- 1Institute of Chemistry and BioMedical Sciences, School of Chemistry and Chemical Engineering, Nanjing University, Nanjing, China
- 2School of Chemistry and Chemical Engineering, Southeast University, Nanjing, China
- 3Key Laboratory of Functional Inorganic Material Chemistry, Ministry of Education, Heilongjiang University, China
- 4State Key Laboratory of Analytical Chemistry for Life Science, School of Chemistry & Chemical Engineering and Center of Materials Analysis, Nanjing University, Nanjing, China
- 5Department of Chemistry and Biochemistry, Texas Tech University, Lubbock, TX, United States
- 6Department of Chemistry, Faculty of Chemical and Life Sciences, Abdul Wali Khan University, Mardan, Pakistan
Chemical synthesis based on Group-Assisted Purification chemistry (GAP) has been prolifically used as a powerful, greener and ecofriendly tool so far. Herein, we report hypervalent iodine (III) mediated regio- and diastereoselective aminobromination of electron-deficient olefins using group-assisted purification (GAP) method. By simply mixing the GAP auxiliary-anchored substrates with TsNH2–NBS as nitrogen/bromine sources and PhI(OAc)2 as a catalyst, a series of vicinal bromoamines with multifunctionalities were obtained in moderate to excellent yields (53–94%). The vicinal bromoamines were obtained without column chromatography and/or recrystallization simply by washing the crude mixtures with cosolvents and thus avoiding wastage of silica, solvents, time, and labor. The GAP auxiliary is recyclable and reusable.
Introduction
Aminohalogenation of olefins, an important difunctionalization reaction, allows the direct construction to C−N and C−halogen double bonds which are versatile synthetic intermediates for pharmaceutically and biologically important molecules (Gao et al., 2005; Yeung et al., 2006b). The intramolecular and/or intermolecular replacement of labile halogen moieties with multifarious nucleophiles leads to precursors like vicinal diamines, lactams, amino alcohols α,β-dehydroamino acids, amino aldehydes and aziridines (Ling et al., 1996; Van and De Kimpe, 2000; Klepacz and Zwierzak, 2001; Chen et al., 2005; Li et al., 2007; Ghorai et al., 2011; Schröder et al., 2017; Thakur et al., 2017).
Since the aminohalogenation reaction was discovered several decades ago, a variety of synthetic techniques have been created to provide this capability. Various reagent systems (halogen/nitrogen sources) such as TsNH2–NBS (Thakur et al., 2003; Chen et al., 2009a; Chen et al., 2009b; Shaikh et al., 2009; Wei et al., 2009a; Wei et al., 2009b; Cai et al., 2011; Yu et al., 2017), cyanamide–NBS (Ponsold and Ihn, 1970), N-bromoacetamide (Yeung et al., 2006a; Yeung et al., 2006b), N,N-dihalosulfonamides (Kharasch and Priestley, 1939), S,S-dimethyl-N-(p-toluenesulfonyl)sulfilimine–NBS (Raghavan et al., 2001), BocNH2/BocNBr2 (Chen et al., 2013), N,N-dihalocarbamates (Śliwińska and Zwierzak, 2003) and N-halocarbamates (Driguez et al., 1978) have been designed to carry out this transformation. To achieve high yields, excellent regioselectivities and diastereoselectivities, our group as well as others have developed efficient catalytic systems which comprise: metal and nonmetal powders (Chen et al., 2009b; Wei et al., 2009a), metal oxides (Thakur et al., 2003; Shaikh et al., 2009) and metal salts (Albone et al., 1998; Ando et al., 1998; Li et al., 1999; Li et al., 2000; Wei et al., 2001; Yeung et al., 2006a; Wang et al., 2008; Chen et al., 2009a; Wei et al., 2009b; Yadav et al., 2009), organic catalysts like hypervalent iodines (Fan et al., 2007; Wang and Wu, 2007; Wu et al., 2008), phosphoric acid or phosphate (Chen et al., 2010; Huang et al., 2011; Alix et al., 2012; Xie et al., 2013), noncatalytic routes which utilize Bronsted acids (Wu and Wang, 2007) such as H2SO4 or ionic liquid media [Bmim][BF4](Xu et al., 2004). Though considerable progress has been made in this area, drastic reaction conditions, procedural complexities, the use of metal catalysts and contamination of materials by metal traces (Chen et al., 2003a; Garrett and Prasad, 2004; Huang and Shaughnessy, 2006) limit their application. Besides, the study of efficient highly regio- and stereoselective methods which could reduce the formation of side products remains challenging. Purification techniques such as column chromatography and recrystallization are commonly used in the above mentioned syntheses.
The development of environmentally benign and eco-friendly greener reaction protocol is ubiquitous both in academia and the pharmaceutical industry (Shi et al., 2008). GAP chemistry, recently introduced by our group, fulfills the afford-mentioned criteria of greener chemistry by avoidance of separation, workup, recrystallization, and column chromatography. The product is obtained by merely washing the reaction mixture with a combination of more polar and less polar solvents (Wang et al., 2013; Chennapuram et al., 2014; Dommaraju and Prajapati, 2015; Seifert et al., 2016; Patel et al., 2019; Li et al., 2020a; Li et al., 2020b; Li et al., 2020c). Polarity difference between the solvents plays a key role in the isolation of products, i.e., the impurities get dissolved in washing solvents and the GAP-coupled product remains insoluble clustered together. Keeping in view the greener aspect of GAP chemistry, here we report for the first time hypervalent iodine (III) mediated regio- and diastereoselective vicinal aminobromination of GAP-tailored electron-deficient olefins via GAP protocol.
Results and Discussion
Based on our prior research, we were interested in aminobromination of α,β-unsaturated cinnamic acids, which are challenging due to the formation of regio- and diastereomeric products. To develop conditions for regio- and diastereoselective transformation, we began to prepare the GAP coupled intermediate 1a-k and 2a-k in our laboratory according to the literature procedure (Rahman et al., 2020) given in supporting information. To optimize the reaction conditions, we initiated the study with the GAP anchored intermediate 1a as the test substrate, p-toluenesulfonamide (4-TsNH2) and N-bromosuccinimide (NBS) as the nitrogen and bromine source respectively. To our delight, product 3a was isolated in 60% yield after 24 h with a dr value 7:1 when 1a was treated with NBS (1.5 eq) and 4-TsNH2 (1.5 eq) in dichloromethane at room temperature without any catalyst. Lower yields were obtained with other bromine sources (Table 1, entries 2–4). With NBS as the bromine source, a series of hypervalent iodine and transition metal catalysts were subsequently employed. The yield was significantly improved with iodobenzene diacetate (PhI(OAc)2), and aminobromine product was isolated in a chemical yield of 78% with diastereoselective ratio of 7:1 (Table 1, entry 8). Refluxing this reaction mixture further enhanced the yield up to 82% (Table 1, entry 13). An even more increase in yield was observed when 2 eq of each NBS and 4-TsNH2 was added to the reaction medium (Table 1, entry 14). The yield was further improved to 90% with a longer reaction time (48 h) (Table 1, entry 15). We then utilized the catalytic activity of other iodine catalysts like PIFA (PhI(OCOCF3)2) and Koser’s reagent (PhI(OH) (4-TsOH)) in this transformation; only PhI(OAc)2 could give the terminal product in higher yield (Table 1, entry 15). Except for CHCl3 and CH3CN, poorer results were obtained at reflux temperature with other solvents examined when the reaction was performed with 20 mol% of PhI(OAc)2 as the catalyst and 2 equiv. of NBS and 4-TsNH2 (Table 1, entries 18–23). A yield of 67% was obtained when the catalyst loading was decreased to 10 mol%. Control experiments showed that both NBS and the 4-TsNH2 were important for the reaction and that using activated molecular sieves 4Å generally increased the yield and selectivity.
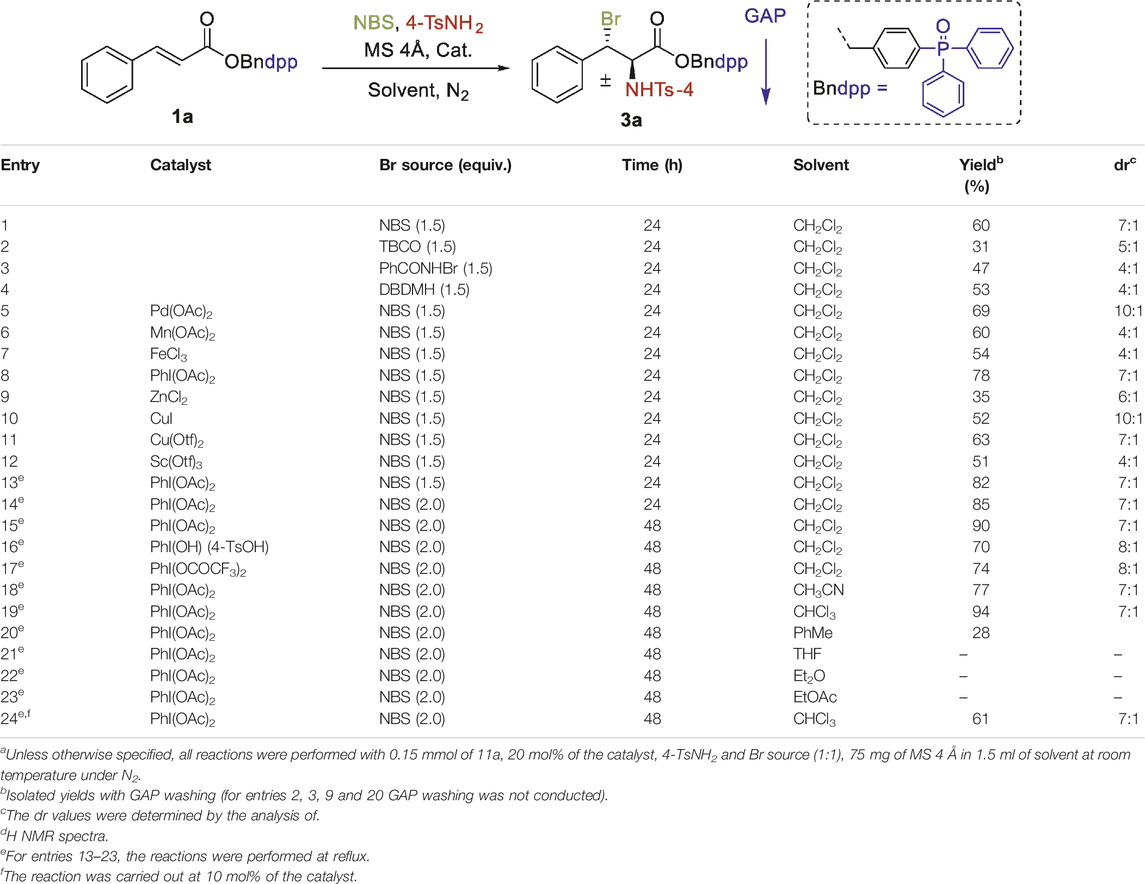
TABLE 1. Optimization of the reaction conditions.a
After optimizing the conditions for aminobromination reaction, the substrate scope was subsequently explored. The results are shown in Table 2. A wide range of N-(4-(diphenylphosphoryl)benzyl) cinnamates 1a-k bearing different aryl groups with a variety of electron-donating (EDG) (such as methyl and methoxy) and electron-withdrawing groups (EWG) (floro, chloro bromo, nitro) were investigated which provided moderate to high yields (53–94%). As shown in Table 2, with regards to the EDG on the aromatic ring of cinnamic substrates 1b-1f, the addition reactions were well tolerated to produce the relevant adducts in good yields (Table 2, 2b-2f). Both the substrates 1b and 1c with an ortho-MeC6H4 and a para-MeC6H4 group delivered the corresponding products 3b and 3c smoothly in 85 and 89% yields respectively. Similarly, the product 3 days with ortho-OMeC6H4 was isolated in a high yield of 80%. The di-OMe and tri-OMe substituted substrates were even more effective for the reaction (Table 2, 3e, 3f). On the other hand, substrates bearing EWG on the aromatic rings generally decreased the yield under the same conditions (Table 2, 3g-3j). Importantly, halogen (Br or F) groups were almost consistent with the conditions, offering 3g, 3h and 3i in moderate yields. The lowest yield of 53% was obtained for 3j, which had a Cl group at the ortho-position and an NO2 group at para-position. The substrate with a naphthyl group reduced the yield to 81% under the same conditions but enhanced the diastereoselectivity (Table 2, 3k).
In addition to N-(4-(diphenylphosphoryl) benzyl) cinnamates, N-(4-(diphenylphosphoryl) benzyl) cinnamamides 2a-k were then exposed to aminobromination under the optimized reaction conditions for 1a-k. The reaction was applicable in the presence of 20 mol% of PhI(OAc)2 in chloroform, substrate 2a was successfully converted in 48 h at reflux temperature to haloamine product 4a in 78% yield with a diastereoselective ratio of 18:1.
As shown in Table 3, this transformation can be extended to a variety of N-(4-(diphenylphosphoryl)benzyl) cinnamamides 2a-k to provide moderate to high yields (56–81%). The substrates with EWG and EDG display substantial variations in reaction reactivity and regioselectivity. Aminobromination was greatly facilitated by the presence of a strong EDG on the benzene ring, affording products in high yields and good to excellent diastereoselectivity (Table 3, 4b–4e). The substrate with EWG on the aromatic ring, as expected, resulted in a lower yield (Table 3, 4f–4j). The substrate with a naphthyl group, however, had no significant effect on the yield under the same conditions and lowered the diastereoselectivity (Table 3, 4k).
From Table 2, 3, we further observed that EWG and EDG on the benzene ring had a significant impact on the diastereoselectivity of cinnamates and cinnamamides which is generally governed by the GAP auxiliaries. In the case of cinnamates, EDG resulted in low diastereoselectivity than EWG. For cinnamamides, however, EDG had higher diastereoselectivity than EWG. This variation in diastereoselectivity of both derivatives could be attributed to stereoelectronic factors.
The feasibility of this procedure was studied by conducting the reaction on a gram scale for the starting materials 1a and 2a, which resulted in 85 and 73% yields for the products 3a and 4a, respectively.
In the presence of Pd/C and NaBH4, the GAP-tailored vicinal aminobromine was deprotected which afforded Bndpp in 93% yield (Schemes 1,2). The mixture is dissolved in a small volume of a solvent, such as ethyl acetate or DCM, and then petroleum ether is used to purify the products. The GAP auxiliary precipitates as a white solid that is filtered and treated with petroleum ether. To achieve the desired β-bromoamine as a white substance, the filtrate is evaporated under a vacuum.
Mechanism
The outcomes of various experimentation within our research team, as well as other (Li et al., 2001; Wei et al., 2001; Wang and Wu, 2007; Wu and Wang, 2008; Chen et al., 2009a), lead to the conclusion that NBS may react with 4-TsNH2 to generate N-bromo-p-toluenesulfonamide (4-TsNHBr) 6 (Scheme 3), which would be oxidized by PhI(OAc)2 to generate intermediate Int-I that may either follow cycle A or cycle B. In cycle A, the Int-I will form aziridinium Int-II with a double bond of 1a or 2a, which is then stereoselectively attacked by the dissociated bromide from the Int-I at the more electrophilic carbon (beta to carbonyl carbon) to yield compound Int-III. Int-III and 16 eventually provide the ultimate bromoamine substance 3a or 4a and restore Int-I. When the fragile N–I bond of Int-I is broken, N-acetoxy-N-halo-p-toluenesulfonamide Int-IV can form, which could then be the active intermediate for cycle B. Int-IV that forms an equilibrium with nitrenium ion Int-V (Kikugawa et al., 2003; Murata et al., 2008) could react with olefin 1a or 2a to afford aziridinium Int-VI which would lead to Int-VII following an SN2 nucleophilic attack by the nearby bromide. Finally, the reaction of the intermediate Int-VII with 6 gives the final product and regenerates Int-IV.
Benefiting from the present methodology and this mechanism analysis, the utilizations of GAP chemistry for aminohalogenation and diamination of a broader scope of substrates (Chen et al., 2003b; Chen et al., 2004), in search for new chirality (Wu et al., 2019a; Wu et al., 2019b; Liu et al., 2020) and on multi-component reactions will be further conducted in our labs (Jiang et al., 2012a; Jiang et al., 2012b).
Experimental Section
Aminobromination of 4-(Diphenylphosphoryl) Benzyl Cinnamates 1a-k and N-(4-(Diphenylphosphoryl) Benzyl) Cinnamamides 2a-k
Typical procedure: Into a dry vial was added 1a or 2a (1 mmol, 1 eq), NBS (356 mg, 2 mmol, 2 eq), 4-TsNH2 (342 mg, 2 mmol, 2 eq), PhI(OAc)2 (64 mg, 20 mol%) and freshly activated 4 Å molecular sieves (500 mg) and capped under nitrogen protection. CHCl3 (3 ml) was added via a syringe and the reaction mixture was allowed to reflux for 48 h. After completion (monitored by TLC), the reaction was quenched with dropwise addition of saturated aqueous Na2SO3 solution (2 ml) and DCM (3 × 10 ml) was added to extract the product. The combined organic layers were washed with brine, dried over anhydrous sodium sulfate, and concentrated under reduced pressure. The mixture was redissolved in the minimal amount of solvents like ethyl acetate or DCM, and then petroleum ether was added. The GAP auxiliary precipitated in the form of a white solid which was filtered and washed with petroleum ether. The filtrate is evaporated under a vacuum to obtain the desired β-aminobromine as a white product.
General Procedure for Deprotection of Group-Assisted Purification Auxiliary BnDpp.
To a 10 ml round bottom flask was added 4a (0.2 g, 0.32 mmol), 10 wt% Pd/C (20 mg) 2 ml MeOH and NaBH4 (15.2 mg, 2 equiv.). To prevent the loss of produced hydrogen and overpressure in the flask, it was sealed with a rubber septum and a deflated balloon. the reaction mixture was drained through a Celite after 2 h and the filtrate was concentrated under reduced pressure before being redissolved in EtOAc. After that, KHSO4 was used to neutralize the reaction mixture. The organic layer was separated, dried over anhydrous Na2SO4 and evaporated to dryness to afford crude GAP auxiliary, which was easily purified using the GAP washing method.
Conclusion
In conclusion, we have demonstrated a new method for the preparation of vicinal aminobrominated products of electron-deficient olefins coupled with GAP auxiliaries dppBnOH and dppBnNH2. Good yields and diastereoselectivities were obtained in a clean and eco-friendly reaction condition comprising the catalyst PhI(OAc)2 with NBS and 4-TsNH2 as the bromine and nitrogen sources. The Group-Assisted Purification (GAP) chemistry was successfully applied and the compounds were obtained as precipitates without column chromatography and recrystallization by merely adding ethyl acetate and petroleum ether. Besides, the GAP auxiliary can be recovered for reuse.
Data Availability Statement
The original contributions presented in the study are included in the article/Supplementary Material, further inquiries can be directed to the corresponding authors.
Author Contributions
GL, AA and AR designed the project. AR, NZ, IK and FF performed the experiments. AA and AR analyzed the data and wrote the manuscript. GL supervised, funded and critically reviewed manuscript.
Funding
We would like to acknowledge the financial support from the National Natural Science Foundation of China (No. 22071102 and 91956110) and Robert A. Welch Foundation (D-1361, United States).
Conflict of Interest
The authors declare that the research was conducted in the absence of any commercial or financial relationships that could be construed as a potential conflict of interest.
Publisher’s Note
All claims expressed in this article are solely those of the authors and do not necessarily represent those of their affiliated organizations, or those of the publisher, the editors and the reviewers. Any product that may be evaluated in this article, or claim that may be made by its manufacturer, is not guaranteed or endorsed by the publisher.
Supplementary Material
The Supplementary Material for this article can be found online at: https://www.frontiersin.org/articles/10.3389/fchem.2021.742399/full#supplementary-material
References
Albone, D. P., Aujla, P. S., Paul C. Taylor*, S., Challenger, S., and Derrick, A. M. (1998). A simple copper catalyst for both aziridination of alkenes and amination of activated hydrocarbons with chloramine-T trihydrate. J. Org. Chem. 63, 9569–9571. doi:10.1021/jo981335q
Alix, A., Lalli, C., Retailleau, P., and Masson, G. (2012). Highly Enantioselective Electrophilic α-Bromination of Enecarbamates: Chiral Phosphoric Acid and Calcium Phosphate Salt Catalysts. J. Am. Chem. Soc. 134, 10389–10392. doi:10.1021/ja304095z
Ando, T., Minakata, S., Ryu, I., and Komatsu, M. (1998). Nitrogen atom transfer to alkenes utilizing chloramine-T as a nitrogen source. Tetrahedron Lett. 39, 309–312. doi:10.1016/s0040-4039(97)10553-6
Cai, Y., Liu, X., Jiang, J., Chen, W., Lin, L., and Feng, X. (2011). Catalytic Asymmetric Chloroamination Reaction of α,β-Unsaturated γ-Keto Esters and Chalcones. J. Am. Chem. Soc. 133, 5636–5639. doi:10.1021/ja110668c
Chen, D., Guo, L., Liu, J., Kirtane, S., Cannon, J. F., and Li, G. (2005). Functionalization of α,β-Unsaturated Esters and Ketones: A Facile and Highly Stereoselective One-Pot Approach to N-Protected α,β-Dehydroamino Acid Derivatives. Org. Lett. 7, 921–924. doi:10.1021/ol050002u
Chen, D., Kim, S. H., Hodges, B., and Li, G. (2003a). The cinnamate-based aminohalogenation provides an easy access to anti methyl 3-aryl-N-p-tosyl- and N-o-nosyl-aziridine-2-carboxylates. Arkivoc 2003, 56–63. doi:10.3998/ark.5550190.0004.c07
Chen, D., Timmons, C., Chao, S., and Li, G. (2004). Regio- and Stereoselective Copper-Catalyzed Synthesis of Vicinal Haloamino Ketones fromα,β-Unsaturated Ketones. Eur. J. Org. Chem. 2004, 3097–3101. doi:10.1002/ejoc.200400050
Chen, D., Timmons, C., Wei, H. X., and Li, G. (2003b). Direct Electrophilic Diamination of Functionalized Alkenes Without the Use of Any Metal Catalysts. J. Org. Chem. 68, 5742–5745. doi:10.1021/jo030098a
Chen, S., Han, J., Li, G., and Pan, Y. (2013). Highly diastereoselective aminobromination of β-methyl-β-nitrostyrenes with t-butyl N,N-dibromocarbamate/t-butyl carbamate as bromine/nitrogen sources. Tetrahedron Lett. 54, 2781–2784. doi:10.1016/j.tetlet.2013.02.113
Chen, Z.-G., Wang, Y., Wei, J.-F., Zhao, P.-F., and Shi, X.-Y. (2010). K3PO4-Catalyzed Regiospecific Aminobromination of β-Nitrostyrene Derivatives with N-Bromoacetamide as Aminobrominating Agent. J. Org. Chem. 75, 2085–2088. doi:10.1021/jo9026879
Chen, Z.-G., Wei, J.-F., Li, R.-T., Shi, X.-Y., and Zhao, P.-F. (2009a). Copper Powder-Catalyzed Regio- and Stereoselective Aminobromination of α,β-Unsaturated Ketones with TsNH2 and NBS as Nitrogen and Halogen Sources. J. Org. Chem. 74, 1371–1373. doi:10.1021/jo8023768
Chen, Z.-G., Wei, J.-F., Wang, M.-Z., Zhou, L.-Y., Zhang, C.-J., and Shi, X.-Y. (2009b). Aluminium Powder-Catalyzed Regio- and Stereoselective Aminobromination of α,β-Unsaturated Carbonyl Compounds and Simple Olefins with thep-Toluenesulfonamide/N-Bromosuccinimide (TsNH2-NBS) System. Adv. Synth. Catal. 351, 2358–2368. doi:10.1002/adsc.200900343
Chennapuram, M., Emmadi, N. R., Bingi, C., Nanubolu, J. B., and Atmakur, K. (2014). Group-assisted purification (GAP) chemistry for dihydrofurans: water as a medium for catalyst free synthesis in a one pot four component reaction. Green. Chem. 16, 3237–3246. doi:10.1039/C4GC00388H
Dommaraju, Y., and Prajapati, D. (2015). A highly efficient group-assisted purification method for the synthesis of poly-functionalized pyrimidin-5-yl-pyrroles via one-pot four-component domino reaction. Mol. Divers. 19, 173–187. doi:10.1007/s11030-014-9547-1
Driguez, H., Vermes, J.-P., and Lessard, J. (1978). The chromous chloride promoted addition of N-haloamides to olefins. V. The addition of N-chloroamides to enol ethers: synthesis of acyloxy and acyl derivatives of α-amino acetals and ketals (aldehydes and ketones) and of 2-amino sugars. Can. J. Chem. 56, 119–130. doi:10.1139/v78-019
Fan, R., Wen, F., Qin, L., Pu, D., and Wang, B. (2007). PhI(OAc)2 induced intramolecular oxidative bromocyclization of homoallylic sulfonamides with KBr as the bromine source. Tetrahedron Lett. 48, 7444–7447. doi:10.1016/j.tetlet.2007.08.085
Fu, H., Wang, Z., Zhang, Y., Jiang, Y., and Zhao, Y. (2008). FeCl2-Catalyzed Aminobromination of Alkenes Using Amides or Sulfonamides and NBS as the Nitrogen and Bromine Sources. Synlett 2008, 2667–2670. doi:10.1055/s-0028-1083509
Gao, G.-Y., Harden, J. D., and Zhang, X. P. (2005). Cobalt-catalyzed efficient aziridination of alkenes. Org. Lett. 7, 3191–3193. doi:10.1021/ol050896i
Garrett, C. E., and Prasad, K. (2004). The art of meeting palladium specifications in active pharmaceutical ingredients produced by Pd-catalyzed reactions. Adv. Synth. Catal. 346, 889–900. doi:10.1002/adsc.200404071
Ghorai, M. K., Sahoo, A. K., and Kumar, S. (2011). Synthetic route to chiral tetrahydroquinoxalines via ring-opening of activated aziridines. Org. Lett. 13, 5972–5975. doi:10.1021/ol2023906
Huang, D., Wang, H., Xue, F., Guan, H., Li, L., Peng, X., et al. (2011). Enantioselective bromocyclization of olefins catalyzed by chiral phosphoric acid. Org. Lett. 13, 6350–6353. doi:10.1021/ol202527g
Huang, R., and Shaughnessy, K. H. (2006). Water-soluble palladacycles as precursors to highly recyclable catalysts for the Suzuki coupling of aryl bromides in aqueous solvents. Organometallics 25, 4105–4112. doi:10.1021/om050940y
Jiang, B., Feng, B.-M., Wang, S.-L., Tu, S.-J., and Li, G. (2012a). Domino constructions of pentacyclic indeno[2,1-c]quinolines and pyrano[4,3-b]oxepines by [4+1]/[3+2+1]/[5+1] and [4+3] multiple cyclizations. Chem. Eur. J. 18, 9823–9826. doi:10.1002/chem.201201109
Jiang, B., Li, Q.-Y., Tu, S.-J., and Li, G. (2012b). Three-component domino reactions for selective formation of indeno[1,2-b]indole derivatives. Org. Lett. 14, 5210–5213. doi:10.1021/ol3023038
Kharasch, M. S., and Priestley, H. M. (1939). The addition of N-haloamides to olefins. J. Am. Chem. Soc. 61, 3425–3432. doi:10.1021/ja01267a057
Kikugawa, Y., Nagashima, A., Sakamoto, T., Miyazawa, E., and Shiiya, M. (2003). Intramolecular Cyclization with Nitrenium Ions Generated by Treatment ofN-Acylaminophthalimides with Hypervalent Iodine Compounds: Formation of Lactams and Spiro-Fused Lactams. J. Org. Chem. 68, 6739–6744. doi:10.1021/jo0347009
Klepacz, A., and Zwierzak, A. (2001). t-Butyl N,N-dibromocarbamate (BBC)-new reagent for aminobromination of terminal alkenes. Tetrahedron Lett. 42, 4539–4540. doi:10.1016/s0040-4039(01)00662-1
Li, G., Kotti, S. R. S. S., and Timmons, C. (2007). Recent Development of Regio‐ and Stereoselective Aminohalogenation Reaction of Alkenes. Eur. J. Org. Chem. 2007, 2745–2758. doi:10.1002/ejoc.200600990
Li, G., Wei, H.-X., and Kim, S. H. (2000). Copper-Catalyzed Aminohalogenation Using the 2-NsNCl2/2-NsNHNa Combination as the Nitrogen and Halogen Sources for the Synthesis of anti-alkyl 3-Chloro-2-(o-nitrobenzenesulfonamido)-3-arylpropionates. Org. Lett. 2, 2249–2252. doi:10.1021/ol000120b
Li, G., Wei, H.-X., Kim, S. H., and Neighbors, M. (1999). Transition metal-catalyzed regioselective and stereoselective aminochlorination of cinnamic esters. Org. Lett. 1, 395–398. doi:10.1021/ol990059e
Li, G., Wei, H.-X., and Kim, S. H. (2001). Unexpected copper-catalyzed aminohalogenation reaction of olefins using N-halo-N-metallo-sulfonamide as the nitrogen and halogen sources. Tetrahedron 57, 8407–8411. doi:10.1016/S0040-4020(01)00847-X
Li, H., Chao, J., Hasan, J., Tian, G., Jin, Y., Zhang, Z., et al. (2020a). Synthesis of tri(4-formylphenyl) phosphonate derivatives as recyclable triple-equivalent supports of peptide synthesis. J. Org. Chem. 85, 6271–6280. doi:10.1021/acs.joc.9b03023
Li, H., Chao, J., Tian, G., Hasan, J., Jin, Y., Zhang, Z., et al. (2020b). Resin-free peptide synthesis mediated by tri(4-benzoylphenyl) phosphate (TBP) derivatives as small-molecule supports. Org. Chem. Front. 7, 689–696. doi:10.1039/C9QO01480B
Li, H., Ren, J., Li, J., Zhang, Z., Chang, N., and Qin, C. (2020c). Greener liquid-phase synthesis and the ACE inhibitory structure-activity relationship of an anti-SARS octapeptide. Org. Biomol. Chem. 18, 8433–8442. doi:10.1039/D0OB01948H
Ling, R., Yoshida, M., and Mariano, P. S. (1996). Exploratory Investigations Probing a Preparatively Versatile, Pyridinium Salt Photoelectrocyclization−Solvolytic Aziridine Ring Opening Sequence. J. Org. Chem. 61, 4439–4449. doi:10.1021/jo960316i
Liu, Y., Wu, G., Yang, Z., Rouh, H., Katakam, N., Ahmed, S., et al. (2020). Multi-layer 3D chirality: New synthesis, AIE and computational studies. Sci. China Chem. 63, 692–698. doi:10.1007/s11426-019-9711-x
Patel, D. M., Vala, R. M., Sharma, M. G., Rajani, D. P., and Patel, H. M. (2019). A Practical Green Visit to the Functionalized [1,2,4]Triazolo[5,1-b ]quinazolin-8(4H )one Scaffolds Using the Group-Assisted Purification (GAP) Chemistry and Their Pharmacological Testing. ChemistrySelect 4, 1031–1041. doi:10.1002/slct.201803605
Ponsold, K., and Ihn, W. (1970). Die addition von cyanamid und halogen an olefine ein neues verfahren zur darstellung von vic.-halogencyanaminen und aziridinen. Tetrahedron Lett. 11, 1125–1128. doi:10.1016/s0040-4039(01)97925-0
Raghavan, S., Ramakrishna Reddy, S., Tony, K. A., Naveen Kumar, C., and Nanda, S. (2001). Bromosulfonamidation of alkenes using S,S-dimethyl-N-(p-toluenesulfonyl)sulfilimine. Synlett 2001, 0851–0853. doi:10.1055/s-2001-14910
Rahman, A. U., Zarshad, N., Zhou, P., Yang, W., Li, G., and Ali, A. (2020). Hypervalent iodine (III) catalyzed regio- and diastereoselective aminochlorination of tailored electron deficient olefins via GAP chemistry. Front. Chem. 8, 1–9. doi:10.3389/fchem.2020.00523
Saito, S., Kikugawa, Y., Murata, K., Tsukamoto, M., and Sakamoto, T. (2008). Hydrazidohydroxylation of styrenes with N-acetylaminophthalimide using Phenyliodine(III) bis(trifluoroacetate) (PIFA). Synthesis 2008, 32–38. doi:10.1055/s-2007-1000819
Schröder, S. P., Van De Sande, J. W., Kallemeijn, W. W., Kuo, C.-L., Artola, M., Van Rooden, E. J., et al. (2017). Towards broad spectrum activity-based glycosidase probes: synthesis and evaluation of deoxygenated cyclophellitol aziridines. Chem. Commun. 53, 12528–12531. doi:10.1039/C7CC07730K
Seifert, C. W., Paniagua, A., White, G. A., Cai, L., and Li, G. (2016). GAP Peptide Synthesis through the Design of a GAP Protecting Group: An Fmoc/tBu Synthesis of Thymopentin Free from Polymers, Chromatography and Recrystallization. Eur. J. Org. Chem. 2016, 1714–1719. doi:10.1002/ejoc.201600026
Shaikh, T. M., Karabal, P. U., Suryavanshi, G., and Sudalai, A. (2009). Titanium superoxide: a heterogeneous catalyst for anti-markovnikov aminobromination of olefins. Tetrahedron Lett. 50, 2815–2817. doi:10.1016/j.tetlet.2009.03.169
Shi, D., Ji, S., Shi, D., Ni, S., and Yang, F. (2008). An efficient and green synthesis of 3,3′-benzylidenebis (4-hydroxy-6-methylpyridin-2(1h)-one) derivatives through multi-component reaction in ionic liquid. J. Heterocycl. Chem. 45, 1275–1280. doi:10.1002/jhet.5570450503
Śliwińska, A., and Zwierzak, A. (2003). Ionic addition of t-butyl N,N-dibromocarbamate (BBC) to alkenes and cycloalkenes. Tetrahedron Lett. 44, 9323–9325. doi:10.1016/j.tetlet.2003.10.040
Thakur, R., Rawal, G. K., and Vankar, Y. D. (2017). Synthesis of Chiral Aziridines from Glycals: Application in the Synthesis of a Piperidine-Azepine Fused Derivative. Eur. J. Org. Chem. 2017, 4235–4241. doi:10.1002/ejoc.201700624
Thakur, V. V., Talluri, S. K., and Sudalai, A. (2003). Transition Metal-Catalyzed Regio- and Stereoselective Aminobromination of Olefins with TsNH2 and NBS as Nitrogen and Bromine Sources. Org. Lett. 5, 861–864. doi:10.1021/ol027530f
Van, T. N., and De Kimpe, N. (2000). Synthesis of chiral cis-1,2,3-trisubstituted aziridines. Tetrahcdron 56, 7299–7304. doi:10.1016/S0040-4020(00)00627-X
Wang, G.-W., and Wu, X.-L. (2007). Mechanochemical aminochlorination of electron-deficient olefins with chloramine-T promoted by (diacetoxyiodo)benzene. Adv. Synth. Catal. 349, 1977–1982. doi:10.1002/adsc.200700020
Wang, H., Liu, X., Feng, X., Huang, Z., and Shi, D. (2013). GAP chemistry for pyrrolyl coumarin derivatives: a highly efficient one-pot synthesis under catalyst-free conditions. Green. Chem. 15, 3307–3311. doi:10.1039/C3GC41799A
Wei, H.-X., Kim, S. H., and Li, G. (2001). The first transition metal-ligand complex-catalyzed regioselective and stereoselective aminohalogenation of cinnamic esters. Tetrahedron 57, 3869–3873. doi:10.1016/S0040-4020(01)00228-9
Wei, J.-F., Chen, Z.-G., Lei, W., Zhang, L.-H., Wang, M.-Z., Shi, X.-Y., et al. (2009a). Silicon Powder: The First Nonmetal Elemental Catalyst for Aminobromination of Olefins with TsNH2 and NBS. Org. Lett. 11, 4216–4219. doi:10.1021/ol9015833
Wei, J.-F., Zhang, L.-H., Chen, Z.-G., Shi, X.-Y., and Cao, J.-J. (2009b). KI-catalyzed aminobromination of olefins with TsNH2-NBS combination. Org. Biomol. Chem. 7, 3280–3284. doi:10.1039/B904789A
Wu, G., Liu, Y., Yang, Z., Jiang, T., Katakam, N., Rouh, H., et al. (2019a). Enantioselective assembly of multi-layer 3D chirality. Natl. Sci. Rev. 7, 588–599. doi:10.1093/nsr/nwz203
Wu, G., Liu, Y., Yang, Z., Katakam, N., Rouh, H., Ahmed, S., et al. (2019b). Multilayer 3D chirality and its synthetic assembly. Research 2019, 1–11. doi:10.34133/2019/6717104
Wu, X.-L., and Wang, G.-W. (2007). Aminochlorination in Water: First Brønsted Acid-Promoted Synthesis of Vicinal Chloramines. J. Org. Chem. 72, 9398–9401. doi:10.1021/jo701957t
Wu, X.-L., and Wang, G.-W. (2008). Aminohalogenation of electron-deficient olefins promoted by hypervalent iodine compounds. Eur. J. Org. Chem. 2008, 6239–6246. doi:10.1002/ejoc.200800842
Wu, X.-L., Xia, J.-J., and Wang, G.-W. (2008). Aminobromination of olefins with TsNH2 and NBS as the nitrogen and bromine sources mediated by hypervalent iodine in a ball mill. Org. Biomol. Chem. 6, 548–553. doi:10.1039/B717333D
Xie, W., Jiang, G., Liu, H., Hu, J., Pan, X., Zhang, H., et al. (2013). Highly Enantioselective Bromocyclization of Tryptamines and its Application in the Synthesis of (−)-Chimonanthine. Angew. Chem. Int. Ed. 52, 12924–12927. doi:10.1002/anie.201306774
Xu, X., Kotti, S. R. S. S., Liu, J., Cannon, J. F., Headley, A. D., and Li, G. (2004). Ionic liquid media resulted in the first asymmetric aminohalogenation reaction of alkenes. Org. Lett. 6, 4881–4884. doi:10.1021/ol048045i
Yadav, J. S., Subba Reddy, B. V., Narasimha Chary, D., and Chandrakanth, D. (2009). InX3-catalyzed haloamidation of vinyl arenes: a facile synthesis of α-bromo- and α-fluoroamides. Tetrahedron Lett. 50, 1136–1138. doi:10.1016/j.tetlet.2008.12.090
Yeung, Y., Gao, X., and Gao, E. J. (2006a). A general process for the haloamidation of olefins. Scope and mechanism. J. Am. Chem. Soc. 128, 9644–9645. doi:10.1021/ja063675w
Yeung, Y., Hong, S., and Hong, E. J. (2006b). A short enantioselective pathway for the synthesis of the anti-influenza neuramidase inhibitor oseltamivir from 1,3-butadiene and acrylic acid. J. Am. Chem. Soc. 128, 6310–6311. doi:10.1021/ja0616433
Keywords: aziridinium, diastereoselectivity, iodobenzene diacetate, nitrogen/halogen source, protecting groups
Citation: Rahman AU, Zarshad N, Khan I, Faiz F, Li G and Ali A (2021) Regio- and Diastereoselective Vicinal Aminobromination of Electron Deficient Olefins via Phosphorus-Based GAP Protocol. Front. Chem. 9:742399. doi: 10.3389/fchem.2021.742399
Received: 16 July 2021; Accepted: 09 August 2021;
Published: 08 September 2021.
Edited by:
Zhendong Jin, University of Iowa, United StatesReviewed by:
Tzenge Lien Shih, Tamkang University, TaiwanYuguo Du, Chinese Academy of Sciences (CAS), China
Copyright © 2021 Rahman, Zarshad, Khan, Faiz, Li and Ali. This is an open-access article distributed under the terms of the Creative Commons Attribution License (CC BY). The use, distribution or reproduction in other forums is permitted, provided the original author(s) and the copyright owner(s) are credited and that the original publication in this journal is cited, in accordance with accepted academic practice. No use, distribution or reproduction is permitted which does not comply with these terms.
*Correspondence: Guigen Li, Z3VpZ2VuLmxpQHR0dS5lZHU=; Asad Ali, YXNhZF9vcmdAeWFob28uY29t