) was purified by pHPLC [CH3CN-1% HAc (30:70, v/v)] to gain 7-epi-11-hydroxychabrolidione A (16, 46.7 mg). Fraction E-9-3-8 (349.4 mg) was separated by pHPLC [CH3CN-1% HAc (55:45, v/v)] to yield oplodiol (10, 69.0 mg), eurylosesquiterpenol H (8, 15.1 mg), along with eurylosesquiterpenol F (6, 8.5 mg). Fraction E-10 (7.8 g) was subjected to Sephadex LH-20 CC (MeOH), and Fr. E-10-1–Fr. E-10-3 were given. Fraction E-10-3 (3.9 g) was fractionated over ODS CC [MeOH-H2O (50:50 →60:40 → 70:30 → 80:20 → 90:10 → 100:0, v/v)], and Fr. E-10-3-1–Fr. E-10-3-14 were furnished. Fraction E-10-3-10 (369.1 mg) was purified by pHPLC [CH3CN-1% HAc (40:60, v/v)] to produce (–)-4α,7β-aromaden-dranediol (17, 52.2 mg). Fraction E-10-3-12 (135.1 mg) was separated by pHPLC [CH3CN-1% HAc (45:55, v/v)] to give eurylosesquiterpenol I (9, 14.4 mg) and eurylosesquiterpenol G (7, 11.3 mg). Fraction E-11 (18.7 g) was chromatographed on Sephadex LH-20 CC eluted with MeOH to furnish Fr. E-11-1–Fr. E-11-3. Fraction E-11-2 (4.6 g) was subjected to ODS CC [MeOH-H2O (40:60 → 50:50 → 60:40 → 70:30 → 80:20 → 90:10 → 100:0, v/v)], and Fr. E-11-2-1–Fr. E-11-2-14 were yielded. Fraction E-11-2-6 (189.5 mg) was separated by pHPLC [CH3CN-1% HAc (27:73, v/v)] to give cadinane-4β,5α,10β-triol (15, 150.4 mg). Fraction E-11-2-12 (279.5 mg) was prepared with pHPLC [CH3CN-1% HAc (43:57, v/v)] to produce aromadendrane-4α,10α-diol (18, 13.2 mg). Fraction E-14 (4.3 g) was fractionated over Sephadex LH-20 CC (MeOH) to gain Fr. E-14–1-Fr. E-14–3. Fraction E-14–3 (123.4 mg) was purified by pHPLC [CH3CN-1% HAc (40:60, v/v)] to furnish 1(R),4β-dihydroxy-trans-eudesm-7-ene-1-O-β-d-glucopyranoside (11, 10.2 mg).
Eurylosesquiterpenoside A (1): White powder; (α)D25 –72.0 (conc 0.15, MeOH); IR νmax (KBr) cm−1: 3,360, 2,936, 2,869, 1,575, 1,384, 1,073, 1,024, 912; 1H NMR (CD3OD, 500 MHz) δH: 3.41 (1H, dd, J = 4.0, 11.5 Hz, H-1), 1.84 (1H, m, Hα-2), 1.60 (1H, dt, J = 3.0, 14.0 Hz, Hβ-2), 1.47 (1H, dt, J = 3.5, 14.0 Hz, Hα-3), 1.76 (1H, dt, J = 3.0, 14.0 Hz, Hβ-3), 1.27 (1H, dd, J = 2.0, 12.5 Hz, H-5), 1.93 (1H, br. d, ca. J = 15 Hz, Hα-6), 1.12 (1H, m, overlapped, Hβ-6), 1.31 (1H, m, H-7), 1.63 (1H, dt, J = 3.0, 11.0 Hz, Hα-8), 1.24 (1H, dt, J = 3.0, 13.0 Hz, Hβ-8), 1.13 (1H, m, overlapped, Hα-9), 2.04 (1H, dt, J = 3.0, 13.0 Hz, Hβ-9), 1.16, 1.18, 0.91, 1.10 (3H each, all s, H3-12, 13, 14, 15), 4.29 (1H, d, J = 8.0 Hz, H-1′), 3.14 (1H, dd, J = 8.0, 8.5 Hz, H-2′), 3.35 (1H, dd, J = 8.5, 9.0 Hz, H-3′), 3.28 (1H, dd, J = 9.0, 9.5 Hz, H-4′), 3.23 (1H, ddd, J = 2.0, 5.5, 9.5 Hz, H-5′), [3.66 (1H, dd, J = 5.5, 11.5 Hz), 3.85 (1H, dd, J = 2.0, 11.5 Hz), H2-6′]; 13C NMR (CD3OD, 125 MHz) δC: see Table 1; ESI-Q-Orbitrap MS m/z 463.25449 (M + COOH)− (calcd for C22H39O10, 463.25377).
Eurylosesquiterpenoside B (2): White powder; (α)D25 –34.9 (conc 1.5, MeOH); IR νmax (KBr) cm−1: 3,370, 2,958, 2,927, 2,870, 1,065, 1,024; 1H NMR (CD3OD, 500 MHz) δH: 3.42 (1H, dd, J = 4.0, 11.5 Hz, H-1), 1.70 (1H, m, Hα-2), 1.87 (1H, m, Hβ-2), 1.48 (1H, dt, J = 3.5, 13.5 Hz, Hα-3), 1.75 (1H, dt, J = 2.5, 13.5 Hz, Hβ-3), 1.30 (1H, dd, J = 5.5, 12.0 Hz, H-5), 2.02 (1H, m, Hα-6), 2.09 (1H, m, Hβ-6), 5.37 (1H, d, J = 5.0 Hz, H-8), 1.90 (1H, m, Hα-9), 2.14 (1H, dd, J = 5.0, 17.5 Hz, Hβ-9), 2.22 (1H, sex like, ca. J = 7 Hz, H-11), [3.38 (1H, dd, J = 5.5, 10.5 Hz), 3.57 (1H, dd, J = 6.0, 10.5 Hz), H2-12], 1.04 (3H, d, J = 7.0 Hz, H3-13), 1.01, 1.14 (3H each, both s, H3-14, 15), 4.32 (1H, d, J = 7.5 Hz, H-1′), 3.16 (1H, dd, J = 7.5, 9.0 Hz, H-2′), 3.35 (1H, dd, J = 9.0, 9.0 Hz, H-3′), 3.27 (1H, dd, J = 9.0, 9.0 Hz, H-4′), 3.22 (1H, ddd, J = 2.0, 5.5, 9.0 Hz, H-5′), [3.66 (1H, dd, J = 5.5, 11.5 Hz), 3.85 (1H, dd, J = 2.0, 11.5 Hz), H2-6']; 13C NMR (CD3OD, 125 MHz) δC: see Table 1; ESI-Q-Orbitrap MS m/z 461.23981 (M + COOH)− (calcd for C22H37O10, 461.23812).
Eurylosesquiterpenoside C (3): White powder; (α)D25 –29.3 (conc 0.9, MeOH); IR νmax (KBr) cm−1: 3,368, 2,958, 2,925, 2,877, 1,072, 1,021; 1H NMR (CD3OD, 500 MHz) δH: 3.41 (1H, dd, J = 4.0, 12.0 Hz, H-1), 1.69 (1H, m, Hα-2), 1.87 (1H, m, Hβ-2), 1.48 (1H, dt, J = 3.5, 13.5 Hz, Hα-3), 1.75 (1H, dt, J = 3.0, 13.5 Hz, Hβ-3), 1.32 (1H, dd, J = 5.0, 12.0 Hz, H-5), 1.96 (1H, m, Hα-6), 2.16 (1H, m, Hβ-6), 5.38 (1H, d, J = 5.5 Hz, H-8), 1.92 (1H, m, Hα-9), 2.11 (1H, dd, J = 5.5, 17.0 Hz, Hβ-9), 2.23 (1H, sex, J = 7.0 Hz, H-11), 3.33, 3.57 (1H each, both dd, J = 7.0, 10.5 Hz, H2-12), 1.04 (3H, d, J = 7.0 Hz, H3-13), 1.00, 1.14 (3H each, both s, H3-14, 15), 4.32 (1H, d, J = 8.0 Hz, H-1′), 3.16 (1H, dd, J = 8.0, 9.0 Hz, H-2′), 3.35 (1H, dd, J = 8.5, 9.0 Hz, H-3′), 3.27 (1H, dd, J = 8.5, 9.0 Hz, H-4′), 3.22 (1H, ddd, J = 2.0, 6.0, 9.0 Hz, H-5′), [3.66 (1H, dd, J = 6.0, 12.0 Hz), 3.85 (1H, dd, J = 2.0, 12.0 Hz), H2-6′]; 13C NMR (CD3OD, 125 MHz) δC: see Table 1; ESI-Q-Orbitrap MS m/z 461.23886 (M + COOH)− (calcd for C22H37O10, 461.23812).
Eurylosesquiterpenoside D (4): White powder; (α)D25 –12.4 (conc 0.55, MeOH); CD (conc 0.002 M, MeOH) mdeg (λnm): −1.04 (227), +0.71 (208), −4.69 (194); IR νmax (KBr) cm−1: 3,356, 2,926, 2,877, 1,072, 1,024; 1H NMR (CD3OD, 500 MHz) δH: 3.44 (1H, dd, J = 4.0, 11.5 Hz, H-1), 1.75 (1H, m, overlapped, Hα-2), 1.95 (1H, m, Hβ-2), 1.48 (1H, dt, J = 4.0, 13.5 Hz, Hα-3), 1.74 (1H, m, overlapped, Hβ-3), 1.87 (1H, m, H-5), 5.56 (1H, br. s, H-6), 1.93 (1H, m, Hα-8), 1.99 (1H, m, Hβ-8), 1.26 (1H, m, Hα-9), 2.05 (1H, m, Hβ-9), 2.28 (1H, sex like, ca. J = 7 Hz, H-11), [3.42 (1H, dd, J = 6.5, 10.0 Hz), 3.55 (1H, dd, J = 7.5, 10.0 Hz), H2-12], 1.03 (3H, d, J = 7.0 Hz, H3-13), 1.01, 1.20 (3H each, both s, H3-14, 15), 4.31 (1H, d, J = 7.5 Hz, H-1′), 3.17 (1H, dd, J = 7.5, 9.0 Hz, H-2′), 3.35 (1H, dd, J = 8.5, 9.0 Hz, H-3′), 3.28 (1H, dd, J = 8.5, 8.5 Hz, H-4′), 3.22 (1H, ddd, J = 2.0, 5.5, 8.5 Hz, H-5′), [3.65 (1H, dd, J = 5.5, 11.5 Hz), 3.85 (1H, dd, J = 2.0, 11.5 Hz), H2-6′]; 13C NMR (CD3OD, 125 MHz) δC: see Table 1; ESI-Q-Orbitrap MS m/z 461.23914 (M + COOH)− (calcd for C22H37O10, 461.23812).
Eurylosesquiterpenol E (5): White powder; (α)D25 –71.8 (conc 1.0, MeOH); UV λmax (MeOH) nm (log ε): 241 (3.93); CD (conc 0.001 M, CH3CN) mdeg (λnm): +3.26 (339), −31.10 (240), +52.31 (206); IR νmax (KBr) cm−1: 3,473, 2,959, 2,935, 2,873, 1,664, 1,452, 1,389, 1,000, 879; 1H NMR (CDCl3, 500 MHz) δH: 1.72 (1H, ddd, J = 3.0, 10.5, 14.0 Hz, H-1), 2.41 (1H, dd, J = 14.0, 16.5 Hz, Hα-2), 2.60 (1H, dd, J = 3.0, 16.5 Hz, Hβ-2), 6.89 (1H, br. s, H-5), 2.40 (1H, m, H-6), 1.16 (1H, m, H-7), 1.52 (2H, m, H2-8), 1.77 (1H, m, Hα-9), 1.45 (1H, m, Hβ-9), 2.25 (1H, m, H-11), 0.85, 0.98 (3H each, both d, J = 7.0 Hz, H3-12, 13), 1.19 (3H, s, H3-14), 1.78 (3H, br. s, H3-15); 1H NMR (C5D5N, 500 MHz) δH: 1.70 (1H, ddd, J = 4.0, 10.5, 14.0 Hz, H-1), 2.79 (1H, dd, J = 14.0, 16.5 Hz, Hα-2), 2.84 (1H, dd, J = 4.0, 16.5 Hz, Hβ-2), 6.93 (1H, br. s, H-5), 2.68 (1H, m, H-6), 1.13 (1H, tt, J = 3.5, 12.0 Hz, H-7), 1.81 (1H, dq, J = 3.5, 13.5 Hz, Hα-8), 1.47 (1H, dq, J = 3.5, 13.5 Hz, Hβ-8), 1.90 (1H, dt, J = 3.5, 13.5 Hz, Hα-9), 1.41 (1H, dt, J = 3.5, 13.5 Hz, Hβ-9), 2.20 (1H, m, H-11), 0.82, 0.95 (3H each, both d, J = 7.0 Hz, H3-12, 13), 1.27 (3H, s, H3-14), 1.93 (3H, br. s, H3-15); 13C NMR (CDCl3 and C5D5N, 125 MHz) δC: see Table 2; ESI-Q-Orbitrap MS m/z 281.17557 (M + COOH)− (calcd for C16H25O4, 281.17474).
Eurylosesquiterpenol F (6): White powder; (α)D25 –36.0 (conc 0.35, MeOH); CD (conc 0.001 M, CH3CN) mdeg (λnm): +3.26 (339), −31.10 (240), +52.31 (206); UV λmax (MeOH) nm (log ε): 241 (3.93); IR νmax (KBr) cm−1: 3,428, 2,954, 2,931, 2,870, 1,628, 1,454, 1,374, 1,118, 879; 1H NMR (CDCl3, 500 MHz) δH: 1.84 (1H, m, H-1), 2.12 (1H, dd, J = 14.0, 16.0 Hz, Hα-2), 2.77 (1H, dd, J = 3.0, 16.0 Hz, Hβ-2), 6.80 (1H, br. s, H-5), 2.08 (1H, m, H-6), 1.21 (1H, m, H-7), 1.69 (1H, m, Hα-8), 1.22 (1H, m, Hβ-8), 1.86 (1H, m, Hα-9), 1.46 (1H, dt, J = 3.5, 13.0 Hz, Hβ-9), 2.23 (1H, m, H-11), 0.83, 0.99 (3H each, both d, J = 7.0 Hz, H3-12, 13), 1.17 (3H, s, H3-14), 1.79 (3H, br. s, H3-15); 1H NMR (C5D5N, 500 MHz) δH: 2.09 (1H, m, H-1), 2.30 (1H, dd, J = 16.0, 16.0 Hz, Hα-2), 2.28 (1H, dd, J = 2.0, 16.0 Hz, Hβ-2), 6.81 (1H, br. s, H-5), 2.11 (1H, m, H-6), 1.13 (1H, m, H-7), 1.55 (1H, dq, J = 3.5, 13.0 Hz, Hα-8), 1.19 (1H, dq, J = 3.5, 13.0 Hz, Hβ-8), 2.00 (1H, dt, J = 3.5, 13.0 Hz, Hα-9), 1.72 (1H, dt, J = 3.5, 13.0 Hz, Hβ-9), 2.14 (1H, m, H-11), 0.81, 0.92 (3H each, both d, J = 7.0 Hz, H3-12, 13), 1.29 (3H, s, H3-14), 1.92 (3H, br. s, H3-15); 13C NMR (CDCl3 and C5D5N, 125 MHz) δC: see Table 2; ESI-Q-Orbitrap MS m/z 281.17548 (M + COOH)− (calcd for C16H25O4, 281.17474).
Eurylosesquiterpenol G. (7): White powder; (α)D25 + 1.7 (conc 0.35, MeOH); CD (conc 0.001 M, CH3CN) mdeg (λnm): −3.07 (240), −4.05 (198); IR νmax (KBr) cm−1: 3,396, 2,958, 2,933, 2,870, 1715, 1,560, 1,454, 1,373, 1,024, 899; 1H NMR (CDCl3, 500 MHz) δH: 1.31 (1H, m, H-1), 2.01 (1H, dt, J = 4.0, 13.0 Hz, Hα-2), 1.55 (1H, dt, J = 4.0, 13.0 Hz, Hβ-2), 4.01 (1H, br. s, H-3), 5.74 (1H, br. s, H-5), 1.92 (1H, t like, ca. J = 11 Hz, H-6), 1.06 (1H, tt, J = 4.0, 12.0 Hz, H-7), 1.49 (1H, m, Hα-8), 1.37 (1H, dq, J = 4.0, 12.0 Hz, Hβ-8), 1.75 (1H, m, Hα-9), 1.45 (1H, dt, J = 4.0, 12.0 Hz, Hβ-9), 2.19 (1H, m, H-11), 0.80, 0.93 (3H each, both d, J = 7.0 Hz, H3-12, 13), 1.24 (3H, s, H3-14), 1.81 (3H, br. s, H3-15); 1H NMR (C5D5N, 500 MHz) δH: 1.83 (1H, ddd, J = 3.0, 10.5, 13.0 Hz, H-1), 2.06 (1H, dt, J = 3.0, 13.0 Hz, Hα-2), 2.49 (1H, dt, J = 3.0, 13.0 Hz, Hβ-2), 4.34 (1H, br. s, H-3), 5.92 (1H, br. s, H-5), 2.46 (1H, dd like, ca. J = 11, 11 Hz, H-6), 1.10 (1H, m, H-7), 1.48 (2H, m, H2-8), 1.99 (2H, m, H2-9), 2.25 (1H, m, H-11), 0.86, 0.92 (3H each, both d, J = 7.0 Hz, H3-12, 13), 1.45 (3H, s, H3-14), 2.08 (3H, br. s, H3-15); 13C NMR (CDCl3 and C5D5N, 125 MHz) δC: Table 2; ESI-Q-Orbitrap MS m/z 283.19107 (M + COOH)− (calcd for C16H27O4, 283.19039).
Eurylosesquiterpenol H (8): White powder; (α)D25 −4.7 (conc 0.65, MeOH); IR νmax (KBr) cm−1: 3,393, 2,954, 2,927, 2,870, 1,454, 1,375, 1,094, 1,024; 1H NMR (CDCl3, 500 MHz) δH: 4.70 (1H, br. d, ca. J = 9 Hz, H-2), 1.60 (1H, m, overlapped, Hα-3), 1.83 (1H, m, Hβ-3), 2.69 (1H, m, H-4), 2.03 (1H, m, H-5), 1.43 (1H, m, Hα-6), 1.47 (1H, dd like, ca. J = 15, 15 Hz, Hβ-6), 1.68 (1H, m, overlapped, H-7), 1.91 (1H, m, Hα-8), 1.68 (1H, m, overlapped, Hβ-8), 2.14 (1H, t like, ca. J = 13 Hz, Hα-9), 1.61 (1H, m, overlapped, Hβ-9), 1.17, 1.30, 1.10 (3H each, all s, H3-12, 13, 14), 0.89 (3H, d, J = 7.0 Hz, H3-15); 1H NMR (C5D5N, 500 MHz) δH: 5.05 (1H, d, J = 6.0 Hz, H-2), 1.95 (2H, m, H2-3), 2.99 (1H, m, H-4), 2.25 (1H, m, H-5), 1.38 (1H, dd like, ca. J = 8, 14 Hz, Hα-6), 1.54 (1H, dd like, ca. J = 14, 14 Hz, Hβ-6), 1.61 (1H, m, H-7), 1.88 (1H, m, Hα-8), 1.70 (1H, m, Hβ-8), 2.43 (1H, ddd, J = 5.0, 13.0, 18.0 Hz, Hα-9), 1.67 (1H, m, Hβ-9), 1.20, 1.31, 1.44 (3H each, all s, H3-12, 13, 14), 0.91 (3H, d, J = 7.5 Hz, H3-15); 13C NMR (CDCl3 and C5D5N, 125 MHz) δC: see Table 2; ESI-Q-Orbitrap MS m/z 317.19626 (M + COOH)− (calcd for C16H29O6, 317.19587).
Eurylosesquiterpenol I (9): White powder; (α)D25 −3.2 (conc 0.25, MeOH); IRνmax (KBr) cm−1: 3,395, 2,926, 2,861, 1704, 1,455, 1,377; 1H NMR (CDCl3, 500 MHz) δH: 1.92 (1H, m, H-1), 1.76 (1H, m, overlapped, Hα-2), 1.43 (1H, m, overlapped, Hβ-2), 1.59 (1H, m, overlapped, Hα-3), 1.74 (1H, m, overlapped, Hβ-3), 1.43 (1H, m, overlapped, H-5), 0.47 (1H, dd, J = 10.0, 10.5 Hz, H-6), 0.69 (1H, ddd, J = 5.5, 10.0, 15.0 Hz, H-7), 1.99 (1H, m, overlapped, Hα-8), 1.12 (1H, m, overlapped, Hβ-8), 1.97 (1H, m, overlapped, Hα-9), 1.58 (1H, m, overlapped, Hβ-9), 2.20 (1H, dt, J = 3.0, 11.0 Hz, H-10), 1.06, 1.09, 1.26 (3H each, all s, H3-12, 13, 15); 1H NMR (C5D5N, 500 MHz) δH: 2.29 (1H, m, H-1), 2.10 (1H, m, Hα-2), 1.93 (1H, m, Hβ-2), 1.70 (1H, ddd, J = 6.0, 8.0, 15.5 Hz, Hα-3), 2.05 (1H, ddd, J = 4.5, 8.0, 15.5 Hz, Hβ-3), 1.83 (1H, dd, J = 10.0, 10.5 Hz, H-5), 0.56 (1H, dd, J = 10.0, 10.5 Hz, H-6), 0.67 (1H, ddd, J = 6.5, 10.0, 15.5 Hz, H-7), 1.96 (1H, m, Hα-8), 1.23 (1H, m, Hβ-8), 2.22 (1H, m, Hα-9), 1.91 (1H, m, Hβ-9), 2.53 (1H, dt, J = 3.0, 10.5 Hz, H-10), 1.08, 1.25, 1.45 (3H each, all s, H3-12, 13, 15); 13C NMR (CDCl3 and C5D5N, 125 MHz) δC: see Table 2; ESI-Q-Orbitrap MS m/z 251.16508 (M—H)− (calcd for C15H23O3, 251.16417).
Acid Hydrolysis of 1–4
A solution of compounds 1–4 (1.0 mg each) in 1 M HCl (1 ml) was heated under reflux for 3 h, the reaction product was extract with EtOAc. The aqueous layer was analyzed by using HPLC [column: Kaseisorb LC NH2-60-5, 4.6 mm i. d. × 250 mm (Tokyo Kasei Co., Ltd, Tokyo, Japan); mobile phase: CH3CN-H2O (80:20, v/v); flow rate: 0.8 ml/min]. As results, d-glucose was identified from 1–4 by comparing their retention time and optical rotation with that of authentic d-glucose (tR: 10.4 min, positive optical rotation).
Computations
Relative configurations of compounds 4–7 were deduced by analyses of their 1D and 2D NMR data assisted by Chem3D modeling. Conformation search was then firstly accomplished under the MMFF94 force field by using CONFLEX 8 software (Takanawa, 2019), and the low energy conformers, which meet the requirements of NOESY analysis, were selected out for further computations. To verify the stabilities of the selected conformers, geometry optimizations and the frequencies pre-calculations were finished by DFT method at the APFD/6-311+G(2d,p) basis set level in methanol (for 4) or acetonitrile (for 5–7), using Gaussian 16 package (Revision C.01) (Frisch et al., 2019). By TD-SCF/DFT method, energies of one hundred excitation states of the optimized conformers were then calculated at the APFD/6-311+G(2d,p) level with a IEFPCM solvent model in MeOH or acetonitrile. With a half bandwidth of ∼0.2 eV, the calculation results were Boltzmann averaged to simulate the ECD spectra after UV correction, which were finally extracted by GaussView 6.0 and Origin Pro 2016 software before comparing with those experimental data.
Experimental Procedures for Bioassay
Materials
Cell viability was measured on BioTek Cytation five-cell imaging multi-mode reader (Winooski, VT, United States); Light damage model and radiation dose were tested on UVB radiation machine (SH4B, Sigma, Shanghai, China) and UVB radiometer (ST90-UVB, 297 nm, Beijing, China), respectively. Dry thermostat (Hangzhou Allsheng Instrusment Co., Itd. Hangzhou, China), western blot electrophoresis and membrane transfer instrument (Bio Rad, United States), and Amersham imager 600 gel imaging system (GE Healthcare, United States) were used to western blot assay.
HaCaT cell lines were gained from Procell Life Science & Technology Co., Ltd. (Wuhan, China); Fetal bovine serum (FBS) was obtained from Biological Industries (Beit-Haemek, Israel); Minimum essential medium (MEM) was ordered from Corning (Shanghai, China); Penicillin and streptomycin were purchased from Thermo Fisher Scientific (Waltham, MA, United States); MTT and dimethyl sulfoxide (DMSO) were gained from Sigma-Aldrich (St. Louis, MO, United States); Vitamin C (Vc) were purchased from Shanghai Yuanye Bio-Technology Co., Ltd. (Shanghai, China); BCA protein quantification kit was ordered from Thermo Fisher Scientific (Waltham, United States); PVDF membrane was purchased from Merch/Millipore (Schwalbach, Germany); Immobilon western chemilumescent HRP substrate was gained from Millipore (Massachusetts, United States); TNF-α (ab6671), COX-2 (ab52237), β-actin (ab8227) JNK (ab208035), and p-JNK (ab4821) were ordered from abcam (Cambs, United Kingdom); p38 (8690S), p-p38 (4511S), ERK (4695S), p-ERK (4370S), and COL1A1 (72026S) were purchased from CST (Massachusetts, United States); MMP-1 (SC-137044) was obtained from Santa Cruz Biotech.INC. (United States).
Cell Culture
HaCaT cells were maintained in MEM medium with 10% FBS, streptomycin (100 μg/ml), penicillin (100 U/mL), and incubated at 5% CO2, 37°C. When the cells grew to 80% confluence, they were seeded in 96-well plates at 1 × 104 cells/well, and then processed the treatment.
Cell Viability Assay
MTT assay was applied to test cell viability. HaCaT cells were incubated at 96-well plates and treated with or without test samples for 24 h, respectively. The culture condition was similar to “Cell Culture.” The medium was removed, then 1% MTT (5 mg/ml) were added into wells to format formazan. After incubating 4 h, the supernatant was removed, then 100 μL dimethyl sulfoxide (DMSO) was added in each well to dissolve the formazan. The absorbance was measured with a microplate reader at 490 nm.
Selection of Ultraviolet B Radiation Dose
After being cultured with MEM medium containing 10% FBS, streptomycin (100 μg/ml), penicillin (100 U/ml) in 96-well plates until grown to 70% confluence, the HaCaT cells were covered with fresh medium for 24 h. Then, the fresh medium was replaced with 100 μL/well PBS, and the cells were exposed to 50, 75, 100, 125, and 150 mJ/cm2 of UVB, respectively. After irradiation, 100 μL/well PBS was removed, and the cells were cultured with 100 μL/well fresh medium for 24 h again. The cell viability was tested in line with “Cell Viability Assay.”
Cell Viabilities of Ultraviolet B Induced HaCaT Cells Pretreated With Compounds
HaCaT cells were seeded in 96-well culture plates with complete medium until grown to 70% confluence, and then treated with fresh medium containing various concentrations of samples (10, 25, and 50 μM) for 24 h. Then, the cells were irradiated with UVB at 125 mJ/cm2 (UVB-irradiated with 0.46 mW cm−2 s−1 for approximately 272 s) in 100 μL PBS. After irradiation, the PBS was immediately replaced by 100 μL fresh medium and incubated for 24 h. Finally, the cell viability was measured by using the same method as that described in the part of “Cell Viability Assay.”
Western Blot Assay
Protein isolation and western blot assay were performed as previously described (Han et al., 2021). Briefly, protein was subjected to SDS-PAGE with 10% or 15% resolving gel, then the proteins on gels were separated, and electrotransferred onto PVDF membranes. Which were incubated at 4°C overnight with primary antibodies against rabbit polyclonal to TNF-α (1:500), COX-2 (1:1,000), β-actin (1:1,000), p38 (1:1,000), p-p38 (1:1,000), ERK (1:1,000), p-ERK (1:1,000), JNK (1:1,000), p-JNK (1:1,000), COL1A1 (1:1,000); and mouse polyclonal to MMP-1 (1:500). After washing with PBST, the PVDF membranes were incubated with a horseradish peroxidase-labeled secondary goat anti-rabbit (1:10,000) antibody (Abcam) or horseradish peroxidase-labeled secondary goat anti-mouse (1:10,000) antibody (Zhongshan Goldbridge Biotechnolog) for 1 h at room temperature, and washed again. Eventually, PVDF membranes were incubated with immobilon western chemilumescent HRP substrate and then scanned with ChemiDoc MP Imaging System to form images. The protein bands were analyzed with the ImageJ software (Version 1.0, National Institutes of Health, Bethesda, MD, United States). The treatment groups were normalized to Nor. Three independent assays were necessary.
Statistical Analysis
All experimental results were presented as the means ± standard error of mean (SEM). SPSS 26.0 was used to conduct the statistics of all data. Unpaired Student’s t-test (when two groups were analyzed) and one-way analysis of variance (ANOVA) (for > 3 groups) were used to analyze results. p < 0.05 was considered to indicate a statistically significant difference.
Conclusion
In summary, in the process of investigating photoprotective constituents from natural products, nine new sesquiterpenes, named as eurylosesquiterpenosides A–D (1–4), eurylosesquiterpenols E–I (5–9), together with ten known ones were obtained and identified from the 70% EtOH extract of O. elatus stems. Though the diverse ingredients such as volatile oil, phenolic acids, lignans, quinic acid esters, anthraquinones, steroids, and aliphatic compounds had been reported from the medicine (Yan et al., 2021), the sesquiterpenes were rarely found in it, which enriched its material base.
Furthermore, our study suggested that the underlying mechanism of active-sesquiterpenes might be relevance with down-regulating MMP-1 expression via the decreasing production of inflammatory mediators and cytokines in UVB-irradiated HaCaT cells.
Data Availability Statement
The original contributions presented in the study are included in the article/Supplementary Material, further inquiries can be directed to the corresponding authors.
Author Contributions
Data collection: JY, MH, and YH; design of the study and write the manuscript: YZ and TW; corrected the data and reviewed literatures: DZ, FS, and HC; perfected the language: JR and JH. All authors discussed, edited, and approved the final version.
Funding
This work was financially supported by the Programs for National Natural Science Foundation of China (No. 82074118), grants from Tianjin Sci-Tech Projects (No. 18ZXXYSY00060), and Important Drug Development Fund, Ministry of Science and Technology of China (No. 2018ZX09735002).
Conflict of Interest
The authors declare that the research was conducted in the absence of any commercial or financial relationships that could be construed as a potential conflict of interest.
Publisher’s Note
All claims expressed in this article are solely those of the authors and do not necessarily represent those of their affiliated organizations, or those of the publisher, the editors and the reviewers. Any product that may be evaluated in this article, or claim that may be made by its manufacturer, is not guaranteed or endorsed by the publisher.
Supplementary Material
The Supplementary Material for this article can be found online at: https://www.frontiersin.org/articles/10.3389/fchem.2021.766041/full#supplementary-material
References
Ando, M., Arai, K., Kikuchi, K., and Isogai, K. (1994). Synthetic Studies of Sesquiterpenes with a Cis-Fused Decalin System, 4. Synthesis of (+)-5βh-Eudesma-3,11-Diene, (-)-5βh-Eudesmane-4β,11-Diol, and (+)-5βh-Eudesmane-4α,11-Diol, and Structure Revision of a Natural Eudesmane-4,11-Diol Isolated from Pluchea Arguta. J. Nat. Prod. 57, 1189–1199. doi:10.1021/np50111a001
Beechan, C. M., Djerassi, C., and Eggert, H. (1978). Terpenoids-LXXIV. Tetrahedron 34, 2503–2508. doi:10.1016/0040-4020(78)88378-1
Cavinato, M., and Jansen-Dürr, P. (2017). Molecular Mechanisms of UVB-Induced Senescence of Dermal Fibroblasts and its Relevance for Photoaging of the Human Skin. Exp. Gerontol. 94, 78–82. doi:10.1016/j.exger.2017.01.009
Choi, H.-J., Alam, M. B., Baek, M.-E., Kwon, Y.-G., Lim, J.-Y., and Lee, S.-H. (2020). Protection against UVB-Induced Photoaging by Nypa fruticans via Inhibition of MAPK/AP-1/MMP-1 Signaling. Oxidative Med. Cell Longevity 2020, 1–14. doi:10.1155/2020/2905362
Ding, Y., Kim, J.-A., Yang, S.-Y., Kim, W.-K., Lee, S.-H., Jang, H.-D., et al. (2011). Antioxidative Sesquiterpenes from Artemisia Iwayomogi. Bull. Korean Chem. Soc. 32, 3493–3496. doi:10.5012/bkcs.2011.32.9.3493
Elmasri, W. A., Hegazy, M.-E. F., Mechref, Y., and Paré, P. W. (2016). Structure-antioxidant and Anti-tumor Activity of Teucrium Polium Phytochemicals. Phytochemistry Lett. 15, 81–87. doi:10.1016/j.phytol.2015.11.007
Frisch, M. J., Trucks, G. W., Schlegel, H. B., Scuseria, G. E., Robb, M. A., Cheeseman, J. R., et al. (2019). Gaussian 16 vC.01 (Revision C.01). Wallingford CT: Gaussian, Inc.
Han, Y., Cheng, D., Hao, M., Yan, J., Ruan, J., Han, L., et al. (2021). The Phenolic Acids from Oplopanax Elatus Nakai Stems and Their Potential Photo-Damage Prevention Activity. J. Nat. Med. doi:10.1007/s11418-021-01546-6
Kesselmans, R. P. W., Wijnberg, J. B. P. A., Minnaard, A. J., Walinga, R. E., and De Groot, A. (1991). Synthesis of All Stereoisomers of Eudesm-11-En-4-Ol. 2. Total Synthesis of selin-11-en-4.alpha.-ol, Intermedeol, Neointermedeol, and Paradisiol. First Total Synthesis of Amiteol. J. Org. Chem. 56, 7237–7244. doi:10.1021/jo00026a012
Kuo, Y.-H., Chyu, C.-F., and Lin, H.-C. (2003). Cadinane-type Sesquiterpenes from the Roots of Taiwania Cryptomerioides HAYATA. Chem. Pharm. Bull. 51, 986–989. doi:10.1248/cpb.51.986
Li, Y., Fang, L., Bi, F., Zhang, C., and Zheng, G. (20062006). Total Synthesis of 4α,5α,10β-Trihydroxycadinane and its C4-Isomer: -Structural Revision of a Natural Sesquiterpenoid. Synlett 2006, 2655–2657. doi:10.1055/s-2006-951480
Moreira, I. C., Lago, J. H. G., Young, M. C. M., and Roque, N. F. (2003). Antifungal Aromadendrane Sesquiterpenoids from the Leaves of Xylopia Brasiliensis. J. Braz. Chem. Soc. 14, 828–831. doi:10.1590/S0103-50532003000500020
Muñoz, O., Galeffi, C., Federici, E., Garbarino, J. A., Piovano, M., and Nicoletti, M. (1995). Boarioside, a Eudesmane Glucoside from Maytenus Boaria. Phytochemistry 40, 853–855. doi:10.1002/chin.19960919310.1016/0031-9422(95)00348-b
Nugroho, A. E., and Morita, H. (2014). Circular Dichroism Calculation for Natural Products. J. Nat. Med. 68, 1–10. doi:10.1007/s11418-013-0768-x
Ono, M., Yamashita, M., Mori, K., Masuoka, C., Eto, M., Kinjo, J., et al. (2008). Sesquiterpenoids, Triterpenoids, and Flavonoids from the Fruits of Schinus Molle. Fstr 14, 499–508. doi:10.3136/fstr.14.499
Parrado, C., Mascaraque, M., Gilaberte, Y., Juarranz, A., and Gonzalez, S. (2016). Fernblock (Polypodium Leucotomos Extract): Molecular Mechanisms and Pleiotropic Effects in Light-Related Skin Conditions, Photoaging and Skin Cancers, a Review. Ijms 17, 1026. doi:10.3390/ijms17071026
Peng, Z., Chen, B., Zheng, Q., Zhu, G., Cao, W., Qin, X., et al. (2020). Ameliorative Effects of Peptides from the Oyster (Crassostrea Hongkongensis) Protein Hydrolysates against UVB-Induced Skin Photodamage in Mice. Mar. Drugs 18, 288. doi:10.3390/md18060288
Pereira, M., da Silva, T., Lopes, L., Krettli, A., Madureira, L., and Zukerman-Schpector, J. (2012). 4,5-Seco-guaiane and a Nine-Membered Sesquiterpene Lactone from Holostylis Reniformis. Molecules 17, 14046–14057. doi:10.3390/molecules171214046
Pittayapruek, P., Meephansan, J., Prapapan, O., Komine, M., and Ohtsuki, M. (2016). Role of Matrix Metalloproteinases in Photoaging and Photocarcinogenesis. Ijms 17, 868. doi:10.3390/ijms17060868
Rittié, L., and Fisher, G. J. (2002). UV-light-induced Signal Cascades and Skin Aging. Ageing Res. Rev. 1, 705–720. doi:10.1016/s1568-1637(02)00024-7
Ro Lee, K., Kyun Lee, I., Hyun Kim, K., and Yong Ryu, S. (2009). Two New Sesquiterpene Glucosides from Gymnaster Koraiensis. Heterocycles 78, 2827–2835. doi:10.3987/COM-09-11773
Shimoma, F., Kondo, H., Yuuya, S., Suzuki, T., Hagiwara, H., and Ando, M. (1998). Enantioselective Total Syntheses of (−)-7βh-Eudesmane-4α,11-Diol and (+)-Ent-7βh-Eudesmane-4α,11-Diol. J. Nat. Prod. 61, 22–28. doi:10.1021/np9702906
Shin, H. J., Lee, S. Y., Kim, J. S., Lee, S., Choi, R. J., Chung, H. S., et al. (2012). Sesquiterpenes and Other Constituents from Dendranthema Zawadskii Var. Latilobum. Chem. Pharm. Bull. 60, 306–314. doi:10.1002/chin.20123220810.1248/cpb.60.306
Soliman, H. S., El-Dib, R., Shalaby, N. M., Duddeck, H., Simon, A., and Tóth, G. (2007). Isolation and Structure Determination of Compounds from Stachys Yemenensis Hedge. Nat. Product. Commun. 2, 1934578X0700201–980. doi:10.1177/1934578X0700201003
Takanawa, Minato-ku. (2019). CONFLEX 8 Rev. B. Tokyo, Japan. Available at: http://www.conflex.co.jp/.
Wu, C.-L., Chien, S.-C., Wang, S.-Y., Kuo, Y.-H., and Chang, S.-T. (2005). Structure-activity Relationships of Cadinane-type Sesquiterpene Derivatives against wood-decay Fungi. Holzforschung 59, 620–627. doi:10.1515/HF.2005.100
Xiao, Y.-C., Ye, L., Zhao, M.-X., Yan, C.-Q., Wang, W., Huang, Q.-S., et al. (2016). Two New Sesquiterpene Glycosides Isolated from the Fresh needles of Pinus Massoniana Lamb. Nat. Product. Res. 31, 341–346. doi:10.1080/14786419.2016.1239089
Yan, J. J., Hao, M. M., Zhao, W., Ruan, J. Y., Wang, T., and Zhang, Y. (2021). Research Progress on Chemical Constituents and Pharmacological Activity of Oplopana Elatus. Tianjin Zhongyiyao Daxue Xuebao 40, 260–266.
Yang, H. W., Jung, Y., Kim, H. D., and Kim, J. (2020). Ribosomal Protein S3-Derived Repair Domain Peptides Regulate UV-Induced Matrix Metalloproteinase-1. Biochem. Biophysical Res. Commun. 530, 149–154. doi:10.1016/j.bbrc.2020.06.094
Yoshikawa, M., Morikawa, T., Zhang, Y., Nakamura, S., Muraoka, O., and Matsuda, H. (2007). Megastigmanes and Their Glucosides from the Whole Plant of Sedum Sarmentosum. J. Nat. Prod. 70, 575–583. doi:10.1021/np068059s
Zhao, Y., Yue, J.-M., He, Y.-N., Lin, Z.-W., and Sun, H.-D. (1997). Eleven New Eudesmane Derivatives from Laggera Pterodonta. J. Nat. Prod. 60, 545–549. doi:10.1021/np960456n
Keywords: Oplopanax elatus stems, sesquiterpenes, HaCaT cells, anti-photoaging, matrix metalloproteinase-1, mitogen-activated protein kinase, inflammatory cytokines
Citation: Yan J, Hao M, Han Y, Ruan J, Zheng D, Sun F, Cao H, Hao J, Zhang Y and Wang T (2021) Sesquiterpenes From Oplopanax elatus Stems and Their Anti-Photoaging Effects by Down-Regulating Matrix Metalloproteinase-1 Expression via Anti-Inflammation. Front. Chem. 9:766041. doi: 10.3389/fchem.2021.766041
Received: 03 September 2021; Accepted: 11 October 2021;
Published: 04 November 2021.
Edited by:
Xiaoxiao Huang, Shenyang Pharmaceutical University, ChinaReviewed by:
Ming Bai, Shenyang Pharmaceutical University, ChinaRui Guo, Shanxi Medical University, China
Copyright © 2021 Yan, Hao, Han, Ruan, Zheng, Sun, Cao, Hao, Zhang and Wang. This is an open-access article distributed under the terms of the Creative Commons Attribution License (CC BY). The use, distribution or reproduction in other forums is permitted, provided the original author(s) and the copyright owner(s) are credited and that the original publication in this journal is cited, in accordance with accepted academic practice. No use, distribution or reproduction is permitted which does not comply with these terms.
*Correspondence: Yi Zhang, emh3d3h6aEB0anV0Y20uZWR1LmNu; Tao Wang, d2FuZ3Rhb0B0anV0Y20uZWR1LmNu
†These authors have contributed equally to this work and share first authorship