- 1Department of Biopharmacy, School of Pharmaceutical Sciences, Jilin University, Changchun, China
- 2School of pharmaceutical science, Institute of Frontier Medical Science, Jilin University, Changchun, China
Raman spectroscopy has emerged as a promising tool in biomedical analysis and clinical diagnosis. The development of surface-enhanced Raman scattering spectroscopy (SERS) improved the detection limit with ultrahigh sensitivity and simplicity. More and more Raman spectroscopy clinical trials (R-PCT) have been conducted recently. However, there is a lack of an up-to-date review summarizing the current status of Raman clinical trials performed until now. Hence, the clinical trials for Raman were retrieved from the International Clinical Trials Registration Platform. We summarized the clinical characteristics of 55 registered Raman spectroscopy clinical trials (R-RSCTs) and 44 published Raman spectroscopy clinical trials (P-RSCTs). This review could assist researchers and clinicians to understand the current status of Raman spectroscopy clinical research and perhaps could benefit the reasonable and accurate design of future SERS studies.
Introduction
After the discovery of inelastic scattering of light by CV Raman in 1928, the phenomenon was reported in biomedical application for the first time in 1970 (Raman and Krishnan, 1928; Lord and Yu, 1970). In the progress of light scattering, most photons maintain the same energy and wavelength after interacting with matter (Raman and Krishnan, 1928; Auner et al., 2018). But a very small portion of photons is linearly inelastically scattered, resulting in loss of energy, and a longer wavelength (Raman and Krishnan, 1928; Wang et al., 2018). The shift of the wavelength is called Raman shift, which is inversely proportional to the change in the photons’ wavelength (Jones et al., 2019). The intensity of the Raman spectrum against Raman shift was expressed in wavenumbers with the units of cm−1 (Raman and Krishnan, 1928; Pilot et al., 2019). The Raman spectrum gives a directly objective picture of the molecular composition (Sahu et al., 2013).
The measurement of Raman spectroscopy is a fast, label-free, and noninvasive progress. Hence, it has many advantages in biomedical applications. The harmfulness of laser used in Raman spectroscopy can be reduced by selecting the right wavelength and power, and Raman signals provide the molecular information of tissue and cells directly (Lee et al., 2019; Chaiken and Peterson, 2021). But the natural intensity of the Raman signal was low, which resulted in low signal-to-noise ratios (Pence and Mahadevan-Jansen, 2016). In recent years, different types of Raman spectroscopy were developed to improve the sensitivity and specificity of Raman scattering, including resonance Raman spectroscopy (RRS), surface-enhanced Raman spectroscopy (SERS), and tip-enhanced Raman spectroscopy (TERS) (Bailo and Deckert, 2008; Stiles et al., 2008; Robert, 2009). The enhancement of Raman signal for RRS, SERS, and TERS was reported by a factor of 102 to 106, 105 to 1010, and 1010, respectively (Auner et al., 2018). The enhancement of Raman spectroscopy attracted attention in clinics because of its high sensitivity (Cialla-May et al., 2017).
SERS is particularly interesting because the Raman signal can be controlled by modifying a designated probe on the surface to detect the specific analytes (Stiles et al., 2008). Raman signals of molecules adsorbed on the metal surface were amplified by generating a localized surface plasmon resonance under an incident electromagnetic field (Lee and Tseng, 2018; Pilot et al., 2019). Based on the knowledge of molecular interaction in vivo, such as the antibody–antigen and complementary sequences of DNA and RNA, SERS is employed to quantify drugs and biomolecules in complex systems such as blood and tissue due to its high sensitivity and specificity (Henry et al., 2016; Xue et al., 2018). SERS was also developed to be applied in surgical margin guidance such as ovarian cancer and brain tumor (Jiang et al., 2019). SERS preferred liquid samples, for example, biofluids and cells, due to the additive metallic nanoparticles and the limited detection distance (less than tens of nanometers) between analytes and metallic surfaces (Pence and Mahadevan-Jansen, 2016). To develop SERS and Raman spectroscopy better, it is necessary to understand the current status of their clinical trials.
Raman spectroscopy has been in clinical phases since 2003 (U.S. National Library of Medicine, 2003). Until now, there has been no review to characterize the clinical status of Raman spectroscopy clinical trials. In this review, we retrieved the registered Raman spectroscopy clinical trials (R-RSCTs) in trial registries of ICTRP with standardized process requirements for the first time. We summarized basic clinical characteristics, disease classification, and sample classification in ongoing and completed clinical trials to update the current status. Published Raman spectroscopy clinical trials (P-PSCTs) were also retrieved and summarized due to the lack of updated results of the R-RSCTs. Moreover, the current SERS clinical application was summarized, and its future prospect was discussed based on the above results. This could benefit the reasonable and accurate designs of future SERS studies.
The Current Status of Raman Spectroscopy Clinical Trials: The Methods
Search Strategy
The preparation for the data of registered Raman spectroscopy clinical trials (R-RSCTs) was conducted (Figure 1): the registration database of WHO registries through the International Clinical Trials Registry Platform (ICTRP) Search Portal (http://apps.who.int/trialsearch) was used to get registration items concerning R-RSCTs by searching for the key word “Raman.” The records of non-related ones will be excluded one by one.
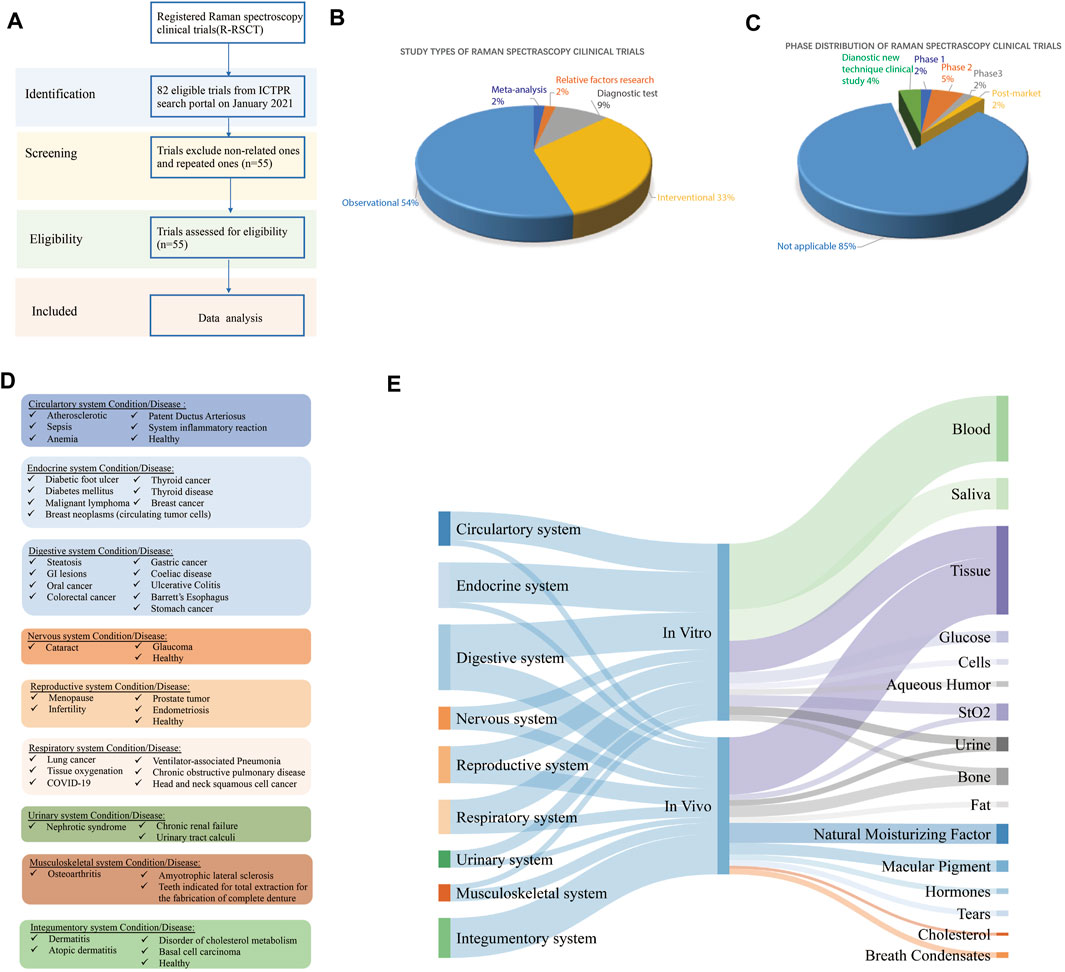
FIGURE 1. (A) Flowchart of Raman spectroscopy clinical trial selection, (B) characterization of study types of registered Raman spectroscopy clinical trials (R-RSCTs), (C) phase distribution of R-RSCTs, (D) disease classification of R-RSCTs according to physiological systems, and (E) the Sankey diagram of physiological systems, in vivo/in vitro, and sample types in R-RSCTs.
The preparation for the data of published Raman spectroscopy clinical trials (P-RSCTs) was conducted: the search term used was “Raman” in PubMed with the article type restriction as “Clinical trials.” The clinical studies were retrieved and followed PRISMA guidelines.
Data Selection and Extraction
Trials Selection and Data Extraction
The retrieved records from the database of WHO registries were exported to Microsoft Excel. The titles, abstracts, and full texts were screened by the first author (XX) to exclude the non-related Raman spectroscopy records. The following data of Raman spectroscopy clinical trials were extracted: a combination of title, abstract, and full-text screen was performed by the first author (XX) to exclude any record that was not a Raman trial or repeated ones. The following data for trial records were extracted: trial identifier, study title, date of registration, register source, trial phase, recruitment status, anticipated enrolment sample size, condition studied, study type, sample type, in vitro/vivo, and the number of evaluation factors (Fan et al., 2020). However, the information of R-RSCTs in ICTRP was not updated on time, which did not reflect the present research status of completed RSCTs (Al-Durra et al., 2020).
The retrieved articles from PubMed were thoroughly screened by the first author (XX), and the following information of published Raman spectroscopy clinical trials was extracted: the Raman type, date of publication, sample type, sample size, condition studied, in vitro/vivo, the number of evaluation factors, sensitivity, and specificity.
Discrepancies
Data extraction was performed by two authors (XX and CL). Any discrepancies were resolved between the two authors.
Data Analysis
Descriptive statistics were used to characterize the trials extracted from the ICTRP Search Portal. All statistical analyses were performed using Microsoft Excel. Missing values were excluded from the analysis unless indicated.
Data Visualization
The flowchart was drawn with Adobe Illustrator. The pie chart was drawn with Microsoft Excel 8.0 (Microsoft, Radmond, United States). Sankey diagrams were generated with SankeyMATIC for data visualization. The illustration figure was drawn with BioRender.
The Current Status of Raman Spectroscopy Clinical Trials: The Results
Characterization of Registered Raman Spectroscopy Clinical Trials
We retrieved 82 records from the ICTRP database, of which 25 records were excluded because their interventions were not Raman spectroscopy (Figure 1A). Two records were excluded after full-text screening since they were the repeated ones. A total of 55 records in the ICTRP were analyzed for basic trial characteristics (Figure 1A). In Table 1, we found that 55 trials were registered in the following seven registries: Australian New Zealand Clinical Trials Registry (ANZCTR) (1), ClinicalTrials.gov (24), Chinese Clinical Trials Registry (ChiCTR) (8), Clinical Trials Registry-India (CTRI) (4), International Standard Randomized Controlled Trial Number (ISRCTN) (2), Japan Primary Registries Network (JRPN) (11), and German Clinical Trials Register (5). For the recruitment status of these trials, 20 (36.36%) are actively recruiting subjects, and most (64%) of the remaining trials have not yet started to recruit participants. As shown in Figure 1B, the study type of 30 (54.5%) R-RSCTs was for “Observational,” followed by “Interventional” (18, 32.7%), “Diagnostic test” (5, 9.1%), “relevant factors research” (1, 1.8%), and “meta-analysis” (1, 1.8%). An “Observational study” aims to observe patients or measure certain outcomes without any specific intervention, and patients will not be assigned into different groups. An “Interventional study” aims to evaluate one or more particular interventions on participants, and participants will be created into different groups. The “Diagnostic test” is a study design to evaluate diagnostic accuracy. “Relative factor research” is a study to investigate multiple factors during disease diagnosis, prognosis, and treatment efficacy evaluations. “Meta-analysis” is a statistical process that combines the findings from individual studies.
For the phase distribution, most of the trials were under the phase “Not Applicable” in Figure 1C. The main reason may be the failure of recruitment. One trial (No. CTRI/2018/01/011139) was under the post-market phase to analyze the physicochemical interactions of the dentin–resin interface (Girija, 2018). One trial (No. NCT00060580) was under Phase 1 to measure the amount of the pigment lutein in the retina (U.S. National Library of Medicine, 2003). Three trials (No. NCT02033512, No. NCT02621853, and No. CTRI/2009/091/000851) were under Phase 2 to detect hormones, fat, and saliva, respectively (U.S. National Library of Medicine, 2014; U.S. National Library of Medicine, 2015; Vedang, 2009). One trial (No. ChiCTR1800016644) was in Phase 3 to monitor dermatitis degree in treatment of asthma by acupoint sticking therapy (Liu, 2018). Two trials (No. ChiCTR-RDC-17012611 and No. ChiCTR1800015711) were categorized to diagnostic new technique clinical study (Qiu, 2017; Qian, 2018). The sample size is mostly distributed in the middle and small sample size, and 83.6% is less than 200 subjects. Of these R-RSCTs, nearly 85% of evaluation factors were multiple. Only eight trials were evaluated by the single factor. In total, 31 trials were conducted in vitro and 24 trials in vivo. These characteristics above and others of the R-RSCTs are summarized in Table 1.
In Figure 1D, 47 diseases of R-RSCTs were classified into nine types according to the physiological systems. The digestive system (nine diseases) had the most number of diseases, followed by the endocrine system (seven diseases), circulatory system (six diseases), and respiratory system (six diseases). It should be noticed that no diseases from the immune system were chosen as the condition in R-RSCTs. In Figure 1E, the samples corresponded to in vitro/in vivo, and the physiological systems were shown as the Sankey diagram. The clinical trials conducted in vitro have nine types of samples. Blood, saliva, and tissue were taken in most of the trials.
Characterization of Published Raman Spectroscopy Clinical Trials
The majority of the above registrars still need to be completed, and the completed clinical trials did not update their results in real time. Therefore, we collected 44 results of the published Raman spectroscopy clinical trials in PubMed to obtain the current status. There are ten types of Raman spectra which have been studied in the clinic, as shown in Table 2: “Confocal Raman” (11), “Probe Raman” (10), conventional Raman spectrometer (6), “Raman microscope” (4), “Resonance Raman” (4), “Micro-Raman” (3), “Transcutaneous Raman” (2), SERS (2), “FT-Raman” (1), and “Kerr-gated Raman” (1). 22 conditions including healthy and 21 diseases are summarized in Table 2. The published articles showed that Raman spectroscopy was widely used in vivo and in vitro. “Confocal Raman,” “Resonance Raman,” and “Transcutaneous Raman” were only conducted in vivo. Transcutaneous Raman and Confocal Raman were both approved to meet the clinical accuracy requirement in the noninvasive detection of glucose in vivo. Confocal Raman, Probe Raman, and conventional Raman were applied to evaluate skin and components of skin under healthy and dermatitis conditions. Probe Raman, Raman microscope, conventional Raman, Kerr-gated Raman, and SERS were used in the diagnosis of 11 types of cancer. FT-Raman and Transcutaneous Raman could provide the fingerprints of bone in vivo and teeth in vitro. The sample size of most studies was less than 100, which accounted for 90.9% of P-RSCTs. P-RSCTs were all single-site studies, and no multicenter clinical trials were published. Multicenter studies were widely recognized to eliminate the bias in a single-site study and generate more convincible evidence by large numbers of hospitals and patients (Zheng and Zelen, 2008). Hence, the published results indicated the primary evaluation of Raman spectroscopy in the clinic.
We summarized the present situation of 55 R-RSCTs and 44 P-RSCTs in this review. Only three studies were published before 2008. From 2003 to 2017, both R-RCTS and P-RCTs gradually increased. From 2018 to 2020, R-RCTs raised up rapidly to around 10 clinical trials each year. No published results were obtained in PubMed since 2018. Collectively, the results are shown in Figure 2. We look forward to the release of these registered clinical trials in the next 5 years.
The Current Status of SERS Clinical Trials: The Results
There are six registered clinical trials (Table 3) related to SERS and two published clinical trials. The illustration of samples and collected outcomes from these trials are shown in Figure 3. The study type of five registered trials was “Observational” and one trial was “Diagnostic test.” The interventional/observational models were “Factorial,” “Cohort,” and “Case–control.” A “Factorial” study aims to evaluate two interventions in the same trial and their interactions between two interventions. A “Cohort” study aims to measure the effect of a suspected risk factor in both groups of people who have a certain condition and who have no condition. A “Case–control” study aims to determine factors associated with a certain condition. Two trials were registered in 2019 and four trials in 2020. Only two trials are actively recruiting subjects. The sample size of four trials was distributed in middle and small sizes. All the trials were under the phase “Not applicable.” The sample types were blood, urine, saliva, and tears. All the trials were conducted in vitro. According to the published results of two studies, SERS had a sensitivity of 80.7% and a specificity of 84.1% in the diagnosis of oral squamous cell carcinoma by analyzing the fingerprints of blood and sensitivity of 326-fM SERS nanoparticles in colon tumor tissue (Zavaleta et al., 2013; Tan et al., 2017). According to the trials, it indicated that SERS preferred the sample type of biofluids and depended on metal nanoparticles to magnify signals.
Discussion and Perspective of Future SERS Clinical Application
This review provides an overview of the current status of Raman spectroscopy clinical registration information and published articles. It is a new and extensive survey of R-RSCTs and P-RSCTs. Most R-RSCTs are registered on platforms from the United States, Japan, China, and Germany. Compared with preclinical studies, the ongoing R-RSCTs are very rare, and most researchers focused on developing new technology in cellular and animal models. As we mentioned above, the completed R-RSCTs in the WHO platform did not update their results in real time. Moreover, the clinical protocol of some studies in the publication was different from the registered one. It is difficult to analyze the results of R-RSCTs. Many researchers only registered a trial about Raman spectroscopy without recruiting any participants. In the summary of P-RSCTs, we found that P-RSCTs were subjected to a single-center study with a sample size of fewer than 100 subjects. Although Raman spectroscopy had many advantages, some types of Raman spectroscopy failed to continue to be developed in the clinic such as the instrument of Kerr-gated Raman, which was filled in two rooms (Prieto et al., 2005). Hence, we suggest that the researchers from universities and research centers may collaborate more with clinicians and industry sponsors to conduct large-scale, high-quality, and multicenter R-RSCTs and publish their results in the corresponding registration platforms. SERS also has the same problems above, and it is in the early stage of clinical development. Most clinicians are familiar with the technologies such as computed tomography (CT) and nuclear magnetic resonance (NMR), but unfamiliar with SERS, and other Raman spectra. This resulted in the slow development of R-RSCTs and P-RSCTs.
SERS depends on the metal surface but is also limited by the interaction surface in the clinic. Most clinical trials chose the wavelength of laser at 785 nm to excite the light scattering and collect the Raman signals, which proved efficiency and safety of the laser (Sigurdsson et al., 2004). But the penetration distance in vivo of the laser was limited to less than 10 mm (Nakagawa et al., 2010; Egawa and Sato, 2015). Hence, SERS can only be used in vitro and ex vivo to make metallic surfaces and samples close enough. There are two methods to use SERS in biomedical research. One is to add nanoparticles without SERS reporter in the samples and amplify the intrinsic Raman signals. Another one is to administrate the nanoparticles with SERS reporter directly to detect the reporter signals. Fingerprints require complex interpretation and the concern of reproducibility. The fluorescent background of samples also brings a low signal-to-noise ratio (SNR). The second method may be a better choice in clinical application due to its higher specificity and higher SNR.
Importantly, several critical issues need to be concerned with the following. First, there is the selection of reliable biomarkers in biofluids: oncology is still the “Gold standard” of diagnosis. Applying biomarkers in diagnosis calls for researchers from basic medicine, optical spectroscopy, and clinicians from hospitals to collaborate. Second, there are multicenter and large-scale clinical trials: large-scale trials should be conducted under standard protocols to prove the advantages of SERS in the clinic compared to other techniques. Third, there is the artificial intelligence of SERS spectra: although SERS signals may have several sharp peaks, it is still difficult to analyze the intensity and the shifts of wavelength directly. Diagnosis models of diseases are necessary to be built by artificial intelligence to transform SERS spectra to a readable clinical standard immediately. Three AI-related Raman spectroscopy clinical trials were registered in 2021. Overall, we look forward to breakthrough developments of SERS in the clinic in the next 5 years.
Author Contributions
XX summarized and analyzed the data. XX wrote the manuscript. CL confirmed the data and supervised the whole study.
Funding
This work was supported by the Department of Science and Technology, Department of Jilin Province (No. 20190304035YY).
Conflict of Interest
The authors declare that the research was conducted in the absence of any commercial or financial relationships that could be construed as a potential conflict of interest.
Acknowledgments
We thank Hang Su for helping complete the Sankey diagram. All authors read and approved the final manuscript.
References
Adigal, S. S., Rizvi, A., Rayaroth, N. V., John, R. V., Barik, A., Bhandari, S., et al. (2019). Human Tear Sample Studies Using High Performance Liquid Chromatography with Laser Induced Fluorescence (HPLC-LIF) and Surface Enhanced Raman Spectroscopy Techniques (SERS).
Ager, J. W., Nalla, R. K., Balooch, G., Kim, G., Pugach, M., Habelitz, S., et al. (2006). On the Increasing Fragility of Human Teeth with Age: a Deep-UV Resonance Raman Study. J. Bone Miner Res. 21 (12), 1879–1887. doi:10.1359/jbmr.060816
Al-Durra, M., Nolan, R. P., Seto, E., and Cafazzo, J. A. (2020). Prospective Registration and Reporting of Trial Number in Randomised Clinical Trials: Global Cross Sectional Study of the Adoption of ICMJE and Declaration of Helsinki Recommendations. BMJ 369, m982. doi:10.1136/bmj.m982
Auner, G. W., Koya, S. K., Huang, C., Broadbent, B., Trexler, M., Auner, Z., et al. (2018). Applications of Raman Spectroscopy in Cancer Diagnosis. Cancer Metastasis Rev. 37 (4), 691–717. doi:10.1007/s10555-018-9770-9
Bailo, E., and Deckert, V. (2008). Tip-enhanced Raman Scattering. Chem. Soc. Rev. 37 (5), 921–930. doi:10.1039/b705967c
Bergholt, M. S., Lin, K., Wang, J., Zheng, W., Xu, H., Huang, Q., et al. (2016). Simultaneous Fingerprint and High-Wavenumber Fiber-Optic Raman Spectroscopy Enhances Real-Timein Vivodiagnosis of Adenomatous Polyps during Colonoscopy. J. Biophoton 9 (4), 333–342. doi:10.1002/jbio.201400141
Blume-Peytavi, U., Rolland, A., Darvin, M. E., Constable, A., Pineau, I., Voit, C., et al. (2009). Cutaneous Lycopene and β-carotene Levels Measured by Resonance Raman Spectroscopy: High Reliability and Sensitivity to Oral Lactolycopene Deprivation and Supplementation. Eur. J. Pharmaceutics Biopharmaceutics 73 (1), 187–194. doi:10.1016/j.ejpb.2009.04.017
Botelho, M., Queiroz, D., Barros, G., Guerreiro, S., Fechine, P., Umbelino, S., et al. (2014). Nanostructured Transdermal Hormone Replacement Therapy for Relieving Menopausal Symptoms: a Confocal Raman Spectroscopy Study. Clinics 69 (2), 75–82. doi:10.6061/clinics/2014(02)01
Camerlingo, C., d'Apuzzo, F., Grassia, V., Perillo, L., and Lepore, M. (2014). Micro-Raman Spectroscopy for Monitoring Changes in Periodontal Ligaments and Gingival Crevicular Fluid. Sensors 14 (12), 22552–22563. doi:10.3390/s141222552
Chaiken, J., Finney, W., Knudson, P. E., Weinstock, R. S., Khan, M., and Bussjager, R. J. (2005). Effect of Hemoglobin Concentration Variation on the Accuracy and Precision of Glucose Analysis Using Tissue Modulated, Noninvasive, In Vivo Raman Spectroscopy of Human Blood: a Small Clinical Study. J. Biomed. Opt. 10 (3), 031111. doi:10.1117/1.1922147
Chaiken, J., and Peterson, C. M. (2021). Noninvasive Blood and Tissue Analysis: Raman Spectroscopy, One Perspective for Monitoring of Glucose and beyond. J. Diabetes Sci. Technol. 15 (1), 28–33. doi:10.1177/1932296820964803
Choe, C., Lademann, J., and Darvin, M. E. (2015). Analysis of Human and Porcine Skin In Vivo/Ex Vivo for Penetration of Selected Oils by Confocal Raman Microscopy. Skin Pharmacol. Physiol. 28 (6), 318–330. doi:10.1159/000439407
Chrit, L., Bastien, P., Sockalingum, G. D., Batisse, D., Leroy, F., Manfait, M., et al. (2006). An In Vivo Randomized Study of Human Skin Moisturization by a New Confocal Raman Fiber-Optic Microprobe: Assessment of a Glycerol-Based Hydration Cream. Skin Pharmacol. Physiol. 19 (4), 207–215. doi:10.1159/000093116
Chrit, L., Hadjur, C., Morel, S., Sockalingum, G., Lebourdon, G., Leroy, F., et al. (2005). In Vivo chemical Investigation of Human Skin Using a Confocal Raman Fiber Optic Microprobe. J. Biomed. Opt. 10 (4), 044007. doi:10.1117/1.2003747
Cialla-May, D., Zheng, X.-S., Weber, K., and Popp, J. (2017). Recent Progress in Surface-Enhanced Raman Spectroscopy for Biological and Biomedical Applications: from Cells to Clinics. Chem. Soc. Rev. 46 (13), 3945–3961. doi:10.1039/c7cs00172j
Crow, P., Stone, N., Kendall, C. A., Uff, J. S., Farmer, J. A. M., Barr, H., et al. (2003). The Use of Raman Spectroscopy to Identify and Grade Prostatic Adenocarcinoma In Vitro. Br. J. Cancer 89 (1), 106–108. doi:10.1038/sj.bjc.6601059
Dos Santos, L., Téllez S, C. A., Sousa, M. P. J., Azoia, N. G., Cavaco-Paulo, A. M., Martin, A. A., et al. (2017). In Vivo confocal Raman Spectroscopy and Molecular Dynamics Analysis of Penetration of Retinyl Acetate into Stratum Corneum. Spectrochimica Acta A: Mol. Biomol. Spectrosc. 174, 279–285. doi:10.1016/j.saa.2016.11.042
Egawa, M., and Iwaki, H. (2008). In Vivoevaluation of the Protective Capacity of Sunscreen by Monitoring Urocanic Acid Isomer in the Stratum Corneum Using Raman Spectroscopy. Skin Res. Technol. 14 (4), 410–417. doi:10.1111/j.1600-0846.2008.00318.x
Egawa, M., and Sato, Y. (2015). In Vivoevaluation of Two Forms of Urea in the Skin by Raman Spectroscopy after Application of Urea-Containing Cream. Skin Res. Technol. 21 (3), 259–264. doi:10.1111/srt.12184
Enejder, A. M., Scecina, T. G., Oh, J., Hunter, M., Shih, W. C., and Sasic, S. (2005). Raman Spectroscopy for Noninvasive Glucose Measurements. J. Biomed. Opt. 10 (3), 031114. doi:10.1117/1.1920212
Fan, S., Zhang, Z., Su, H., Xu, P., Qi, H., Zhao, D., et al. (2020). Panax Ginseng Clinical Trials: Current Status and Future Perspectives. Biomed. Pharmacother. 132, 110832. doi:10.1016/j.biopha.2020.110832
Ghana Shyam, C. (2020). Design and Development of an Optical Setup for Cell Membrane Targeted Surface Enhanced Raman Spectroscopy Using Vortex Beams. Available from: http://www.ctri.nic.in/Clinicaltrials/pmaindet2.php?trialid=45056.
Girija, S. (2018). Raman Spectroscopic Analysis of Physicochemical Interactions of Dentin –resin Interface – an Invivo Study. Study of the effect of dental filling material on human tooth. Available from: http://www.ctri.nic.in/Clinicaltrials/pmaindet2.php?trialid=18609.
González, F. J., Alda, J., Moreno-Cruz, B., Martínez-Escanamé, M., Ramírez-Elías, M. G., Torres-Álvarez, B., et al. (2011). Use of Raman Spectroscopy for the Early Detection of Filaggrin-Related Atopic Dermatitis. Skin Res. Technol. 17 (1), 45–50. doi:10.1111/j.1600-0846.2010.00461.x
Henry, A.-I., Sharma, B., Cardinal, M. F., Kurouski, D., and Van Duyne, R. P. (2016). Surface-Enhanced Raman Spectroscopy Biosensing: In Vivo Diagnostics and Multimodal Imaging. Anal. Chem. 88 (13), 6638–6647. doi:10.1021/acs.analchem.6b01597
Hesterberg, K., Lademann, J., Patzelt, A., Sterry, W., and Darvin, M. E. (2009). Raman Spectroscopic Analysis of the Increase of the Carotenoid Antioxidant Concentration in Human Skin after a 1-week Diet with Ecological Eggs. J. Biomed. Opt. 14 (2), 024039. doi:10.1117/1.3119257
Huang, Z., Teh, S. K., Zheng, W., Lin, K., Ho, K. Y., Teh, M., et al. (2010). In Vivo detection of Epithelial Neoplasia in the Stomach Using Image-Guided Raman Endoscopy. Biosens. Bioelectron. 26 (2), 383–389. doi:10.1016/j.bios.2010.07.125
Jiang, C., Wang, Y., Song, W., and Lu, L. (2019). Delineating the Tumor Margin with Intraoperative Surface-Enhanced Raman Spectroscopy. Anal. Bioanal. Chem. 411 (18), 3993–4006. doi:10.1007/s00216-019-01577-9
Jones, R. R., Hooper, D. C., Zhang, L., Wolverson, D., and Valev, V. K. (2019). Raman Techniques: Fundamentals and Frontiers. Nanoscale Res. Lett. 14 (1), 231. doi:10.1186/s11671-019-3039-2
Kanter, E. M., Majumder, S., Kanter, G. J., Woeste, E. M., and Mahadevan-Jansen, A. (2009). Effect of Hormonal Variation on Raman Spectra for Cervical Disease Detection. Am. J. Obstet. Gynecol. 200 (5), 512 e1–5. doi:10.1016/j.ajog.2008.11.024
Koljenovic, S., Bakker Schut, T. C., Wolthuis, R., de Jong, B., Santos, L., and Caspers, P. J. (2005). Tissue Characterization Using High Wave Number Raman Spectroscopy. J. Biomed. Opt. 10 (3), 031116. doi:10.1117/1.1922307
Lee, C. W., and Tseng, F. G. (2018). Surface Enhanced Raman Scattering (SERS) Based Biomicrofluidics Systems for Trace Protein Analysis. Biomicrofluidics 12 (1), 011502. doi:10.1063/1.5012909
Lee, J. B., Lee, D. R., Choi, N. C., Jang, J., Park, C. H., Yoon, M. S., et al. (2015). Efficient Dermal Delivery of Retinyl Palmitate: Progressive Polarimetry and Raman Spectroscopy to Evaluate the Structure and Efficacy. Eur. J. Pharm. Sci. 78, 111–120. doi:10.1016/j.ejps.2015.07.009
Lee, K. S., Palatinszky, M., Pereira, F. C., Nguyen, J., Fernandez, V. I., Mueller, A. J., et al. (2019). An Automated Raman-Based Platform for the Sorting of Live Cells by Functional Properties. Nat. Microbiol. 4 (6), 1035–1048. doi:10.1038/s41564-019-0394-9
Lin, K., Zheng, W., Lim, C. M., and Huang, Z. (2017). Real-time In Vivo Diagnosis of Nasopharyngeal Carcinoma Using Rapid Fiber-Optic Raman Spectroscopy. Theranostics 7 (14), 3517–3526. doi:10.7150/thno.16359
Liu, L. (2018). Clinical Study in Treatment of Asthma by Acupoint Sticking Therapy Based on Raman Spectrum in Monitoring of Dermatitis Degree. Available from: http://www.chictr.org.cn/showproj.aspx?proj=28245.
Lohbauer, U., Pelka, M., Belli, R., Schmitt, J., Mocker, E., Jandt, K. D., et al. (2010). Degree of Conversion of Luting Resins Around Ceramic Inlays in Natural Deep Cavities: a Micro-raman Spectroscopy Analysis. Oper. Dent 35 (5), 579–586. doi:10.2341/10-012-l
Lord, R. C., and Yu, N.-T. (1970). Laser-excited Raman Spectroscopy of Biomolecules. J. Mol. Biol. 51 (2), 203–213. doi:10.1016/0022-2836(70)90137-3
Maheedhar, K., Bhat, R. A., Malini, R., Prathima, N. B., Keerthi, P., Kushtagi, P., et al. (2008). Diagnosis of Ovarian Cancer by Raman Spectroscopy: a Pilot Study. Photomed. Laser Surg. 26 (2), 83–90. doi:10.1089/pho.2007.2128
Matousek, P., Draper, E. R. C., Goodship, A. E., Clark, I. P., Ronayne, K. L., and Parker, A. W. (2006). Noninvasive Raman Spectroscopy of Human Tissue In Vivo. Appl. Spectrosc. 60 (7), 758–763. doi:10.1366/000370206777886955
Moncada, B., Castillo-Martínez, C., Arenas, E., León-Bejarano, F., Ramírez-Elías, M. G., and González, F. J. (2016). Raman Spectroscopy Analysis of the Skin of Patients with Melasma before Standard Treatment with Topical Corticosteroids, Retinoic Acid, and Hydroquinone Mixture. Skin Res. Technol. 22 (2), 170–173. doi:10.1111/srt.12245
Nakagawa, N., Matsumoto, M., and Sakai, S. (2010). In Vivomeasurement of the Water Content in the Dermis by Confocal Raman Spectroscopy. Skin Res. Technol. 16 (2), 137–141. doi:10.1111/j.1600-0846.2009.00410.x
Navarra, C. O., Breschi, L., Turco, G., Diolosà, M., Fontanive, L., Manzoli, L., et al. (2012). Degree of Conversion of Two-step Etch-And-Rinse Adhesives: In Situ Micro-raman Analysis. J. Dentistry 40 (9), 711–717. doi:10.1016/j.jdent.2012.05.001
Nguyen, J. Q., Gowani, Z. S., O'Connor, M., Pence, I. J., Nguyen, T.-Q., Holt, G. E., et al. (2016). Intraoperative Raman Spectroscopy of Soft Tissue Sarcomas. Lasers Surg. Med. 48 (8), 774–781. doi:10.1002/lsm.22564
Pascaretti-Grizon, F., Guillaume, B., Terranova, L., Arbez, B., Libouban, H., and Chappard, D. (2017). Maxillary Sinus Lift with Beta-Tricalcium Phosphate (β-TCP) in Edentulous Patients: A Nanotomographic and Raman Study. Calcif Tissue Int. 101 (3), 280–290. doi:10.1007/s00223-017-0280-5
Paula, S. S., Soares, L. E., do Espírito Santo, A. M., Martin, A. A., Cavalli, V., and Liporoni, P. C. (2010). FT-Raman and Energy Dispersive X-ray Fluorescence Spectrometric Analyses of Enamel Submitted to 38% Hydrogen Peroxide Bleaching, an Acidic Beverage, and Simulated Brushing. Photomed. Laser Surg. 28 (3), 391–396. doi:10.1089/pho.2008.2426
Pence, I., and Mahadevan-Jansen, A. (2016). Clinical Instrumentation and Applications of Raman Spectroscopy. Chem. Soc. Rev. 45 (7), 1958–1979. doi:10.1039/c5cs00581g
Pilot, R., Signorini, R., Durante, C., Orian, L., Bhamidipati, M., and Fabris, L. (2019). A Review on Surface-Enhanced Raman Scattering. Biosensors (Basel) 9 (2). doi:10.3390/bios9020057
Prieto, M. C. H., Matousek, P., Towrie, M., Parker, A. W., Wright, M., Ritchie, A. W., et al. (2005). Use of Picosecond Kerr-Gated Raman Spectroscopy to Suppress Signals from Both Surface and Deep Layers in Bladder and Prostate Tissue. J. Biomed. Opt. 10 (4), 044006. doi:10.1117/1.1991848
Qian, Z. (2018). The Value of Raman Spectroscopy Analysis of Peripheral Blood in the Identification of Atherosclerotic Plaque Stability. Available from: http://www.chictr.org.cn/showproj.aspx?proj=26730.
Qiu, Y. (2017). The Clinical Research of Raman Spectroscopy in Real-Time Diagnosis of Oral and Maxillofacial Tumors. Available from: http://www.chictr.org.cn/showproj.aspx?proj=21048.
Raman, C. V., and Krishnan, K. S. (1928). A New Type of Secondary Radiation. Nature 121 (3048), 501–502. doi:10.1038/121501c0
Richters, R. J. H., Falcone, D., Uzunbajakava, N. E., Varghese, B., Caspers, P. J., Puppels, G. J., et al. (2017). Sensitive Skin: Assessment of the Skin Barrier Using Confocal Raman Microspectroscopy. Skin Pharmacol. Physiol. 30 (1), 1–12. doi:10.1159/000452152
Robert, B. (2009). Resonance Raman Spectroscopy. Photosynth Res. 101 (2-3), 147–155. doi:10.1007/s11120-009-9440-4
Rohleder, D., Kocherscheidt, G., Gerber, K., Kiefer, W., Köhler, W., and Möcks, J. (2005). Comparison of Mid-infrared and Raman Spectroscopy in the Quantitative Analysis of Serum. J. Biomed. Opt. 10 (3), 031108. doi:10.1117/1.1911847
Sahu, A., Sawant, S., Mamgain, H., and Krishna, C. M. (2013). Raman Spectroscopy of Serum: an Exploratory Study for Detection of Oral Cancers. Analyst 138 (14), 4161–4174. doi:10.1039/c3an00308f
Schleusener, J., Gluszczynska, P., Reble, C., Gersonde, I., Helfmann, J., Cappius, H.-J., et al. (2015). Perturbation Factors in the Clinical Handling of a Fiber-Coupled Raman Probe for Cutaneous In Vivo Diagnostic Raman Spectroscopy. Appl. Spectrosc. 69 (2), 243–256. doi:10.1366/14-07482
Sigurdsson, S., Philipsen, P. A., Hansen, L. K., Larsen, J., Gniadecka, M., and Wulf, H. C. (2004). Detection of Skin Cancer by Classification of Raman Spectra. IEEE Trans. Biomed. Eng. 51 (10), 1784–1793. doi:10.1109/tbme.2004.831538
Stiles, P. L., Dieringer, J. A., Shah, N. C., and Van Duyne, R. P. (2008). Surface-enhanced Raman Spectroscopy. Annu. Rev. Anal. Chem. 1, 601–626. doi:10.1146/annurev.anchem.1.031207.112814
Tan, Y., Yan, B., Xue, L., Li, Y., Luo, X., and Ji, P. (2017). Surface-enhanced Raman Spectroscopy of Blood Serum Based on Gold Nanoparticles for the Diagnosis of the Oral Squamous Cell Carcinoma. Lipids Health Dis. 16 (1), 73. doi:10.1186/s12944-017-0465-y
Tanito, M., Obana, A., Gohto, Y., Okazaki, S., Gellermann, W., and Ohira, A. (2012). Macular Pigment Density Changes in Japanese Individuals Supplemented with Lutein or Zeaxanthin: Quantification via Resonance Raman Spectrophotometry and Autofluorescence Imaging. Jpn. J. Ophthalmol. 56 (5), 488–496. doi:10.1007/s10384-012-0157-0
U.S. National Library of Medicine (2015). Clinical Validation of a Raman Spectroscope to Determine Hepatic Fat Content. Available from: https://clinicaltrials.gov/ct2/show/NCT02621853.
U.S. National Library of Medicine (2020a). Detection of Circulating Tumor Cells in Breast Cancer Patients Using a Novel Microfluidic and Raman Spectrum Device. Available from: https://clinicaltrials.gov/ct2/show/NCT04239105.
U.S. National Library of Medicine (2020b). Non-invasive Assessment of Mechano-Chemical Properties of Urine Proteins by Hybrid Brillouin-Raman Spectroscopy. Available from: https://clinicaltrials.gov/ct2/show/NCT04311684.
U.S. National Library of Medicine (2020c). Raman Analysis of Saliva as Biomarker of COPD (BIO-RAnCh). Available from: https://clinicaltrials.gov/ct2/show/NCT04628962.
U.S. National Library of Medicine (2003). Raman Scattering Spectroscopy to Measure Macular Pigment. Available from: https://clinicaltrials.gov/ct2/show/NCT00060580.
U.S. National Library of Medicine (2014). Transdermal Hrt Relieving Postmenopausal Symptoms (Thrt). Available from: https://clinicaltrials.gov/ct2/show/NCT02033512.
Vedang, M. (2009). Toxicity with Tomotherapy Based Intensity Modified Radiation Therapy in Head and Neck Cancers and In Vivo Prediction of Radio-Responsiveness of Oropharyngeal Cancers Using Raman Spectroscopy. Available from: http://www.ctri.nic.in/Clinicaltrials/pmaindet2.php?trialid=1011.
Vuiblet, V., Fere, M., Bankole, E., Wynckel, A., Gobinet, C., and Birembaut, P. (2016). Raman-based Detection of Hydroxyethyl Starch in Kidney Allograft Biopsies as a Potential Marker of Allograft Quality in Kidney Transplant Recipients. Sci. Rep. 6, 33045. doi:10.1038/srep33045
Wang, W.-t., Zhang, H., Yuan, Y., Guo, Y., and He, S.-x. (2018). Research Progress of Raman Spectroscopy in Drug Analysis. AAPS PharmSciTech 19 (7), 2921–2928. doi:10.1208/s12249-018-1135-8
Wang, Y. (2020). Construction of Artificial Intelligence-Assisted Prostate Tumor Early Diagnosis System Based on Surface Enhanced Raman Spectroscopy. Available from: http://www.chictr.org.cn/showproj.aspx?proj=60141.
Xue, L., Sun, P., Ou, D., Chen, P., Chen, M., and Yan, B. (2014). Diagnosis of Pathological Minor Salivary Glands in Primary Sjogren's Syndrome by Using Raman Spectroscopy. Lasers Med. Sci. 29 (2), 723–728. doi:10.1007/s10103-013-1398-y
Xue, L., Yan, B., Li, Y., Tan, Y., Luo, X., and Wang, M. (2018). Surface-enhanced Raman Spectroscopy of Blood Serum Based on Gold Nanoparticles for Tumor Stages Detection and Histologic Grades Classification of Oral Squamous Cell Carcinoma. Ijn 13, 4977–4986. doi:10.2147/ijn.s167996
Zavaleta, C. L., Garai, E., Liu, J. T. C., Sensarn, S., Mandella, M. J., Van de Sompel, D., et al. (2013). A Raman-Based Endoscopic Strategy for Multiplexed Molecular Imaging. Proc. Natl. Acad. Sci. 110 (25), E2288–E2297. doi:10.1073/pnas.1211309110
Keywords: surface-enhanced raman spectroscopy, clinical trial, raman spectroscopy, disease, sample selection
Citation: Xi X and Liang C (2021) Perspective of Future SERS Clinical Application Based on Current Status of Raman Spectroscopy Clinical Trials. Front. Chem. 9:665841. doi: 10.3389/fchem.2021.665841
Received: 09 February 2021; Accepted: 18 June 2021;
Published: 20 July 2021.
Edited by:
Agata Królikowska, University of Warsaw, PolandReviewed by:
Xiaoru Zhang, Qingdao University of Science and Technology, ChinaPiotr Piotrowski, University of Warsaw, Poland
Copyright © 2021 Xi and Liang. This is an open-access article distributed under the terms of the Creative Commons Attribution License (CC BY). The use, distribution or reproduction in other forums is permitted, provided the original author(s) and the copyright owner(s) are credited and that the original publication in this journal is cited, in accordance with accepted academic practice. No use, distribution or reproduction is permitted which does not comply with these terms.
*Correspondence: Chongyang Liang, liang@jlu.edu.cn