- 1Department of Chemistry, Tianjin Key Laboratory of Molecular Optoelectronic Science, Tianjin University, Tianjin, China
- 2College of Physics and Optoelectronic Engineering, Shenzhen University, Shenzhen, China
Conjugated microporous polymers (CMPs) with robust architectures, facilely tunable pore sizes and large specific surface areas have emerged as an important class of porous materials due to their demonstrated prospects in various fields, e.g. gas storage/separation and heterogeneous catalysis. Herein, two new pyrrole-based CMPs with large specific surface areas and good stabilities were successfully prepared by one-step oxidative self-polycondensation of 1,2,4,5-tetra (pyrrol-2-ly)benzene or 1,3,5-tri (pyrrol-2-ly)benzene, respectively. Interestingly, both CMPs showed very high catalytic activity toward Knoevenagel condensation reaction, which was attributed to the inherent pore channels, high specific surface areas and abundant nitrogen sites within CMPs. Additionally, both CMPs displayed excellent recyclability with negligible degradation after 10 cycles. This work provides new possibilities into designing novel nitrogen-rich high-performance heterogeneous catalysts.
Introduction
Porous materials play significantly important roles in many fields of science and technology and have resurged with great popularity within last two decades. This, in part, is due to the emerging several kinds of unprecedented architectures with intriguing properties, e.g. metal-organic frameworks (Jiao et al., 2019), covalent organic frameworks (Cote et al., 2005) and conjugated microporous polymers (CMPs) (Cooper, 2009). CMPs are conjugated 2-dimensional or 3-dimensional polymers in contrast to many other porous materials and therefore are rigid and shape-persistent. Different from COFs, CMPs (Yue et al., 2020; Xu et al., 2021) are synthesized under kinetic control and are generally amorphous; thereby CMPs are more stable and obtained easier than COFs due to the much more kinds of reactions available for construction of CMPs, such as Sonogashira-Hagihara coupling reaction (Jiang et al., 2007) and Buchwald-Hartwig coupling reaction (Liao et al., 2018). Besides extended conjugation and high flexibility in structural design, CMPs also bear the merits of permanent porosity and tunable pore sizes. These characteristics of CMPs confer them with diverse potential applications (Lee and Cooper, 2020). For example, their π-conjugation has endowed CMPs with abundant electronic properties which have been employed to develop photocatalysts (Zhao et al., 2018) and light harvesting materials (Chen et al., 2010a). In addition, CMPs also exhibit promising prospects in heterogeneous catalysis (Chen et al., 2010a; Jiang et al., 2011), gas adsorption (Dawson et al., 2011; Lu et al., 2012), light emission (Xu et al., 2011), chemical sensors (Liu et al., 2012), energy storage (Kou et al., 2011; Xu et al., 2014; Yue et al., 2020), and biosensing (Gu et al., 2014; Ding and Han, 2015; Tan et al., 2015; Rengaraj et al., 2016).
Catalyst is an indispensable part of organic synthesis. Unfortunately, thus far, many catalysts used in industry are still non recyclable. For a sustainable future, developing reusable heterogeneous catalysts is regarded as an environmentally benign approach due to their easy separation and cleaning processes after reactions (Sartori et al., 2004). In this regard, porous materials, e.g. MOFs (Huang et al., 2020), COFs (Zhao et al., 2020) and CMPs (Tantisriyanurak et al., 2020; Xu et al., 2021) have been demonstrated as promising platforms to develop recyclable heterogeneous catalysts in part due to their large specific surface areas which could accommodate abundant guest molecules and afford many nanoreactors. In particular, researchers have been actively studying CMP-based heterogeneous catalysts over the last decade due to their insolubility in common organic solvents, high stability, inherent porosity and tailor-made functionality through facile structural design. For example, CMPs could serve as the nanoporous scaffolds for metals support to mediate catalysis (Schmidt et al., 2009; Chan-Thaw et al., 2010; Hasell et al., 2010; Gu et al., 2014). In addition, CMPs could also function as catalysts for various chemical transformations, e.g. CO2 reduction reaction (Hou et al., 2020), water splitting for hydrogen production (Zhao et al., 2018), erobic oxidations (Jiang et al., 2020), a-alkylation of aldehydes (Luo et al., 2015), Knoevenagel condensation (Feng et al., 2017) and singlet oxygen generation (Zhang et al., 2013). However, the cost-effective CMP based heterogeneous catalysts with excellent catalytic performances is still very rare. Thus, the development of CMP based heterogeneous catalysts is highly desired and continuously attracting growing research interests.
Pyrrole is a widely used monomer for constructing various functional materials. For example, polypyrrole represents as one of the state-of-the-art conductive polymer (Vernitskaya and Efimov, 1997), and three-dimensional polypyrroles were developed due to their enhanced performances in supercapacitors, sensors, etc. compared with linear polypyrroles. Porphyrin as a 4-fold pyrrole analog serves as a versatile monomer to construct all kinds of architectures like porphyrin-based belts (Minotto et al., 2021), polymers (Day et al., 2015), MOFs (Zhang et al., 2015), COFs (Hao et al., 2019) and CMPs (Chen et al., 2010b) for diverse applications. On account of the many functions and broad prospects of pyrrole-based materials, it is interesting to develop new kind of pyrrole-based architectures and explore their properties and applications. In this respect, even though several porphyrin-based CMPs have been reported (Chen et al., 2010b; Modak et al., 2013; Liu et al., 2014; Xu et al., 2019; Zhu et al., 2020), to the best of our knowledge, pyrrole-based CMPs are very rare (Lee and Cooper, 2020).
Herein, we designed and synthesized two new pyrrole-based CMPs (TrPB-CMP and TePB-CMP) through a simple FeCl3-oxidized self-condensation of multitopic pyrrole monomers (Scheme 1). We further characterized the structures and explored the properties of both CMPs with different techniques and then evaluated their catalytic performances toward Knoevenagel condensation reaction. Remarkably, both CMPs exhibit excellent catalytic activity and show superior recyclability.
Experimental
Synthesis of Pyrrole-Based Monomers and CMPs
The corresponding pyrrole-based monomers, i.e. 1,3,5-tri (pyrrol-2-ly) benzene and 1,2,4,5-tetra (pyrrol-2-ly) benzene (Xue et al., 2019) (Scheme 1) were readily prepared by one-step Suzuki coupling reaction between 1-(tert-butoxycarbonyl)-pyrrole-2-boronic acid and 1,3,5-tribromobenzene or 1,2,4,5-tetra-bromobenzene respectively (supporting information). Subsequently, both CMPs were synthesized by oxidative self-polymerization within chloroform at room temperature (Supplementary, ESI).
Results and Discussion
Structural Characterizations
The structures of both CMPs were characterized by Fourier transform infrared (FT-IR) and solid-state 13C cross-polarization magic angle spinning nuclear magnetic resonance (CP-MAS NMR) spectroscopies. As for FT-IR spectra of both pyrrole-based CMPs (Supplementary Figures S5, S6, ESI), the bands between 3450 and 3200 cm−1 correspond to the stretching vibrations of amino moieties (-NH-) originated from pyrroles (Soliman et al., 2007; Mohamed et al., 2008; Karabacak and Cinar, 2012). In addition, the bands at 1250 cm−1 are attributed to the -C-N- stretching vibrations (Zhang et al., 2004; Cai et al., 2011). The peaks at 1408 cm−1 for both CMPs are assignable to the stretching vibrations of -C=C- in the aromatic rings (Svatos and Attygalle, 1997; Samran et al., 2004). 13CP-MAS NMR spectra display broad signals between 100 and 140 ppm, which are attributed to the carbon signals from pyrrole and benzene rings (Supplementary Figures S13, S14, ESI) and the positions of these peaks are also in accordance with those of the monomers.
Properties of CMPs
The crystallinities of these polymers were determined by powder X-ray diffraction (PXRD) measurements (Supplementary Figure S10, ESI). Both materials show merely a broad diffraction band between 15° and 35°, which suggests both TrPB-CMP and TePB-CMP are amorphous in nature.
To gauge the thermal stabilities of the CMPs, thermal gravimetric analysis (TGA) under nitrogen atmosphere were carried out for both materials. The curves indicate that the weights remain 97% for TrPB-CMP at 221°C and TePB-CMP at 234°C respectively (Supplementary Figure S11, ESI), further increasing the temperature renders rapid weight losses with 69% of the initial weights at 800°C, which corresponds to the degradation of the materials. To probe the photophysical properties of the CMPs, solid state diffuse reflectance UV-vis spectra of the TrPB-CMP and TePB-CMP were measured (Supplementary Figure S12, ESI). Both TrPB-CMP and TePB-CMP exhibited broad absorption band centered at 572 and 526 nm, respectively, which is assignable to the π-π* transitions of pyrrole-based conjugated networks within CMPs. Remarkably, the absorption edges of both CMPs extend to the short-wavelength infrared region (up to 2000 nm). Moreover, the morphologies of both microporous polymers were investigated by field-emission scanning electron microscopy (FE-SEM) and transmission electron microscopy (TEM). SEM images reveal TrPB-CMP consists of submicrometer-sized spheres while TePB-CMP is composed of submicrometer-sized flakes (Figure 1). In addition, TEM images show that the pore does not produce a specific texture which verify the amorphous nature of both CMPs.
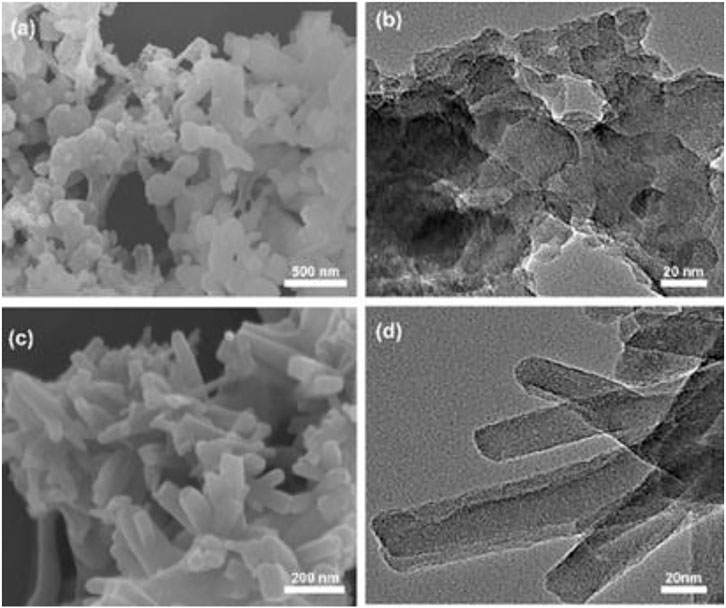
FIGURE 1. (A) SEM image of TrPB-CMP, (B) TEM image of TrPB-CMP, (C) SEM image of TePB-CMP and (D) TEM image of TePB-CMP.
The porosities of CMPs were evaluated by nitrogen (N2) sorption measurements. As shown in Figure 2, the nitrogen adsorption rate is extremely fast in the low relative pressure range, which indicates CMPs possess micropores. The hysteresis loop appeared in the middle pressure range of N2 adsorption curves indicates the existence of mesopores in CMPs (Thommes et al., 2015). The Brunauer-Emmett-Teller (BET) specific surface areas of TrPB-CMP and TePB-CMP were calculated as 810 and 800 m2g−1 respectively. The pore size distributions (PSDs) of CMPs were computed based on the adsorption branch by nonlocal density functional theory (NLDFT) method, which showed the average pore sizes of TrPB-CMP and TePB-CMP were around 1.53 and 0.80 nm respectively. Interestingly, the pore size of TrPB-CMP obtained by theoretically modeling one hexagonal segment (Supplementary Figure S18, ESI) was around 1.51 nm, which was in good consistence with the experimental result. While the pore size of TePB-CMP obtained by theoretically modeling one hexagonal segment (Supplementary Figure S19, ESI) was around 0.7 nm, which was also close to the experimental result.
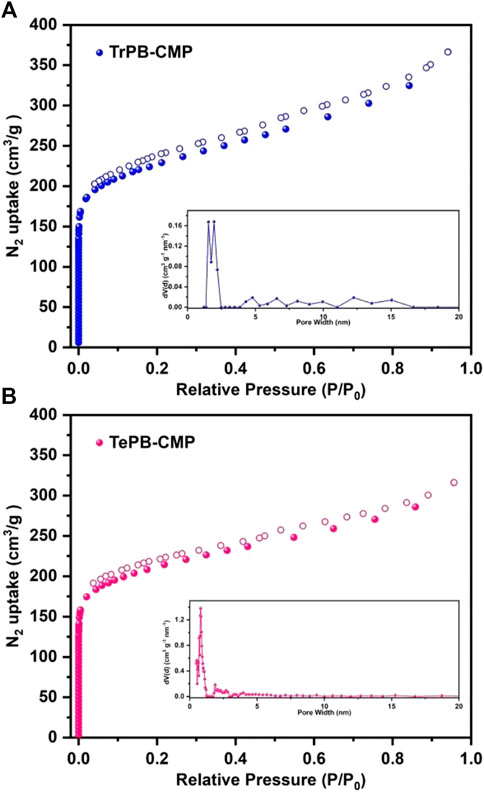
FIGURE 2. Nitrogen adsorption and desorption isotherms of TrPB-CMP (A) and TePB-CMP (B) and simulated pore size distributions of CMPs (insets) at 77 K.
Catalytic Performances Toward Knoevenagel Condensation
Considering the presence of weakly basic pyrrole moieties within both CMPs, the CMPs might be used as heterogeneous catalysts for base-catalyzed reactions which are extremely important in catalyzing the synthesis of various small molecules for chemical and pharmaceutical industries (Perryman et al., 2013; Volchkov and Lee, 2013; Denmark et al., 2014). In this respect, base-catalyzed Knoevenagel condensation was selected as the model reaction to evaluate the catalytic activity of both CMPs. Knoevenagel condensation as a well-known and powerful reaction to formulate -C=C- bonds, exhibits broad applications in producing natural products, fine chemicals and pharmaceuticals (Knoevenagel and Dtsch, 1898; Khare et al., 2019). Recently, some representative exploratory researches on Knoevenagel condensation with porous materials as the catalysts were reported including benzimidazole-based porous organic polymers (Wang et al., 2015), 3D imine-linked COF (Fang et al., 2014), and porphyrin-based porous polymer (Modak et al., 2013).
Various reaction substrates were used to test the catalytic activities of the CMP catalysts under classical reaction conditions (Wang et al., 2015; Taher et al., 2020). In addition, the reaction temperature, solvent and reaction temperature were investigated in details to find the best conditions for the reaction (Supplementary Table S2, ESI). The yields of the substrates in the Knoevenagel condensation reaction were summarized in Table 1. As displayed in Table 1, remarkably, the reactions were completed after 1 h and the yields for all substrates under the catalysis of CMPs were quite high, which was much higher than that without addition of CMPs (44%) (Supplementary Table S3, entry 1, ESI). As for benzaldehydes with strong electron-withdrawing substituents in the para-position, the catalytic efficiency of both TrPB-CMP and TePB-CMP are basically the same with nearly quantitative conversions (entries 4, 5). The catalytic effects of both CMPs proved to be obviously different when the electron-withdrawing strength of the para-substituent on benzaldehyde was weakened (entries 2 and 3). It suggests TrPB-CMP renders higher conversions than TePB-CMP for the benzaldehyde substrates, which is probably due to more adequate interactions between the substrates and the basic sites within the pores of TrPB-CMP than those of TePB-CMP rendered by the bigger pore size of TrPB-CMP (entries 1 and 2). Moreover, for larger size molecules, there is a significant difference in catalytic efficiency, probably because the steric hindrance of the larger substrate molecules is not conducive to entering the micropores (entry 7). When using benzaldehyde substrates with electron-donating substituents, the catalytic yields of both TrPB-CMP and TePB-CMP were lower (entries 8 and 9). In addition, compared with the results reported in the previous literatures, the reaction conditions of the current work have advantages over others, e.g., metal-free catalysis and shorter reaction time (Supplementary Table S4, ESI).
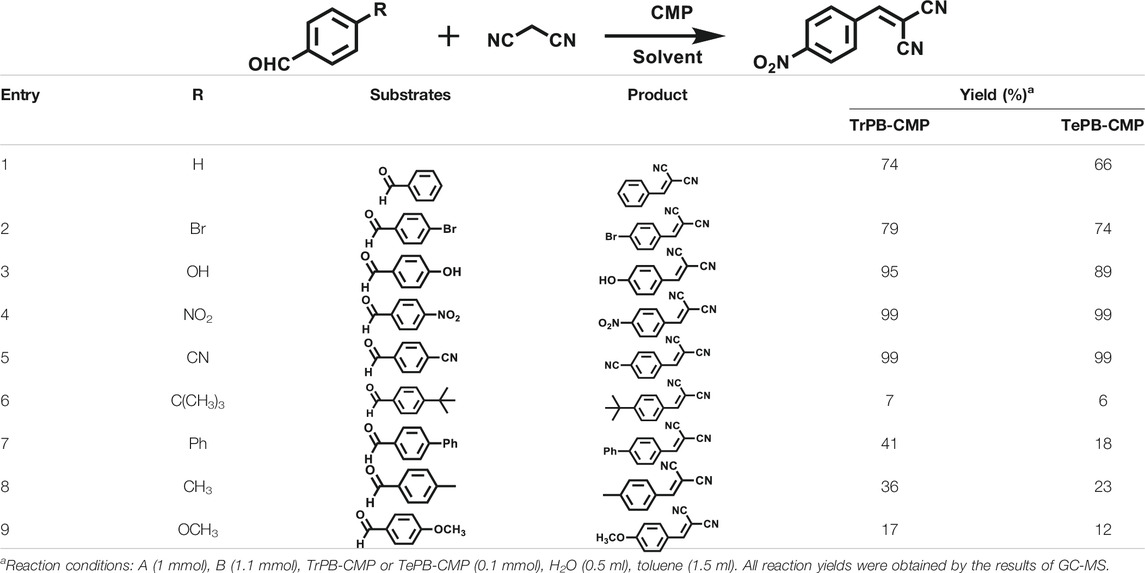
TABLE 1. Catalytic activities of TrPB-CMP or TePB-CMP toward Knoevenagel condensation with different aromatic aldehyde substrates.
To illustrate the high catalytic performance and gain further insights into the catalytic mechanism, additional comparison experiments were performed to evaluate the catalytic activity of pyrrole-based small molecules, i.e. TrPB and TePB (Scheme 1) and linear polypyrrole toward Knoevenagel condensation under the same conditions as those of CMPs. As shown in Supplementary Table S3, the catalytic activity of TrPB, TePB and polypyrrole was not obvious, which was similar to without any catalyst. Consequently, compared with non-porous analogues, the open porous structure allows the reactants to easily enter the catalytic center. In addition, the larger the specific surface area of the pore, the better the catalytic performance. Due to the high specific surface area and microporous character, benzimidazole-based CMPs (BPOP-1 and BPOP-2) was favorable for the accessibility of substrates to catalytic active sites inside the framework (Wang et al., 2015), which make the heteroatoms on the pore wall fully exhibit catalytic activity. Moreover, Similar pore restriction effects also appeared in other catalytic reactions (Mackintosh et al., 2008; Hu et al., 2020; Yang et al., 2020).
As for testing the rates of the reactions, p-nitrobenzaldehyde was used as the substrate which catalyzed by both TrPB-CMP and TePB-CMP (Supplementary Figures S15, S16, ESI). The results suggested the substrates are quickly converted into the products within 30 min for both CMP-catalyzed reactions and reached the maximum conversion within 1 h. The conversion rate of TrPB-CMP is faster than that of TePB-CMP, which was probably benefited from the bigger pore size and specific surface area of TrPB-CMP. As to the recyclability of both CMP catalysts, as shown in Figure 3, the catalytic activities of both CMPs are basically unchanged within 10 cycles. After 10 cycles, FT-IR spectra of both recycled CMPs appeared the same as those of the pristine CMPs, which suggests the structures of both CMPs are robust and intact (Supplementary Figures S7, S8, ESI). In addition, after 10 cycles, the N2 adsorption tests indicated the BET specific surface areas of TrPB-CMP and TePB-CMP were 800 and 781 m2g−1, respectively, both of which were only slightly decreased compared with those of the pristine CMPs (Supplementary Figures S17, ESI). Consequently, it reveals both CMPs serve as efficient heterogeneous catalysts with excellent recyclability.
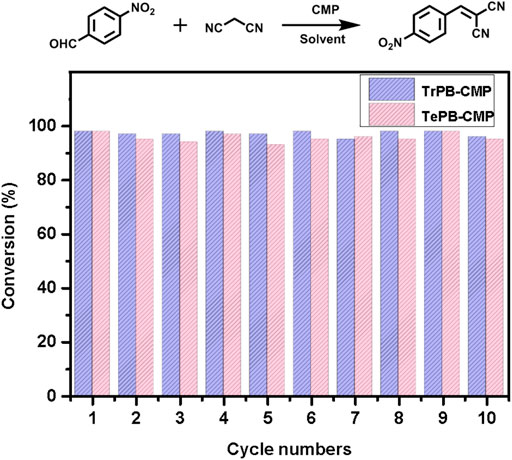
FIGURE 3. Recyclability of TrPB-CMP (blue) and TePB-CMP (red) as catalysts with p-nitrobenzaldehyde as the substrate.
Conclusion
In summary, two new pyrrole-based conjugated microporous polymers were successfully synthesized by self-polymerization of 1,3,5-tri-(pyrrol-2-ly)benzene or 1,2,4,5-tetra (pyrrol-2-ly)benzene. These two CMPs effectively catalyzed Knoevenagel condensation reaction with diverse substrates and showed excellent recycling performance, which was attributed to the open pore channels, large specific surface area and abundant heteroatoms as active sites within CMPs. This work suggests a new approach to fabricate pyrrole-based heterogenous catalysts. Additionally, both CMPs exhibit broad absorptions between 250 and 2400 nm, which might promise application potentials in photocatalysis.
Data Availability Statement
The original contributions presented in the study are included in the article/Supplementary Material, further inquiries can be directed to the corresponding authors.
Author Contributions
GZ and YL designed the work. RG carried out the experimental part. RG, GZ, FL, and YL organized and wrote the manuscript.
Funding
This work was financially supported by National Natural Science Foundation of China (21602154).
Conflict of Interest
The authors declare that the research was conducted in the absence of any commercial or financial relationships that could be construed as a potential conflict of interest.
Supplementary Material
The Supplementary Material for this article can be found online at: https://www.frontiersin.org/articles/10.3389/fchem.2021.687183/full#supplementary-material
References
Cai, Y.-m., Qin, Z.-y., and Chen, L. (2011). Effect of Electrolytes on Electrochemical Properties of Graphene Sheet Covered with Polypyrrole Thin Layer. Prog. Nat. Sci. Mater. Int. 21 (6), 460–466. doi:10.1016/S1002-0071(12)60083-5
Chan-Thaw, C. E., Villa, A., Katekomol, P., Su, D., Thomas, A., and Prati, L. (2010). Covalent Triazine Framework as Catalytic Support for Liquid Phase Reaction. Nano Lett. 10 (2), 537–541. doi:10.1021/nl904082k
Chen, L., Honsho, Y., Seki, S., and Jiang, D. (2010a). Light-harvesting Conjugated Microporous Polymers: Rapid and Highly Efficient Flow of Light Energy with a Porous Polyphenylene Framework as Antenna. J. Am. Chem. Soc. 132 (19), 6742–6748. doi:10.1021/ja100327h
Chen, L., Yang, Y., and Jiang, D. (2010b). CMPs as Scaffolds for Constructing Porous Catalytic Frameworks: a Built-In Heterogeneous Catalyst with High Activity and Selectivity Based on Nanoporous Metalloporphyrin Polymers. J. Am. Chem. Soc. 132 (26), 9138–9143. doi:10.1021/ja1028556
Cooper, A. I. (2009). Conjugated Microporous Polymers. Adv. Mater. 21 (12), 1291–1295. doi:10.1002/adma.200801971
Cote, A. P., Benin, A. I., Ockwig, N. W., Keeffe, M., Matzger, A. J., and Yaghi, O. M. (2005). Porous, Crystalline, Covalent Organic Frameworks. Science 310 (5751), 1166–1170. doi:10.1126/science.1120411
Dawson, R., Adams, D. J., and Cooper, A. I. (2011). Chemical Tuning of CO2 Sorption in Robust Nanoporous Organic Polymers. Chem. Sci. 2 (6), 1173–1177. doi:10.1039/C1SC00100K
Day, N. U., Wamser, C. C., and Walter, M. G. (2015). Porphyrin Polymers and Organic Frameworks. Polym. Int. 64 (7), 833–857. doi:10.1002/pi.4908
Denmark, S. E., Wilson, T. W., and Burk, M. T. (2014). Enantioselective Construction of Quaternary Stereogenic Carbon Atoms by the Lewis Base Catalyzed Additions of Silyl Ketene Imines to Aldehydes. Chem. Eur. J. 20 (30), 9268–9279. doi:10.1002/chem.201403342
Ding, X., and Han, B.-H. (2015). Metallophthalocyanine-based Conjugated Microporous Polymers as Highly Efficient Photosensitizers for Singlet Oxygen Generation. Angew. Chem. Int. Ed. 54 (22), 6536–6539. doi:10.1002/anie.201501732
Fang, Q., Gu, S., Zheng, J., Zhuang, Z., Qiu, S., and Yan, Y. (2014). 3D Microporous Base-Functionalized Covalent Organic Frameworks for Size-Selective Catalysis. Angew. Chem. Int. Ed. 53 (11), 2878–2882. doi:10.1002/anie.201310500
Feng, L.-J., Wang, M., Sun, Z.-Y., Hu, Y., and Deng, Z.-T. (2017). Hypercrosslinked Porous Polyporphyrin by Metal-free Protocol: Characterization, Uptake Performance, and Heterogeneous Catalysis. Designed Monomers Polym. 20, 344–350. doi:10.1080/15685551.2016.1259831
Gu, C., Huang, N., Gao, J., Xu, F., Xu, Y., and Jiang, D. (2014). Controlled Synthesis of Conjugated Microporous Polymer Films: Versatile Platforms for Highly Sensitive and Label-free Chemo- and Biosensing. Angew. Chem. Int. Ed. 53 (19), 4850–4855. doi:10.1002/anie.201402141
Hao, W., Chen, D., Li, Y., Yang, Z., Xing, G., Li, J., et al. (2019). Facile Synthesis of Porphyrin Based Covalent Organic Frameworks via an A2B2 Monomer for Highly Efficient Heterogeneous Catalysis. Chem. Mater. 31 (19), 8100–8105. doi:10.1021/acs.chemmater.9b02718
Hasell, T., Wood, C. D., Clowes, R., Jones, J. T. A., Khimyak, Y. Z., Adams, D. J., et al. (2010). Palladium Nanoparticle Incorporation in Conjugated Microporous Polymers by Supercritical Fluid Processing. Chem. Mater. 22 (2), 557–564. doi:10.1021/cm9030446
Hou, Y., Zhang, E., Gao, J., Zhang, S., Liu, P., Wang, J.-C., et al. (2020). Metal-free Azo-Bridged Porphyrin Porous Organic Polymers for Visible-Light-Driven CO2 Reduction to CO with High Selectivity. Dalton Trans. 49 (22), 7592–7597. doi:10.1039/D0DT01436B
Hu, X., Sun, X., Song, Q., Zhu, Y., Long, Y., and Dong, Z. (2020). N,S Co-doped Hierarchically Porous Carbon Materials for Efficient Metal-free Catalysis. Green. Chem. 22 (3), 742–752. doi:10.1039/c9gc03863a
Huang, S., Chen, G., Ye, N., Kou, X., Zhang, R., Shen, J., et al. (2020). Iron-mineralization-induced Mesoporous Metal-Organic Frameworks Enable High-Efficiency Synergistic Catalysis of Natural/nanomimic Enzymes. ACS Appl. Mater. Inter. 12 (51), 57343–57351. doi:10.1021/acsami.0c16689
Jiang, J.-X., Su, F., Trewin, A., Wood, C. D., Campbell, N. L., Niu, H., et al. (2007). Conjugated Microporous Poly(aryleneethynylene) Networks. Angew. Chem. Int. Ed. 46 (45), 8574–8578. doi:10.1002/anie.200701595
Jiang, J.-X., Wang, C., Laybourn, A., Hasell, T., Clowes, R., Khimyak, Y. Z., et al. (2011). Metal-organic Conjugated Microporous Polymers. Angew. Chem. Int. Ed. 50 (5), 1072–1075. doi:10.1002/anie.201005864
Jiang, J., Liang, Z., Xiong, X., Zhou, X., and Ji, H. (2020). A Carbazolyl Porphyrin‐Based Conjugated Microporous Polymer for Metal‐Free Photocatalytic Aerobic Oxidation Reactions. ChemCatChem 12 (13), 3523–3529. doi:10.1002/cctc.202000199
Jiao, L., and Jiang, H. L. (2019). Metal-Organic-Framework-Based Single-Atom Catalysts for Energy Applications. Chem. 5(4), 786–804. doi:10.1016/j.chempr.2018.12.011
Karabacak, M., and Cinar, M. (2012). FT-IR, FT-Raman, UV Spectra and DFT Calculations on Monomeric and Dimeric Structure of 2-Amino-5-Bromobenzoic Acid. Spectrochimica Acta A: Mol. Biomol. Spectrosc. 86, 590–599. doi:10.1016/j.saa.2011.11.022
Khare, R., Pandey, J., Smriti, S., and Ruchi, R. (2019). The Importance and Applications of Knoevenagel Reaction (Brief Review). Orient. J. Chem. 35 (1), 423–429. doi:10.13005/ojc/350154
Knoevenagel, E., and Dtsch, Ber. (1898). Condensationen zwischen malonester und aldehyden unter dem einfluss von ammoniak und organischen aminen. Ber. Dtsch. Chem. Ges. 31, 2585–2595. doi:10.1002/cber.18980310307
Kou, Y., Xu, Y., Guo, Z., and Jiang, D. (2011). Supercapacitive Energy Storage and Electric Power Supply Using an Aza-Fused π-Conjugated Microporous Framework. Angew. Chem. Int. Ed. 50 (37), 8753–8757. doi:10.1002/anie.201103493
Lee, J.-S. M., and Cooper, A. I. (2020). Advances in Conjugated Microporous Polymers. Chem. Rev. 120 (4), 2171–2214. doi:10.1021/acs.chemrev.9b00399
Liao, Y., Wang, H., Zhu, M., and Thomas, A. (2018). Efficient Supercapacitor Energy Storage Using Conjugated Microporous Polymer Networks Synthesized from Buchwald-Hartwig Coupling. Adv. Mater. 30 (12), 1705710. doi:10.1002/adma.201705710
Liu, X., A, S., Zhang, Y., Luo, X., Xia, H., Li, H., et al. (2014). A Porphyrin-Linked Conjugated Microporous Polymer with Selective Carbon Dioxide Adsorption and Heterogeneous Organocatalytic Performances. RSC Adv. 4 (13), 6447–6453. doi:10.1039/c3ra46988c
Liu, X., Xu, Y., and Jiang, D. (2012). Conjugated Microporous Polymers as Molecular Sensing Devices: Microporous Architecture Enables Rapid Response and Enhances Sensitivity in Fluorescence-On and Fluorescence-Off Sensing. J. Am. Chem. Soc. 134 (21), 8738–8741. doi:10.1021/ja303448r
Lu, W., Sculley, J. P., Yuan, D., Krishna, R., Wei, Z., and Zhou, H.-C. (2012). Polyamine-tethered Porous Polymer Networks for Carbon Dioxide Capture from Flue Gas. Angew. Chem. Int. Ed. 51 (30), 7480–7484. doi:10.1002/anie.201202176
Luo, J., Zhang, X., and Zhang, J. (2015). Carbazolic Porous Organic Framework as an Efficient, Metal-free Visible-Light Photocatalyst for Organic Synthesis. ACS Catal. 5 (4), 2250–2254. doi:10.1021/acscatal.5b00025
Mackintosh, H. J., Budd, P. M., and Mckeown, N. B. (2008). Catalysis by Microporous Phthalocyanine and Porphyrin Network Polymers. J. Mater. Chem. 18 (5), 573–578. doi:10.1039/b715660j
Minotto, A., Bulut, I., Rapidis, A. G., Carnicella, G., Patrini, M., Lunedei, E., et al. (2021). Towards Efficient Near-Infrared Fluorescent Organic Light-Emitting Diodes. Light Sci. Appl. 10 (1), 18. doi:10.1038/s41377-020-00456-8
Modak, A., Mondal, J., and Bhaumik, A. (2013). Porphyrin Based Porous Organic Polymer as Bi-functional Catalyst for Selective Oxidation and Knoevenagel Condensation Reactions. Appl. Catal. A: Gen. 459, 41–51. doi:10.1016/j.apcata.2013.03.036
Mohamed, T. A., Soliman, U. A., Hanafy, A. I., and Hassan, A. M. (2008). Conformational Stability, Barriers to Internal Rotation of 2-aminothiophenol (D0 and D3): A Combined Vibrational and Theoretical Approach. J. Mol. Struct. THEOCHEM 865 (1-3), 14–24. doi:10.1016/j.theochem.2008.06.021
Perryman, M. S., Harris, M. E., Foster, J. L., Joshi, A., Clarkson, G. J., and Fox, D. J. (2013). Trichloromethyl Ketones: Asymmetric Transfer Hydrogenation and Subsequent Jocic-type Reactions with Amines. Chem. Commun. 49 (85), 10022–10024. doi:10.1039/C3CC46070C
Rengaraj, A., Puthiaraj, P., Haldorai, Y., Heo, N. S., Hwang, S.-K., Han, Y.-K., et al. (2016). Porous Covalent Triazine Polymer as a Potential Nanocargo for Cancer Therapy and Imaging. ACS Appl. Mater. Inter. 8 (14), 8947–8955. doi:10.1021/acsami.6b00284
Samran, J., Phinyocheep, P., Daniel, P., Derouet, D., and Buzaré, J.-Y. (2004). Raman Spectroscopic Study of Non-catalytic Hydrogenation of Unsaturated Rubbers. J. Raman Spectrosc. 35 (12), 1073–1080. doi:10.1002/jrs.1256
Sartori, G., Ballini, R., Bigi, F., Bosica, G., Maggi, R., and Righi, P. (2004). Protection (And Deprotection) of Functional Groups in Organic Synthesis by Heterogeneous Catalysis. Chem. Rev. 104 (1), 199–250. doi:10.1021/cr0200769
Schmidt, J., Weber, J., Epping, J. D., Antonietti, M., and Thomas, A. (2009). Microporous Conjugated Poly(thienylene Arylene) Networks. Adv. Mater. 21 (6), 702–705. doi:10.1002/adma.200802692
Soliman, U. A., Hassan, A. M., and Mohamed, T. A. (2007). Conformational Stability, Vibrational Assignmenents, Barriers to Internal Rotations and Ab Initio Calculations of 2-aminophenol (D0 and D3). Spectrochimica Acta Part A: Mol. Biomol. Spectrosc. 68 (3), 688–700. doi:10.1016/j.saa.2006.12.047
Svatos, A., and Attygalle, A. B. (1997). Characterization of Vinyl-Substituted, Carbon-Carbon Double Bonds by GC/FT-IR Analysis. Anal. Chem. 69 (10), 1827–1836. doi:10.1021/ac960890u
Taher, A., Lumbiny, B. J., and Lee, I.-M. (2020). A Facile Microwave-Assisted Knoevenagel Condensation of Various Aldehydes and Ketones Using Amine-Functionalized Metal Organic Frameworks. Inorg. Chem. Commun. 119, 108092. doi:10.1016/j.inoche.2020.108092
Tan, J., Wan, J., Guo, J., and Wang, C. (2015). Self-sacrificial Template-Induced Modulation of Conjugated Microporous Polymer Microcapsules and Shape-dependent Enhanced Photothermal Efficiency for Ablation of Cancer Cells. Chem. Commun. 51 (98), 17394–17397. doi:10.1039/C5CC05478H
Tantisriyanurak, S., Duguid, H. N., Peattie, L., and Dawson, R. (2020). Acid Functionalized Conjugated Microporous Polymers as a Reusable Catalyst for Biodiesel Production. ACS Appl. Polym. Mater. 2 (9), 3908–3915. doi:10.1021/acsapm.0c00595
Thommes, M., Kaneko, K., Neimark, A. V., Olivier, J. P., Rodriguez-Reinoso, F., Rouquerol, J., et al. (2015). Physisorption of Gases, with Special Reference to the Evaluation of Surface Area and Pore Size Distribution (IUPAC Technical Report). Pure Appl. Chem. 87 (9-10), 1051–1069. doi:10.1515/pac-2014-1117
Vernitskaya, T. y. V., and Efimov, O. N. (1997). Polypyrrole: a Conducting Polymer; its Synthesis, Properties and Applications. Russ. Chem. Rev. 66 (5), 443–457. doi:10.1070/rc1997v066n05abeh000261
Volchkov, I., and Lee, D. (2013). Asymmetric Total Synthesis of (−)-Amphidinolide V through Effective Combinations of Catalytic Transformations. J. Am. Chem. Soc. 135 (14), 5324–5327. doi:10.1021/ja401717b
Wang, Y., Wang, L., Liu, C., and Wang, R. (2015). Benzimidazole-containing Porous Organic Polymers as Highly Active Heterogeneous Solid-Base Catalysts. Chemcatchem 7 (10), 1559–1565. doi:10.1002/cctc.201500244
Xu, F., Chen, X., Tang, Z., Wu, D., Fu, R., and Jiang, D. (2014). Redox-active Conjugated Microporous Polymers: a New Organic Platform for Highly Efficient Energy Storage. Chem. Commun. 50 (37), 4788–4790. doi:10.1039/C4CC01002G
Xu, Y., Chen, L., Guo, Z., Nagai, A., and Jiang, D. (2011). Light-emitting Conjugated Polymers with Microporous Network Architecture: Interweaving Scaffold Promotes Electronic Conjugation, Facilitates Exciton Migration, and Improves Luminescence. J. Am. Chem. Soc. 133 (44), 17622–17625. doi:10.1021/ja208284t
Xu, Y., Cui, D., Zhang, S., Xu, G., and Su, Z. (2019). Facile Synthesis of Conjugated Microporous Polymer-Based Porphyrin Units for Adsorption of CO2 and Organic Vapors. Polym. Chem. 10 (7), 819–822. doi:10.1039/c8py01476k
Xu, Y., Sprick, R. S., Brownbill, N. J., Blanc, F., Li, Q., Ward, J. W., et al. (2021). Bottom-up Wet-Chemical Synthesis of a Two-Dimensional Porous Carbon Material with High Supercapacitance Using a Cascade Coupling/cyclization Route. J. Mater. Chem. A. 9 (6), 3303–3308. doi:10.1039/d0ta11649a
Xue, S., Kuzuhara, D., Aratani, N., and Yamada, H. (2019). Synthesis of a porphyrin(2.1.2.1) Nanobelt and its Ability to Bind Fullerene. Org. Lett. 21 (7), 2069–2072. doi:10.1021/acs.orglett.9b00329
Yang, F., Li, Y., Zhang, T., Zhao, Z., Xing, G., and Chen, L. (2020). Docking Site Modulation of Isostructural Covalent Organic Frameworks for CO 2 Fixation. Chem. Eur. J. 26 (20), 4510–4514. doi:10.1002/chem.202000552
Yue, Y., Xu, Y., Kong, F., Li, Q., and Ren, S. (2020). Bulk-synthesis and Supercapacitive Energy Storage Applications of Nanoporous Triazine-Based Graphdiyne. Carbon 167, 202–208. doi:10.1016/j.carbon.2020.06.001
Zhang, K., Kopetzki, D., Seeberger, P. H., Antonietti, M., and Vilela, F. (2013). Surface Area Control and Photocatalytic Activity of Conjugated Microporous Poly(benzothiadiazole) Networks. Angew. Chem. Int. Ed. 52 (5), 1432–1436. doi:10.1002/anie.201207163
Zhang, W., Jiang, P., Wang, Y., Zhang, J., and Zhang, P. (2015). Synthesis of Two Metal-Porphyrin Frameworks Assembled from Porphyrin Building Motifs, 5, 10, 15, 20-tetrapyridylporphyrin and Their Base Catalyzed Property. Inorg. Chem. Commun. 61, 100–104. doi:10.1016/j.inoche.2015.09.002
Zhang, X., Zhang, J., Liu, Z., and Robinson, C. (2004). Inorganic/organic Mesostructure Directed Synthesis of Wire/ribbon-like Polypyrrole nanostructuresElectronic Supplementary Information (ESI) Available: FT-IR Spectra, Powder XRD Pattern and Conductivities of As-Made PPy Nanostructures. Chem. Commun. (16), 1852–1853. doi:10.1039/B405255B
Zhao, Y., Ma, W., Xu, Y., Zhang, C., Wang, Q., Yang, T., et al. (2018). Effect of Linking Pattern of Dibenzothiophene-S,s-Dioxide-Containing Conjugated Microporous Polymers on the Photocatalytic Performance. Macromolecules 51 (23), 9502–9508. doi:10.1021/acs.macromol.8b02023
Zhao, Y., Zhao, Y., Qiu, J., Li, Z., Wang, H., and Wang, J. (2020). Facile Grafting of Imidazolium Salt in Covalent Organic Frameworks with Enhanced Catalytic Activity for CO2 Fixation and the Knoevenagel Reaction. ACS Sustain. Chem. Eng. 8 (50), 18413–18419. doi:10.1021/acssuschemeng.0c05294
Zhu, Z., Yang, Z., Fan, Y., Liu, C., Sun, H., Liang, W., et al. (2020). Calcination of Porphyrin-Based Conjugated Microporous Polymers Nanotubes as Nanoporous N-Rich Metal-free Electrocatalysts for Efficient Oxygen Reduction Reaction. ACS Appl. Energ. Mater. 3 (6), 5260–5268. doi:10.1021/acsaem.0c00079
Keywords: heterogeneous catalysis, pyrrole, knoevenagel condensation, conjugated microporous polymers, photocatalysis
Citation: Gao R, Zhang G, Lu F, Chen L and Li Y (2021) Pyrrole-Based Conjugated Microporous Polymers as Efficient Heterogeneous Catalysts for Knoevenagel Condensation. Front. Chem. 9:687183. doi: 10.3389/fchem.2021.687183
Received: 29 March 2021; Accepted: 26 April 2021;
Published: 10 May 2021.
Edited by:
Mengmeng Li, Institute of Microelectronics, ChinaReviewed by:
San-Yuan Ding, Lanzhou University, ChinaShijie Ren, Sichuan University, China
Jia-Xing Jiang, Shaanxi Normal University, China
Copyright © 2021 Gao, Zhang, Lu, Chen and Li. This is an open-access article distributed under the terms of the Creative Commons Attribution License (CC BY). The use, distribution or reproduction in other forums is permitted, provided the original author(s) and the copyright owner(s) are credited and that the original publication in this journal is cited, in accordance with accepted academic practice. No use, distribution or reproduction is permitted which does not comply with these terms.
*Correspondence: Guang Zhang, emhhbmdnQHRqdS5lZHUuY24=; Yang Li, bGl5YW5nMjAxNEBzenUuZWR1LmNu