- 1Polymers and Functional Materials Division, CSIR-Indian Institute of Chemical Technology, Hyderabad, India
- 2Academy of Scientific and Innovative Research (AcSIR), Ghaziabad, India
- 3Advanced Centre of Research in High Energy Materials (ACRHEM), University of Hyderabad, Hyderabad, India
A new series of non-aqueous phthalocyanines having 3,4,5-trimethoxy phenyl group at peripheral positions in which the central cavity possessing Cu(II), Zn(II), and without metals has been synthesized, and its absorption, fluorescence (steady-state and excited state lifetimes), electrochemical, and third-order nonlinear optical (NLO) properties were evaluated. Absorption studies data suggest that all three phthalocyanines obey Beer–Lambert’s law, and the redox properties indicate that both oxidation and reduction reactions are macrocyclic centered. The singlet quantum yields were measured in different solvents and were found to be in the range of 0.2–0.5 in the case of free-base, whereas it was in the range of 0.1–0.5 in zinc derivative, while the time-resolved fluorescence data revealed lifetimes of typically a few ns. The third-order NLO properties were investigated using the Z-scan technique with kilohertz (for retrieving true electronic nonlinearities) and megahertz repetition rate femtosecond pulses at 800 nm. Intensity-dependent Z-scan studies revealed robust NLO coefficients for solutions and thin films (two-photon absorption cross-sections of 9,300–57,000 GM) of these molecules suggesting a strong potential for optical switching, imaging, and optical limiting applications.
Introduction
In the current era of optoelectronics, nonlinear optics (NLO) and related technologies play a significant role in its evolution (Dini et al., 2016; Gounden et al., 2020; Mrinalini et al., 2021). To revive the study of the potential of NLO in the field of telecommunication, photonics, biomedicine, and optical signal processing, several NLO moieties were designed through various π-conjugated organic molecules (Mardar, 2006; Bures et al., 2012; Sofiani et al., 2016; Biswas et al., 2020). For this reason, a great variety of small organic molecules and macrocyclic systems, such as porphyrins (Rao et al., 2000; Senge et al., 2007; Swain et al., 2014a; Katturi et al., 2020b; Rathi et al., 2020; Vijisha et al., 2020; Kumar et al., 2021a), phthalocyanines (de la Torre et al., 2004; Kumar et al., 2007; Swain et al., 2014b; Zhang et al., 2014; Bharati et al., 2018; Venkatram et al., 2018a; Venkatram et al., 2018b; Bhattacharya et al., 2019a; Bhattacharya et al., 2019b; Bhattacharya et al., 2021), corroles (Katturi et al., 2020a; Lu et al., 2021), porphycenes (Sarma et al., 2011; Swain et al., 2012; Pati et al., 2020), and BODIPY dyes (Ngoy et al., 2019), have been investigated for their NLO properties. Of these systems, we are particularly interested in phthalocyanine-based macrocyclic systems toward these applications. Phthalocyanines (Pcs) are large aromatic pigment molecules composed of four isoindole units linked by nitrogen atoms (Giribabu et al., 2008). Unlike porphyrins, phthalocyanines are human-made compounds which have a wide range of advantages in diversified areas such as sensor technology, catalysts, electrochromism, liquid crystals, photodynamic therapy, and energy storage devices to name a few (Gregory, 2000; Zagal et al., 2010; Tuncel et al., 2012; Kuzmina et al., 2019; Buyukeksi et al., 2020; Kumar et al., 2021b; Halaskova et al., 2021). Because of their electronic delocalization, innumerable properties arise which make them of great potential in various fields of science and technology. Despite all these applications, phthalocyanines are aggregates even at micromolar concentration due to planarity. In addition to this, the phthalocyanine macrocycle is insoluble in almost all organic solvents and is a major hurdle for many optoelectronic applications. To overcome these constraints, one has to incorporate alkyl or alkoxy chains either at the peripheral or non-peripheral positions of phthalocyanines (Singh et al., 2014). Furthermore, phthalocyanines offer for hosting more than 70 different elements in the central cavity. Phthalocyanines possess an absorption band in the vicinity of 350 nm (Soret band) and one or two bands in the 500–700 nm region (Q band/s) with high molar absorption coefficient (ε = 105) with high triplet state quantum yields and long lifetimes. Furthermore, the phthalocyanine macrocycle has rich electrochemistry, and it involves both oxidation and reduction reactions. The incorporation of alkyl or alkoxy groups as well as metals in its central cavity not only affects its optical but also its redox reactions.
Recent research trends have undeniably demonstrated the need for the development of optical limiters and nonlinear materials for the protection of sensitive optical devices and components from laser-induced damage (Dini et al., 2016). The study of optical nonlinearities, i.e., intense light when interacting with matter, is significant for the development of various photonic/optoelectronic devices. Generally, electronic distribution of the material does not get disturbed when light with low intensity falls on it, whereas when light with high intensity falls on the matter, it has enough power to pass the threshold limit which leads to the change in properties of the transmitted light. Optical nonlinearity arises at the molecular level due to the presence of delocalized π electrons. These electrons are easily polarized by the strong electric field of the input laser pulses. Phthalocyanines and metallated phthalocyanines (MPc) do have all the structural requirements for high polarizable π-systems and demonstrate large nonlinear optical (refraction/absorption) coefficients with ultrafast response times (typically in the sub-picosecond and femtosecond time scales). Along with these, Pcs also exhibit strong optical limiting mechanism which is observed due to phenomena such as reverse saturation absorption (RSA) and two-photon absorption (TPA) processes. Due to intersystem crossing (from S1 to T1 states) phthalocyanines exhibit strong RSA when excited with long (nanosecond) laser pulses. In this study, we designed, synthesized, and characterized a series of phthalocyanines possessing the 3,4,5-trimethoxy phenol group at their peripheral positions and Cu(II) or Zn(II) metals at their central cavities (see Figure 1 schematic depicting the synthesis procedure). The detailed optical, electrochemical, and NLO properties of these phthalocyanines are thoroughly studied. The NLO properties were evaluated in solution and thin film forms. The input wavelength was 800 nm and femtosecond (kHz and MHz repetition rates) laser pulses were used for the NLO studies. Complete NLO coefficients such as nonlinear refraction (n2), nonlinear absorption/two-photon absorption coefficients (β), third-order nonlinear optical susceptibility [χ(3)], and two-photon absorption cross-sections [σ2 or σTPA] were extracted from the Z-scan data.
Experimental Details
Materials: 4-Nitrophthalonitrile, 3,4,5-trimethoxyphenol, 1-pentanol, and 1,8-diazabicyclo[5.4.0] undec-7-ene (DBU) were procured from Sigma Aldrich and used without any further purification. Copper acetate, zinc acetate, and potassium carbonate were purchased from Finar Ltd. and used as such. All the solvents, dichloromethane (DCM), tetrahydrofuran (THF), dimethylformamide (DMF), toluene, n-hexane, acetonitrile, and methanol, were purchased from SD Fine Chemicals Ltd. and were dried before use.
Synthesis
3,4,5-Trimethoxyphenoxy phthalonitrile (1): 4-Nitrophthalonitrile (2 g, 11.56 mmol) and 3,4,5-trimethoxyphenol (1.57 g, 11.56 mmol) were taken in a clean dry round bottom flask. To this, potassium carbonate (8.32 g, 22.2 mmol) and dimethylformamide (25 ml) were added and stirred for 24 h at 60°C under N2 atmosphere. The reaction mixture was poured onto ice and the obtained product was filtered off. The obtained solid was further purified by silica gel column chromatography by eluting with hexane-ethyl acetate (85:15 v/v) to get the desired compound. Anal. calcd for C17H14N2O4%(310.10): C, 65.80; H, 4.55; N, 9.03. Found C, 65.84; H, 4.52; N, 9.01. 1H NMR (300 MHz, CDCl3): 7.75 (d, 2H), 8.20–8.40 (m, 3H), 3.90 (s, 3H), 3.85 (s, 6H).
Synthesis of TmPc: 3,4,5-Trimethoxyphenoxy phthalonitrile (0.20 g, 0.645 mmol) was taken in a clean dry 25 ml round bottom flask in which 1-pentanol was added and stirred at 200°C. After 15 min, DBU was added in catalytic amount and continued to reflux for 8 h under nitrogen atmosphere. The reaction was cooled down to room temperature and precipitated with methanol, and washed with hexane. It was further purified with silica gel column chromatography using dichloromethane. The obtained product was dried and the yield was 40%. Anal. calcd for C72H62N4O16%(1,239.30): C, 69.78; H, 5.04; N, 4.52. Found C, 69.80; H, 5.02; N, 5.51. MALDI-TOF MS calcd m/z (C72H62N4O16) 1,239.30, found 1,241.41.
Synthesis of ZnTmPc: 3,4,5-Trimethoxyphenoxy phthalonitrile (0.2 g, 0.645 mmol) and zinc acetate (0.20 g, 1.098 mmol) were taken in a clean dry 25 ml round bottom flask in which 1-pentanol was added and stirred at 200°C. After 15 min, DBU was added in catalytic amount and continued to reflux for 6 h under nitrogen atmosphere. The reaction was cooled down to room temperature and precipitated with methanol, and washed with hexane. It was further purified with silica gel column chromatography using dichloromethane. The obtained product was dried and the yield was 45%. Anal. calcd for C72H60N4O16Zn %(1,302.66): C, 66.39; H, 4.64; N, 4.30. Found C, 66.40; H, 4.02; N, 4.31. MALDI-TOF MS calcd m/z (C72H60N4O16Zn) 1,302.66, found 1,304.49.
Synthesis of CuTmPc: Similar procedure was followed as of Zn-TmPc; copper acetate was used instead of zinc acetate, and the yield was about 35%. Anal. calcd for C72H60N4O16Cu %(1,300.83): C, 66.48; H, 4.65; N, 4.31. Found C, 66.46; H, 4.62; N, 4.31. MALDI-TOF MS calcd m/z (C72H60N4O16Cu) 1,300.83, found 1,304.49.
Thin films: The thin films were prepared by coating 2 mM concentration solution of Pc dissolved in DCM on a glass plate. Glass plates of size 2.5 × 2.5 mm each were cut and washed with water and acetone under sonication and dried at 100°C. A dried glass plate was inserted on the base of the spin coater and 0.1 ml of solution was added using a micropipette onto the glass plate to obtain the thin film at a speed of 2000 rpm. Later, the glass plate was dried in a hot furnace at 60°C to obtain the thin films. The thickness was monitored using a profilometer, and the obtained values were in the range of 47–56 µm. See Supplementary Figure S18 for thickness data and Supplementary Figure S19 for absorption data of the thin films. It was observed that the Q and B bands of the absorption spectra in thin films were broadened and slightly red-shifted.
Nonlinear Optical Studies
Z-scan is a promising NLO technique found by Sheik Bahae and co-workers (1990). We have carried out the Z-scan experiments to probe the NLO properties of the title molecules extracting clear evidence about the sign and magnitude of NLO susceptibility [χ(3)] in an ultrafast excitation regime. Now, the input excitation beam being a Gaussian profile, the normalized transmittance can be mathematically followed up properly by bringing in the multi-photon absorption theory. In an open and closed aperture configuration, the normalized transmittance turns out to be attending the mathematical expressions as per the following equations. The generic multi-photon absorption-influenced transmittance nature (Kumar et al., 2007; Anusha et al., 2012; Bhattacharya et al., 2021) of the Z-scan process can be signified in a mathematical form as follows:
While in the case of TPA phenomena, it can be reduced to a simpler form as
And in the case of the closed aperture, it is supposed to be
So, here the sample effective is estimated by
Methods and Instrumentation
Characterization
All the 1H NMR spectra were recorded in CDCl3 solution using a spectrophotometer (AVANCE, 300 MHz). Matrix-assisted laser desorption ionization time-of-flight (MALDI–TOF) mass spectrometry measurements were performed on Shimadzu Biotech AximaPerformance 2.9.3.20110624: Mode was Reflectron-Hi Res, Power: 85 using TMS as standard. FT-IR spectra (with KBr pellets) were recorded on a Bruker spectrometer.
Optical Studies
A UV-Visible-NIR spectrophotometer (Shimadzu UV-3600) was used to record the absorption spectra of the title compounds. A spectrofluorometer (Fluorolog-3, Spex model, JobinYvon) was utilized to obtain the steady-state fluorescence spectra of solutions. The optical density at the excitation wavelength (λex) was ∼0.06. Zinc tert-butyl phthalocyanine (=0.37 in benzene) was used as a reference to calculate the fluorescence quantum yields (ϕ) by integrating their fluorescence bands (Lawrence and Whitten, 1996). Picosecond time-correlated single photon counting setup (consisting of FluoroLog3-Triple Illuminator, IBH Horiba JobinYvon) was employed to record the fluorescence lifetime data using a picosecond light emitting diode laser (NanoLED, λexof670 nm) as the source of excitation. The decay curves were obtained by from the fluorescence emission maxima of the phthalocyanine (λem = 700 nm). A photomultiplier tube (R928P from Hamamatsu) was used as the detector. The lamp profile was achieved by placing a scattered (dilute Ludox solution in water) in place of the sample. The width of the instrument function was limited by FWHM of the excitation source, which was ∼635 ps at 670 nm. The obtained decay curves were analyzed using nonlinear least-squares iteration procedure using a software [IBH DAS6 (version 2.3) decay analysis]. The quality of the fits was adjudicated by the χ2 values and the residual distribution.
Electrochemical Studies
Electrochemical measurements were performed on a potentiostat that was computer controlled [CH instruments, model CHI 620C]. The experiments were performed in solutions of respective phthalocyanines in DCM (using 1 mM concentration) and at a scan rate of 100 mV/s using 0.1 M tetrabutyl ammonium perchlorate as the supporting electrolyte. The working electrode was glassy carbon, and a standard calomel electrode was the reference electrode while a platinum wire was used as an auxiliary electrode. After cyclic voltammograms (CVs) have been recorded, ferrocene was added, and a second voltammogram was measured.
Theoretical Calculations
By using the Gaussian 09 package, loaded on a personal computer, all the theoretical analyses were performed (following Becke, 1993). The optimized energy minimized structures of all three phthalocyanines were found to be stable with global minimal energy. These studies were accomplished using the density functional theory (DFT) at B3LYP hybrid functional theory at 6-31G(d,p) basis set in the Gaussian program (see Peterson and Al-Laham, 1991; Frisch et al., 2009). The excited state properties such as 1) percentage of molecular contribution, 2) oscillatory strength, and 3) singlet transition energy in the tetrahydrofuran solvent were obtained by performing the time-dependent DFT (TD-DFT) calculations. The integral equation formalism polarizable continuum model within the self-consistent reaction field theory was used in the TD-DFT calculations to describe with M06-2x function and the solvation of the phthalocyanines in THF (Miertus et al., 1981; Cossi et al., 1996). GaussSum 2.2.5 was employed to simulate the most important portions of the absorption spectra and to construe the nature of various transitions (O’Boyle et al., 2008; Dennington et al., 2009).
Results and Discussions
The synthetic approach utilized for preparing three phthalocyanines is displayed in Figure 1. The compound 4-(3,4,5-trimethoxyphenoxy)phthalonitrile (1) was synthesized by the condensation of 3,4,5-trimethoxyphenol with 4-hydroxy phthalonitrile using K2CO3 in DMF. The cyclo tetramerization of 1 was achieved using DMF and pentanol method to get the free-base phthalocyanine (TmPc) and the corresponding metallo derivatives were achieved by using DBU, pentanol with respective metal acetates. All three phthalocyanines are characterized by various spectroscopic and electrochemical methods. The results obtained from the elemental analysis of all three compounds were found to be satisfactory. The presence of molecular ion peak in MALDI-Ms spectra of each compound confirmed the respective phthalocyanine (see Supplementary Figures S1–S8 for all the details).
Optical and Electrochemical Properties
It is well known in literature that phthalocyanine macrocycles are usually insoluble in organic solvents mainly due to the planarity of the macrocycle that promotes aggregation even at very low (micromolar) concentrations (Giribabu et al., 2008). This can be minimized by incorporation of various organic substituents at its peripheral position and metals at its central cavity (Singh et al., 2014). The optical absorption spectra of all three compounds, i.e., TmPc, CuTmPc, and ZnTmPc were measured in the DCM solvent and the respective absorption spectra are displayed in Figure 2A. The corresponding absorption maxima (λmax) and logarithmic value of the molar extinction coefficients (log ε) are presented in Table 1. The less intense peak in the 300-400 nm spectral region is attributed to the Soret band, which arises because of the transition from deeper π-levels to LUMO. In contrast, the intense peak(s) in 600-750 nm region belongs to the Q band, which arises due to well-known π-π* transitions. The lower intensity at higher energy side of Q band, i.e., 625 nm arises due to the aggregation of phthalocyanine. Metallophthalocyanines typically exhibit a single Q band in the visible spectral region whereas in the corresponding free base Pc, a split Q band is noticed which is due to lowering of symmetry, which is found to lie in 640-690 nm region. In addition, we have also measured absorption spectra of all three compounds in non-coordinating solvents such as toluene and acetonitrile, and coordinating solvents such as THF and DMF so that the solvent coordinates at axial positions of CuTmPc and ZnTmPc that lead to further minimization of aggregation [Figure 2B and Supplementary Figures S9–S11]. Furthermore, we measured emission spectra of both TmPc and ZnTmPc by exciting at 650 nm in various solvents, namely, toluene, DCM, acetonitrile, THF, and DMF [Figure 2C and Supplementary Figure S12]. It is well known and reported in literature that copper is a paramagnetic metal and due to heavy atom effect the emission is almost quenched and will be not be observed in the spectra. The corresponding emission maxima and quantum yields are presented in Table 1. The quantum yield data presented in Table 1 evaluates the polarity of the solvents. It was observed that the quantum yields are reduced when the polarity of the solvent increased due to aggregation phenomena and it influences even in the excited state life time. The radiative decay life times of newly synthesized phthalocyanines in different solvents (toluene, DCM, THF, DMF, and acetonitrile) are demonstrated in Figure 1D and Supplementary Figure S13. The corresponding excited state (singlet) lifetime data are summarized in Table 1.
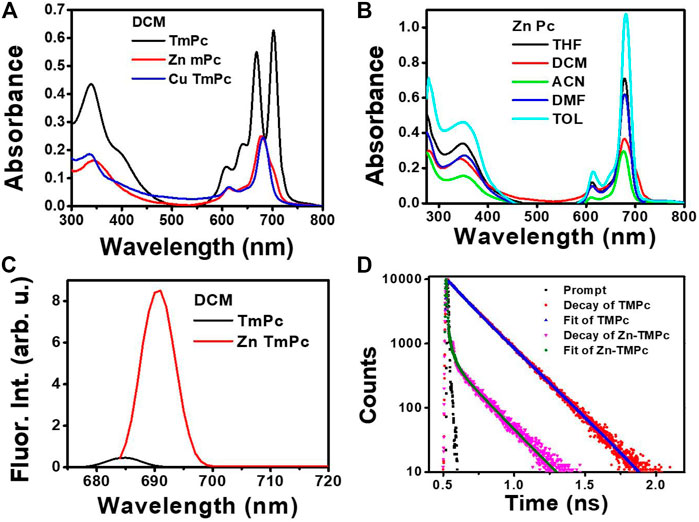
FIGURE 2. (A) Absorption spectra of TmPC, CuTmPc, and ZnTmPc in the DCM solvent (B) Absorption spectra of ZnTmPc in different solvents (C) Emission spectra of TmPc and ZnTmPc in the DCM solvent (D) Fluorescence decay curves of TmPc and ZnTmPc in the DCM solvent. “arb. u.” stands for arbitrary units.
The redox behavior of all three phthalocyanines was measured by using cyclic voltammetric technique (CV). In general, phthalocyanines tend to lose one or two electrons in its oxidation state while it gains one to four electrons in its reduction state. To appraise the redox potentials of all three phthalocyanines, we performed CV using tetrabutylammonium hexfluorophosphte (TBAPF6) in DCM as supporting electrolyte and ferrocene as external standard. Supplementary Figure S13 illustrates CV of all three phthalocyanines and the corresponding redox data presented in Table 1. The redox reactions are either cathodically or anodically shifted while metallation. All three phthalocyanines exhibited two sequential oxidation potentials at 0.85 and 1.26 V vs. SCE (TmPc), 0.70 and 1.27 V vs. SCE (CuTmPc), while ZnTmPC at 0.88 and 1.28 V vs. SCE. In addition, one quasi-reversible reduction was observed in all three macrocycles. Based on literature information, all redox behaviors are macrocyclic centered and certainly not metal centered.
To gain further insights into the structural, optical, and redox properties of these phthalocyanines, we have adopted DFT and TD-DFT techniques [with a functional basis set of the B3LYP/6-31G (d,p) level] from the Gaussian 09 package. The optimized structures of all three phthalocyanines obtained are displayed in Supplementary Figure S14. The optimized structures suggested that the phthalocyanine macrocycle is in a plane where the substituent 3,4,5-trimethoxyphenyl moiety is in tilted position and this tends to minimize the aggregation of the phthalocyanine, which is reflected in the absorption spectra [see Figure 2A].
Quantum Mechanical Calculations
Figure 3 and Supplementary Table S1 depict the HOMO, LUMO, and the HOMO-LUMO gap energies and ground state dipole moment (in Debye units), respectively. The energy levels and electron density distribution of all three phthalocyanines have shown same results estimated at HOMO: 4.75 eV and LUMO 2.65 eV with energy band gap of 2.10 eV (Figure 2). Furthermore, HOMO to HOMO-2 and LUMO+2 to LUMO energy distributions and their levels are shown in Supplementary Figures S15–S17. These data suggest that HOMOs are occupied by higher electron density on the trimethoxyphenyl ring while LUMOs are occupied by more electron clouds on the acceptor Pc macrocycle. In general, the metallation of phthalocyanine central cavity reduces the HOMO-LUMO gap. However, in the present case, the HOMO-LUMO gap was found to be marginally enhanced, possibly due to the presence of electron releasing groups at its peripheral positions (Louazri et al., 2015; Seifallah et al., 2020).
Nonlinear Optical Measurements
Promising novel organic molecules, possessing significant nonlinear optical (NLO) characteristics, are in high demand for various device applications in the fields of photonics, biomedical imaging, optical communications, etc., (Anusha et al., 2015; Venkatram et al., 2008a). All these organic molecules, like phthalocyanines (Rao et al., 2000; Maya et al., 2003; Swain et al., 2014b; Bharati et al., 2018; Venkatram et al., 2008b), porphyrins, and corroles (Anusha et al., 2012; Sarma et al., 2011) are reported frequently possessing strong third-order NLO coefficients and cross-sections (Swain et al., 2014a; Bhattacharya et al., 2019a; Clavian et al., 2019; Katturi et al., 2020a; Katturi et al., 2020b; Pati et al., 2020; Rathi et al., 2020; Kumar et al., 2021). Phthalocyanines generally have strong colors and are used as dyes. Phthalocyanines, being categorically from the aromatic organic macromolecules group, possess a huge number of delocalized electrons. The central cavity ring component in the structure of these phthalocyanines play a crucial role in their response toward laser-matter interaction. In a recent work, Cu, Zn incorporated tri-methoxy-phenoxy-phthalocyanine (TMPc, Cu-TMPc, Zn-TMPc) were synthesized in the form of macromolecules utilizing the matrix-assisted laser desorption ionization (MALDI) technique and extensive nonlinear optical measurements were carried out using the same.
To begin with, solution forms of these novel phthalocyanines were probed with MHz femtosecond pulses (at 800 nm) and the light-matter nonlinear interaction (Sutherland et al., 2003) were studied thoroughly. We have obtained the Z-scan experimental results and were able to extract various NLO parameters such as 1) two photon absorption coefficient β (cm.W−1), 2) two-photon absorption cross section σTPA (GM), 3) nonlinear refractive index n2 (cm2.W−1), and 4) nonlinear optical susceptibility index [χ(3)] by fitting the experimental data to mathematical/theoretical expressions. All the phthalocyanine molecules have exhibited purely two-photon absorbed reverse saturation absorption (RSA) nature in open aperture configuration while interacting with 80 MHz, 150 fs pulses at 800 nm. Intensity-dependent study has been carried out for all the samples, which clearly revealed an increase in two-photon absorption coefficient (β) with an increase in intensity order gradually. The RSA nature of the open aperture curves signifies the clear downfall in output transmittance, after the sample, with fine increase in intensity while approaching the focal point, clarifying the occurrence of nonlinear absorption. Whereas, in closed aperture mode, prominent peak-valley nature has been observed for all three phthalocyanines, which stands for a defocusing Kerr lens effect taking place due to high intensity dependence.
Figures 4A–C illustrate the intensity varied open aperture mode Z-scan data obtained for the phthalocyanines TMPc, Cu-TMPc, Zn-TMPc. The peak intensity has been varied from 8 MW/cm2 to 16 MW/cm2 and finally up to 24 MW/cm2, and we measured the corresponding normalized transmittance with the scanning position on and around the Rayleigh range. The estimated two–photon absorption coefficient (β) values for TMPc have been found increasing from (3.8–42) × 10−6 cm.W−1. Similarly, the β values corresponding to Cu-TMPc molecules have been found escalating from (4-11) × 10−6 cm.W−1, and the same for Zn-TMPc phthalocyanine has been observed to be in the range of (4.2–140) × 10−6 cm.W−1. Zn-TMPc has clearly shown significantly superior nonlinearity, making it a potential candidate for photonic applications. The two-photon absorption cross-sections (σ2) have also been calculated to be found in the order of ∼108 GM. From Figures 4D–F, closed aperture Z-scan data have revealed the nonlinear refractive index (n2) values as of 3.6 × 10−11, 3.5 × 10−11, 5.7 × 10−11 cm2/W for TMPc, Cu-TMPc, Zn-TMPc according to the order. Overall, the nonlinear optical susceptibility [χ(3)] has also been determined to be in the order of ∼10−10e.s.u. All the detailed estimations of the NLO parameters have been tabulated in Table 2.
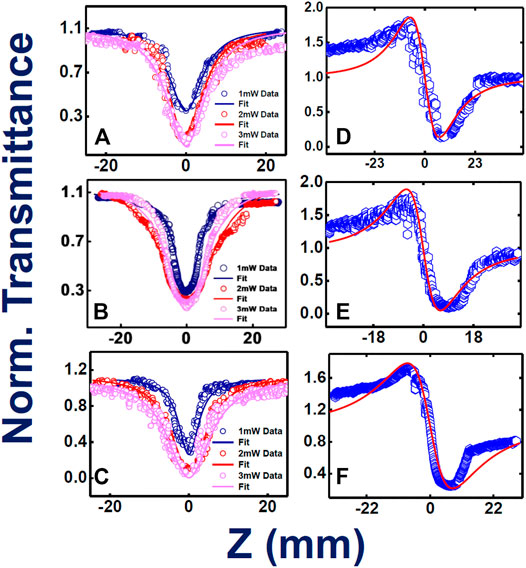
FIGURE 4. Femtosecond MHz pulses experimentally and theoretically fitted Z-scan data (intensity dependent) open aperture (A–C) and closed aperture configurations (D–F) for TMPc, Cu-TMPc, Zn-TMPc, respectively, in solution form. Open symbols are the experimental data while the solid lines represent theoretical fits.
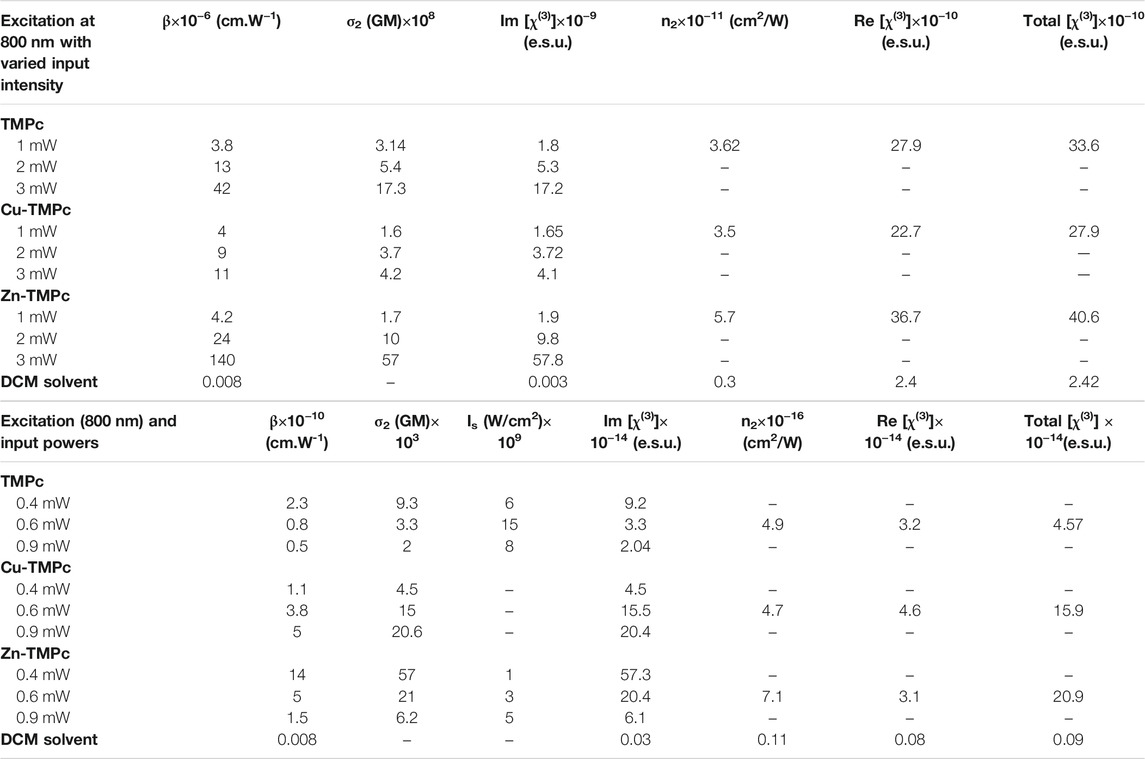
TABLE 2. Summary of the NLO coefficients of phthalocyanines studied in this work in solution form. Femtosecond MHz pulses at 800 nm were used for these investigations.
High repetition rate fs, MHz pulses generally have contribution from thermal nonlinearity, leading to higher NLO coefficients of the system extracted from Z-scan data. To access the actual/electronic nonlinearity, we have performed the same measurements with the already mentioned solutions utilizing 50 fs, 1 kHz pulses of 800 nm single beam. For, TMPc and Zn-TMPc, the study has revealed an exotic “M-shaped” open aperture Z-scan data, displaying combined nature of reverse saturation absorption (RSA) and saturation absorption (SA). For initial intensities, the samples depicted SA and for higher peak intensities it switched to RSA. Occurrence of SA shifting/switching to RSA phenomenon has been noticed in several works recently (Allu et al., 2019). With decreasing intensity, there has been a significant reduction in the nonlinear absorption coefficient, whereas a clear rise in SA nature has been pointed out. We have evaluated the saturation intensity (Is) where we observed this “M-shaped” behavior. In the case of Cu-TMPc, pure RSA nature has been observed, with decrease in transmission with increasing peak intensities. This kind of behavior was not observed with MHz fs pulses at 800 nm and this could possibly be explained based on the spectral bandwidth of both the pulses used. In the kHz case, the pulses were broad enough with a typical FWHM of >25 nm and an overall spectral width of >50 nm whereas in the case of MHz pulses, they had a FWHM of 8-10 nm with an overall spectral width of 25 nm. These molecules depicted small absorption close to 800 nm and the kHz pulses were able to access this small absorption and this resulted in saturation at initial (low) peak intensities. For higher peak intensities, the SA was overcome by the RSA as we have observed. These promising properties make these compounds potential candidates for optical switching applications. In the case of closed aperture Z-scan data, all three phthalocyanines have illustrated a focusing Kerr lens effect, revealing a downfall in the transmittance followed by a rise in it.
Figures 5A–C provide the information about the open aperture data of phthalocyanines TMPc, Cu-TMPc, Zn-TMPc, respectively, with a gradual variation in peak power/intensity. The peak intensities where the measurement has been performed were within the range of 400–600 GW/cm2. In the case of kHz pulses, peak intensities turned out to be much higher compared to MHz pulses but we were sure of probing only the electronic nonlinearity because of the repetition rate. At the lowest peak intensity, two-photon absorption coefficients (β) value for TMPc, Cu-TMPc, Zn-TMPc revealed themselves to be 2.3 × 10−10 cm.W−1, 14 × 10−10 cm.W−1, and 1.1 × 10−10 cm.W−1 and the corresponding saturation intensity (Is) turned out to be 6 × 109 W/cm2, 1 × 109 W/cm2 for sample without central element (TMPc) and “Zn” central element incorporated phthalocyanine, respectively. In the case of pure RSA nature for Cu-TMPc molecules, the β values have been found to be in the range of (1-5)×10−10 cm.W−1. In this study also, Zn-TMPc has demonstrated superior (nonlinear) two-photon absorption compared to the other two molecules. Evidently, TmPc and Zn-TmPc data conspicuously unveiled a decreasing nature in their TPA coefficient with increasing input power of the laser excitation, we believe that the reason behind this is the inherent occurrence of stronger bleaching with growing laser excitation intensity, leading to prominence in enhanced transparency, i.e., saturation absorption (SA). Whereas, in the case of Cu-TmPc, the reason behind upsurge in the TPA coefficient with intensity can possibly be attributed to the near resonant excitation circumstances (800 nm laser excitation and two-photon states above 400 nm being available as evident from the absorption spectra). It is pertinent to note that in the case of TmPc and Zn-TMPc, an additional parameter of Is was used in the fittings along with β.
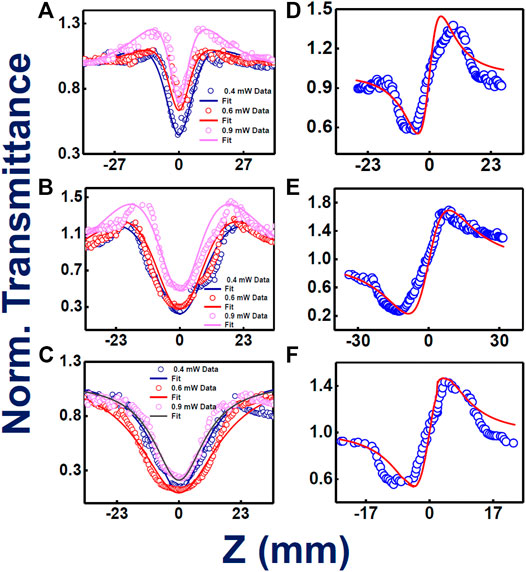
FIGURE 5. Femtosecond kHz pulses involved experimental and theoretically fitted Z-scan data (intensity dependent) open aperture (A–C) and closed aperture configurations (D–F) for TMPc, Zn-TMPc, Cu-TMPc, respectively, in solution form. Open symbols are the experimental data while the solid lines represent theoretical fits.
The NLO absorption cross sections (σ2) has been extracted and found to be ∼103 GM. Figures 5D–F exhibit the closed aperture Z-scan data, clarifying the n2 values to be 4.9 × 10−16, 7.1 × 10−16, 4.7 × 10−16 cm2/W for without any component, and Cu, Zn incorporated central cavity phthalocyanines, respectively. The total χ(3) has been calculated of the order of 10−14 e.s.u., Zn-TMPc having the highest value of 20.9 × 10−14e.s.u. Table 2 summarizes all the extracted NLO parameters in detail. The variation in the magnitude of NLO coefficients (Zn-TmPc > TmPc > Cu-TmPc) can possibly be explained in terms of the fine differences in the absorption mechanisms and the excited state lifetimes. Cu-TmPc being non-fluorescent renders the population in the excited state for a shorter time (∼few ps) whereas the other two being fluorescent molecules have lifetimes of the first excited state in the ns regime. Furthermore, TmPc has a slightly stronger absorption in the 800 nm vicinity and saturation effects are expected to be dominant at lower input intensities. In the case of Zn-TmPc, the second excited states are observed at lower energies (below 450 nm) whereas in the Cu-TmPc case they start below 400 nm. The two-photon excitation will be resonant in the case of Zn-TmPc and, therefore, expected to depict a higher NLO coefficient compared to the Cu case. Additional five-level modeling (Rao et al., 1998) along with all the details of excited state dynamics is required to completely decipher the nonlinear absorption mechanisms.
In Figures 6A–C, we present the fs, MHz open aperture data for thin films with a corresponding peak intensity of ∼15 MW/cm2. The β values obtained for the phthalocyanine films TMPc, Cu-TMPc, and Zn-TMPc, respectively, were 2.3 × 10−7 cm.W−1, 1.7 × 10−7 cm.W−1, and 3.7 × 10−7 cm.W−1. Subsequently, their corresponding TPA cross section values (σ2) have been determined as 0.9 × 107 GM, 0.7 × 107 GM, and 1.5 × 107 GM. In Figures 6D–F, closed aperture Z-scan data have been illustrated. The extracted magnitudes of n2 from the theoretical fits to experimental data turned out to be 1.85 × 10−11 cm2.W−1, 1.1 × 10−11 cm2.W−1, and 2.6 × 10−11 cm2.W−1 according to the same order of molecules. Finally, the order of magnitude of NLO susceptibilities [χ(3)] have been found to be ∼10−10e.s.u. The nonlinear absorption coefficients in thin films were an order of magnitude lower than in solution, while the n2 values were found to be similar in magnitude. This could possibly be attributed to the change in absorption spectra in thin films. Table 3 presents all the details of the measured NLO parameters.
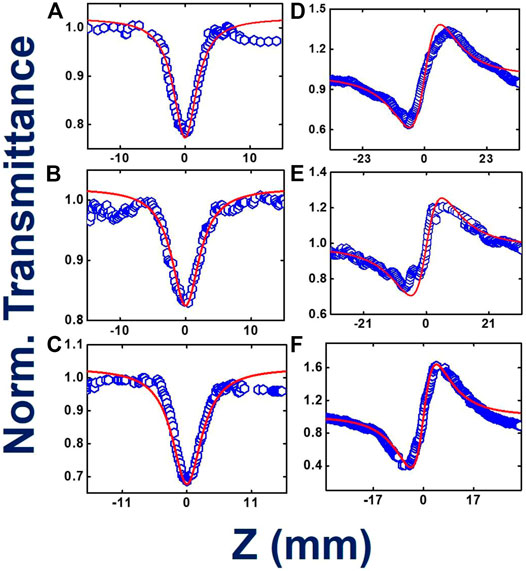
FIGURE 6. Femtosecond MHz pulses involved experimental and theoretically fitted Z-scan data in open aperture (A–C) and closed aperture configurations (D–F), for TMPc, Cu-TMPc, Zn-TMPc, respectively, in thin film form. Open symbols are the experimental data while the solid lines represent theoretical fits.
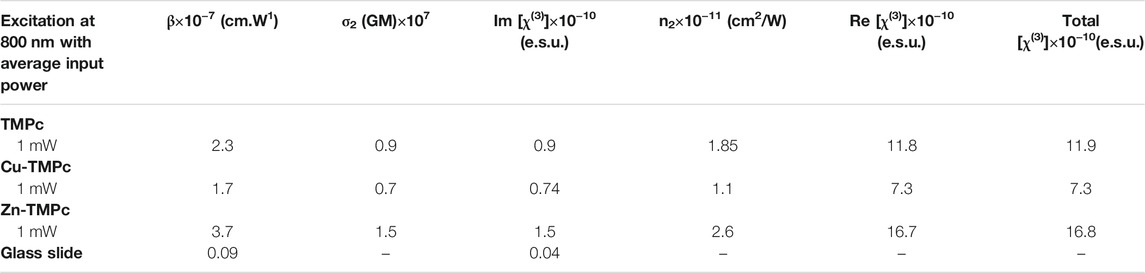
TABLE 3. Summary of the NLO coefficients of phthalocyanine thin films studied at 800 nm using 150 fs, 80 MHz pulses.
To completely understand and confirm the origin of these fine optical nonlinearities, in each case, the NLO contribution from the corresponding solvents and/or base material (bare glass slide in thin films case) have been verified sincerely by recording their Z-scan data and they have been found to possess negligible magnitude (see Supplementary Figure S20 of the SI file for the data). In both the cases, the contribution of the substrate/solvent was found to be at least 100/10 times smaller than the contribution of the solution/film and, therefore, was ignored. The detailed data have been incorporated in the SI. For better understanding, a clear comparison has been presented in tabular form in Table 4, where we have clearly pointed out that the reported novel phthalocyanine molecules in this recent work have exhibited significantly higher NLO properties, making them superior candidates for various optical applications. Throughout these detailed studies and the results obtained, it is evident that significant NLO behavior has been noticed in these molecules. As mentioned above, the vital change observed in the open aperture response from MHz to kHz studies can be attributed to the decisive differences in their spectral bandwidth. Essentially so, the fundamental difference in the optical response corresponding to nonlinear refractive index of the three compounds can be explained in terms of the Kerr lensing effect. MHz data depicted peak-valley structure or negative n2, due to high repetition rate inducing thermal nonlinearities, whereas the kHz studies depicted valley-peak structure or positive n2 in all the molecules (e.g., see Christodoulides et al., 2010 for all the mechanisms responsible for n2 in organic molecules). The reason behind the ascending order of magnitude of n2 for Cu-TMPc, TMPc, and Zn-TMPc could arise from the respective electronic distribution among the excited states or their orientation when the intense light pulse is incident. The absorption cross sections, imaginary as well as the real parts of the NLO susceptibility, are the fundamental parameters for any novel third-order NLO material developed, and these have given a promising clarity to reach out for a decisive conclusion that Zn-TMPc has turned out to be a potential compound by exhibiting significantly superior optical nonlinearity in comparison with Cu-TMPc and TMPc. Another crucial nature has got revealed corresponding to the Zn-TMPc and TMPc molecule, from their fine exotic “M curve nature”, as it stands for a tunable/shifting NLO absorption behavior depending on variable peak intensities. In regard of an unwavering structural-nonlinear property observation, usually revealed out from the complete study, “Zn” incorporation in the central cavity of tri-methoxy-phenoxy-phthalocyanine, has seemed to open up a possibility of much superior optical nonlinearity.
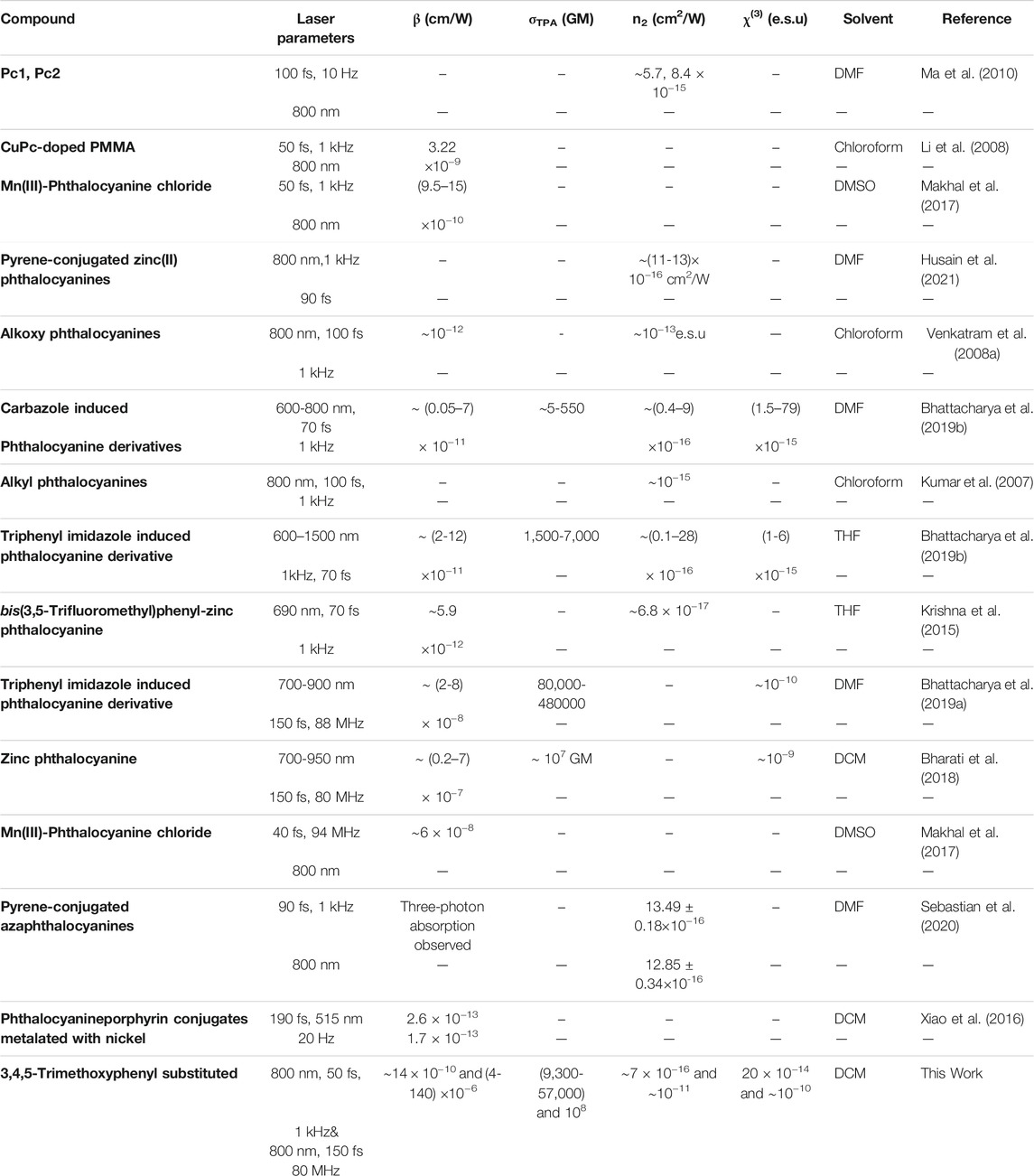
TABLE 4. Summary of various novel phthalocyanines and their derivatives reported recently and their NLO coefficients.
To put the NLO performance in perspective of the studied molecules, we compared the NLO coefficients of title compounds with some of the newly reported phthalocyanine molecules under similar experimental conditions. A similar comparison exercise has been reported by us in our recent work on porphyrins (Kumar et al., 2021b) and phthalocyanines (Bhattacharya et al., 2021). There have been several NLO reports on novel phthalocyanines but with nanosecond pulses (see, e.g., Schmidt and Calvete, 2021), and we have not considered those in this comparison study. Recently a dimeric phthalocyanine zinc complex (bis-[2-hydroxy-9(10), 16(17), 23(24)-tri-tert-butylphthalocyanine]zinc–J-[OHPctZn]2) was prepared and the NLO properties were investigated using ∼280 fs pulses (Kazak et al., 2021). They observed two-photon and three-photon absorption and reported coefficients of βeff = 312 cmGW−1 and a γeff = 8.8 cm3GW−2. Phthalocyanine nanoprobes were reported recently, and they have been demonstrated as highly desirable for photoacoustic molecular imaging (Li et al., 2021). Two-photon absorption coefficients of ∼10−13 cm/W were reported for phthalocyanine porphyrin conjugates metalated with nickel by Xiao et al., 2016. Interestingly, n2 values with magnitudes of 10−16 cm2/W were reported recently for pyrene-conjugated azaphthalocyanines (Sebastian et al., 2020) and pyrene-conjugated Zinc(II)phthalocyanines (Husain et al., 2021), respectively. However, they observed strong three-photon absorption in the open aperture data.
Our group has recently reported the NLO properties of two unsymmetrically substituted novel porphycenes, i.e., 2,7,12,13-tetraethyl-9,10,19,20-tetramethyl(OAPo-T) and 2,3,16,17-tetraethyl-9,10,19,20-tetramethyl (OAPo-C) porphycenes with TPA cross-sections of 159 and 145 GM, respectively, obtained with exact experimental conditions (kHz, fs pulses at 800 nm) used in the present study. Similarly, one of our earlier works (Rathi et al., 2020) reported fs, MHz NLO data of novel porphyrins [H2TPP(TPA)2NO2, H2TPP(TPA)2CHO, ZnTPP(TPA)2NO2, and ZnTPP(TPA)2CHO]. The obtained β values were ∼10−8 cm/W, σTPA values of ∼105 GM, n2 values of ∼10−13 cm2/W, and χ(3) values of ∼10−11e.s.u. for those molecules. The coefficients retrieved in our present case (see Table 2) are at least one order higher compared to these and this could possibly be attributed to the bulky substituents at the periphery and/or large π electrons. Our group has again reported fs, kHz NLO data (Bhattacharya et al., 2021) of ImCuPc and ImZnPc (similar central metal ions in the present case) wherein we obtained β values of ∼10−12 cm/W, n2 values of ∼10−15 cm2/W (in the present case they were ∼10−10 cm/W and ∼10−16 cm2/W). Another work reported (Kumar et al., 2021a) the fs, kHz NLO data with β values of series of “push–pull” meso-substituted trans-A2BC porphyrins, where A = mesityl, B = phenothiazine(push) and C = o/p-nitrophenyl moiety (pull) and M = 2H, Ni(II), Cu(II), and Zn(II) with coefficients of β∼10−11 cm/W, n2 ∼10−15 cm2/W. Katturi et al. (2020b) reported fs, kHz NLO studies of D-π-D porphyrins with coefficients of β ∼10−11 cm/W, n2 ∼10−15 cm2/W. The same group (Katturi et al., 2020a) again reported the fs, kHz NLO coefficients of β ∼10−11 cm/W, n2 ∼10−15 cm2/W newly synthesized donor-acceptor-based free-base [(C6F5)3] and phosphorus [P-(OH)2(C6F5)3] corroles. These data clearly indicate that the present molecules have strong NLO coefficients and potential for photonic applications (e.g., in optical imaging, optical limiting, and optical switching).
Conclusion
As concluding remarks, we state that we have synthesized a new series of non-aqueous phthalocyanines possessing the 3,4,5-trimethoxyphenyl group at peripheral positions. A thorough NLO investigation with femtosecond MHz and kHz pulses has been carried out, successfully probing into the molecules’ NLO coefficients to figure out their ultrafast interaction response nature. Significant achievements have been obtained in the NLO domain with both MHz and kHz pulse excitations. Some of the highlights are as follows:
• Strong TPA coefficients and cross-sections (kHz NLO coefficients were found to be smaller in magnitude than the MHz coefficients and this is as expected). These coefficients were found to be on par or superior to some of the recently reported other phthalocyanine moieties.
• A complicated nonlinear absorption behavior observed with kHz pulse excitation. This has been explained using the absorption profile and spectral bandwidths of the pulses used.
• Thin film NLO coefficients were also evaluated because for practical applications, these are imperative.
After extensive analysis, promising revelations have been clarified about optical nonlinearity being in deterministic relation molecular structural as well as central cavity components of the corresponding phthalocyanine molecules. This may definitely be an advantage in synthesizing novel phthalocyanine molecules according to desirable and tunable optical nonlinearity.
Data Availability Statement
The original contributions presented in the study are included in the article/Supplementary Material, and further inquiries can be directed to the corresponding author.
Author Contributions
KS, RR, and LG designed, synthesized, and characterized the molecules. DB and JR performed the NLO experiments and worked on the complete data analysis. LG and VS conceived the idea, supervised the whole research, critically reviewed/edited, and completed the manuscript for submission.
Conflict of Interest
The authors declare that the research was conducted in the absence of any commercial or financial relationships that could be construed as a potential conflict of interest.
Publisher’s Note
All claims expressed in this article are solely those of the authors and do not necessarily represent those of their affiliated organizations, or those of the publisher, the editors and the reviewers. Any product that may be evaluated in this article, or claim that may be made by its manufacturer, is not guaranteed or endorsed by the publisher.
Acknowledgments
We thank the financial support of DRDO, India, through the project# ERIP/ER/1501138/M/01/319/D(R&D) dated 27.02.2017. LG acknowledges the financial support of CSIR (through MLP-0051 and SRA scheme). KS thanks DST for the Inspire fellowship. DB acknowledges Syed Hamad for his guidance in the fitting of certain Z-scan data. We thank the Director, CSIR-IICT, for his support and encouragement.
Supplementary Material
The Supplementary Material for this article can be found online at: https://www.frontiersin.org/articles/10.3389/fchem.2021.713939/full#supplementary-material
References
Abid, S., Ben Hassine, S., Richy, N., Camerel, F., Jamoussi, B., Blanchard-Desce, M., et al. (2020). Phthalocyanine-Cored Fluorophores with Fluorene-Containing Peripheral Two-Photon Antennae as Photosensitizers for Singlet Oxygen Generation. Molecules 25, 239–269. doi:10.3390/molecules25020239
Allu, R., Banerjee, D., Avasarala, R., Hamad, S., Rao, S. V., and Podagatlapalli, G. K. (2019). Broadband Femtosecond Nonlinear Optical Properties of Silver Nanowire Films. Opt. Mater. 96, 109305. doi:10.1016/j.optmat.2019.109305
Anusha, P. T., Swain, D., Hamad, S., Giribabu, L., Prashant, T. S., Tewari, S. P., et al. (2012). Ultrafast Excited-State Dynamics and Dispersion Studies of Third-Order Optical Nonlinearities in Novel Corroles. J. Phys. Chem. C 116 (33), 17828–17837. doi:10.1021/jp305497b
Anusha, P. T., Thomas, A. R., Philip, R., and Rao, S. V. (2015). Nonlinear Absorption and Excited State Dynamics of Porphyrin and Phthalocyanine in the Presence of Explosive Molecules. Chem. Phys. Lett. 641, 23–28. doi:10.1016/j.cplett.2015.10.049
Becke, A. D. (1993). Density‐functional Thermochemistry. III. The Role of Exact Exchange. J. Chem. Phys. 98, 5648–5652. doi:10.1063/1.464913
Bharati, M. S. S., Bhattacharya, S., Suman Krishna, J. V., Giribabu, L., and Venugopal Rao, S. (2018). Femtosecond, Broadband Nonlinear Optical Studies of a Zinc Porphyrin and Zinc Phthalocyanine. Opt. Laser Technol. 108, 418–425. doi:10.1016/j.optlastec.2018.07.008
Bhattacharya, S., Biswas, C., Raavi, S. S. K., Venkata Suman Krishna, J., Koteshwar, D., Giribabu, L., et al. (2019a). Optoelectronic, Femtosecond Nonlinear Optical Properties and Excited State Dynamics of a Triphenyl Imidazole Induced Phthalocyanine Derivative. RSC Adv. 9 (63), 36726–36741. doi:10.1039/c9ra07758h
Bhattacharya, S., Biswas, C., Raavi, S. S. K., Venkata Suman Krishna, J., Vamsi Krishna, N., Giribabu, L., et al. (2019b). Synthesis, Optical, Electrochemical, DFT Studies, NLO Properties, and Ultrafast Excited State Dynamics of Carbazole-Induced Phthalocyanine Derivatives. J. Phys. Chem. C 123 (17), 11118–11133. doi:10.1021/acs.jpcc.9b01531
Bhattacharya, S., Reddy, G., Paul, S., Hossain, S. S., Kumar Raavi, S. S., Giribabu, L., et al. (2021). Comparative Photophysical and Femtosecond Third-Order Nonlinear Optical Properties of Novel Imidazole Substituted Metal Phthalocyanines. Dyes Pigm. 184, 108791. doi:10.1016/j.dyepig.2020.108791
Biswas, C., Katturi, N. K., Duvva, N., Giribabu, L., Soma, V. R., and Raavi, S. S. K. (2020). Multistep Electron Injection Dynamics and Optical Nonlinearity Investigations of π-Extended Thioalkyl-Substituted Tetrathiafulvalene Sensitizers. J. Phys. Chem. C 124, 24039–24051. doi:10.1021/acs.jpcc.0c06010
Bureš, F., Čermáková, H., Kulhánek, J., Ludwig, M., Kuznik, W., Kityk, I. V., et al. (2012). Structure-Property Relationships and Nonlinear Optical Effects in Donor-Substituted Dicyanopyrazine-Derived Push-Pull Chromophores with Enlarged and Varied π-Linkers. Eur. J. Org. Chem. 2012, 529–538. doi:10.1002/ejoc.201101226
Christodoulides, D. N., Khoo, I. C., Salamo, G. J., Stegeman, G. I., and Van Stryland, E. W. (2010). Nonlinear Refraction and Absorption: Mechanisms and Magnitudes. Adv. Opt. Photon. 2, 60–200. doi:10.1364/AOP.2.000060
Clavian, L. M., Rajesh Kumar, P. C., Anil Kumar, K. V., Rao, D. N., Shihab, N. K., and Ganesh, S. (2019). Enhanced Third Order Optical Nonlinearity in Ultrathin Amorphous Film of Tetraphenyl-Porphyrin in Picosecond Regime. Opt. Laser Technol. 119, 105642. doi:10.1016/j.optlastec.2019.105642
Cossi, M., Barone, V., Cammi, R., and Tomasi, J. (1996). Ab Initio study of Solvated Molecules: a New Implementation of the Polarizable Continuum Model. Chem. Phys. Lett. 255, 327–335. doi:10.1016/0009-2614(96)00349-1
de la Torre, G., Vázquez, P., Agulló-López, F., and Torres, T. (2004). Role of Structural Factors in the Nonlinear Optical Properties of Phthalocyanines and Related Compounds. Chem. Rev. 104, 3723–3750. doi:10.1021/cr030206t
Dennington, R., Keith, T., and Millam, J. (2009). GaussView, Version 5. Shawnee Mission KS: Semichem Inc.
Dini, D., Calvete, M. J. F., and Hanack, M. (2016). Nonlinear Optical Materials for the Smart Filtering of Optical Radiation. Chem. Rev. 116, 13043–13233. doi:10.1021/acs.chemrev.6b00033
Frisch, M. J., Trucks, G. W., Schlegel, H. B., Scuseria, G. E., Robb, M., Cheeseman, A. J. R., et al. (2009). Gaussian 09. Pittsburgh, PA: Gaussian, Inc.
Giribabu, L., Vijay Kumar, C., Raghavender, M., Somaiah, K., Yella Reddy, P., and Venkateswara Rao, P. (2008). Durable Unsymmetrical Zinc Phthalocyanine for Near IR Sensitization of Nanocrystalline TiO2 Films with Non-Volatile Redox Electrolytes. J. Nano Res. 2, 39–48. doi:10.4028/www.scientific.net/jnanor.2.39
Gounden, D., Nombona, N., and van Zyl, W. E. (2020). Recent Advances in Phthalocyanines for Chemical Sensor, Non-linear Optics (NLO) and Energy Storage Applications. Coord. Chem. Rev. 420, 213359. doi:10.1016/j.ccr.2020.213359
Gregory, P. (2000). Industrial Applications of Phthalocyanines. J. Porphyrins Phthalocyanines 4, 432–437. doi:10.1002/(sici)1099-1409(200006/07)4:4<432::aid-jpp254>3.3.co;2-e
Halaskova, M., Rahali, A., Almeida-Marrero, V., Machacek, M., Kucera, R., Jamoussi, B., et al. (2021). Peripherally Crowded Cationic Phthalocyanines as Efficient Photosensitizers for Photodynamic Therapy. ACS Med. Chem. Lett. 12, 502–507. doi:10.1021/acsmedchemlett.1c00045
Husain, A., Ganesan, A., Sebastian, M., and Makhseed, S. (2021). Large Ultrafast Nonlinear Optical Response and Excellent Optical Limiting Behaviour in Pyrene-Conjugated Zinc(II) Phthalocyanines at a Near-Infrared Wavelength. Dyes Pigm. 184, 108787. doi:10.1016/j.dyepig.2020.108787
Işık Büyükekşi, S., Karatay, A., Orman, E. B., Açar Selçuki, N., Özkaya, A. R., Salih, B., et al. (2020). A Novel AB3-type Trimeric Zinc(ii)-Phthalocyanine as an Electrochromic and Optical Limiting Material. Dalton Trans. 49, 14068–14080. doi:10.1039/D0DT02460K
Katturi, N. K., Balahoju, S. A., Ramya, A. R., Biswas, C., Raavi, S. S. K., Giribabu, L., et al. (2020a). Ultrafast Photophysical and Nonlinear Optical Properties of Novel Free Base and Axially Substituted Phosphorus (V) Corroles. J. Mol. Liquids 311, 113308. doi:10.1016/j.molliq.2020.113308
Katturi, N. K., Reddy, G., Biswas, C., Kumar Raavi, S. S., Giribabu, L., and Soma, V. R. (2020b). Ultrafast Nonlinear Optical Properties and Excited-State Dynamics of Soret-Band Excited D-π-D Porphyrins. Opt. Mater. 107, 110041. doi:10.1016/j.optmat.2020.110041
Kazak, A. V., Marchenkova, M. A., Khorkov, K. S., Kochuev, D. A., Rogachev, A. V., Kholodkov, I. V., et al. (2021). Ultrathin Langmuir-Schaefer Films of Slipped-Cofacial J-type Phthalocyanine Dimer: Supramolecular Organization, UV/Vis/NIR Study and Nonlinear Absorbance of Femtosecond Laser Radiation. Appl. Surf. Sci. 545, 148993. doi:10.1016/j.apsusc.2021.148993
Krishna, N. V., Singh, V. K., Swain, D., Rao, S. V., and Giribabu, L. (2015). Optical, Electrochemical, Third Order Nonlinear Optical, and Excited State Dynamics Studies of Bis(3,5-Trifluoromethyl)phenyl-Zinc Phthalocyanine. RSC Adv. 5 (27), 20810–20817. doi:10.1039/c4ra14390f
Kumar, A., Vashistha, V., and Kumar Das, D. (2021a). Recent Development on Metal Phthalocyanines Based Materials for Energy Conversion and Storage Applications. Coord. Chem. Rev. 431, 213678. doi:10.1016/j.ccr.2020.213678
Kumar, R. S. S., Rao, S. V., Giribabu, L., and Rao, D. N. (2007). Femtosecond and Nanosecond Nonlinear Optical Properties of Alkyl Phthalocyanines Studied Using Z-Scan Technique. Chem. Phys. Lett. 447 (4-6), 274–278. doi:10.1016/j.cplett.2007.09.028
Kumar, S., Acharyya, J. N., Banerjee, D., Soma, V. R., Vijaya Prakash, G., and Sankar, M. (2021b). Strong Two-Photon Absorption and Ultrafast Dynamics of Meso-Functionalized “Push-Pull” Trans-a2bc Porphyrins. Dalton Trans. 50, 6256–6272. doi:10.1039/D1DT00378J
Kuzmina, E. A., Dubinina, T. V., and Tomilova, L. G. (2019). Recent Advances in Chemistry of Phthalocyanines Bearing Electron-Withdrawing Halogen, nitro and N-Substituted Imide Functional Groups and Prospects for Their Practical Application. New J. Chem. 43, 9314–9327. doi:10.1039/C9NJ01755K
Lawrence, D. S., and Whitten, D. G. (1996). Photochemistry and Photophysical Properties of Novel, Unsymmetrically Substituted Metallophthalocyanines. Photochem. Photobiol. 64, 923–935. doi:10.1111/j.1751-1097.1996.tb01857.x
Li, F., Lu, P., Long, H., Yang, G., Li, Y., and Zheng, Q. (2008). Nonlinear Absorption in CuPc-Doped PMMA Thin Film in the Femtosecond Regime: Experimental and Theoretical Studies. Opt. Express 16 (19), 14571–14581. doi:10.1364/OE.16.014571
Li, Y., Shi, Y., Zhang, Z., and Xing, D. (2021). Nonlinearly Enhanced Photoacoustic Microscopy by Picosecond-Laser-Pumped Excited State Absorption of Phthalocyanine Nanoprobes. Appl. Phys. Lett. 118, 193701. doi:10.1063/5.0050767
Louazri, L., Amine, A., Bouzzine, S. M., Hamidi, M., and Bouachrine, M. (2015). Study of the Effect of Substitution on Phtalocyanine Based Compounds for Photovoltaic Application. Int. J. Chem. Mater. Res. 3, 65–78. doi:10.18488/journal.64/2015.3.3/64.3.65.78
Lu, G., Zhang, P., Fang, Y., Gao, Y., and Hu, Q. (2021). Synthesis, Characterization and Third Order Nonlinear Optical Properties of Trans-a2b-type Cobalt Corroles. New J. Chem. 45, 2103–2109. doi:10.1039/D0NJ05436D
Ma, L., Zhang, Y., and Yuan, P. (2010). Nonlinear Optical Properties of Phenoxy-Phthalocyanines at 800nm with Femtosecond Pulse Excitation. Opt. Express 18, 17666–17671. doi:10.1364/oe.18.017666
Makhal, K., Mathur, P., Maurya, S., and Goswami, D. (2017). Extracting Third Order Optical Nonlinearities of Mn(III)-phthalocyanine Chloride Using High Repetition Rate Femtosecond Pulses. J. Appl. Phys. 121 (5), 053103. doi:10.1063/1.4974966
Marder, S. R. (2006). Organic Nonlinear Optical Materials: where We Have Been and where We Are Going. Chem. Commun. 131, 131–134. doi:10.1039/b512646k
Maya, E. M., Snow, A. W., Shirk, J. S., Pong, R. G. S., Flom, S. R., and Roberts, G. L. (2003). Synthesis, Aggregation Behavior and Nonlinear Absorption Properties of lead Phthalocyanines Substituted with Siloxane Chains. J. Mater. Chem. 13 (7), 1603–1613. doi:10.1039/b301566a
Miertus, S., Scrocco, E., and Tomasi, J. (1981). Electrostatic Interaction of a Solute with a Continuum. A Direct Utilization of Ab Initio Molecular Potentials for the Prevision of Solvent Effects. J. Chem. Phys. 55, 117–129. doi:10.1016/03010104(81)85090-2
Mrinalini, M., Naresh, M., Prasanthkumar, S., and Giribabu, L. (2021). Porphyrin-based Supramolecular Assemblies and Their Applications in NLO and PDT. J. Porphyrins Phthalocyanines 25, 382–395. doi:10.1142/S1088424621500243
Ngoy, B. P., May, A. K., Mack, J., and Nyokong, T. (2019). Optical Limiting and Femtosecond Pump-Probe Transient Absorbance Properties of a 3,5-distyrylBODIPY Dye. Front. Chem. 7, 740. doi:10.3389/fchem.2019.00740
O'boyle, N. M., Tenderholt, A. L., and Langner, K. M. (2008). Cclib: a Library for Package-independent Computational Chemistry Algorithms. J. Comput. Chem. 29, 839–845. doi:10.1002/jcc.20823
Pati, N. N., Sahoo, S., Sahoo, S. S., Banerjee, D., Rao, S. V., and Panda, P. K. (2020). Chromatographically Separable Ruffled Non-planar Isomeric Octaalkylporphycenes: Consequences of Unsymmetrical Substitution upon Structure and Photophysical Properties. New J. Chem. 44 (23), 9616–9620. doi:10.1039/d0nj01744b
Petersson, G. A., and Al‐Laham, M. A. (1991). A Complete Basis Set Model Chemistry. II. Open‐shell Systems and the Total Energies of the First‐row Atoms. J. Chem. Phys. 94, 6081–6090. doi:10.1063/1.460447
Rao, S. V., Rao, D. N., Akkara, J. A., DeCristofano, B. S., and Rao, D. V. G. L. N. (1998). Dispersion Studies of Non-linear Absorption in C60 Using Z-Scan. Chem. Phys. Lett. 297, 491–498. doi:10.1016/S0009-2614(98)01166-X
Rao, S. V., Srinivas, N. K. M. N., Rao, D. N., Giribabu, L., Maiya, B. G., Philip, R., et al. (2000). Studies of Third-Order Optical Nonlinearity and Nonlinear Absorption in Tetra Tolyl Porphyrins Using Degenerate Four Wave Mixing and Z-Scan. Opt. Commun. 182 (1-3), 255–264. doi:10.1016/S0030-4018(00)00808-7
Rathi, P., Ekta, R., Kumar, S., Banerjee, D., Soma, V. R., and Sankar, M. (2020). Unsymmetrical β-functionalized 'push-Pull' Porphyrins: Synthesis and Photophysical, Electrochemical and Nonlinear Optical Properties. Dalton Trans. 49, 3198–3208. doi:10.1039/C9DT04252K
Sarma, T., Panda, P. K., Anusha, P. T., and Rao, S. V. (2011). Dinaphthoporphycenes: Synthesis and Nonlinear Optical Studies. Org. Lett. 13 (2), 188–191. doi:10.1021/ol102378g
Schmidt, A. M., and Calvete, M. J. F. (2021). Phthalocyanines: An Old Dog Can Still Have New (Photo)Tricks! Molecules 26, 2823. doi:10.3390/molecules26092823
Sebastian, M., Ganesan, A., Behbehani, H., Husain, A., and Makhseed, S. (2020). Ultrafast Nonlinear Optical Characteristics of Pyrene-Conjugated Azaphthalocyanines with Optical Limiting Behavior. J. Phys. Chem. C 124, 21740–21750. doi:10.1021/acs.jpcc.0c06565
Senge, M. O., Fazekas, M., Notaras, E. G. A., Blau, W. J., Zawadzka, M., Locos, O. B., et al. (2007). Nonlinear Optical Properties of Porphyrins. Adv. Mater. 19, 2737–2774. doi:10.1002/adma.200601850
Sheik-Bahae, M., Said, A. A., Wei, T.-H., Hagan, D. J., and Van Stryland, E. W. (1990). Sensitive Measurement of Optical Nonlinearities Using a Single Beam. IEEE J. Quan. Electron. 26, 760–769. doi:10.1109/3.53394
Singh, V. K., Kanaparthi, R. K., and Giribabu, L. (2014). Emerging Molecular Design Strategies of Unsymmetrical Phthalocyanines for Dye-Sensitized Solar Cell Applications. RSC Adv. 4, 6970–6984. doi:10.1039/c3ra45170d
Sofiani, Z., Khannyra, S., Boucetta, A., Eljouad, M., Bouchouit, K., Serrar, H., et al. (2016). Nonlinear Optical Properties of New Synthesized Conjugated Organic Molecules Based on Pyrimidine and Oxazepine. Opt. Quant Electron. 48, 282. doi:10.1007/s11082-016-0549-3
Sutherland, R. L., Mclean, D. G., and Kirkpatrick, S. (2003). Handbook of Non-linear Optics. New York, NY: CRC Press. doi:10.1201/9780203912539
Swain, D., Anusha, P. T., Shuvan Prashant, T., Tewari, S. P., Sarma, T., Panda, P. K., et al. (2012). Ultrafast Excited State Dynamics and Dispersion Studies of Nonlinear Optical Properties in Dinaphthoporphycenes. Appl. Phys. Lett. 100, 141109. doi:10.1063/1.3701274
Swain, D., Rana, A., Panda, P. K., and Venugopal Rao, S. (2014a). Strong Two-Photon Absorption Properties and Ultrafast Pump-Probe Studies of Novel Porphyrin Derivatives. Chem. Phys. Lett. 610-611, 310–315. doi:10.1016/j.cplett.2014.07.013
Swain, D., Singh, R., Singh, V. K., Krishna, N. V., Giribabu, L., Rao, S. V., et al. (2014b). Sterically Demanding Zinc(ii) Phthalocyanines: Synthesis, Optical, Electrochemical, Nonlinear Optical, Excited State Dynamics Studies. J. Mater. Chem. C 2, 1711–1722. doi:10.1039/C3TC31640H
Tuncel, S., Banimuslem, H. A. J., Durmuş, M., Gürek, A. G., Ahsen, V., Basova, T. V., et al. (2012). Liquid Crystalline Octasubstituted lead(ii) Phthalocyanines: Effects of Alkoxy and Alkylthio Substituents on Film Alignment and Electrical Properties. New J. Chem. 36, 1665–1672. doi:10.1039/C2NJ40247E
Venkatram, N., Narayana Rao, D., Giribabu, L., and Venugopal Rao, S. (2008a). Femtosecond Nonlinear Optical Properties of Alkoxy Phthalocyanines at 800nm Studied Using Z-Scan Technique. Chem. Phys. Lett. 464 (4-6), 211–215. doi:10.1016/j.cplett.2008.09.029
Venkatram, N., Narayana Rao, D., Giribabu, L., and Venugopal Rao, S. (2008b). Nonlinear Optical and Optical Limiting Studies of Alkoxy Phthalocyanines in Solutions Studied at 532 Nm with Nanosecond Pulse Excitation. Appl. Phys. B 91 (1), 149–156. doi:10.1007/s00340-008-2934-5
Vijisha, M. V., Ramesh, J., Arunkumar, C., and Chandrasekharan, K. (2020). Impressive Nonlinear Optical Responses of a Cationic Porphyrin Derivative in a Flexible All-Polymer Bragg Stack on Optical Tamm Mode Coupling. J. Mater. Chem. C 8, 12689–12697. doi:10.1039/d0tc01874k
Xiao, Z., Li, Z., Wu, X., Fang, Y., Ao, G., Huang, J., et al. (2016). Ultrafast Excited-State Dynamics of Ni-Contained Covalently Bonded Phthalocyanine-Porphyrin Conjugates. Dyes Pigm. 127, 197–203. doi:10.1016/j.dyepig.2015.10.026
Zagal, J. H., Griveau, S., Silva, J. F., Nyokong, T., and Bedioui, F. (2010). Metallophthalocyanine-based Molecular Materials as Catalysts for Electrochemical Reactions. Coord. Chem. Rev. 254, 2755–2791. doi:10.1016/j.ccr.2010.05.001
Keywords: phthalocyanine, Z-scan, two-photo absorption, femtosecond, optical switching
Citation: Srivishnu KS, Banerjee D, Ramnagar RA, Rathod J, Giribabu L and Soma VR (2021) Optical, Electrochemical, Third-Order Nonlinear Optical Investigations of 3,4,5-Trimethoxy Phenyl Substituted Non-Aqueous Phthalocyanines. Front. Chem. 9:713939. doi: 10.3389/fchem.2021.713939
Received: 28 May 2021; Accepted: 29 July 2021;
Published: 09 September 2021.
Edited by:
Moyuan Cao, Tianjin University, ChinaReviewed by:
Sabari Girisun T. C, Bharathidasan University, IndiaPengwei Wang, Hong Kong Polytechnic University, China
Copyright © 2021 Srivishnu, Banerjee, Ramnagar, Rathod, Giribabu and Soma. This is an open-access article distributed under the terms of the Creative Commons Attribution License (CC BY). The use, distribution or reproduction in other forums is permitted, provided the original author(s) and the copyright owner(s) are credited and that the original publication in this journal is cited, in accordance with accepted academic practice. No use, distribution or reproduction is permitted which does not comply with these terms.
*Correspondence: Venugopal Rao Soma, c3Zyc3BAdW9oeWQuZXJuZXQuaW4=, c29tYV92ZW51QHVvaHlkLmFjLmlu