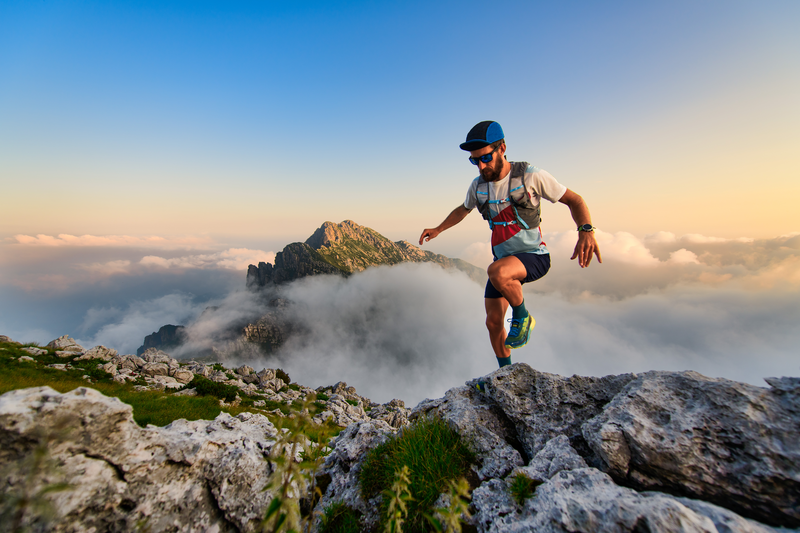
94% of researchers rate our articles as excellent or good
Learn more about the work of our research integrity team to safeguard the quality of each article we publish.
Find out more
ORIGINAL RESEARCH article
Front. Chem. , 30 April 2021
Sec. Medicinal and Pharmaceutical Chemistry
Volume 9 - 2021 | https://doi.org/10.3389/fchem.2021.608438
This article is part of the Research Topic Discovery and Development of Drugs for Neglected Diseases: Chagas Disease, Human African Trypanosomiasis, and Leishmaniasis. View all 11 articles
Current drugs for Chagas disease have long treatment regimens with occurrence of adverse drug effects leading to poor treatment compliance. Novel and efficacious medications are therefore highly needed. We previously reported on the discovery of NPD-0227 (2-isopropyl-5-(4-methoxy-3-(pyridin-3-yl)phenyl)-4,4-dimethyl-2,4-dihydro-3H-pyrazol-3-one) as a potent in vitro inhibitor of Trypanosoma cruzi (pIC50 = 6.4) with 100-fold selectivity over human MRC-5 cells. The present work describes a SAR study on the exploration of substituents on the phenylpyrazolone nitrogen. Modifications were either done directly onto this pyrazolone nitrogen or alternatively by introducing a piperidine linker. Attention was pointed toward the selection of substituents with a cLogP preferably below NPD-0227s cLogP of 3.5. Generally the more apolar compounds showed better activities then molecules with cLogPs <2.0. Several new compounds were identified with potencies that are in the same range as NPD-0227 (pIC50 = 6.4) and promising selectivities. While the potency could not be improved, valuable SAR was obtained. Furthermore the introduction of a piperidine linker offers new opportunities for derivatization as valuable novel starting points for future T. cruzi drug discovery.
The protozoan parasite Trypanosoma cruzi is the causative agent of Chagas disease. It is estimated that over six million people are infected worldwide, the majority in Latin-America where the parasite is endemic. (Lidani et al., 2019; WHO, 2021). T. cruzi is spread by several insect vectors, most of which belong to the triatomine family (Kollien and Schaub, 2000; Martinez et al., 2019). Other ways of transmission are via blood transfusions, laboratory accidents or parental transmission from mother to infant (Schmunis, 1999; Torrico et al., 2004; Rassi et al., 2010). The life cycle of T. cruzi consists of several developmental stages in the mammalian host and insect vector (Rassi et al., 2010). While the vector appears unaffected by the parasite, infected humans can develop life-threatening symptoms and pathologies. The acute stage of the disease starts shortly after the parasite enters the body but a strong immune response will cause a large reduction of the initial peak of parasitemia, however, without full elimination of the parasite leading to persistent infection of certain tissues (Dias, 1984). The acute symptoms are non-specific, such as fever, mild splenomegaly and edema, making diagnosis diffucult (Prata, 2001). After 2 months, the disease enters the chronic phase in which the parasite becomes dormant and no symptoms are observed. This dormant phase can last for over 10 years up to lifelong (Prata, 2001). Estimates of further evolution of chronic infection toward clinical symptoms, such as cardiopathy or megaesophagus and megacolon, ranges between 10–60% of infected people and varies widely in different regions (Coura and Viñas, 2010; Coura and Borges-Pereira, 2011). Patients can become life-long asymptomatic carriers thus representing a parasite reservoir.
Vector control, early diagnosis and effective chemotherapy are essential to combat Chagas disease. Two drugs are currently marketed: the nitro-imidazole benznidazole (1,Figure 1) and the nitro-furan nifurtimox (2) (Rassi et al., 2010). Both drugs contain a nitro-aromatic functionality which is commonly associated with toxic side effects. Furthermore, the treatment regimens of both vary between 60–90 days and are known to cause various side effects which cause patients to discontinue their treatment (Castro et al., 2006; Pinazo et al., 2013). Benznidazole (1) is currently used as first-line treatment as it has a better overall safety profile (Alpern et al., 2017). While the efficacy of both drugs is well established in the acute phase, much debate is ongoing on about their efficacy in the chronic phase (Sgambatti de Andrade et al., 1996; Zhang and Tarleton, 1999; Bern, 2011). The recent BENEFIT trial investigated the efficacy of Benznidazole (1) on chronic Chagas’ heart disease. While reduced parasitemia was observed by PCR, this did not result in significant reduction of clinical deterioration of cardiac function after 5 years (Morillo et al., 2015). Afterward several concerns were raised in literature with regard to the PCR analysis and benznidazole dosage, precluding firm conclusions on the efficacy potential in the chronic phase of infection (Hamers et al., 2016).
FIGURE 1. Current drugs used against Chagas disease: the nitro-imidazole benznidazole (1) and nitro-furan nifurtimox (2), and the recently discovered T. cruzi inhibitor NPD-0227 (3).
The overall need for new and improved chemotherapies for Chagas disease is high, both for current and future patients. New medication is also needed as contingency measure for upcoming resistance against the available current drugs (Coura and Viñas, 2010; Mejia et al., 2012). Unfortunately, the pipeline toward new drugs is largely empty. Most research on T. cruzi is performed in academia though the last few years several public-private collaborations have been initiated. In this paper, we describe part of the research of the EU-funded, public-private consortium PDE4NPD, that focuses on 3′5′-cyclic nucleotide phosphodiesterases (PDEs) as targets against several neglected tropical diseases. The present work further elaborates the SAR of the previously reported T. cruzi inhibitor NPD-0227 (3, Figure 1) and investigates the role of different substituents on the pyrazolone nitrogen (Sijm et al., 2019). Besides the SAR aiming at improving potency, compounds were also specifically designed to improve physicochemical properties, such as cLogP and solubility. As T. cruzi ultimately proceeds via a dormant intracellular form, a possible drug needs to pass several cell membranes before reaching the parasite. During this process the drug is transferred multiple times between hydrophobic and hydrophilic phases, as such an optimal cLogP is thought to be between 0 and 2 and the drug having no charges (Basore et al., 2015; Bennion et al., 2017).
Phenylpyrazolone analogues with a substituent directly on the pyrazolone nitrogen atom were synthesized using two different routes. The first route started with a Suzuki reaction to obtain dihydropyrazolone 5 (Route A, Scheme 1) which was then functionalized using sodium hydride and various acyl chlorides and sulfonylchlorides to obtain compounds 6–10 (Table 1). The second route (Route B) started with alkylation of the dihydropyrazolone 4, either using potassium carbonate to yield intermediates 11–12, or using sodium hydride to generate intermediates 13–18. These intermediates were then converted to the 3-pyridinyl derivatives via a Suzuki cross coupling, yielding final compounds 20–26 (Scheme 1, Table 1).
SCHEME 1. Reagents and conditions: a: 3-pyridinyl-B (OH)2, Pd (dppf)Cl2. DCM, Na2CO3, DME:H2O, 120 °C, 1 h, 12–78%, b: R-COCl (6, 7, 8) or R-SO2Cl (9, 10), NaH, DMF, rt, 18 h, 39–77%, c: epoxide (11–12), K2CO3, DMF, 100 °C, 16 h, 59–63%, d: R-Br (13–17) or R-Cl (18), NaH, DMF, rt, 18 h, 36–94%.
TABLE 1. In vitro activity against intracellular amastigotes of T.cruzi (Tulahuen strain)a and MRC-5 cellsa of phenyldihydropyrazolones with modifications directly on the pyrazolone nitrogen.
Introduction of methylene-oxadiazoles on the pyrazolone head group started with the previously synthesized ester analogues 13 and 14 (Scheme 2). Subsequent hydrazination yielded the corresponding hydrazides (27 and 28) which were ring-closed with either triethylorthoformate or cyanuric bromide to deliver the unsubstituted oxadiazoles (29 and 31) and the amine substituted oxadiazoles (30 and 32) respectively. These oxadiazoles were then converted to the corresponding 3-pyridinyl analogues via a Suzuki cross-coupling to yield compounds 33–36.
SCHEME 2. Reagents and conditions: a: N2H4, EtOH, rt, 18 h, 90–94%, b: (EtO)3CH, reflux,18 h, 34–78% (29–30), c: BrCN, NaHCO3, MeOH, H2O, o. n., 22–46% (31–32), d: 3-pyridinyl-B (OH)2, Pd (dppf) Cl2. DCM, Na2CO3, DME:H2O, 120 °C, 1 h 36–74%.
The synthesis of the piperidine substituted dihydropyrazolones started with keto-ester 37 (Scheme 3) which was prepared according to previously reported methodology (Sijm et al., 2019). This keto-ester was condensed with 4-hydrazinylpiperidine to yield piperidine substituted dihydropyrazolone 38. The piperidine nitrogen atom was protected using boc-anhydride to give intermediate (39) which could be used in a Suzuki cross-coupling to install the 3-pyridine moiety. Subsequent deprotection of 40 with 4 M HCl in dioxane yielded the free amine building block (41) which was used to install the desired electrophiles (42–83, Tables 2,3) via various methodologies.
SCHEME 3. Reagents and conditions: a: 4-hydrazinylpiperidine.2HCl, MeOH, H2O, reflux, 3 days, 80%, b: Boc2O, TEA, DCM, rt, 4 h, 89%, c: 3-pyridinyl-B (OH)2, Pd (dppf) Cl2. DCM, Na2CO3, DME:H2O, 120 °C, 1 h, 86%, d: 4 M HCl in dioxane, rt, 18 h, 84%, e: R-COCl (42, 43), R-SO2Cl (48), NaH, DMF, rt, 2 h, 38–42%, f: EDCI, HOBt, RCOOH (44, 46), DIPEA, DCM, 18–30 h, 23–31%, g: oxazole-5-carboxylic acid (45), T3P, DIPEA, EtOAc, 50 °C, 70 h, 7%, h: R-COCl (47) or R-SO2Cl (49–54) or OCN-R (55, 57–59, 61) or Cl (CO)NR1R2 (56, 60), TEA, DCM, rt, 2–42 h, 13–79%, i: epoxide (62–66), DMAP, i-PrOH, 50–100 °C, 2–120 h, 4–30%, j: formic acid (67), formaldehyde, 18 h, rt, 15%, k: R-CO-R (68–69, 71, 74), NaBH (OAc)3, AcOH, DCE, 70 °C, 18–72 h, 10–53%, l: R-Br (70), K2CO3, DMF, rt, 48 h, 10%, m: methylacrylate (72), DBN, ACN, 90h, rt, 45%, n: ClCH2CO-R (73), K2CO3, ACN, 24 h, rt, 39%, m: HCO-R (74–78), NaBH (OAc)3, AcOH, DCE, 22–72 h, 5–48%, q: Ar-Br (79–80), Pd2 (dba)3, BINAP, NaOtBu, 80 °C, 7 days, 11–19%, r: Cl-Ar (81), Cs2CO3, DMF, 90 °C, 4 h, 24%, s: F-Ar (82), DMSO, K2CO3, 110 °C, 6 days, 16% t: i: (83) NaHCO3, 4-NO2Ph-chloroformate, dioxane, rt, 22 h, ii: K2CO3, morpholine, DMF, rt, 4 days.
TABLE 2. In vitro activity against intracellular amastigotes of T.cruzi (Tulahuen strain)a and MRC-5 cellsa of phenyldihydropyrazolones with a piperidine linker.
TABLE 3. In vitro activity against intracellular amastigotes of T.cruzi (Tulahuen strain)a and MRC-5 cellsa toxicity by phenyldihydropyrazolones with a piperidine linker.
All compounds were tested for their trypanocidal activity against intracellular forms of T. cruzi (Tulahuen CL2, β-galactosidase strain (drug sensitive strain (discrete typing units, DTU VI)) as well as for cytotoxicity on MRC-5SV2 cells (human lung fibroblasts). (Blaazer et al., 2014).
In the previous work by Sijm et al., the pyrazolone nitrogen was substituted with various (cyclo)-alkyl moieties resulting in the identification of NPD-0227 (3) as a potent (pIC50 = 6.4) T. cruzi inhibitor with 100-fold selectivity over human MRC-5 cells (Table 1) (Sijm et al., 2019; Sijm et al., 2020). To further explore SAR and to investigate molecules with varying cLogPs, more polar and diverse substituents was explored.
Introduction of a carbonyl-alkyl moieties directly onto the pyrazolone nitrogen (6–8, Table 1) resulted in weak inhibitors with pIC50’s around 4.5. Similarly, introduction of sulphonyl-alkyl moieties such as dimethylsulfonamide (9) or sulphonylcyclopentyl (10) did not lead to inhibitors with pIC50 values above 5.0. Epoxides were used to introduce β-alcohols on the pyrazolone ring resulting in compounds 19 and 20. These alcohols also showed a large decrease in activity compared to NPD-0227 (pIC50 = 6.4) with pIC50’s of 4.4 and 5.1, respectively. Similarly, the introduction of ethyl-esters (21, 22) resulted in decreased potencies compared to NPD-0227 with both compounds showing pIC50 values of only 5.2.
While the substituents with a polar moiety (6–10, 19–22, Table 1) showed decreased activities, as expected, introduction of more apolar moieties such as methyl-cyclohexyl (23) and pyrazole (24) showed moderate activity with pIC50 values of 5.5. Introduction of more polar substituents attached to an aliphatic linker, such as imidazole 25 and morpholine 26 with cLogPs around 2.3 resulted in lower potencies (pIC50 < 4.6). While the introduction of various oxadiazoles (33–36) on this position yielded compounds with a more desirable cLogP’s, this did not lead to very active compounds, with 1-propan-oxazole (34) showed the highest potency (pIC50 = 5.2). As activities were generally close to the lower detection limit of the assay, selectivity indexes were quite low or defined as “bigger then”.
The initial attempts to introduce polar functional groups such as carbonyls, sulphonyls and alcohols close to the pyrazolone ring had a negative effect on their potency. One of the most potent compounds so far was methylcyclohexyl 23, a bulky and apolar substituent. It was thought that introduction of a piperidine would introduce a similar sized moiety which would improve options to introduce polarity as a new handle to further modify.
This piperidine linker was first investigated by forming amides with corresponding acid chlorides or carboxylic acids, which led to analogues 42–47 (Table 2). These groups introduced some polarity but these modifications did not lead to potent ligands (pIC50 < 5.3). Introducing sulphonamides instead of regular amides gave similar results as the majority showed moderately low activity (48–54). Alkyl-sulphonamides with a cyclopropyl (49), isopropyl (50), dimethylamino (51) and cyclopentyl (52) all showed pIC50’s around 5.1, while the smaller methyl substituted sulphonamide (48) had a slightly lower activity (pIC50 = 4.5). Introduction of an aromatic ring on to the sulphonamide did not have a positive effect. Tosylate 53 (cLogP = 4.4) was inactive with a pIC50 below 4.2. Introduction of an extra carbon between the sulphonamide and the benzene ring was successful as methylbenzyl 54 (cLogP = 3.8) showed a similar potency as NPD-0227 (3, Table 1) with a pIC50 value of 6.2 and >100-fold selectivity over human MRC-5.
The boc-protected analogue 40 (cLogP = 3.6; pIC50 = 5.9, Table 2) which was obtained during the synthesis of the free-amine building block showed that relatively large substituents are allowed on the piperidine linker, which was confirmed by sulphonamide 54. The preference for larger, bulky substituents is also apparent with the urea linked analogues (55–59). While the carbamide is inactive (55, cLogP = 1.6; pIC50 = <4.2), introduction of larger apolar groups increased activity (57–59), with the most potent compound being the 4-fluorophenyl 59 (pIC50 = 5.9; cLogP = 4.0, 25-fold selectivity). The even more bulky disopropyl analogue (60), however, was totally inactive (pIC50 < 4.2). Introduction of more polar substituents attached to the urea linker did not improve activity with pIC50 values of <4.2 for ethylacetate derivative 61 (cLogP = 1.8) and 4.9 for the morpholine analogue (83, cLogP = 1.8). Two types of epoxides were used to introduce β-alcohols. While the aliphatic analogues 62–64 (Table 3) showed low potencies (pIC50 < 4.6), the aromatic derivatives (65–66) showed micromolar activities with pIC50 values of 5.6 and 5.7. Selectivity of the unsubstituted phenyl ring (65) was however a lot better than the 4-fluorophenyl (66).
As shown in Table 1 and in previous work, aliphatic substituents on the dihydropyrazolone nitrogen led to the most potent compounds (Sijm et al., 2019). Introduction of aliphatic substituents on the piperidine nitrogen resulted in poor potencies, possibly caused by the introduction of a tertiary amine which can be protonated, as charged compounds are less likely to cross the cell membrane. The unsubstituted piperidine (41, cLogP = 2.3, Table 3) is totally inactive (pIC50 < 4.2) and the alkylated analogues (67–73) are not very active inhibitors (pIC50 < 5.0). Analogues with an aromatic ring showed higher activities: the 2-furanylmethyl (74, cLogP = 3.5) and the 3-pyridineylmethyl (77, cLogP = 4.4) showed pIC50 values of 5.0 and 5.3, respectively. The benzyl substituted piperidine 75 and the 4-pyridinemethyl 78 both showed pIC50 values around 5.6, while 4-fluorobenzyl 76 (cLogP = 4.6) showed sub-micromolar potency with a pIC50 of 6.1. Selectivity indexes of these compounds were moderate to poor, with the best compound, 4-pyridinemethyl 78 showing 16-fold selectivity toward T. cruzi over MRC-5 cells.
The final set of substituents on the piperidine linker were aromatic rings (Table 3, 79–82). This sub-series showed moderate activities with the 3-cyanophenyl (79), 4-methylbenzoate (81) and methyl-5-picolinate (82) all having low micromolar activities (pIC50 = 5.7). The 3-pyridinyl analogue (80) was less active with a pIC50 of 5.2. Best selectivity index was observed for 3-cyanophenyl 79 and methyl-5-picolinate 82, which both had over 32-fold selectivity.
Overall, the introduction of polar moieties on the phenyl-dihydropyrazolones generally led to low activities against T. cruzi. Especially the introduction of a polar moiety directly next to the pyrazolone nitogen such as all carbonyl, sulphonyl and β-alcohol linked moieties (6–10, 19, 20) resulted in analogues with low potencies. Introduction of aliphatic substituents or aromatic moieties resulted in interesting new anti-T. cruzi inhibitors with the best compounds 54 and 76 reaching sub micromolar potency. Installing a piperidine linker allowed for the introduction of a variety of substituents and it became apparent that aromatic moieties, either directly, with a methylene bridge or with a 2-atom linker performed best, showing pIC50’s around 6.0.
Our study results in valuable SAR data that has been obtained by introducing a variety of substituents on the dihydropyrazolone nitrogen atom. While decorating this position is synthetically very efficient and a wide variety of chemical functionalities are allowed, our studies did not lead to compounds that have a better activity than NPD-0227. Especially the piperidine linker opens a whole new range of options to introduce substituents and analogues reached low micromolar inhibitory activities against T. cruzi. The most active compounds were obtained if the piperidine is substituted with apolar moieties such as aryl or benzyl rings. Introduction of more polar substituents, such as imidazole 25 (cLogP = 2.3), succinimide 46 (cLogP = 0.9) and morpholine 73 (cLogP = 1.6) generally lead to compounds with high micromolar activities. The structural moiety performing best is a 2-atom linker followed by an aromatic moiety, of which sulphonamide 54 (cLogP = 3.8), urea 59 (cLogP = 4.0) and β-alcohols 65 and 66 (cLogP = 3.8/3.9) performed best. These molecules all have activities around 6.0 (pIC50) and especially sulphonamide 54 is promising with a pIC50 of 6.2 and >100-fold selectivity over MRC-5 cells. Also other aromatic substituents performed well, such as 4-fluorobenzyl (76), 3-cyanophenyl (79) and 5-methylnicotinate (82) show promising activities around 6.0, with 79 and 82 showing best selectivies (>32-fold) toward T. cruzi. While these results of this study are important in the design of novel ‘drug leads’ for Chagas disease, the original goal, of identifying high potency molecules with a lower cLogP did not succeed. Most potent compounds with a cLogP below 2.0 were oxadiazole 33 and sulfamide 51, which both showed a pIC50 of 5.1.
Trypanosoma cruzi in vitro assay. Bloodstream trypomastigotes of the Y strain of T. cruzi were obtained by cardiac puncture of infected Swiss Webster mice on the parasitaemia peak (Meirelles et al., 1986; Batista et al., 2010). For the standard in vitro susceptibility assay on intracellular amastigotes, T. cruzi Tulahuen CL2, β-galactosidase strain (nifurtimox-sensitive) was used. The strain is maintained on MRC-5SV2 (human lung fibroblast) cells in MEM medium, supplemented with 200 mM L-glutamine, 16.5 mM NaHCO3 and 5% inactivated fetal calf serum (FCSi). All cultures and assays were conducted at 37°C under an atmosphere of 5% CO2. Benznidazole was included in the assays as a positive control.
MRC-5 cytotoxicity in vitro assay. MRC-5-SV2 cells, originally from a human diploid lung cell line, were cultivated in MEM supplemented with L-glutamine (20 mM), 16.5 mM sodium hydrogen carbonate and 5% FCSi. For the assay, 104 cells/well were seeded onto the test plates containing the pre-diluted sample and incubated at 37°C and 5% CO2 for 72 h. Cell viability was assessed fluorometrically 4 h after addition of resazurin (excitation 550 nm, emission 590 nm). The results are expressed as percentage reduction in cell viability compared to untreated controls. Tamoxifen was included in the assays as a positive control.
The use of laboratory rodents was carried out in strict accordance to all mandatory guidelines (EU directives, including the Revised Directive 2010/63/EU on the Protection of Animals used for Scientific Purposes that came into force on January 1, 2013, and the Declaration of Helsinki in its latest version).
Chemicals and reagents were obtained from commercial suppliers and were used without further purification. Anhydrous DMF, THF, and DCM were obtained by passing them through an activated alumina column prior to use. Microwave reactions were executed using a Biotage® Initiator microwave system. 1H NMR spectra were recorded on a Bruker Avance 250 (250 MHz), Bruker Avance 400 (400 MHz), Bruker Avance 500 (500 MHz) or Bruker 600 Avance (600 MHz) spectrometer. Data are reported as follows: chemical shift, integration, multiplicity (s = singlet, d = doublet, dd = double doublet, t = triplet, dt = double triplet, q = quartet, p = pentet, h = heptet, bs = broad singlet, m = multiplet), and coupling constants (Hz). Chemical shifts are reported in ppm with the natural abundance of deuterium in the solvent as the internal reference [CDCl3: δ 7.26, (CD3)2SO: δ 2.50]. 13C NMR spectra were recorded on a Bruker Avance 500 (126 MHz) or Bruker Avance 600 (150 MHz). Chemical shifts are reported in ppm with the solvent resonance resulting from incomplete deuteration as the internal reference [CDCl3: δ 77.16 or (CD3)2SO: δ 39.52]. Systematic names for molecules according to IUPAC rules were generated using the Chemdraw AutoName program. LC-MS data was gathered using a Shimadzu HPLC/MS workstation with a LC-20AD pump system, SPD-M20A diode array detection, and a LCMS-2010 EV mass spectrometer. The column used is an Xbridge C18 5 μm column (100 mm × 4.6 mm). Solvents used were the following: solvent B = ACN, 0.1% formic Acid; solvent A = water, 0.1% formic acid. The analysis was conducted using a x?ow rate of 1.0 ml/min, start 5% B, linear gradient to 90% B in 4.5 min, then 1.5 min at 90% B, linear gradient to 5% B in 0.5 min and then 1.5 min at 5% B, total run time of 8 min. All reported compounds have purities >95%, measured at 254 nm, unless otherwise mentioned. All HRMS spectra were recorded on a Bruker micrOTOF mass spectrometer using ESI in positive-ion mode. In case of bromine containing molecules, the lowest peak of 79Br was reported. Column puri?cations were either carried out automatically using Biotage equipment or manually, using 60–200 mesh silica. TLC analyses were performed with Merck F254 alumina silica plates using UV visualization. All reactions were done under N2 atmosphere, unless specifically mentioned. The cLogPs were calculated using CDD vault, CDD vault uses the ionic logP algorithm.
Compound 5, 6, 15, 23, 27, 29, 33, 38–42 and 83 are described below, other compounds can be found in the supporting information.
Pyrazolone 4 (1.0 g, 3.4 mmol) and pyridin-3-ylboronic acid (0.62 g, 5.1 mmol) were charged to a microwave tube after which DME (12 ml) and 1 M Na2CO3 (6.7 ml, 6.7 mmol) were added. The mixture was degassed with N2 for 5 m after which Pd (dppf)Cl2 (0.28 g, 34 mmol) was added. The reaction was heated at 120°C for 1 h in the microwave. The reaction mixture was diluted with EtOAc (30 ml) and filtered over Celite. The residue was washed with saturated NaHCO3 (2 ml × 30 ml) and brine (1 ml × 30 ml). The organic phase was dried over Na2SO4, filtered and concentrated in vacuo. The remaining crude was purified over SiO2 using a gradient of 50% EtOAc in heptane toward 5% MeOH in EtOAc to yield 700 mg (2.4 mmol, 70%) of the title compound as a white solid. 1H NMR (500 MHz, CDCl3) δ 11.50 (s, 1H), 8.69 (s, 1H), 8.55 (d, J = 3.8 Hz, 1H), 7.91 (apparent dt, J = 7.9, 1.9 Hz, 1H), 7.84 (dd, J = 8.7, 2.3 Hz, 1H), 7.73 (d, J = 2.3 Hz, 1H), 7.46 (dd, J = 7.9, 4.8 Hz, 1H), 7.22 (d, J = 8.8 Hz, 1H), 3.83 (s, 3H), 1.37 (s, 6H). 13C NMR (126 MHz, CDCl3) δ 181.1, 161.6, 157.7, 150.0, 148.6, 137.1, 133.7, 128.1, 128.0, 127.4, 124.4, 123.8, 112.4, 56.3, 46.9, 22.5. LC-MS (ESI) m/z found: 296 (M + H)+; retention time: 2.67 min. HRMS-ESI (M + H)+ calculated for C17H18N3O2: 296.1394, found: 296.1391.
Pyrazolone 5 (50 mg, 0.17 mmol) was dissolved in DMF (2 ml) and sodium hydride (60% in mineral oil) (10 mg, 0.25 mmol) was added. After stirring for 30 min cyclopropanecarbonyl chloride (20 mg, 0.19 mmol) was added and the mixture was stirred at rt for 18 h after which the reaction was quenched with water (25 ml) and extracted with EtOAc (25 ml). The organic layer was washed with sat. aq. NaHCO3 (2 ml × 20 ml), brine (20 ml) and dried over MgSO4 after which volatiles were evaporated yielding 24 mg (0.07 mmol, 39%) of the title compound as a white solid. 1H NMR (500 MHz, CDCl3) δ 8.76 (d, J = 2.0 Hz, 1H), 8.60 (dd, J = 4.8, 1.7 Hz, 1H), 7.95–7.84 (m, 3H), 7.38 (dd, J = 7.8, 4.8 Hz, 1H), 7.09–7.03 (m, 1H), 3.89 (s, 3H), 3.05–2.97 (m, 1H), 1.61 (s, 6H), 1.32–1.25 (m, 2H), 1.11–1.02 (m, 2H). 13C NMR (126 MHz, CDCl3) δ 178.0, 171.9, 162.3, 158.7, 150.0, 148.3, 137.1, 133.3, 129.5, 128.7, 127.8, 123.1, 122.9, 111.2, 55.8, 49.9, 23.2, 13.5, 11.3. LC-MS (ESI) m/z found: 364 (M + H)+; retention time: 3.43 min. HRMS-ESI (M + H)+ calculated for C21H22N3O3: 364.1656, found: 364.1653.
Pyrazolone 4 (5.0 g, 16.8 mmol) was dissolved in DMF (35 ml) and sodium hydride (60% in mineral oil) (0.49 g, 20.2 mmol) was added. After stirring for 30 min ethyl 2-bromoacetate (2.23 ml, 20.2 mmol) was added and the mixture was heated to 50°C for 3 h after which the reaction was quenched with water (250 ml). Solids were filtered off and dried in vacuo, yielding 5.6 g (14.6 mmol, 87%) of the title compound as a white solid. 1H NMR (500 MHz, CDCl3) δ 8.05 (d, J = 2.2 Hz, 1H), 7.70 (dd, J = 8.6, 2.2 Hz, 1H), 6.93 (d, J = 8.7 Hz, 1H), 4.55 (s, 2H), 4.23 (q, J = 7.1 Hz, 2H), 3.95 (s, 3H), 1.53 (s, 6H), 1.29 (t, J = 7.1 Hz, 3H). 13C NMR (126 MHz, CDCl3) δ 179.1, 167.8, 160.9, 157.2, 131.4, 126.7, 124.6, 112.3, 111.5, 61.7, 56.4, 48.1, 45.8, 22.5, 14.1. LC-MS (ESI) m/z found: 383 (M + H)+; retention time: 4.75 min. HRMS-ESI (M + H)+ calculated for C16H20BrN2O4: 383.0601, found: 383.0588.
Pyrazolone 4 (100 mg, 0.30 mmol) was dissolved in DMF (3 ml) and sodium hydride (60% in mineral oil) (15 mg, 0.38 mmol) was added. After stirring for 30 min (bromomethyl)cyclohexane (63 mg, 0.67 mmol) was added and the mixture was stirred at rt for 18 h after which the reaction was quenched with water (25 ml) and extracted with EtOAc (25 ml). The organic layer was washed with brine (2 ml × 20 ml) and dried over MgSO4 after which volatiles were evaporated yielding 105 mg (0.27 mmol, 91%) of the title compound as a transparent oil. 1H NMR (500 MHz, CDCl3) δ 8.03 (d, J = 2.2 Hz, 1H), 7.66 (dd, J = 8.6, 2.2 Hz, 1H), 6.90 (d, J = 8.7 Hz, 1H), 3.92 (s, 3H), 3.56 (d, J = 7.2 Hz, 2H), 1.89–1.80 (m, 1H), 1.74–1.68 (m, 2H), 1.67–1.61 (m, 3H), 1.45 (s, 6H), 1.28–1.13 (m, 5H), 1.08–0.89 (m, 2H). 13C NMR (126 MHz, CDCl3) δ 178.6, 160.1, 156.9, 131.2, 126.5, 125.0, 112.3, 111.5, 56.4, 50.3, 48.3, 36.9, 30.5, 26.4, 25.7, 22.8. LC-MS (ESI) m/z found: 393 (M + H)+; retention time: 6.05 min. HRMS-ESI (M + H)+ calculated for C19H26BrN2O2: 393.1172, found: 393.1156.
Pyrazolone 15 (100 mg, 0.16 mmol) and pyridin-3-ylboronic acid (47 mg, 0.38 mmol) were charged to a microwave tube after which DME (3 ml) and 1 M Na2CO3 (0.8 ml, 0.8 mmol) were added. The mixture was degassed with N2 for 5 m after which Pd (dppf) Cl2 (21 mg, 25 µmol) was added. The reaction was heated at 120°C for 1 h in the microwave. The reaction mixture was diluted with EtOAc (30 ml) and filtered over Celite. The residue was washed with saturated NaHCO3 (2 ml × 30 ml) and brine (1 ml × 30 ml). The organic phase was dried over Na2SO4, filtered and concentrated in vacuo. The remaining crude was purified over SiO2 using a gradient of 50% EtOAc in heptane toward 100% EtOAc to yield 64 mg (0.16 mmol, 64%) of the title compound as a white solid. 1H NMR (500 MHz, CDCl3) δ 8.78 (d, J = 2.1 Hz, 1H), 8.59 (d, J = 4.8 Hz, 1H), 7.88 (apparent dt, J = 7.8, 1.9 Hz, 1H), 7.82 (d, J = 2.2 Hz, 1H), 7.77 (dd, J = 8.6, 2.2 Hz, 1H), 7.37 (dd, J = 7.9, 4.9 Hz, 1H), 7.03 (d, J = 8.6 Hz, 1H), 3.87 (s, 3H), 3.58 (d, J = 7.2 Hz, 2H), 2.05–1.79 (m, 2H), 1.76–1.60 (m, 5H), 1.50 (s, 6H), 1.29–1.10 (m, 3H), 1.06–0.95 (m, 2H). 13C NMR (126 MHz, CDCl3) δ 178.7, 161.0, 157.8, 150.1, 148.2, 137.0, 133.6, 128.7, 127.6, 127.5, 124.2, 123.1, 111.1, 55.8, 50.3, 48.4, 36.9, 30.5, 26.4, 25.7, 22.9. LC-MS (ESI) m/z found: 392 (M + H)+; retention time: 4.54 min. HRMS-ESI (M + H)+ calculated for C24H30N3O2: 392.2333, found: 392.2319.
Ester 13 (1.5 g, 3.91 mmol) was refluxed in hydrazine monohydrate (1.89 ml, 39.1 mmol) and ethanol (25 ml) for 18 h. After that the mixture was allowed to cool down, the mixture was concentrated and 20 ml of water was added. The solids were filtered off and dried in vacuo yielding 1.36 g (3.7 mmol, 94%) of the title compound as a white solid. 1H NMR (500 MHz, DMSO-d6) δ 9.29 (s, 1H), 7.96 (s, 1H), 7.79 (d, J = 8.6 Hz, 1H), 7.17 (d, J = 8.6 Hz, 1H), 4.29 (d, J = 13.2 Hz, 4H), 3.90 (s, 3H), 1.39 (s, 6H). 13C NMR (126 MHz, DMSO-d6) δ 178.9, 166.4, 160.0, 157.0, 130.5, 127.6, 124.6, 113.1, 111.8, 56.9, 47.7, 45.9, 22.4. LC-MS (ESI) m/z found:369 (M + H)+; retention time: 3.59 min.
Hydrazide 27 (200 mg, 0.54 mmol) was stirred in triethylorthoformate (0.9 ml, 5.4 mmol) and PTSA (10.30 mg, 0.054 mmol) was added. The mixture was heated at 80°C for 18 h after which the volatiles were evaporated and the resulting crude was purified over SiO2 using a gradient of 20% EtOAc in heptane toward 80% EtOAc in heptane. This yielded 70 mg (0.19 mmol, 34%) of the title compound as a white solid. 1H NMR (500 MHz, CDCl3) δ 8.42 (s, 1H), 7.99 (d, J = 2.2 Hz, 1H), 7.66 (dd, J = 8.7, 2.2 Hz, 1H), 6.90 (d, J = 8.7 Hz, 1H), 5.22 (s, 2H), 3.92 (s, 3H), 1.52 (s, 6H). 13C NMR (126 MHz, CDCl3) δ 178.5, 161.9, 161.6, 157.4, 153.6, 131.4, 126.9, 124.2, 112.3, 111.6, 56.4, 48.1, 39.0, 22.6. LC-MS (ESI) m/z found: 397 (M + H)+; retention time: 4.27 min. HRMS-ESI (M + H)+ calculated for C15H16BrN4O3: 379.0400, found: 379.0391.
Pyrazolone 29 (50 mg, 0.13 mmol) and pyridin-3-ylboronic acid (24 mg, 0.38 mmol) were charged to a microwave tube after which DME (3 ml) and 1 M Na2CO3 (0.5 ml, 0.5 mmol) were added. The mixture was degassed with N2 for 5 m after which Pd (dppf) Cl2 (11 mg, 13 µmol) was added. The reaction was heated at 120°C for 1 h in the microwave. The reaction mixture was diluted with EtOAc (30 ml) and filtered over Celite. The residue was washed with saturated NaHCO3 (2 ml × 30 ml) and brine (1 ml × 30 ml). The organic phase was dried over Na2SO4, filtered and concentrated in vacuo. The remaining crude was purified over SiO2 using a gradient of 50% EtOAc in heptane toward 100% EtOAc to yield 36 mg (0.10 mmol, 72%) of the title compound as a white solid. 1H NMR (500 MHz, CDCl3) δ 8.79 (s, 1H), 8.61 (s, 1H), 8.41 (s, 1H), 7.95–7.83 (m, 1H), 7.83–7.73 (m, 2H), 7.41 (s, 1H), 7.03 (d, J = 8.4 Hz, 1H), 5.23 (s, 2H), 3.87 (s, 3H), 1.56 (s, 6H). 13C NMR (126 MHz, CDCl3) δ 178.6, 162.4, 162.0, 158.2, 153.6, 149.5, 147.6, 137.5, 133.9, 128.9, 128.0, 127.4, 123.4, 111.2, 55.9, 48.2, 39.0, 22.8. LC-MS (ESI) m/z found: 378 (M + H)+; retention time: 2.93 min. HRMS-ESI (M + H)+ calculated for C20H20N5O3: 378.1562, found: 378.1562.
Keto-ester 37 (32 g, 102 mmol) was dissolved in methanol (200 ml) and stirred. To this solution 4-hydrazinylpiperidine dihydrochloride (76 g, 406 mmol) dissolved in Water (80 ml) was added. The mixture was refluxed for 72 h after which 150 ml water was added and MeOH was removed in vacuo. To the remaining solution 10 M NaOH was added slowly until the pH was ∼13. Solids were collected and washed with water (20 ml) to yield 31 g (82 mmol, 80%) of the title compound. 1H NMR (500 MHz, CDCl3) δ 8.00 (d, J = 2.2 Hz, 1H), 7.78 (dd, J = 8.6, 2.2 Hz, 1H), 7.16 (d, J = 8.8 Hz, 1H), 4.06–3.94 (m, 1H), 3.90 (s, 3H), 3.04–2.93 (m, 2H), 2.53–2.51 (m, 2H), 1.84–1.75 (m, 2H), 1.66–1.56 (m, 2H), 1.35 (s, 6H). 13C NMR (126 MHz, CDCl3) δ 177.5, 159.7, 156.9, 130.4, 127.4, 124.8, 113.1, 111.9, 56.9, 51.5, 48.4, 45.6, 31.7, 22.4. LC-MS (ESI) m/z found: 380 (M + H)+; retention time: 3.19 min. HRMS-ESI (M + H)+ calculated for C17H23BrN3O2: 380.0968, found: 380.0968.
Piperidine 38 (25 g, 65.7 mmol) was added to a round-bottom flask after which DCM (500 ml) was added, followed by di-tert-butyl dicarbonate (15.6 ml, 67.1 mmol) and triethylamine (9.4 ml, 67.1 mmol). The reaction mixture was extracted with water (2 ml × 400 ml) and brine (400 ml) and the organic layer was dried over Na2SO4. Solids were filtered off and volatiles were evaporated yielding 28 g (58 mmol, 89%) of the title compound as a light-brown solid. 1H NMR (500 MHz, CDCl3) δ 8.05 (d, J = 2.1 Hz, 1H), 7.70 (dd, J = 8.7, 2.2 Hz, 1H), 6.94 (d, J = 8.6 Hz, 1H), 4.31–4.20 (m, 3H), 3.96 (s, 3H), 2.87 (t, J = 12.9 Hz, 2H), 2.03 (apparent qd, J = 12.5, 4.5 Hz, 2H), 1.83–1.78 (m, 2H), 1.51 (s, 9H), 1.48 (s, 6H). LC-MS (ESI) m/z found: 424 (M + H, -t-Bu)+; retention time: 5.34 min. HRMS-ESI (M + H)+ calculated for C22H31N3O4: 480.1492, found: 480.1477.
Boc-protected piperidine 39 (25.2 g, 52.5 mmol) and pyridin-3-ylboronic acid (9.0 g, 73 mmol) were charged to a round-bottom flask after which DME (400 ml) and 1 M Na2CO3 (210 ml, 210 mmol) were added. The mixture was degassed with N2 for 15 m after which Pd (dppf) Cl2 (2.1 g, 2.6 mmol) was added. The reaction was heated at 80°C for 16 h. The reaction mixture was diluted with MTBE (500 ml) and filtered over Celite. The residue was washed with saturated NaHCO3 (2 ml × 600 ml) and brine (1 ml × 600 ml). The organic phase was dried over Na2SO4, filtered and concentrated in vacuo to yield 21.5 g (44.9 mmol, 86%) of the title compound. 1H NMR (500 MHz, CDCl3) δ 8.75 (s, 1H), 8.56 (d, J = 4.0 Hz, 1H), 7.84 (d, J = 7.9 Hz, 1H), 7.78 (d, J = 2.0 Hz, 1H), 7.75 (dd, J = 8.6, 2.1 Hz, 1H), 7.35 (dd, J = 7.7, 4.9 Hz, 1H), 7.01 (d, J = 8.7 Hz, 1H), 4.33–4.08 (m, 3H), 3.85 (s, 3H), 2.82 (s, 2H), 1.99 (apparent q, J = 11.2 Hz, 2H), 1.76 (d, J = 11.6 Hz, 2H), 1.47 (s, 7H), 1.45 (s, 9H). 13C NMR (126 MHz, CDCl3) δ 178.0, 161.2, 157.8, 154.7, 150.1, 148.3, 136.8, 133.5, 128.7, 127.7, 127.5, 124.0, 123.1, 111.1, 79.7, 55.8, 50.8, 48.8, 30.0, 28.4, 22.7. LC-MS (ESI) m/z found: 479 (M + H)+; retention time: 4.03 min. HRMS-ESI (M + H)+ calculated for C27H35N4O4: 479.2653, found: 479.2651.
Boc-protected piperidine 40 (25.1 g, 52.4 mmol) was dissolved in dioxane (100 ml) and hydrogen chloride in dioxane (4 N) (131 ml, 524 mmol) was added in portions. The reaction was stirred for 40 h after which volatiles were evaporated. Attempts were made to recrystallize the resulting dark-oil from MTBE, iPr-OH, iPr-OH:H2O to no avail. The crude was redissolved in EtOAc (800 ml) and extracted with aq. sat. Na2CO3 (2 ml × 500 ml) and brine (500 ml). The organic layer was dried over Na2SO4 and volatiles were evaporated. Attempts to recrystallize from i-PrOH or iPrOH:H2O were again unsuccessful. Evaporation of i-PrOH:H2O ultimately yielded 16.7 g (44.1 mmol, 84%) of the title compound as a light brown powder. 1H NMR (500 MHz, CDCl3) δ 8.70–8.67 (m, 1H), 8.56 (dd, J = 4.9, 1.6 Hz, 1H), 7.91 (apparent dt, J = 8.0, 1.9 Hz, 1H), 7.85 (dd, J = 8.7, 1.9 Hz, 1H), 7.76 (d, J = 1.8 Hz, 1H), 7.47 (dd, J = 7.7, 4.9 Hz, 1H), 7.23 (d, J = 8.8 Hz, 1H), 4.08–3.96 (m, 1H), 3.84 (s, 3H), 3.00 (d, J = 12.3 Hz, 2H), 2.58–2.51 (m, 2H), 1.81 (apparent qd, J = 12.3, 4.1 Hz, 2H), 1.68–1.58 (m, 2H), 1.39 (s, 6H). 13C NMR (126 MHz, CDCl3) δ 177.2, 160.3, 157.5, 149.7, 149.6, 148.3, 148.2, 136.7, 133.2, 127.8, 127.2, 123.5, 123.4, 112.0, 55.9, 51.0, 48.0, 45.2, 31.2, 22.2. LC-MS (ESI) m/z found: 379 (M + H)+; retention time: 2.40 min. HRMS-ESI (M + H)+ calculated for C22H27N4O2: 379.2129, found: 379.2121.
Amine 41 (100 mg, 0.26 mmol) was stirred with sodium hydride (60% in mineral oil) (16 mg, 0.40 mmol) in DMF (1 ml). After 30 min cyclopropanecarbonyl chloride (0.026 ml, 0.29 mmol) was added. The reaction was stirred for 2 h after which the reaction was quenched with water (20 ml) and extracted with EtOAc (20 ml). The organic layer was washed with sat. aq. NaHCO3 solution (2 ml × 20 ml), brine (20 ml) and dried over MgSO4. Volatiles were evaporated and the remaining crude was purified over SiO2 using a gradient of 50% EtOAc in heptane toward 5% MeOH in EtOAc, yielding 45 mg (0.10 mmol, 38%) of the title compound as a white solid. 1H NMR (500 MHz, CDCl3) δ 8.78 (s, 1H), 8.60 (d, J = 4.0 Hz, 1H), 7.89 (d, J = 7.9 Hz, 1H), 7.80 (d, J = 2.2 Hz, 1H), 7.77 (dd, J = 8.6, 2.2 Hz, 1H), 7.41 (dd, J = 7.7, 4.9 Hz, 1H), 7.03 (d, J = 8.7 Hz, 1H), 4.75 (d, J = 12.9 Hz, 1H), 4.41–4.29 (m, 2H), 3.88 (s, 3H), 3.24 (t, J = 12.7 Hz, 1H), 2.72 (apparent t, J = 12.4 Hz, 1H), 2.14–1.74 (m, 7H), 1.50 (s, 6H), 1.05–0.95 (m, 2H), 0.77 (dd, J = 7.9, 3.5 Hz, 2H). 13C NMR (126 MHz, CDCl3) δ 178.0, 171.9, 161.3, 157.8, 149.8, 148.0, 137.2, 133.7, 128.7, 127.6, 127.5, 124.0, 123.3, 111.2, 55.8, 50.7, 48.8, 44.7, 41.4, 30.8, 29.7, 22.8, 11.1, 7.5, 7.4. LC-MS (ESI) m/z found: 447 (M + H)+; retention time: 3.20 min. HRMS-ESI (M + H)+ calculated for C26H31N4O3: 447.2391, found: 447.2390.
Amine 41 (400 mg, 1.1 mmol) was dissolved in dioxane (15 ml) and 25 ml of saturated NaHCO3 was added followed by a dropwise addition of a 4-nitrophenyl chloroformate (639 mg, 3.2 mmol) in dioxane (10 ml) solution. The reaction mixture was stirred for 22 h, diluted with EtOAc and washed with a saturated sodium bicarbonate solution. The organic fraction was dried with brine, evaporated to dryness in the presence of SiO2 and purified over the same medium using 1:3 EtOAc:c-hexane with 1% TEA toward 100% EtOAc with 1% TEA. After confirming identify of the product by semi-crude NMR it was used in the following step. K2CO3 (25 mg, 0.18 mmol) and morpholine (0.016 ml, 0.18 mmol) were dissolved in DMF (5 ml) to which the earlier isolated intermediate (50 mg, 0.092 mmol) was added. This reaction mixture was stirred at room temperature for 4 days and an additional equivalent morpholine (0.016 ml, 0.18 mmol) was added after 2 days. The reaction mixture was diluted with EtOAc (25 ml) and washed with sat. aq. Na2CO3 (25 ml). The aqueous layer was back extracted with EtOAc (25 ml) and the combined organic layers were washed with brine (25 ml), dried over Na2SO4 and concentrated in vacuo. The remaining crude was coated on SiO2 and purified over SiO2 using a gradient from EtOAc (1% TEA): cyclohexane 1:1 (v/v) toward 1% MeOH in EtOAc (1% TEA), evaporation of volatiles yielded a colourless oil which was re-dissolved in DCM and concentrated to afford 19 mg (0.039 mmol, 43%). 1H NMR (600 MHz, CDCl3) δ 8.83–8.78 (m, 1H), 8.62–8.59 (m, 1H), 8.04–7.98 (m, 1H), 7.82 (d, J = 2.2 Hz, 1H), 7.77 (dd, J = 8.7, 2.2 Hz, 1H), 7.49 (dd, J = 7.8, 5.1 Hz, 1H), 7.03 (d, J = 8.7 Hz, 1H), 4.29–4.23 (m, 1H), 3.87 (s, 3H), 3.85–3.80 (m, 2H), 3.70–3.63 (m, 4H), 3.31–3.25 (m, 4H), 2.94–2.83 (m, 2H), 2.10–2.02 (m, 2H), 1.85–1.78 (m, 2H), 1.49 (s, 6H). 13C NMR (151 MHz, CDCl3) δ 178.0, 163.9, 161.1, 157.8, 148.5, 146.5, 138.5, 134.4, 128.7, 128.0, 126.9, 124.2, 123.7, 111.2, 66.7, 55.8, 51.0, 48.8, 47.4, 46.1, 30.0, 22.7. LC-MS (ESI) m/z found: 492 (M + H)+; retention time: 3.07 min. HRMS-ESI (M + H)+ calculated for C27H34N5O4+: 492.2605, found 492.2615.
The original contributions presented in the study are included in the article/Supplementary Material, further inquiries can be directed to the corresponding author.
MS contributed to the synthesis of the molecules, LM and GC did the parasitological screenings, MS, GS, IE, and RL were involved in the design of the molecules, LM, IE, and RL were responsible for obtaining the required funding, the manuscript was written by MS, GS, and RL and all authors made corrections and gave feedback to the manuscript.
The PDE4NPD project was funded by the European Union under the FP-7-Health program, project ID: 602,666.
The authors declare that the research was conducted in the absence of any commercial or financial relationships that could be construed as a potential conflict of interest.
Authors would like to thank D. Ahrens, A. Chen, M. Roseboom, J. Teeken H. Custers for technical assistance and M. Wijtmans for valuable advice.
ACN acetonitrile
AcOH acetic acid
BINAP 2,2′-bis(diphenylphosphino)-1.,1′-binaphthyl
bs broad singlet
d days
d doublet
DBN 1,5-Diazabicyclo[4.3.0]non-5-ene
DCE dichlorethane
DCM dichloromethane
dd double doublet
DIPEA N,N-diisopropylethylamine
DMAP 4-dimethylaminopyridine
DME dimethoxyethane
DMF dimethylformamide
DMSO dimethylsulfoxide
dt double triplet
DTU discrete typing unit
EDCI 1-Ethyl-3-(3-dimethylaminopropyl)carbodiimide
ESI electron spray ionization
EtOAc ethylacetate
EtOH ethanol
FCS fetal calf serum
h heptet hour
h heptet hour
HOBt hydroxybenzotriazole
HPLC high pressure liquid chromatography
HRMS high resolution mass spectroscopy
Hz Hertz
i-PrOH isopropanol
LC-MS liquid chromatography mass spectroscopy
m minute
m multiplet
MeOH methanol
MRC-5 medical research council strain 5
MTBE methyl tertbutyl ether
NaOtBu sodium tert-butoxide
o.n. overnight
p pentet
PDE4NPD phosphodiesterase inhibitors for neglected parasitic disease
pIC50 -log of the value at which 50% of growth is inhibited
rt room temperature
s singlet
SAR structure activity relationship
t triplet
T3P propylphosphonic anhydride
Tcr Trypanosoma cruzi
TEA triethylamine
q quartet
THF tetrahydrofuran
TLC thin layer chromatography
UV ultraviolet
Alpern, J. D., Lopez-Velez, R., and Stauffer, W. M. (2017). Access to Benznidazole for Chagas Disease in the United States—Cautious Optimism? PLoS Negl. Trop. Dis. 11, e0005794. doi:10.1371/journal.pntd.0005794
Basore, K., Cheng, Y., Kushwaha, A. K., Nguyen, S. T., and Desai, S. A. (2015). How Do Antimalarial Drugs Reach Their Intracellular Targets? Front. Pharmacol. 6. doi:10.3389/fphar.2015.00091
Batista, D. d. G. J., Batista, M. M., Oliveira, G. M. d., Amaral, P. B. d., Lannes-Vieira, J., Britto, C. C., et al. (2010). Arylimidamide DB766, a Potential Chemotherapeutic Candidate for Chagas’ Disease Treatment. Antimicrob. Agents Chemother. 54, 2940–2952. doi:10.1128/aac.01617-09
Bennion, B. J., Be, N. A., McNerney, M. W., Lao, V., Carlson, E. M., Valdez, C. A., et al. (2017). Predicting a Drug’s Membrane Permeability: A Computational Model Validated with In Vitro Permeability Assay Data. J. Phys. Chem. B 121, 5228–5237. doi:10.1021/acs.jpcb.7b02914
Bern, C. (2011). Antitrypanosomal Therapy for Chronic Chagas' Disease. N. Engl. J. Med. 364, 2527–2534. doi:10.1056/nejmct1014204
Blaazer, A. R., Orrling, K. M., Shanmugham, A., Jansen, C., Maes, L., Edink, E., et al. (2014). Fragment-Based Screening in Tandem with Phenotypic Screening Provides Novel Antiparasitic Hits. J. Biomol. Screen. 20, 10. doi:10.1177/1087057114549735
Castro, J. A., deMecca, M. M., and Bartel, L. C. (2006). Toxic Side Effects of Drugs Used to Treat Chagas' Disease (American Trypanosomiasis). Hum. Exp. Toxicol. 25, 471–479. doi:10.1191/0960327106het653oa
Coura, J. R., and Borges-Pereira, J. (2011). Chronic Phase of Chagas Disease: Why Should it Be Treated? A Comprehensive Review. Mem. Inst. Oswaldo Cruz 106, 641–645. doi:10.1590/s0074-02762011000600001
Coura, J. R., and Viñas, P. A. (2010). Chagas Disease: a New Worldwide Challenge. Nature 465, S6–S7. doi:10.1038/nature09221
Dias, J. C. P. (1984). Acute Chagas’ Disease. Mem. Inst. Oswaldo Cruz 79, 85–91. doi:10.1590/s0074-02761984000500017
Hamers, R. L., Gool, T. V., Goorhuis, A., Cordeiro, M. A. S., Urbina, J. A., Gascon, J., et al. (2016). Benznidazole for Chronic Chagas' Cardiomyopathy. N. Engl. J. Med. 374, 189–190. doi:10.1056/NEJMc1514453
Kollien, A., and Schaub, G. (2000). The Development of Trypanosoma Cruzi in Triatominae. Parasitol. Today 16, 381–387. doi:10.1016/s0169-4758(00)01724-5
Lidani, K. C. F., Andrade, F. A., Bavia, L., Damasceno, F. S., Beltrame, M. H., Messias-Reason, I. J., et al. (2019). Chagas Disease: From Discovery to a Worldwide Health Problem. Front. Public Health 7, 166. doi:10.3389/fpubh.2019.00166
Martinez, F., Perna, E., Perrone, S. V., and Liprandi, A. S. (2019). Chagas Disease and Heart Failure: An Expanding Issue Worldwide. Eur. Cardiol. 14, 82–88. doi:10.15420/ecr.2018.30.2
Meirelles, M. N., de Araujo-Jorge, T. C., Miranda, C. F., de Souza, W., and Barbosa, H. S. (1986). Interaction of Trypanosoma Cruzi with Heart Muscle Cells: Ultrastructural and Cytochemical Analysis of Endocytic Vacuole Formation and Effect upon Myogenesis In Vitro. Eur. J. Cel Biol 41, 198–206.
Mejia, A. M., Hall, B. S., Taylor, M. C., Gómez-Palacio, A., Wilkinson, S. R., Triana-Chávez, O., et al. (2012). Benznidazole-Resistance in Trypanosoma Cruzi Is a Readily Acquired Trait that Can Arise Independently in a Single Population. J. Infect. Dis. 206, 220–228. doi:10.1093/infdis/jis331
Morillo, C. A., Marin-Neto, J. A., Avezum, A., Sosa-Estani, S., Rassi, A., Rosas, F., et al. (2015). Randomized Trial of Benznidazole for Chronic Chagas’ Cardiomyopathy. N. Engl. J. Med. 373, 1295–1306. doi:10.1056/nejmoa1507574
Pinazo, M.-J., Guerrero, L., Posada, E., Rodríguez, E., Soy, D., and Gascon, J. (2013). Benznidazole-Related Adverse Drug Reactions and Their Relationship to Serum Drug Concentrations in Patients with Chronic Chagas Disease. Antimicrob. Agents Chemother. 57, 390–395. doi:10.1128/aac.01401-12
Prata, A. (2001). Clinical and Epidemiological Aspects of Chagas Disease. Lancet Infect. Dis. 1, 92–100. doi:10.1016/s1473-3099(01)00065-2
Rassi, A., Rassi, A., and Marin-Neto, J. A. (2010). Chagas Disease. The Lancet 375, 1388–1402. doi:10.1016/s0140-6736(10)60061-x
Schmunis, G. A. (1999). Prevention of Transfusional Trypanosoma Cruzi Infection in Latin America. Mem. Inst. Oswaldo Cruz 94, 93–101. doi:10.1590/s0074-02761999000700010
Sgambatti de Andrade, A. L. S., Zicker, F., de Oliveira, R. M., Almeida e Silva, S., Luquetti, A., Travassos, L. R., et al. (1996). Randomised Trial of Efficacy of Benznidazole in Treatment of Early Trypanosoma Cruzi Infection. Lancet 348, 1407–1413. doi:10.1016/s0140-6736(96)04128-1
Sijm, M., Siciliano de Araújo, J., Kunz, S., Schroeder, S., Edink, E., Orrling, K. M., et al. (2019). Phenyldihydropyrazolones as Novel Lead Compounds against Trypanosoma Cruzi. ACS Omega 4, 6585–6596. doi:10.1021/acsomega.8b02847
Sijm, M., Sterk, G. J., Caljon, G., Maes, L., Esch, I. J. P., and Leurs, R. (2020). Structure‐Activity Relationship of Phenylpyrazolones against Trypanosoma Cruzi. ChemMedChem 15, 1310–1321. doi:10.1002/cmdc.202000136
Torrico, F., Carlier, Y., Truyens, C., Suarez, E., Dramaix, M., Alonso-vega, C., et al. (2004). Maternal Trypanosoma Cruzi Infection, Pregnancy Outcome, Morbidity, and Mortality of Congenitally Infected and Non-infected Newborns in Bolivia. Am. J. Trop. Med. Hyg. 70, 201–209. doi:10.4269/ajtmh.2004.70.201
WHO (2021). Chagas Disease (American Trypanosomiasis). Available at: http://www.who.int/mediacentre/factsheets/fs340/en/ (Accessed November 05, 2018).
Keywords: structure activity relationship, chagas disease, phenotypic optmization, trypanosama cruzi, phenylpyrazolones
Citation: Sijm M, Maes L, de Esch IJP, Caljon G, Sterk GJ and Leurs R (2021) Structure Activity Relationship of N-Substituted Phenyldihydropyrazolones Against Trypanosoma cruzi Amastigotes. Front. Chem. 9:608438. doi: 10.3389/fchem.2021.608438
Received: 20 September 2020; Accepted: 16 April 2021;
Published: 30 April 2021.
Edited by:
Gildardo Rivera, Instituto Politécnico Nacional (IPN), MexicoReviewed by:
Gabriella Gabriella, University of Florence, ItalyCopyright © 2021 Sijm, Maes, de Esch, Caljon, Sterk and Leurs. This is an open-access article distributed under the terms of the Creative Commons Attribution License (CC BY). The use, distribution or reproduction in other forums is permitted, provided the original author(s) and the copyright owner(s) are credited and that the original publication in this journal is cited, in accordance with accepted academic practice. No use, distribution or reproduction is permitted which does not comply with these terms.
*Correspondence: Rob Leurs, ci5sZXVyc0B2dS5ubA==
Disclaimer: All claims expressed in this article are solely those of the authors and do not necessarily represent those of their affiliated organizations, or those of the publisher, the editors and the reviewers. Any product that may be evaluated in this article or claim that may be made by its manufacturer is not guaranteed or endorsed by the publisher.
Research integrity at Frontiers
Learn more about the work of our research integrity team to safeguard the quality of each article we publish.