- Department of Chemistry, Rasht Branch, Islamic Azad University, Rasht, Iran
Tannic acid–linked silica-coated Fe3O4 nanoparticles (Fe3O4@SiO2@Tannic acid) were prepared and characterized by transmission electron microscope (TEM), field emission scanning electron microscope (FE-SEM), X-ray powder diffraction (XRD), X-ray spectroscopy (EDX), vibrating sample magnetometry (VSM), and Fourier transform infrared (FT-IR) spectroscopy. Fe3O4@SiO2@Tannic acid supplies an environmentally friendly procedure for the synthesis of some novel 5-amino-pyrazole-4-carbonitriles through the three-component mechanochemical reactions of synthetized azo-linked aldehydes, malononitrile, and phenylhydrazine or p-tolylhydrazine. These compounds were produced in high yields and at short reaction times. The catalyst could be easily recovered and reused for six cycles with almost consistent activity. The structures of the synthesized 5-amino-pyrazole-4-carbonitrile compounds were confirmed by 1H NMR, 13C NMR, and FTIR spectra, and elemental analyses.
Introduction
One of the largest groups of heterocyclic compounds is five-membered rings with more than one heteroatom. One of the 5-membered rings with 2-heteroatom heterocycles is pyrazoles. Pyrazoles and their salts have numerous biological and pharmaceutical properties such as anti-inflammatory, sedative, hypnotic, fever-resistant, antifungal, and antibacterial. For example, 1) phenylbutazone acts as an anti-inflammatory agent, 2) diphenucate acts as a herbicide, 3) tartazine acts as a food coloring agent, 4) cecluxib acts as an anti-inflammatory agent, and 5) pyrazophine acts as a natural antibiotic and antitumor agent (Figure 1) (Bekhite and Aziem, 2004; Liu et al., 2008).
The synthesis of pyrazoles is specific because they are found in several different structures, including pyrazoles such as pyrazolotriazines (Karci and Demircah, 2008), pyrazolotetrazinones and pyrazolopyrimidines (Wu et al., 2006), and pyrazolo-pyrazines (El-Emary, 2006).
The simplest and most common method to synthesize pyrazoles is to use 1,3-dicarbonyl compounds or similar compounds such as acetals and imines with hydrazines (Bekhite and Aziem, 2004). In recent years, the synthesis of pyrazoles has become widespread. Saleh et al. (2012) prepared pyrazolo[3,4-b]pyridines from the reaction of phenyl sulfone synthon with N-phenyl benzene carbohydrazonyl chloride. The compounds produced in this study have anti-inflammatory properties. Trofimov et al. synthesized 3-amino-3-hydroxyalkyl-1-amino thiocarbonyl pyrazoles through the stereospecific cyclization reaction of 1, 2-acetylene-3-hydroxynitriles with thiosemicarbazide (Trofimov et al., 2008). Ortiz et al. (2006) prepared a new series of pyrazoles during the Diels–Alder reaction of 1,2,3-triazoles with diethyl acetylene dicarboxylate (DMAD) followed by pyrolysis of 3,4-dicarboxylate under solvent-free conditions.
Recently, different methods have been proposed to synthesize diverse pyrazole derivatives (Salaheldin et al., 2007). These include the synthesis of 4-substituted pyrazoles during the 1,3-dipolar ring-forming reaction between diazo compounds with triple bonds (Martin et al., 2006); the three-component reaction between aromatic aldehydes, malononitrile, and phenylhydrazine (Bhale et al., 2014); the Michael addition reaction using 2-methyl-3-nitrochrome as a starting material (Takagi et al., 1987); the preparation of oxoalkanenitrile or aminonitrile derivatives (Al-Qalaf et al., 2009), from a four-component reaction with aryl aldehydes, hydrazines, ethylacetoacetate, and malononitrile (Kiyani et al., 2013), and the reaction of enamines with hydroxylamine hydrochloride (Tominaga et al., 1990).
Some methods have also been reported for the synthesis of pyrazoles with azo bridges, such as the preparation of azo dyes from pyrazoles with a nitro group, such as 1-aryl-5-amino-4-cyano-pyrazole as the starting material (Towne et al., 1968), and the coupling reaction between pyrazolo[3,4-d]-pyrazine with phenol and 1-naphthol (Kasimogullari et al., 2010).
Chemistry is advancing toward new approaches that focus on the environment. Chemists try to use green techniques such as nontoxic solvents (such as water), solvent-free syntheses, cheap and available catalysts, and one-step multicomponent reactions; nanocatalysts play an essential role in green synthesis. Nano-dimensions provide tremendous advantages for using nanoparticles as catalysts. By reducing the particle size of the catalyst, there is an increase in contact surface with the reactants, and the catalytic power is improved, resulting in maximum efficiency with a small amount of catalyst. Another useful feature of nanocatalysts is their heterogeneity with high catalytic activity, so at the end of the reaction, the catalyst can be separated from the reaction mixture by smoothing and reused (Polshettiwar and Varma, 2010; Fihri et al., 2011; Fardood et al., 2017; Rezaei et al., 2017).
Compared to other nanoparticles, magnetite (Fe3O4), due to its unique magnetic properties (Xin et al., 2020), easy magnetic separation (Hamedi et al., 2018), low toxicity (Zhao et al., 2014), environmental compatibility (Eslahi et al., 2021), and chemically modifiable surface (Bai et al., 2013), has attracted scientists. Therefore, applications of these magnetic nanoparticles (MNPs) have been developed in drug delivery, cancer treatment, magnetic resonance imaging, tissue repairing, contrast agents, magnetic storage media, biosensing, magnetic inks for jet printing, and catalysis (Inaloo et al., 2020; Eslahi et al., 2021). However, MNPs easily aggregate in aqueous solutions due to their anisotropic dipolar attraction (Sardarian et al., 2019). Also, they are unstable in acidic environments and may be oxidized by air, which can alter their magnetic properties, reduce adsorption capacity, and limit the range of application (Pourjavadi et al., 2012). To overcome this limitation, stabilization of MNPs is performed. Magnetic shells, with core advantages and a wide range of shells, have attracted much attention in intensive research (Zhao et al., 2015).
This research is essential to design an efficient, green, and simple method to prepare 5-amino-pyrazole-4-carbonitriles. Herein, we report the mechanochemical synthesis of new 5-amino-pyrazole-4-carbonitriles with azo-linked aldehydes, malononitrile, and phenylhydrazine or p-tolylhydrazine at room temperature in the presence of tannic acid–functionalized silica-coated Fe3O4 nanoparticles (Fe3O4@SiO2@Tannic acid).
Experimental
Material and Method
Chemicals were purchased from Merck and Fluca and used as raw materials of standard purity. Melting temperatures were measured on electro-thermal 9100 devices and were uncorrected. For ultrasound reactions, the ultrasound apparatus Astra 3D (9.5 dm3, 45 kHz, 305 W) from TECNO-GAZ was used. FT-IR spectra were obtained on a Shimadzu FT-IR-8400S spectrometer. A Bruker DRX-500 Avance spectrometer was used to obtain the 1H NMR and 13C NMR spectra with DMSO-d6 as the solvent and TMS as internal standard. Elemental analyses were recorded on a Carlo-Erba EA1110CNNO-S analyzer. All mechanochemical reactions were carried out using a Retsch MM400 vibrational ball mill, equipped with Retsch 25 ml screw-top vessels, containing a 13.6-g stainless steel ball of 15 mm diameter unless otherwise stated. The operating frequency was set at 25 Hz for each experiment. The products were dried in a Carbolite PF60 oven set at 80°C.
Synthesis of Silica-Coated Fe3O4 (Fe3O4@SiO2@Tannic Acid) MNPs
A. Synthesis of Fe3O4 MNPs and Fe3O4@SiO2-Cl MNPs
The Fe3O4 and Fe3O4@SiO2‐Cl MNPs were synthesized by the research group (Nikpassand et al., 2015; Nikpassand et al., 2017b).
B. Synthesis of Fe3O4@SiO2@Tannic Acid Nanoparticles
Then Fe3O4@SiO2-Cl MNPs, tannic acid, and 15 ml of distilled water were stirred for 24 h. Then, 4 ml of 10% NaOH was added to the reaction mixture and stirred for 5 h. Then, 5 ml of triethylamine was added to the reaction mixture, and after stirring with a magnet, it was separated and incubated in the oven at 50°C for 24 h (Figure 1). The structure of nanocatalysts was confirmed by FT-IR, XRD, EDX, VSM, TEM, and SEM techniques (Figures 2–8).
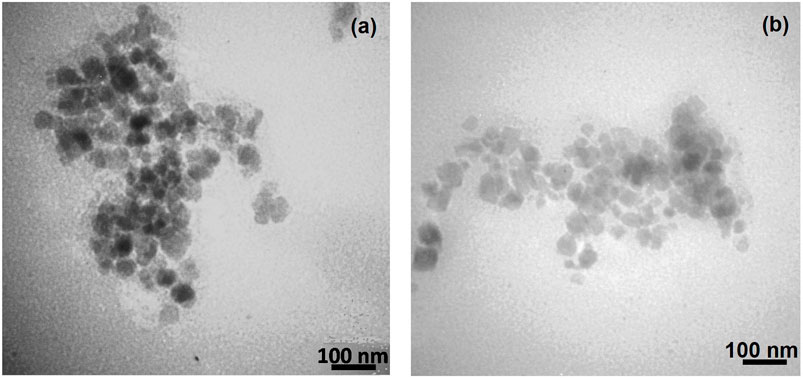
FIGURE 5. TEM images of synthesized Fe3O4@SiO2@Tannic acid MNPs: (a) after one cycle of reaction; (b) after six cycles of reaction.
General Procedure for Preparation of 5-Amino-Pyrazole-4-Carbonitriles 4a-k
A mixture of synthesized azo-linked salicylaldehyde (1 mmol), phenylhydrazine (1 mmol, 0.108 g), or paratolylhydrazine (1 mmol, 0.120 g), malononitrile (1 mmol, 0.065 g), and 0.1 g Fe3O4@SiO2@Tannic acid was added to a Retsch 50-ml screw-top vessel equipped with a 20-mm stainless steel ball, and set to shake for the required reaction time (Table 2). Ball milling was performed at 20–25 Hz frequency at room temperature for the time given in Table 2. The progress of the reaction was investigated by thin-layer chromatography (TLC Silica gel 60 F₂₅₄, ethyl acetate: n-hexane 1: 2). After completion of the reaction, the resulting mixture was dissolved in hot ethanol (20 ml), and the catalyst was separated by a 1.4 T external magnet and washed with hot distilled water (5 ml) and ethanol (5 ml) two times. The resulting 3-pyrazolyl-4H-1,2,4-triazole was isolated and purified using column chromatography (silica gel 60 (0.063–0.200 mm); ethyl acetate: n-hexane 1: 2).
Characterization Data
5-Amino-3-(5-((4-chlorophenyl)diazenyl)-2-hydroxyphenyl)-1-phenyl-1H-pyrazole-4-carbonitrile (4a); yellow solid, m.p.: 235–237°C, FT-IR (KBr, cm−1) νmax 3,292 (N-H stretch), 3,064 (O-H stretch), 2,390 (C≡N stretch), 1,602 (N=N stretch), 1,566, 1,544, and 1,492 (C=C stretch), 1,276 (C-N stretch), 1,255 (C-O stretch), and 1,002 (C-Cl stretch) cm−1. 1H NMR (500 MHz, DMSO-d6): δ (ppm): 6.82 (t, J = 7.2 Hz, 1H), 7.04–7.11 (m, 4H), 7.28 (t, J = 7.5 Hz, 2H), 7.66 (dd, J = 6.3, 2.1 Hz, 2H), 7.80 (dd, J = 8.7, 2.4 Hz, 1H), 7.90 (dd, J = 6.3, 2.1 Hz, 2H), 8.25 (s, 2H, NH2), and 10.60 (s, 1H, OH) ppm. 13C NMR (125 MHz, DMSO-d6): δ (ppm): 112.3, 117.2, 119.6, 122.2, 122.3, 123.9, 124.4, 129.7, 129.9, 134.4, 135.1, 135.5, 142.6, 143.2, 145.1, 145.7, 151.1, and 159.2 ppm. Anal. calcd for C22H15ClN6O: C, 63.69; H, 3.64; and N, 20.26. Found: C, 63.72; H, 3.63; and N, 20.25.
5-Amino-3-(5-((2-chlorophenyl)diazenyl)-2-hydroxyphenyl)-1-phenyl-1H-pyrazole-4-carbonitrile (4b); brown solid, m.p.: 176–178°C, FT-IR (KBr, cm−1) νmax 3,288 (N-H stretch), 3,060 (O-H stretch), 2,390 (C≡N stretch), 1,602 (N=N stretch), 1,566 and 1,494 (C=C stretch), 1,276 (C=N stretch), 1,255 (C-O stretch), and 1,029 (C-Cl stretch) cm−1. 1H NMR (500 MHz, DMSO-d6): δ (ppm): 4.50 (s, 2H, NH2), 6.80 (t, J = 7.8 Hz, 1H), 7.06 (d, J = 7.8 Hz, 2H), 7.16 (d, J = 7.8 Hz, 1H), 7.27 (t, J = 7.5 Hz, 2H), 7.36–7.55 (m, 2H), 7.68 (dd, J = 7.5, 1.2 Hz, 2H), 7.80 (dd, J = 7.8, 2.4 Hz, 1H), and 8.28 (s, 1H, OH) ppm. 13C NMR (125 MHz, DMSO-d6): δ (ppm): 112.3, 112.5, 117.3, 118.00, 119.6, 122.3, 123.2, 123.6, 128.4, 129.7, 130.2, 131.1, 132.2, 133.8, 135.0, 145.2, 146.1, 148.5, and 159.5 ppm. Anal. calcd for C22H15ClN6O: C, 63.69; H, 3.64; and N, 20.26. Found: C, 63.67; H, 3.65; and N, 20.28.
5-Amino-3-(2-hydroxy-5-((2-methyl-4-nitrophenyl)diazenyl)phenyl)-1-phenyl-1H-pyrazole-4-carbonitrile (4c); red solid, m.p.: 193–195°C, FT-IR (KBr, cm−1) νmax 3,421 (N-H stretch), 3,330 (O-H stretch), 2,196 (C≡N stretch), 1,683 (N=N stretch), 1,600 and 1,564 (C=C stretch), 1,519 (NO2 stretch), 1,492 (C=C stretch), 1,334 (NO2 stretch), and 1,255 (C-O stretch) cm−1. 1H NMR (500 MHz, DMSO-d6): δ (ppm): 2.42 (s, 3H, CH3), 6.81 (t, J = 7.2 Hz, 1H), 7.04–7.10 (m, 3H), 7.27 (t, J = 8.1 Hz, 2H), 7.68 (d, J = 9.0 Hz, 1H), 7.80 (dd, J = 9.0, 2.7 Hz, 1H), 8.12 (dd, J = 8.7, 2.7 Hz, 1H), 8.22 (s, 1H), 8.26 (d, J = 2.1 Hz, 1H), and 10.61 (s, 1H, OH) ppm. 13C NMR (125 MHz, DMSO-d6): δ (ppm): 25.51, 112.3, 117.0, 117.3, 118.4, 119.6, 122.3, 122.5, 122.9, 123.7, 123.8, 126.6, 129.7, 134.9, 138.5, 144.5, 145.1, 146.4, 148.0, 154.0, 154.0, and 159.9 ppm. Anal. calcd for C23H17N7O3: C, 62.87; H, 3.90; and N, 22.31. Found: C, 62.87; H, 3.89; and N, 22.33.
5-Amino-3-(2-hydroxy-5-((4-nitrophenyl)diazenyl)phenyl)-1-phenyl-1H-pyrazole-4-carbonitrile (4d); red solid, m.p.: 149–151°C, FT-IR (KBr, cm−1) νmax 3,461 (N-H stretch), 3,307 (N-H stretch), 3,037 (O-H stretch), 2,189 (C≡N stretch), 1,662 (N=N stretch), 1,641, 1,598, and 1,566 (C=C stretch), 1,515 and 1,340 (NO2 stretch), and 1,251 (C-O stretch) cm−1. 1H NMR (500 MHz, DMSO-d6): δ (ppm): 6.78–7.30 (m, 6H), 7.49–7.54 (m, 1H), 7.96–8.02 (m, 2H), 8.16 (s, 1H), 8.23–8.25 (m, 1H), 8.34–8.39 (m, 1H), and 10.27 (s, 1H, OH) ppm. 13C NMR (125 MHz, DMSO-d6): δ (ppm): 112.1, 112.3, 116.3, 117.6, 119.4, 119.8, 119.9, 120.8, 123.5, 125.3, 127.8, 129.6, 129.7, 136.8, 137.8, 145.0, 145.1, and 156.1 ppm. Anal. calcd for C22H15N7O3: C, 62.11; H, 3.55; and N, 23.05. Found: C, 62.09; H, 3.57; and N, 23.06.
5-Amino-3-(5-((4-bromophenyl)diazenyl)-2-hydroxyphenyl)-1-phenyl-1H-pyrazole-4-carbonitrile (4e); off-white solid, m.p.: 218–220°C, FT-IR (KBr, cm−1) νmax 3,433 (N-H stretch), 3,321 (N-H stretch), 3,051 (O-H stretch), 2,190 (C≡N stretch), 1,683 (N=N stretch), 1,658 and 1,568 (C=C stretch), 1,255 (C-O stretch), and 1,002 (C-Br stretch) cm−1. 1H NMR (500 MHz, DMSO-d6): δ (ppm): 6.79 (t, J = 6.9 Hz, 1H), 7.04–7.11 (m, 2H), 7.28 (t, J = 5.8 Hz, 2H), 7.76–7.84 (m, 5H), 8.24–8.26 (m, 2H), and 10.60 (s, 1H, OH) ppm. 13C NMR (125 MHz, DMSO-d6): δ (ppm): 112.1, 112.3, 117.2, 119.6, 122.2, 122.4, 123.9, 124.6, 124.8, 129.7, 132.8, 133.1, 135.3, 145.1, 145.7, 151.4, 156.1, and 159.2 ppm. Anal. calcd for C22H15BrN6O: C, 57.53; H, 3.29; and N, 18.30. Found: C, 57.55; H, 3.30; and N, 18.29.
5-Amino-3-(2-hydroxy-5-((4-methoxyphenyl)diazenyl)phenyl)-1-phenyl-1H-pyrazole-4-carbonitrile (4f); yellow solid, m.p.: 184–186°C, FT-IR (KBr, cm−1) νmax 3,419 (N-H stretch), 3,315 (O-H stretch), 2,189 (C≡N stretch), 1,686 (N=N stretch), 1,647, 1,600, and 1,577 (C=C stretch), and 1,244 (C-O stretch) cm−1. 1H NMR (500 MHz, DMSO-d6): δ (ppm): 3.85 (s, 3H, CH3O), 6.82 (t, J = 7.2 Hz, 1H), 7.09–7.20 (m, 5H), 7.29 (t, J = 7.6 Hz, 2H), 7.71–7.77 (m, 1H), 7.88 (d, J = 8.4 Hz, 2H), 8.19–8.29 (m, 1H), and 10.61 (s, 1H, OH) ppm. 13C NMR (125 MHz, DMSO-d6): δ (ppm): 55.9, 112.3, 114.9, 115.1, 115.6, 117.1, 119.6, 121.8, 122.1, 123.4, 124.1, 124.6, 129.7, 130.7, 136.1, 145.1, 145.8, 146.7, and 158.4 ppm. Anal. calcd for C23H18N6O2: C, 67.31; H, 4.42; and N, 20.48. Found: C, 67.33; H, 4.41; and N, 20.50.
5-Amino-3-(5-((4-chlorophenyl)diazenyl)-2-hydroxyphenyl)-1-(p-tolyl)-1H-pyrazole-4-carbonitrile (4 g); brown solid, m.p.: 234–236°C, FT-IR (KBr, cm−1) νmax 3,292 (N-H stretch), 3,064 (O-H stretch), 2,339 (C≡N stretch), 1,602 (N=N stretch), 1,1566, 1,544 and 1,452 (C=C stretch), 1,276 (C-N stretch), 1,255 (C-O stretch), and 1,002 (C-Cl stretch) cm−1. 1H NMR (500 MHz, DMSO-d6): δ (ppm): 2.25 (s, 3H, CH3), 6.94 (d, J = 8.4 Hz, 2H), 7.08–7.11 (m, 4H), 7.66 (dt, J = 8.4, 3.0 Hz, 2H), 7.78 (dd, J = 8.6, 2.4 Hz, 1H), 7.91 (dt, J = 8.4, 3.0 Hz, 2H), 8.21–8.22 (s, 2H), and 10.49 (s, 1H, OH) ppm. 13C NMR (125 MHz, DMSO-d6): δ (ppm): 20.7, 112.4, 117.1, 122.3, 122.4, 123.3, 123.7, 124.3, 128.2, 129.9, 130.2, 132.5, 134.7, 135.5, 137.6, 142.8, 145.7, 151.1, and 159.1 ppm. Anal. calcd for C23H17ClN6O: C, 64.41; H, 4.00; and N, 19.60. Found: C, 64.39; H, 3.99; and N, 19.63.
5-Amino-3-(2-hydroxy-5-((2-methyl-4-nitrophenyl)diazenyl)phenyl)-1-(p-tolyl)-1H-pyrazole-4-carbonitrile (4h); brown solid, m.p.: 190–192°C, FT-IR (KBr, cm−1) νmax 3,423 and 3,328 (N-H stretch), 3,218 (O-H stretch), 2,268 (C≡N stretch), 1,681 (N=N stretch), 1,664 (C=N stretch), 1,618, 1,583, and 1,564 (C=C stretch), 1,515 and 1,340 (NO2 stretch), and 1,255 (C-O stretch) cm−1.1H NMR (500 MHz, DMSO-d6): δ (ppm): 2.33 (s, 3H, CH3), 2.74 (s, 3H, CH3), 4.51 (s, 2H, NH2), 6.94 (d, J = 8.4 Hz, 2H), 7.06–7.18 (m, 3H), 7.66 (d, J = 8.4 Hz, 1H), 7.78 (dd, J = 8.7 2.4 Hz, 1H), 8.15 (dd, J = 8.7, 2.7 Hz, 1H), 8.20 (s, 1H), 8.24 (d, J = 2.4 Hz, 1H), and 10.19 (s, 1H, OH) ppm. 13C NMR (125 MHz, DMSO-d6): δ (ppm): 17.4, 20.6, 112.4, 112.5, 112.7, 117.0, 117.3, 122.4, 122.5, 123.4, 123.7, 124.4, 126.6, 128.2, 130.1, 134.4, 138.5, 142.9, 146.4, 148.0, 154.1, and 159.9 ppm. Anal. calcd for C24H19N7O3: C, 63.57; H, 4.22; and N, 21.62. Found: C, 63.59; H, 4.20; and N, 21.63.
5-Amino-3-(5-((2-chlorophenyl)diazenyl)-2-hydroxyphenyl)-1-(p-tolyl)-1H-pyrazole-4-carbonitrile (4i); brown solid, m.p.: 172–174°C, FT-IR (KBr, cm−1) νmax 3,218 (N-H stretch), 3,035 (O-H stretch), 2,290 (C≡N stretch), 1,667 (N=N stretch), 1,664, 1,612, and 1,581 (C=C stretch), 1,247 (C-O stretch), and 1,056 (C-Cl stretch) cm−1. 1H NMR (500 MHz, DMSO-d6): δ (ppm): 2.23 (s, 3H, CH3), 7.53 (s, 2H, NH2), 6.92–7.10 (m, 5H), 7.46–7.56 (m, 3H), 7.68–7.78 (m, 2H), 8.22 (s, 1H), and 10.23 (s, 1H, OH) ppm. 13C NMR (125 MHz, DMSO-d6): δ (ppm): 20.7, 112.3, 112.5, 115.3, 118.0, 122.4, 123.6, 128.2, 128.4, 128.5, 129.7, 130.1, 131.0, 131.1, 133.7, 134.3, 134.5, 142.9, 146.1, 148.1, and 159.5 ppm. Anal. calcd for C23H17ClN6O: C, 64.41; H, 4.00; and N, 19.60. Found: C, 64.39; H, 4.01; and N, 19.59.
5-Amino-3-(2-hydroxy-5-((4-nitrophenyl)diazenyl)phenyl)-1-(p-tolyl)-1H-pyrazole-4-carbonitrile (4j); brown solid, m.p.: 131–133°C, FT-IR (KBr, cm−1) νmax 3,477 and 3,309 (N-H stretch), 3,045 (O-H stretch), 2,360 (C≡N stretch), 1,614 (N=N stretch), 1,589, 1,564, and 1,488 (C=C stretch), 1,402 (NO2 stretch), and 1,271 (C-O stretch) cm−1. 1H NMR (500 MHz, DMSO-d6): δ (ppm): 12.23 (s, 3H, CH3), 4.52 (s, 2H, NH2), 6.87–6.98 (m, 1H),7.05–7.09 (m, 1H), 7.14–7.20 (m, 1H), 7.37 (s, 1H), 7.49–7.54 (m, 2H), 7.80–7.84 (dd, J = 8.6, 1.8 Hz, 1H), 8.05 (d, J = 8.6 Hz, 1H), 8.14 (s, 1H), 8.23–8.27 (m, 1H), 8.40 (d, J = 8.6 Hz, 1H), and 8.92 (s, 1H, OH) ppm. 13C NMR (125 MHz, DMSO-d6): δ (ppm): 20.7, 112.2, 112.4, 112.5, 116.3, 119.7, 120.9, 122.6, 123.5, 125.4, 127.7, 128.0, 129.4, 130.1, 137.3, 142.9, 145.9, 148.3, and 156.1 ppm. Anal. calcd for C23H17N7O3: C, 62.87; H, 3.90; and N, 22.31. Found: C, 62.85; H, 3.88; and N, 22.33.
5-Amino-3-(5-((4-bromophenyl)diazenyl)-2-hydroxyphenyl)-1-(p-tolyl)-1H-pyrazole-4-carbonitrile (4k); yellow solid, m.p.: 180–183°C, FT-IR (KBr, cm−1) νmax 3,218 (N-H stretch), 3,037 (O-H stretch), 2,366 (C≡N stretch), 1,668 (N=N stretch), 1,573, 1,517, and 1,483 (C=C stretch), 1,280 (C-O stretch), and 1,006 (C-Br stretch) cm−1.1H NMR (500 MHz, DMSO-d6): δ (ppm): 2.22 (s, 3H, CH3), 4.56 (s, 2H, NH2), 6.93–6.98 (m, 3H),7.05–7.09 (m, 3H), 7.14 (d, J = 8.7 Hz, 1H), 7.27–7.81 (m, 2H), 8.17 (d, J = 2.4 Hz, 1H), and 8.26 (s, 1H) ppm. 13C NMR (125 MHz, DMSO-d6): δ (ppm): 20.6, 112.4, 112.5, 115.3, 117.2, 122.3, 124.5, 126.1, 128.1, 129.7, 130.1, 130.8, 132.8, 134.6, 142.9, 143.6, 145.6, 151.4, and 159.3 ppm. Anal. calcd for C23H17BrN6O: C, 58.36; H, 3.62; and N, 17.76. Found: C, 58.34; H, 3.63; and N, 17.77.
Results and Discussion
Synthesis and Characterization of Fe3O4@SiO2@Tannic Acid
In continuation of our research for the green synthesis of organic compounds (Nikpassand et al., 2012; Nikpassand et al., 2016; Nikpassand and Pirdelzendeh, 2016; Nikpassand et al., 2017; Aghazadeh and Nikpassand, 2019; Nikpassand et al., 2018; Masoumi Shahi et al., 2019; Zare Fekri et al., 2019; Nikpassand, 2020), herein we wish to report the synthesis of novel azo-linked 5-amino-pyrazole-4-carbonitriles catalyzed by tannic acid–functionalized silica-coated Fe3O4 nanoparticles (Fe3O4@SiO2@Tannic acid).
The structure of the Fe3O4@SiO2@Tannic acid nanoparticles is synthesized in three steps from existing commercial materials, as shown in Figure 2. Fe3O4@SiO2 core-shell structures were sequentially treated with 3-chloropropyltrimethoxysilane. Next, it was treated with tannic acid to produce Fe3O4@SiO2@Tannic acid (Figure 2).
FT-IR spectroscopy of Fe3O4@SiO2@Tannic acid MNPs was performed to identify the functional groups of the synthesized nanoparticles. The strong stretching bond at 3,409 cm−1 is related to the O-H stretching vibrations of the phenolic moiety of the nano-catalyst, and C=O stretching bands of carboxylic acid were shown at 1704 cm−1, which confirms the presence of tannic acid in the structure of nanoparticles. The bonds at 1,620, 1,506, and 1,453 cm−1 are assigned to the C=C stretching vibrations of the aromatic moiety. Also, vibrations of Si-O-Si bonds in the SiO2 shell were observed at 1,116 and 906 cm−1 (Figure 3).
The size and morphology of the Fe3O4@SiO2@Tannic acid MNPs were studied using transmission electron microscopy and field emission scanning electron microscopy (Figures 4, 5). The transmission electron microscope (TEM) and field emission scanning electron microscope (FE-SEM) images in Figures 4, 5 show that the Fe3O4@SiO2@Tannic acid nanoparticles have an almost spherical morphology with a particle size of 10–20 nm. In addition, TEM images show aggregation that confirms the successful bonding of tannic acid with magnetic nanoparticles (Figures 4, 5).
The data from the energy-dispersive X-ray spectroscopy (EDX) analysis of the synthesized Fe3O4@SiO2@Tannic acid MNPs confirm the nanoparticle structure. Thus, the presence of Fe (21.35 w/w %), O (52.38 w/w %), Si (0.36 w/w %), and C (25.92 w/w %) atoms in the structure proves the presence of Fe3O4 core in the structure of Fe3O4@SiO2@Tannic acid MNPs (Figure 6).
The VSM plot of the Fe3O4@SiO2@Tannic acid MNPs is presented in Figure 7. As can be seen, the saturation magnetization of the MNPs is smaller than that of the pure Fe3O4. VSM was measured during solid sampling at the tip of a vibrating rod at room temperature and analyzed in an applied magnetic field from −10 to 10 kOe (Figure 7).
XRD analysis of the Fe3O4@SiO2@Tannic acid catalyst in contact with pure Fe3O4 confirms the formation of Fe3O4 MNPs. This pattern shows characteristic peaks at 2θ = 21.3, 25.1, 35.2, 41.5, 43.4, 49.0, 50.4, 51.3, 55.7, 60.0, 63.2, 66.0, 67.4, 74.3, 75.5, and 78.3. These peaks indicate the pure face-centered cubic structure of Fe3O4, and the broad peak at 10–30° is related to Fe3O4 covered by SiO2 (Figure 8).
Catalytic Application
To evaluate the catalytic capability of the synthesized heterogeneous catalyst (Fe3O4@SiO2@Tannic acid) in organic reactions, we chose to examine its activity in a one-pot mechanochemical reaction between synthetized azo-linked aldehydes, malononitrile, and phenylhydrazine or p-tolylhydrazine (Scheme 1).
Initially, 5-((4-chlorophenyl)diazenyl)-2-hydroxybenzaldehyde 1a (1 mmol, 0.260 g), malononitrile 2 (1 mmol, 0.065 g), phenylhydrazine 3a (1 mmol, 0.108 g), and 0.1 g of Fe3O4@SiO2@Tannic acid were employed to produce 5-amino-3-(5-((4-chlorophenyl)diazenyl)-2-hydroxyphenyl)-1-phenyl-1H-pyrazole-4-carbonitrile (4a), and the effect of various factors such as the type of catalyst, its relative amount of raw material, and reaction temperature on this sample reaction was investigated (Tables 1–3).
Effect of Catalyst Type
To find the appropriate catalyst to synthesize the derivatives of azo-linked 5-amino-pyrazole-4-carbonitriles, the reaction of 5-((4-chlorophenyl)diazenyl)-2-hydroxybenzaldehyde 1a (1 mmol, 0.260 g), malononitrile 2 (1 mmol, 0.065 g), and phenylhydrazine 3a (1 mmol, 0.108 g) in the presence of 0.1 g of available catalysts at 80°C under different conditions was used, and the efficiency and reaction rates were compared (Table 1).
Effect of Fe3O4@SiO2@Tannic Acid Catalyst Value
The synthesis of product 4a with different amounts of Fe3O4@SiO2@Tannic acid at room temperature was investigated, and it was found that 0.1 g of the desired catalyst per 1 mmol of substrate gave a better yield in a shorter reaction time (Table 2).
To present the efficiency and generality of the mechanochemical reaction, various azo-linked aldehydes, malononitrile, and phenylhydrazine or p-tolylhydrazine were reacted in the presence of Fe3O4@SiO2@Tannic acid at room temperature (Scheme 1 and Table 3).
The recyclability and reusability of a catalyst were studied in the model one-pot mechanochemical reaction between various azo-linked aldehydes, diverse hydrazines, and malononitrile. At the end of the reaction, the separated catalyst can be reused after washing with warm EtOH and drying at 80°C. Fe3O4@SiO2@Tannic acid was used again for subsequent experiments under similar reaction conditions. The catalyst could be reused for the next cycle without any considerable loss of its activity. The yields of the product decreased only slightly after reusing the catalyst six times (Table 4). TEM images of the synthesized Fe3O4@SiO2@Tannic acid MNPs after one cycle of reaction and after six cycles of reaction are shown in Figure 5.
Conclusion
In conclusion, Fe3O4@SiO2@Tannic acid was synthesized and investigated as a new, environmentally friendly, inexpensive, mild, and reusable catalyst for the mechanochemical synthesis of azo-linked 5-amino-pyrazole-4-carbonitriles. High yield, a simple work-up procedure, observance of green chemistry principles, eco-friendly procedure using natural ingredients, ease of separation, recyclability of the magnetic catalyst, and waste reduction are some advantages of this method.
Data Availability Statement
The original contributions presented in the study are included in the article/Supplementary Material. Further inquiries can be directed to the corresponding author.
Author Contributions
MN carried out experimental studies and wrote the original draft and analyzed spectral characterization of synthesized molecules and project planning, proofreading, and editing.
Conflict of Interest
The authors declare that the research was conducted in the absence of any commercial or financial relationships that could be construed as a potential conflict of interest.
Publisher’s Note
All claims expressed in this article are solely those of the authors and do not necessarily represent those of their affiliated organizations, or those of the publisher, the editors, and the reviewers. Any product that may be evaluated in this article, or claim that may be made by its manufacturer, is not guaranteed or endorsed by the publisher.
Acknowledgments
Financial support from the Research Council of Rasht Branch, Islamic Azad University, Rasht, Iran, is sincerely acknowledged.
Supplementary Material
The Supplementary Material for this article can be found online at: https://www.frontiersin.org/articles/10.3389/fchem.2021.724745/full#supplementary-material
References
Aghazadeh, B., and Nikpassand, M. (2019). 2-Amino Glucose" as a Substrate for Synthesis of Magnetically Recoverable Nanocatalyst NiFe2O4@SiO2@amino Glucose for the green Synthesis of Novel Bis (1,2-Dihydro-4-Hydroxy-2-Oxoquinolin-3-Yl)methanes. Carbohydr. Res. 483, 107755. doi:10.1016/j.carres.2019.107755
Al-Qalaf, F., Mandani, F., Abdelkhalik, M. M., and Bassam, A. A. (2009). Synthesis of 5-Substituted 3-Amino-1h-Pyrazole-4-Carbonitriles as Precursors for Microwave Assisted Regiospecific Syntheses of Pyrazolo[1,5-a]Pyrimidines. Molecules 14, 78–88. doi:10.3390/molecules14010078
Bai, G., Shi, L., Zhao, Z., Wang, Y., Qiu, M., and Dong, H. (2013). Preparation of a Novel Fe3O4@SiO2@Ni-La-B Magnetic Core-Shell Nanocomposite for Catalytic Hydrogenation. Mater. Lett. 96, 93–96. doi:10.1016/j.matlet.2013.01.018
Bekhit, A. A., and Abdel-Aziem, T. (2004). Design, Synthesis and Biological Evaluation of Some Pyrazole Derivatives as Anti-inflammatory-antimicrobial Agents. Bioorg. Med. Chem. 12, 1935–1945. doi:10.1016/j.bmc.2004.01.037
Bhale, P. S., Sakharam, B., and Chanshetti, U. B. (2014). Simple Grinding, Catalyst-free, One-Pot, Three-Component Synthesis of Polysubstituted Amino Pyrazole. Res. J. Chem. Sci. 4, 16–21.
El-Emary, T. I. (2006). Synthesis and Biological Activity of Some New Pyrazolo[3,4-b]Pyrazines. Jnl Chin. Chem. Soc. 53, 391–401. doi:10.1002/jccs.200600050
Eslahi, H., Sardarian, A. R., and Esmaeilpour, M. (2021). Green and Sustainable Palladium Nanomagnetic Catalyst Stabilized by Glucosamine-Functionalized Fe3O4@SiO2 Nanoparticles for Suzuki and Heck Reactions. Appl. Organomet. Chem. 34, e6260
Fardood, S. T., Ramazani, A., and Moradi, S. (2017). Green Synthesis of Ni-Cu-Mg Ferrite Nanoparticles Using Tragacanth Gum and Their Use as an Efficient Catalyst for the Synthesis of Polyhydroquinoline Derivatives. J. Sol-gel Sci. Technol. 82, 432–439. doi:10.1007/s10971-017-4310-6
Fihri, A., Bouhrara, M., Nekoueishahraki, B., Basset, J.-M., and Polshettiwar, V. (2011). Nanocatalysts for Suzuki Cross-Coupling Reactions. Chem. Soc. Rev. 40, 5181–5203. doi:10.1039/C1CS15079K
Hamedi, R., B.G. Aghaie, A., and Hadjmohammadi, M. R. (2018). Magnetic Core Micelles as a Nanosorbent for the Efficient Removal and Recovery of Three Organophosphorus Pesticides from Fruit Juice and Environmental Water Samples. J. Sep. Sci. 41, 2037–2045. doi:10.1002/jssc.201701090
Inaloo, I. D., Majnooni, S., Eslahi, H., and Esmaeilpour, M. (2020). Air-Stable Fe3O4@SiO2-EDTA-Ni(0) as an Efficient Recyclable Magnetic Nanocatalyst for Effective Suzuki-Miyaura and Heck Cross-Coupling via Aryl Sulfamates and Carbamates. Appl. Organomet. Chem. 34, e5662. doi:10.1002/aoc.5662
Karcı, F., and Karcı, F. (2008). Synthesis of Some Novel Pyrazolo[5,1-C][1,2,4]triazine Derivatives and Investigation of Their Absorption Spectra. Dyes Pigm. 76, 97–103. doi:10.1016/j.dyepig.2006.08.011
Kasimogullari, R., Zengin, B., Maden, M., Mert, S., and Kazaz, C. (2010). Synthesis of New Derivatives of 1-(3-Amino-Phenyl)-4- Benzoyl-5-Phenyl-1h-Pyrazole-3-Carboxylic Acid. Jscs 75, 1625–1635. doi:10.2298/JSC101018135K
Kiyani, H., Samimi, H. A., Ghorbani, F., and Esmaieli, S. (2013). One-pot, Four-Component Synthesis of Pyrano[2,3-C]pyrazoles Catalyzed by Sodium Benzoate in Aqueous Medium. Curr. Chem. Lett. 2, 197–206. doi:10.5267/j.ccl.2013.07.002
Liu, X.-H., Cui, P., Song, B.-A., Bhadury, P. S., Zhu, H.-L., and Wang, S.-F. (2008). Synthesis, Structure and Antibacterial Activity of Novel 1-(5-Substituted-3-Substituted-4,5-Dihydropyrazol-1-Yl)ethanone Oxime Ester Derivatives. Bioorg. Med. Chem. 16, 4075–4082. doi:10.1016/j.bmc.2008.01.035
Martín, R., Rodríguez Rivero, M., and Buchwald, S. L. (2006). Domino Cu-Catalyzed C‐N Coupling/Hydroamidation: A Highly Efficient Synthesis of Nitrogen Heterocycles. Angew. Chem. Int. Ed. 45, 7079–7082. doi:10.1002/anie.200602917
Shahi, A. M., Nikpassand, M., and Fekri, L. Z. (2019). An Efficient and green Synthesis of New Benzo[f]chromenes Using 1,4-Disulfo-1,4-Diazoniabicyclo[2.2.2]Octane Chloride as a Novel Medium. Org. Prep. Proced. Int. 51, 521–529. doi:10.1080/00304948.2019.1666637
Nikpassand, M., and Pirdelzendeh, D. (2016). Green Synthesis of Novel Azo-Linked 2-phenyl Benzimidazoles Using Ionic Liquid [BDBDMIm]Br. Dyes Pigm. 130, 314–318. doi:10.1016/j.dyepig.2016.03.038
Nikpassand, M., Zare, L., and Saberi, M. (2012). Ultrasound-assisted L-Proline Catalyzed Synthesis of Novel Derivatives of Azo-Linked Dihydropyridines. Monatsh. Chem. 143, 289–293. doi:10.1007/s00706-011-0575-6
Nikpassand, M., Fekri, L., Karimian, L., and Rassa, M. (2015). Synthesis of Biscoumarin Derivatives Using Nanoparticle Fe3O4 as an Efficient Reusable Heterogeneous Catalyst in Aqueous media and Their Antimicrobial Activity. Curr. Org. Synth. 12, 358–362. doi:10.2174/1570179411666141101001949
Nikpassand, M., Fekri, L. Z., and Farokhian, P. (2016). An Efficient and green Synthesis of Novel Benzoxazole under Ultrasound Irradiation. Ultrason. Sonochem. 28, 341–345. doi:10.1016/j.ultsonch.2015.08.014
Nikpassand, M., Fekri, L. Z., and Nabatzadeh, M. (2017). Fe3O4@SiO2@KIT-6 as an Efficient and Reusable Catalyst for the Synthesis of Novel Derivatives of 3,3'-((Aryl-1-Phenyl-1h-Pyrazol-4- Yl)methylene)bis (1H-Indole). Cchts 20, 533–538. doi:10.2174/1386207320666170425123248
Nikpassand, M., Zare Fekri, L., and Sanagou, S. (2017). Green Synthesis of 2-Hydrazonyl-4-Phenylthiazoles Using KIT-6 Mesoporous Silica Coated Magnetite Nanoparticles. Dyes Pigm. 136, 140–144. doi:10.1016/j.dyepig.2016.08.044
Nikpassand, M., Fekri, L. Z., and Pourahmad, A. (2018). One-pot Synthesis of New Azo-Linked 4H-Benzo[d][1,3]Oxazine-2,4-Diones from Carbon Dioxide Using CuO@RHA/MCM-41 Nanocomposite in Green Media. J. CO2 Util. 27, 320–325. doi:10.1016/j.jcou.2018.08.011
Nikpassand, M. (2020). NiFe2O4@SiO2@glucose Amine Nanoparticle Catalyzed Reaction of Azo-Linked Thiosalicylic Acid with CO2: Access to Azo-Linked Benzo[d]Oxathiine-2,4-Diones. Dyes Pigm. 173, 107936. doi:10.1016/j.dyepig.2019.107936
Díaz-Ortiz, Á., de Cózar, A., Prieto, P., de la Hoz, A., and Moreno, A. (2006). Recyclable Supported Catalysts in Microwave-Assisted Reactions: First Diels-Alder Cycloaddition of a Triazole Ring. Tetrahedron Lett. 47, 8761–8764. doi:10.1016/j.tetlet.2006.10.006
Polshettiwar, V., and Varma, R. S. (2010). Green Chemistry by Nano-Catalysis. Green. Chem. 12, 743–754. doi:10.1039/B921171C
Pourjavadi, A., Hosseini, S. H., Doulabi, M., Fakoorpoor, S. M., and Seidi, F. (2012). Multi-Layer Functionalized Poly(Ionic Liquid) Coated Magnetic Nanoparticles: Highly Recoverable and Magnetically Separable Brønsted Acid Catalyst. ACS Catal. 2, 1259–1266. doi:10.1021/cs300140j
Rezaei, A., Ramazani, A., Gouranlou, F., and Joo, S. (2017). Silica Nanoparticles/nanosilica Sulfuric Acid as a Reusable Catalyst for Fast, Highly Efficient and green Synthesis of 2-(Heteroaryl)Acetamide Derivatives. Loc 14, 86–92. doi:10.2174/1570178614666170126154256
Salaheldin, A. M., Oliveira-Campos, A. M. F., and Rodrigues, L. M. (2007). N-bromosuccinimide Assisted Oxidation of 5-aminopyrazoles: Formation of Bis Diazenylderivatives. Tetrahedron Lett. 48, 8819–8822. doi:10.1016/j.tetlet.2007.10.079
Saleh, T. S., Eldebss, T. M. A., and Albishri, H. M. (2012). Ultrasound Assisted One-Pot, Three-Components Synthesis of Pyrimido[1,2-A]benzimidazoles and Pyrazolo[3,4-B]pyridines: A New Access via Phenylsulfone Synthon. Ultrason. Sonochem. 19, 49–55. doi:10.1016/j.ultsonch.2011.05.003
Sardarian, A. R., Eslahi, H., and Esmaeilpour, M. (2019). Green, Cost-Effective and Efficient Procedure for Heck and Sonogashira Coupling Reactions Using Palladium Nanoparticles Supported on Functionalized Fe3O4@SiO2 by Polyvinyl Alcohol as a Highly Active, Durable and Reusable Catalyst”. Appl. Organomet. Chem. 33, e4856. doi:10.1002/aoc.4856
Takagi, K., Tanka, M., Murakami, Y., Ogura, K., Ishii, K., Morita, H., et al. (1987). Synthesis of nitro and Amino N‐heterocycles via Ring Transformation of 2‐methyl‐3‐nitrochromone. J. Hetero. Chem. 24, 1003–1007. doi:10.1002/jhet.5570240420
Tominaga, Y., Matsuoka, Y., Oniyama, Y., Uchimura, Y., Komiya, H., Hirayama, M., et al. (1990). Polarized Ethylenes. IV. Synthesis of Polarized Ethylenes Using Thioamides and Methyl Dithiocarboxylates and Their Application to Syntheses of Pyrazoles, Pyrimidines, Pyrazolo[3,4-D]pyrimidines, and 5-aza[2.2.3]cyclazines. J. Hetero. Chem. 27, 647–660. doi:10.1002/jhet.5570270332
Towne, E. B., Moore, W. H., and Dickey, J. B. (1968). White (Eastman Kodak Co.), US-Pat. 3.336–285, Chem. Abstr. 68, 14072 r. (15.8.1967) doi:10.1016/b978-0-08-003566-6.50034-1
Trofimov, B. A., Mal’kina, A. G., Borisova, A. P., Nosyreva, V. V., Shemyakina, O. A., Kazheva, O. N., et al. (2008). Expedient Synthesis of Pyrazoles Substituted with Amino, Hydroxyl and Thioamide Groups. Tetrahedron Lett. 49, 3104–3107. doi:10.1016/j.tetlet.2008.03.046
Wu, Y.-C., Chen, Y.-J., Li, H.-J., Zou, X.-M., Hu, F.-Z., and Yang, H.-Z. (2006). Synthesis of Trifluoromethyl-Promoted Functional Pyrazolo[1,5-A]pyrimidine and Pyrazolo[5,1-D][1,2,3,5]tetrazine-4(3h)-Ones. J. Fluorine Chem. 127, 409–416. doi:10.1016/j.jfluchem.2006.02.001
Lv, M., Xin, Q., Yin, D., Jia, Z., Yu, C., Wang, T., et al. 2020). Magnetically Recoverable Bifunctional Catalysts for the Conversion of Cellulose to 1,2-Propylene Glycol. ACS Sustainable Chem. Eng. 8, 3617–3625. doi:10.1021/acssuschemeng.9b06264
Fekri, L. Z., Nikpassand, M., and Khakshoor, S. N. (2019). Green, Effective and Chromatography Free Synthesis of Benzoimidazo[1,2-A]pyrimidine and Tetrahydrobenzo [4,5]imidazo [1,2-D]quinazolin-1(2h)-One and Their Pyrazolyl Moiety Using Fe3O4@SiO2@ -Proline Reusable Catalyst in Aqueous Media. J. Organomet. Chem. 894, 18–27. doi:10.1016/j.jorganchem.2019.05.004
Zhao, Y., Li, J., Zhao, L., Zhang, S., Huang, Y., Wu, X., et al. (2014). Synthesis of Amidoxime-Functionalized Fe3O4@SiO2 Core-Shell Magnetic Microspheres for Highly Efficient Sorption of U(VI). Chem. Eng. J. 235, 275–283. doi:10.1016/j.cej.2013.09.034
Keywords: 5-amino-pyrazole-4-carbonitriles, Fe3O4@SiO2@Tannic acid, malononitrile, three-component reaction, tannic acid
Citation: Pashaki FS and Nikpassand M (2021) Synthesis of Novel Azo-Linked 5-Amino-Pyrazole-4-Carbonitrile Derivatives Using Tannic Acid–Functionalized Silica-Coated Fe3O4 Nanoparticles as a Novel, Green, and Magnetically Separable Catalyst. Front. Chem. 9:724745. doi: 10.3389/fchem.2021.724745
Received: 14 June 2021; Accepted: 27 September 2021;
Published: 12 November 2021.
Edited by:
Suresh Maddila, Gandhi Institute of Technology and Management (GITAM), IndiaReviewed by:
William Brittain, Durham University, United KingdomNagaraju Kerru, Gandhi Institute of Technology and Management (GITAM), India
Palakondu Lavanya, Annamacharya Institute of Technology and Sciences, India
Copyright © 2021 Pashaki and Nikpassand. This is an open-access article distributed under the terms of the Creative Commons Attribution License (CC BY). The use, distribution or reproduction in other forums is permitted, provided the original author(s) and the copyright owner(s) are credited and that the original publication in this journal is cited, in accordance with accepted academic practice. No use, distribution or reproduction is permitted which does not comply with these terms.
*Correspondence: Mohammad Nikpassand, TmlrcGFzc2FuZEBpYXVyYXNodC5hYy5pcg==