- College of Furnishings and Industrial Design, Nanjing Forestry University, Nanjing, China
This paper briefly discussed the research progress of biomass-based transparent wood (BBTW), and summarized the key technologies and potential application prospects of BBTW in replacing architectural glass. Based on the introduction of the preparation process of BBTW, the advantages of BBTW and their feasibility to replace architectural glass are illustrated with a view to the requirements and conditions of architectural glass for different use functions. The limitations of BBTW are discussed and the development prospects of BBTW are also prospected. The research shows that BBTW has the advantages of green and renewable materials that can meet the requirements of good lighting conditions, flame retardant, heat insulation and safety, which are in line with the sustainable development trend. Further studies are needed to continuously break through its limitations with an aim to expand the application of this new biomass-based material.
Introduction
Biomass materials are new materials with excellent performance and high added value that are processed and manufactured by a series of high technology means using woody, grassy and vine plants (Sun and Liu, 2019; Wu et al., 2020; Zhao et al., 2020a; 2020b). Biomass materials are widely available and renewable, with low processing costs. In the current situation of depletion of non-renewable resources, the development and utilization of new materials can effectively alleviate the serious problems of energy tension and environmental pollution if renewable biomass materials are used as the “cornerstone” of new materials instead of traditional petrochemical and mineral-based materials (Liu et al., 2019; Wang et al., 2019; Chen L. et al., 2020).
Therefore, the research of biomass materials has received a lot of attention from scholars at home and abroad in recent years, such as transparent wood, wood sponge, straw plate, etc. Among them, biomass-based transparent wood (BBTW), as an emerging result of biomass materials research, have been widely studied for their light weight, light transmission, environmental protection, and high mechanical strength (Zhou and Wu, 2020). BBTW are mainly prepared renewable natural plant materials as raw materials and modifying their intrinsic structures or compounding them with other materials to achieve their light transmission and other functions.
At present, BBTW are still in the development and exploration stage, and the related research is focused on the preparation, but not yet put into actual production. The current researchs for BBTW are mainly focused on replacing architectural glass, luminescent materials, energy storage, battery substrates and other fields. This paper briefly discussed the research progress of BBTW, and summarizes the key technologies and potential application prospects of BBTW in replacing architectural glass.
Preparation of BBTW and Light Transmission Principle
The development history of BBTW can be divided into three stages. 1) The first stage is the pre-exploration of the BBTW. In 1998, (Murayama et al., 1998) were the first to report the use of nanocellulose to prepare a translucent paper with high tensile strength. Following this innovation, many domestic and foreign scholars have initiated research on transparent paper. However, the process of preparing transparent paper has limitations such as high energy consumption and time consuming. 2) The second stage is the period of rapid development of BBTW. A new type of transparent wood (TW) was reported by Liangbing Hu’s team in 2016. As a result, the preparation of TW gradually became a research hotspot. 3) The third stage is the period of improvement and enhancement of the transparency of TW. In recent years, many scholars have also conducted research on the transparency of TW other than wood and proposed many methods to improve the performance of BBTW.
Natural wood contains lignin and other light-absorbing components, and the porous structure of the wood will scatter visible light, resulting in opacity of the wood, which can not meet the demand for lighting and can not be used as a building glass material. There are two main reasons for the opacity of wood: 1) The wood contains a large amount of light-absorbing substance that is lignin, which accounts for 20–30% of the total weight of wood; 2) The porosity of wood is as high as 30–80%, and a large number of pores have diameters larger than the wavelength of visible light (380–780 nm), which will cause severe light scattering. Therefore, by removing the lignin in the wood, it is possible to remove the chromogenic substances while retaining the wood skeleton structure. The pore structure in wood mainly includes a microcapillary system (mainly formed by the dynamic connection of tiny pores with a size below 10 nm in the fine running wall) and a large capillary system (mainly composed of conduits and screens). These capillary channels are connected to each other, so a transparent resin with a very high refractive index matching with the cellulose can be injected into it to fill the pores in the wood, thereby achieving transparency.
As shown in Table 1, BBTW were prepared by different preparation methods. The preparation of BBTW is divided into two main steps, preparation of delignified biobased templates (DBT) and impregnation of resins with matching refractive indices. The main methods for preparing DBT are acid, alkali, bioenzymatic and lignin modification methods. Sodium hypochlorite solution is usually used as a high frequency reagent for acid delignification. Sodium hypochlorite destroys the aromatic ring structure of lignin by oxidation and chlorination, thus achieving the effect of lignin removal. The most commonly used reagent for alkali delignification is a mixture of sodium hydroxide and sodium sulfite solution. In addition to the common treatment with sodium hydroxide solution, other alkaline solutions have also been used. Biological enzymatic delignification is a green delignification process, which degrades lignin by enzymes in order to achieve the purpose of delignification. By modifying the lignin, the chromophore groups are removed to achieve bleaching. After obtaining DBT, it is necessary to impregnate it with a resin matching its refractive index to obtain BBTW. The commonly used resins are: methyl methacrylate (MMA), epoxy resin, polyvinyl alcohol (PVA), and polyvinyl pyrrolidone (PVP). The choice of resins is not limited to the requirement of a high refractive index match with DBT, but is also increasingly focused on the environmental and physical properties of the resin itself. Current polymers are mainly petroleum-based products. One of the challenges is to replace fossil polymers in the manufacture of sustainable TW. Recently, Montanari et al. (2021) have successfully developed an environmentally friendly alternative: limonene acrylate, a monomer made from limonene. A substance extracted from orange juice is used to make a polymer that restores the strength of the delignification and allows light to pass through, producing BBTW.
Requirements and Limitations of Architectural Glass
Requirements for Flat Glass in Architectural Glass
GB116114-2009 (China National Standardization Administration Committee 2010) provides relevant regulations on some properties of flat glass in building glass doors and windows. The standard specifies the minimum value of visible light transmission ratio of flat glass of different nominal thickness in architectural glass. The transmission ratio, also known as transparency, transmittance or transmission coefficient, is the ratio of transmitted luminous flux to incident luminous flux (Wang et al., 2020). As shown in Table 2, the minimum value of the visible transmittance ratio is 89% when the nominal thickness of flat glass is 2 mm, and as the nominal thickness increases, the minimum value of visible transmittance ratio is gradually reduced, and the minimum value of visible transmittance ratio should reach 67% when the engineering thickness of flat glass reaches 25 mm.
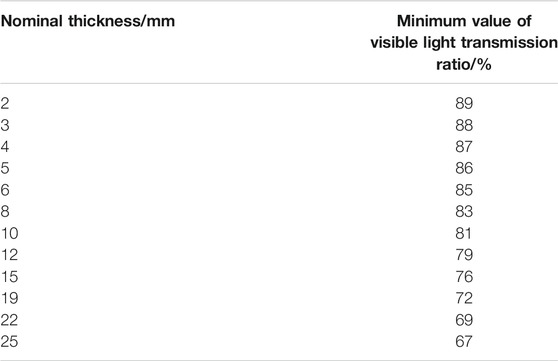
TABLE 2. Minimum visible light transmittance of colorless transparent flat glass (Wang et al., 2020).
Requirements for Fireproof Glass in Architectural Glass
GB15763 ″Safety Glass for Buildings” (China National Standardization Administration Committee 2009a) contains the following four types of glass: fireproof glass, tempered glass, laminated glass and homogeneous tempered glass. As shown in Table 3, the fire resistance performance is specified in GB15763.1-2009 “Fireproof Glass” (China National Standardization Administration Committee 2009b).

TABLE 3. Fire-resistant properties of fireproof glass (China National Standardization Administration Committee 2009b).
And the standard also specifies the impact resistance of single fire glass. Composite fire glass is not destroyed means that the glass after the test to meet one of the following conditions: first, the glass is not broken; second, the glass is broken but the steel ball did not penetrate the specimen (China National Standardization Administration Committee 2009a).
Requirements of Thermal Insulation Glass for Building
JC/T2304-2015 Technical conditions of thermal insulation glass for building (Ministry of Industry and Information Technology of the People’s Republic of China 2015) stipulates that there are three types of thermal insulation glass for building, which are thermal insulation type BW, thermal insulation type GR, thermal insulation type BG. As shown in Table 4, for the performance of three different types of thermal insulation glass, this standard stipulates its conduction coefficient (K), light to heat ratio (LSG), solar radiation spectrum transmittance ratio (GIR).
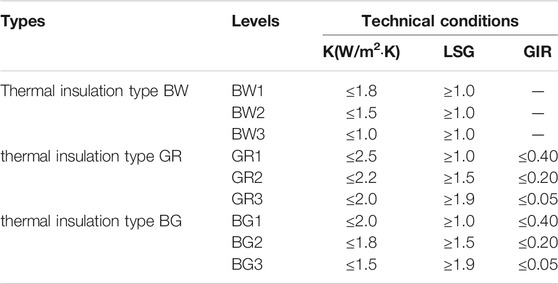
TABLE 4. Technical conditions for insulating glass (Ministry of Industry and Information Technology of the People’s Republic of China 2015).
Limitations of Architectural Glass
Architectural glass have many advantages, such as high transmittance, strong sense of fashion, good permeability, rich modeling, wide application, etc. However, there are some limitations of glass windows and doors, specifically in the following aspects.
First, they can produce discomfort glare to threaten public safety. Glare is the visual discomfort caused by the extreme contrast of brightness. The human eye can not adapt to this unsuitable brightness distribution, which may give people a sense of nausea and discomfort. In cities, many buildings are decorated with large-format glass windows on their facades, although when the light is strong, the glare from glass windows may be directed to the eyes of surrounding pedestrians and vehicle drivers, causing visual fatigue and even causing traffic accidents.
Second, the high brittleness of glass will make the cracks in glass very easy to expand by the fact of loosen in the crystal structure due to the less dense of crystallization with coarse grains. Therefore, in the handling, installation, and use process of glass, when accidental colliding happens, it is easy to produce fragments, threatening people’s safety.
Third, there are drawbacks in the production process of flat glass industry. The SO2, NOx emission reduction pressure is still greater, and the energy saving and emission reduction technology needs to be improved (Zhao et al., 2017). As China’s flat glass environmental protection facilities put into use for a relatively short period of time, the periodically “change fire” operation of kiln in the production process always results in unstable concentration of exhaust gas emissions.
Advantages and Limitations of BBTW as Alternative Glass Materials
Advantages of BBTW as Alternative Glass Materials
Optical Properties of BBTW Meet the Requirements of Architectural Glass
BBTW should not only meet the basic requirements of glass when used in place of glass for architectural glass, as shown in Figure 1 (blow), Zhu et al., 2016 prepared 2 mm TW with a transparency of up to 90%, and this study met the requirements of GB116114-2009 on the visible light transmission ratio of flat glass. Therefore, through certain means and techniques, the light transmission ratio of BBTW can reach the requirements of architectural glass.
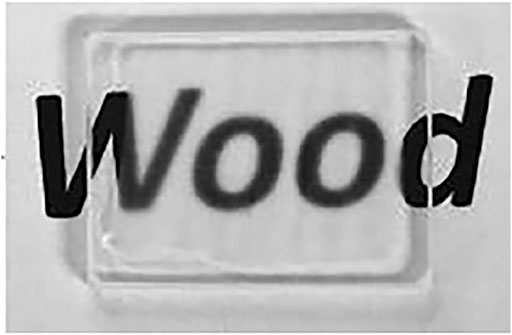
FIGURE 1. Macroscopic observation diagram of TW (Zhu et al., 2016).
BBTW also need to compensate on the disadvantages and shortcomings of glass. As shown in Figure 2 (blow), Li et al., 2016 compared glass and TW against the Sun; glass produces glare due to its extremely uneven brightness, and glare interferes with the clarity of the visual image, while TW has a more uniform and comfortable light due to its uniform texture.
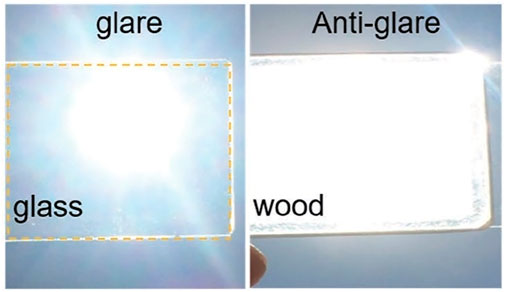
FIGURE 2. Glare contrast between glass and TW (Li et al., 2016).
As shown in Figure 3 (blow), Li et al., 2016 simulated the skylight of a building with glass and TW, and chose six different locations respectively. With glass as the skylight material, the light intensity in the brightest part of the house was 35 times greater than that in the darkest part, and the light was clearly uneven. On the contrary, the difference in light intensity between the brightest and the darkest parts of the house was only 2.3 times when TW was used as the skylight material.
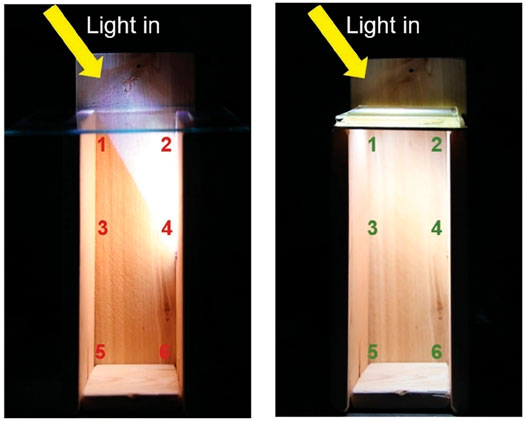
FIGURE 3. Light contrast between glass and TW (Li et al., 2016).
BBTW Is Safer and has Excellent Mechanical Properties
As shown in Figure 4 (blow), glass immediately shatters into sharp fragments when struck by a falling sharp object. In contrast, even after a sudden impact, TW only bends and splits, rather than shattering into multiple sharp fragments. Therefore, compared to glass materials, BBTW do not have safety hazards caused by shattering. Also, BBTW exhibit excellent mechanical properties. For example, the tensile strength of transparent bamboo (TB) prepared by Wang et al. (2018) reached 92 MPa; the maximum tensile strength of TW prepared by Wu et al. (2019) reached 165.1 ± 1.5 MPa.
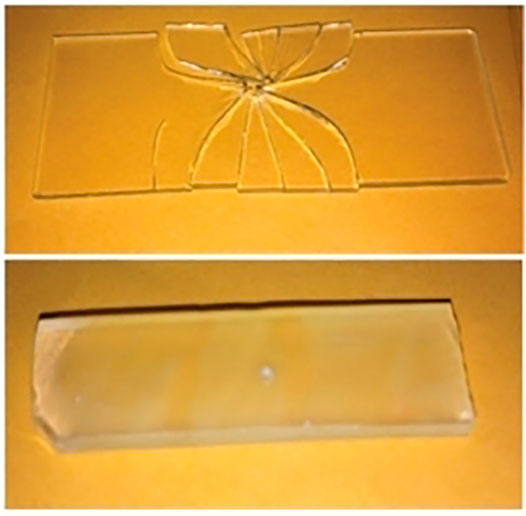
FIGURE 4. Brittle contrast between glass and TW (Li et al., 2016) (Upper right: glass; Lower right: TW).
Green Production Process
Green process technology is in the key part of the whole process of product life cycle and is the key process to make a green product. In the production process of flat glass, the emission of pollutants and the loss of energy are very huge (Zhao et al., 2019). In contrast, the process of biomass material transparency is free from the emission of toxic and harmful gases. For example, the epoxy resin as impregnating material is free from irritating odor. Recently, Montanari et al. (2021) have successfully developed an environmentally friendly alternative: limonene acrylate, a monomer made from limonene. Besides, the biomass used as raw materials have the advantages of being very widely sourced, green and renewable, which are in line with the trend of sustainable development.
Higher Aesthetic Value
Wood materials not only have practical value but also have high aesthetic value in terms of texture, and the patterns formed by the different arrangement of cell distribution are variable (Yin and Liu, 2020). Compared to glass, most wood materials have a unique texture that is maintained after the transparency treatment, and more types of patterns can be achieved by superimposing two layers of transparent wood. As shown in Figure 5 (blow), Ruiyu Mi et al. (2020a) designed various lattice patterns by stacking two layers of TW rotated at opposite angles. In addition, using this universal manufacturing method, the BBTW developed from wood with natural texture can increase the aesthetic value of the building.
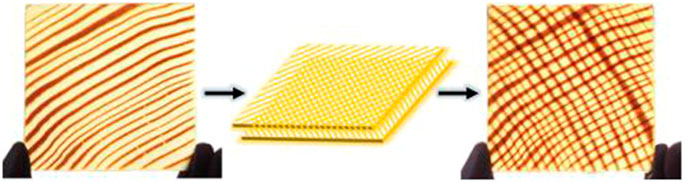
FIGURE 5. Design of TW (Mi et al., 2020a).
Good Thermal Insulation Properties
Zhang Y. et al. (2018) compared the radial thermal conductivity of glass, TW, transparent wood fiber (TWF) and unmodified wood (UW), and the thermal conductivity of glass, TW, TWF and UW were 1.033, 0.164, 0.178 and 0.124 W m−1K −1. The thermal conductivity of TWF and TW was significantly lower than that of glass and slightly higher than that of UW. As shown in Table 5, the thermal conductivity of the transparent materials prepared from different biomass materials were all significantly lower, which fully satisfied the requirements for thermal conductivity of thermal insulation glass in the above Technical Conditions for Thermal Insulation Glass for Buildings. Wang et al. (2018) found that transparent wood has better thermal insulation performance. Under the condition of an external temperature of 4°C, the indoor temperature of a simulated house using transparent wood dropped from 35°C to 20.1°C. At the same time, the simulation using glass The indoor temperature of the house dropped from 35.1°C to 9.1°C. Applying BBTW to replace architectural glass will help slow down internal temperature fluctuations and reduce energy consumption.

TABLE 5. Comparison of thermal conductivity of transparent materials prepared from different materials.
Challenges for BBTW to Replace Architectural Glass
Increasing the Width
At present, the research on the BBTW is still in the laboratory stage, and the size of the prepared BBTW is limited. If BBTW are to be used in architectural glass, the first thing to consider is to increase the width. Since it takes more time to delignify a large size biomass material (e.g. a whole piece of wood or a whole section of bamboo) and it is difficult to fill it completely with resin when impregnating it, it is inevitably more difficult to prepare a whole biomass material directly for direct transparent processing. The whole biomass material can be processed into fibers and the biomass fibers can be transparently treated, which can reduce the preparation difficulty and at the same time can prepare BBTW of arbitrary size to adapt to the dimensional changes of architectural glass in different scenarios. As could be seen from Figure 6 (blow), Wang xuan et al. (2018) prepared TW by this method not only with the size of 300 mm × 300 mm × 10 mm and 68% light transmission, but also with high preparation efficiency, which is suitable for mass production.
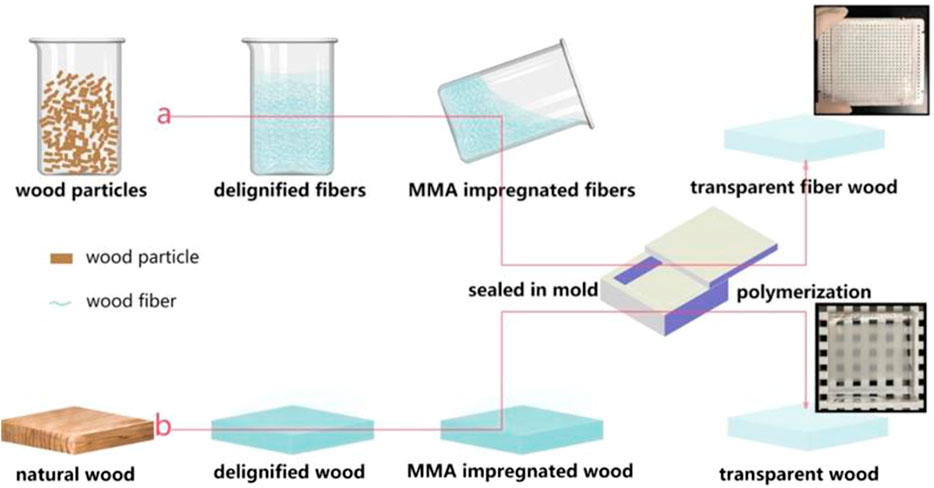
FIGURE 6. Comparison and of the whole wood material transparency and wood fiber transparency process (Wang et al., 2018).
Improve Light Transmission
Although the light transmittance of existing BBTW is being improved, there is still a gap between them and the standard of flat glass used in architectural glass. Therefore, it is crucial to improve the light transmission of BBTW. The reason why the light transmission of BBTW can not reach the standard of flat glass is due to the debonding phenomenon at the interface of various parts of the composite material, i.e., cracks at the interface between the polymer and the cell wall of the biomass material, which leads to light scattering or reflection, thus reducing the light transmission of BBTW. This limitation can be improved by acetylation treatment. Li et al. (2018a) prepared high transmittance BBTW with a thickness of 1.5 mm and 93% light transmission by acetylation treatment of TW (see Figure 7B blow). As shown in Figure 7A, acetylation treatment is the reaction of introducing acetyl CH3CO- on the nitrogen, oxygen, and carbon atoms in the molecules of organic compounds. The acetylation treatment increases the compatibility of the cell wall of the biomass material with the polymer, thus increasing the light transmission.
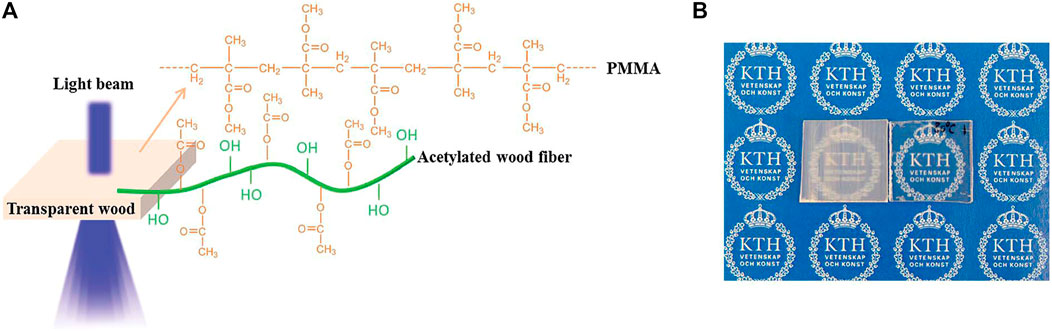
FIGURE 7. (A) Schematic representation showing the structure of the modifified highly transparent wood. The wood template was acetylated to have better compatibility with PMMA. (B) Photograph shows the non-acetylated transparent wood (left) and acetylated transparent wood (right). (Li et at. 2018b).
Improve Flame Retardancy
Flame retardancy is also a key performance indispensable for architectural glass. Improving the flame retardancy of BBTW can be achieved by adding flame retardant materials for fire protection purposes. For example, polyimide can be added to biomass materials in the process of transparency. Polyimide (PI) is a polymer with imide ring on the main chain, which has the advantages of high thermal stability, excellent mechanical strength, radiation resistance, non-toxicity, and self-extinguishing, etc. Chen et al. (2020b) demonstrated that after adding PI, BBTW maintain good self-extinguishing performance within 2 s after leaving the fire source, the reason is that the thermal decomposition temperature of PI is generally 500°C, and the decomposition products are mainly non-polluting, non-combustible nitrogen-containing gases, which can both dilute the concentration of combustible gases and achieve the pursuit of flame retardancy.
Smart Dimming
If BBTW are used to replace architectural glass, they should not only meet some basic performance requirements, but also conform to the current concept and development trend of smart home. For example, it needs to have the function of adjustable haze, so that it can meet the role of privacy protection in certain scenarios and can be switched for different needs and different scenarios. Qiu et al. (2020) prepared TW with thermally reversible optical properties by infiltrating copolymers of monomer styrene (St), butyl acrylate (BA), and octadecene (ODE) into the treated wood. The introduction of ODE chains leads to TW with repeatable optical properties. With increasing temperature, TW can change repeatedly from lower visibility (transmittance: 23.7%, haze: 8.3%) to transparency (transmittance: 74.9%, haze: 36%), with the opposite change occurring during cooling. This technique promotes the potential application of BBTW as a material for smart windows and doors.
Self-Cleaning Properties
In addition to some basic performance, BBTW can also add some other functions to extend the life cycle of BBTW. For example, self-cleaning function is very important. If architectural glass is to be self-cleaning, it needs to be coated with hydrophobic paint on the surface of the glass. Hydrophobic paint is sprayed on the glass surface, and a durable nano protective film can be formed within a few seconds, which plays a role of self-cleaning and care. The unique nano-structured protective film imitates the super hydrophobic and self-cleaning function of the natural lotus leaf. The water droplets on the coating surface slide quickly as if falling on the lotus leaf, and the coating surface is kept clean. However, BBTW itself has self-cleaning properties. For example, the transparent luminous wood prepared by Salhah et al. (2021) has superhydrophobic activity, and the water contact angle of the transparent luminous wood is increased from 151.9° to 162.8°, which has extremely superior self-cleaning characteristics.
UV and IR Shielding Performance
Ordinary architectural glass does not have the ability to resist ultraviolet radiation, which will accelerate the aging of human skin. Antimony-doped tin oxide (ATO) particles have excellent ultraviolet and near-infrared shielding properties, and are usually used in shielding glass. Qiu et al. (2019) used modified antimony-doped tin oxide (ATO) Preparation of ATO/transparent wood. When the addition amount of ATO is 0.7% (wt), the UV shielding effect of ATO/transparent wood is as high as 80%. When applied to windows, it can reduce the damage of ultraviolet rays to human skin.
Future Prospects of BBTW
General Windows and Doors
A well-lit environment can bring more comfortable living experience, while on the contrary, a poorly lit environment can have a negative impact on the human body and mind. Compared with glass, BBTW could meet the conditions of no glare and uniform light exposure, which are important for lighting in houses. BBTW can be used so that artificial lighting can be partially replaced by sunlight to reduce energy (Li et al., 2018b). This initiative is in line with the current concept of green development and sustainability. BBTW are also highly non-toxic and can ensure a more uniform and soft transmission of light, thus protecting interior privacy. However, its application to ordinary windows and doors also needs to be continuously increased and improved light transmission rate, so as to meet the conditions of use of flat glass. Attention should also be paid to the requirement of flame retardancy by adding thermal insulation and fire retardant reagents. The application of BBTW as an alternative to colorless transparent flat glass in architectural glass is promising.
Thermal Insulated Windows and Doors
In addition to ordinary flat glass, BBTW can be used for thermal insulated windows and doors applications. Li et al. (2018a) prepared TW for thermal insulated windows in buildings. Compared to glass windows, transparent wood windows have less temperature change under continuous solar radiation, which contributes to energy saving and environmental protection. The use of transparent cross-laminated wood can be considered to enhance the stability of the structure, but still sufficient thermal resistance (V Karl’a 2019.) Energy-efficient buildings with BBTW as insulated windows and doors consume less energy, which not only significantly reduces electricity consumption, but also promotes natural and comfortable interior lighting.
Intelligent Doors and Windows
With the continuous improvement of people’s living standards, the concept of smart home has come into being, and smart windows and doors are an indispensable part of it (Cao et al., 2018). The application of BBTW in smart windows and doors has the following target directions. First, when the ambient temperature of biomass transparent windows and doors is adjusted, the light transmittance and haze of the windows and doors will change accordingly. For example, when reaching a higher temperature, the light transmittance of windows and doors decreases and the haze increases, which can protect the privacy of users, and when the temperature decreases, the light transmittance of windows and doors increases, the haze decreases and the lighting is good. Secondly, BBTW can be used as a smart door and window to protect against ultraviolet rays to protect the indoor environment and protect the skin health of the occupants, and delay skin aging.
Replacement of Glass Curtain Wall
Building glass curtain wall uses a lot of glass materials, a certain extent that can meet the role of beautifying the appearance of the building, but its disadvantages can not be underestimated. Glass curtain wall will not only cause light pollution, but also security risks. Due to the long-term adverse effects of the natural environment, the structure of the glass curtain wall is easy to aging, resulting in the fall of the glass curtain wall. BBTW not only has aesthetic value, but also has unique texture and color. At the same time, the texture of BBTW is even, even if it is impacted, it will not break into sharp pieces. Due to its excellent optical performance, fire and heat insulation performance and high safety factor, BBTW has a broad prospect in replacing building glass curtain wall.
Conclusion
Compared with the steel and concrete structures commonly used in modern architecture, house components made of wood materials can make occupants closer to nature and more relaxed. At the same time, BBTW meet the requirements of good lighting conditions as well as flame retardancy, heat insulation and safety, and have the advantages of very wide material sources, green and renewable, which are in line with the trend of sustainable development. However, due to issues such as process, time, cost and safety, there is still a certain gap between transparent wood and actual large-scale application. Improving the transparency of large-size materials and finding industrialized production methods are the hotspots and difficulties in the current research on transparent wood. Judging from the current research, two problems need to be solved 1) to achieve large-size and high light transmittance biomass-based transparent wood; 2) to find new ideas for preparation, adding more functions, intelligence, composite and other related content, Such as phase change energy storage, electric heating, flame retardant, self-cleaning, smart dimming, decoration, etc. The application of biomass-based transparent wood in architectural glass need gain more experience that would be replicable and scalable in the future with an aim to achieve large-scale and batch production and achieve sustainable development.
Author Contributions
WJ ang ZJ contributed to conception and design of the study. ZJ organized the frame. WJ wrote the first draft of the manuscript. ZJ wrote sections of the manuscript. All authors contributed to manuscript revision, read, and approved the submitted version.
Funding
The authors are grateful the support of the Joint Research program of Sino-foreign Cooperation in Running Schools of Jiangsu Province, China.
Conflict of Interest
The authors declare that the research was conducted in the absence of any commercial or financial relationships that could be construed as a potential conflict of interest.
Publisher’s Note
All claims expressed in this article are solely those of the authors and do not necessarily represent those of their affiliated organizations, or those of the publisher, the editors and the reviewers. Any product that may be evaluated in this article, or claim that may be made by its manufacturer, is not guaranteed or endorsed by the publisher.
References
Bi, Z., Li, T., Su, H., Ni, Y., and Yan, L. (2018). Transparent wood Film Incorporating Carbon Dots as Encapsulating Material for white Light-Emitting Diodes. ACS Sustainable Chem. Eng. 6 (7), 9314–9323. doi:10.1021/acssuschemeng.8b01618
Cao, Y., Mei, J., and Dong, Z. (2018). Intelligent Door and Window System. Digital Technology Appl. 36 (8), 821. doi:10.19695/j.cnki.cn12-1369.2018.08.04
Chen, H., Baitenov, A., Li, Y., Vasileva, E., Popov, S., Sychugov, I., et al. (2019). Thickness Dependence of Optical Transmittance of Transparent Wood: Chemical Modification Effects. ACS Appl. Mater. Inter. 11, 35451–35457. doi:10.1021/acsami.9b11816
Chen, L., Xu, Z., Wang, F., Duan, G., Xu, W., Zhang, G., et al. (2020a). A Flame-Retardant and Transparent wood/polyimide Composite with Excellent Mechanical Strength. Composites Commun. 20, 100355. doi:10.1016/j.coco.2020.05.001
Chen, Z., Gao, H., Li, W., Li, S., Liu, S., and Li, J. (2020b). Advances in Research on Biomass-Based Photographic Materials. J. For. Eng. 5 (3), 1–12. doi:10.13360/j.issn.2096-1359.201906020
China National Standardization Administration Committee (2010). GB11614-2009 Code for Flat Glass. China: China Standard Press. (In Chinese).
China National Standardization Administration Committee (2009a). GB15763 Code for Safety Glass for Buildings. China: China Standard Press. (In Chinese).
China National Standardization Administration Committee (2009b). GB15763.1-2009 Code for Flame Resistant Glass. China: China Standard Press. (In Chinese).
Höglund, M., Johansson, M., Sychugov, I., and Berglund, L. A. (2020). Transparent Wood Biocomposites by Fast UV-Curing for Reduced Light-Scattering through Wood/Thiol-ene Interface Design. ACS Appl. Mater. Inter. 12 (41), 46914–46922. doi:10.1021/acsami.0c12505
Karl’a, V. (2019). Update on Research on Transparent wood. IOP Conf. Ser. Mater. Sci. Eng. 566, 012015. doi:10.1088/1757-899X/566/1/012015
Li, T., Zhu, M., Yang, Z., Song, J., Dai, J., Yao, Y., et al. (2016). Wood Composite as an Energy Efficient Building Material: Guided Sunlight Transmittance and Effective thermal Insulation. Adv. Energ. Mater. 6 (22), 1601122. doi:10.1002/aenm.201601122
Li, Y., Cheng, M., Jungstedt, E., Xu, B., Sun, L., and Berglund, L. (2019). Optically Transparent wood Substrate for Perovskite Solar Cells. ACS Sustainable Chem. Eng. 7 (6), 6061–6067. doi:10.1021/acssuschemeng.8b06248
Li, Y., Vasileva, E., Sychugov, I., Popov, S., and Berglund, L. (2018a). Optically Transparent wood: Recent Progress, Opportunities, and Challenges. Adv. Opt. Mater. 6 (14), 1800059. doi:10.1002/adom.201800059
Li, Y., Yang, X., Fu, Q., Rojas, R., Yan, M., and Berglund, L. (2018b). Towards Centimeter Thick Transparent wood through Interface Manipulation. J. Mater. Chem. A. 6 (3), 1094–1101. doi:10.1039/c7ta09973h
Liu, Y., Wu, Z., and Xu, W. (2019). Application of Life Cycle Evaluation in Furniture Industry. World For. Res. 2, 56–60. doi:10.13348/j.cnki.sjlyyj.2019.0016.y
Mi, R., Chen, C., Keplinger, T., Pei, Y., He, S., Liu, D., et al. (2020a). Scalable Aesthetic Transparent wood for Energy Efficient Buildings. Nat. Commun. 11 (1), 3836. doi:10.1038/s41467-020-17513-w
Mi, R., Li, T., Dalgo, D., Chen, C., Kuang, Y., He, S., et al. (2020b). A clear, strong, and Thermally Insulated Transparent wood for Energy Efficient Windows. Adv. Funct. Mater. 30 (1), 1907511. doi:10.1002/adfm.201907511
Ministry of Industry and Information Technology of the People's Republic of China (2015). JC/T2304-2015 Code for Technical Conditions for Building Thermal Insulation Glass. China: China Building Materials Industry Press. (In Chinese).
Montanari, C., Ogawa, Y., Olsen, P., and Berglund, L. A. (2021). High Performance, Fully Bio‐Based, and Optically Transparent Wood Biocomposites. Adv. Sci. 8 (12), 2100559. doi:10.1002/ADVS.202100559
Murayama, T., Taniguchi, Y., and Iwasawa, K. (1998). High-Ionization Nuclear Emission-Line Region in the Seyfert Galaxy Tololo 0109−383. Astronomical J. 115, 460–471. doi:10.1086/300218
Qiu, Z., Wang, S., Wang, Y., Li, J., Xiao, Z., Wang, H., et al. (2020). Transparent wood with Thermo-Reversible Optical Properties Based on Phase-Change Material. Composites Sci. Technology 200, 108407. doi:10.1016/j.compscitech.2020.108407
Qiu, Z., Xiao, Z., Gao, L., Li, J., Wang, H., Wang, Y., et al. (2019). Transparent wood Bearing a Shielding Effect to Infrared Heat and Ultraviolet via Incorporation of Modified Antimony-Doped Tin Oxide Nanoparticles. Composites Sci. Technology 172, 43–48. doi:10.1016/j.compscitech.2019.01.005
Sun, M., and Liu, Y. (2019). Ecological Design Based on Life Cycle of Furniture Products. Study Furniture and Interior Decoration 6, 74–76. doi:10.16771/j.cn43-1247/ts.2019.06.019
Wang, G., Zhu, J., Hou, H., and Li, X. (2019). A Case Study on the Implementation of green Manufacturing Technology System in Furniture Enterprises (Part II). Furniture 6, 85–90. doi:10.16610/j.cnki.jiaju.2019.06.018
Wang, X., Zhan, T., Liu, Y., Shi, J., Pan, B., Zhang, Y., et al. (2018). Large‐Size Transparent Wood for Energy‐Saving Building Applications. ChemSusChem 11 (23), 4086–4093. doi:10.1002/cssc.201801826
Wang, Y., Wu, Y., Zhou, J., Huang, Q., Lian, X., and Li, J. (2020). Preparation and Properties of Two Transparent wood. Study J. Southwest For. Univ. 5, 151–158. doi:10.11929/j.swfu.201910040
Wu, Y., Wang, Y., Yang, F., Wang, J., and Wang, X. (2020). Study on the Properties of Transparent Bamboo Prepared by Epoxy Resin Impregnation. Polymers 12 (4), 863. doi:10.3390/polym12040863
Wu, Y., Wu, J., Yang, F., Tang, C., and Huang, Q. (2019). Effect of H2O2 Bleaching Treatment on the Properties of Finished Transparent Wood. Polymers 11 (5), 776. doi:10.3390/polym11050776
Yin, Q., and Liu, H. (2020). Application of wood Material in Automobile interior. Study For. Machinery Woodworking Equipment 11, 51–53. doi:10.13279/j.cnki.fmwe.2020.0134
Zhang, L., Wang, A., Zhu, T., Chen, Z., Wu, Y., and Gao, Y. (2020). Transparent wood Composites Fabricated by Impregnation of Epoxy Resin and W-Doped VO2 Nanoparticles for Application in Energy-Saving Windows. ACS Appl. Mater. Inter. 12 (31), 34777–34783. doi:10.1021/acsami.0c06494
Zhao, P., Li, F., Zhang, N., and Li, K. (2019). Investigation and Analysis on Safety Production Status of Flat Glass Industry. Sci. Technology Wind 11, 143. doi:10.19392/j.cnki.1671-7341.201911121
Zhao, W., Ni, S., Wang, H., and Feng, H. (2017). Problems and Countermeasures of Air Pollution Prevention and Control in Flat Glass Industry. J. China Inst. Environ. Management 5, 78–81. doi:10.13358/j.issn.1008-813x.2017.05.20
Zhao, Z., Huang, C., Wu, D., Chen, Z., Zhu, N., Gui, C., et al. (2020a). Utilization of Enzymatic Hydrolysate from Corn stover as a Precursor to Synthesize an Eco-Friendly Plywood Adhesive. Ind. Crops Prod. 152, 112501. doi:10.1016/j.indcrop.2020.112501
Zhao, Z., Wu, D., Huang, C., Zhang, M., Umemura, K., and Yong, Q. (2020b). Utilization of Enzymatic Hydrolysate from Corn stover as a Precursor to Synthesize an Eco-Friendly Adhesive for Plywood II: Investigation of Appropriate Manufacturing Conditions, Curing Behavior, and Adhesion Mechanism. J. Wood Sci. 66 (1), 85. doi:10.1186/s10086-020-01933-9
Zhou, J., and Wu, Y. (2020). Advances in Preparation Technology and Application of Transparent wood. For. Product. Industry 10, 17–22. doi:10.19531/j.issn1001-5299.202010004
Zhu, M., Jia, C., Wang, Y., Fang, Z., Dai, J., Xu, L., et al. (2018). Isotropic Paper Directly from Anisotropic wood: Top-Down green Transparent Substrate toward Biodegradable Electronics. ACS Appl. Mater. Inter. 10 (34), 28566–28571. doi:10.1021/acsami.8b08055
Keywords: biomass materials, transparent materials, transparent wood, green and renewable energy, architectural glass;
Citation: Wang J and Zhu J (2021) Prospects and Applications of Biomass-Based Transparent Wood: An Architectural Glass Perspective. Front. Chem. 9:747385. doi: 10.3389/fchem.2021.747385
Received: 26 July 2021; Accepted: 06 October 2021;
Published: 20 October 2021.
Edited by:
Jia-Long Wen, Beijing Forestry University, ChinaReviewed by:
Yan Xia, Southwest Forestry University, ChinaYi Liu, Beijing Forestry University, China
Copyright © 2021 Wang and Zhu. This is an open-access article distributed under the terms of the Creative Commons Attribution License (CC BY). The use, distribution or reproduction in other forums is permitted, provided the original author(s) and the copyright owner(s) are credited and that the original publication in this journal is cited, in accordance with accepted academic practice. No use, distribution or reproduction is permitted which does not comply with these terms.
*Correspondence: Jing Wang, WangJing_9711@163.com; Jian’gang Zhu, austin_zhu@njfu.edu.cn