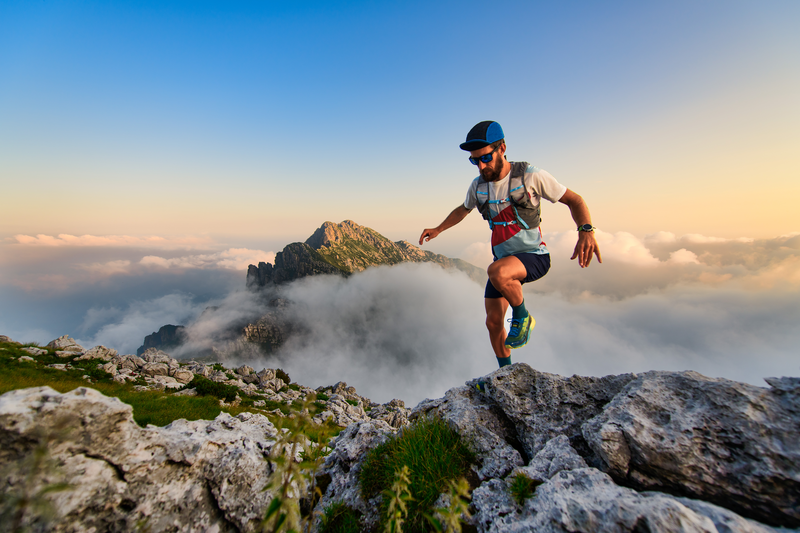
95% of researchers rate our articles as excellent or good
Learn more about the work of our research integrity team to safeguard the quality of each article we publish.
Find out more
MINI REVIEW article
Front. Chem. , 20 November 2020
Sec. Organic Chemistry
Volume 8 - 2020 | https://doi.org/10.3389/fchem.2020.599026
This article is part of the Research Topic New Hypervalent Iodine Reagents for Oxidative Coupling View all 10 articles
In recent years, the chemistry of heteroaryliodonium(III) salts has undergone significant developments. Heteroaryliodonium(III) salts have been found to be useful synthetic tools for the transfer of heteroaryl groups under metal-catalyzed and metal-free conditions for the preparation of functionalized heteroarene-containing compounds. Synthetic transformations mediated by these heteroaryliodonium(III) salts are classified into two categories: (1) reactions utilizing the high reactivity of the hypervalent iodine(III) species, and (2) reactions based on unique and new reactivities not observed in other types of conventional diaryliodonium salts. The latter feature is of particular interest and so has been intensively investigated in recent decades. This mini-review therefore aims to summarize the recent synthetic applications of heteroaryliodonium(III) salts as highly reactive electrophiles.
Over the past few decades, the hypervalent iodine(III) reagent has been widely recognized as a versatile oxidant for performing environmentally friendly oxidation reactions. It has gained great importance as a promising alternative to toxic heavy metal oxidants, such as Pb(IV), Tl(III), and Hg(II) because of its low toxicity, high stability, and easy handling (Stang and Zhdankin, 1996; Zhdankin and Stang, 2002, 2008; Zheng et al., 2014; Yoshimura and Zhdankin, 2016). Diaryliodonium salts (Ar1Ar2I+X−), which have two aryl groups and one counter-anion on the trivalent iodine atom, represent one of the most useful classes of hypervalent iodine(III) compounds (Merritt and Olofsson, 2009; Yusubov et al., 2011; Aradi et al., 2016; Fañanás-Mastral, 2017; Stuart, 2017). Due to the highly electron-deficient nature of the iodine(III) center in diaryliodonium salts and the excellent leaving-group ability of the iodobenzene group, they serve as highly reactive arylating agents toward a wide range of nucleophiles, even under metal-free conditions. Although the chemistry of conventional diaryliodonium salts is well developed and widely applied, synthetic transformations using heteroaryliodonium salts have received little attention to date.
In early studies, symmetric diaryliodonium salts (Ar1Ar2I+X−; Ar1 = Ar2) were preferred over unsymmetric salts (Ar1Ar2I+X−; Ar1 ≠ Ar2) to avoid selectivity issues during the aryl-transfer processes. Meanwhile, the introduction of a “dummy aryl” into asymmetric salts to act as a nontransferable group has been favored in recent studies to modulate and control the reactivities of the diaryliodonioum salts. On the other hand, the reactions of heteroaryliodonium salts have some limitations in the past; for example, the heteroaryl group present in phenyl(heteroaryl)iodonium salts tends to serve as a “dummy aryl,” and it was not possible to transfer the heteroaryl group during the reactions (Martín-Santamaría et al., 2000). However, during the last decade or so, quite interesting and highly valuable transformations utilizing aryl(heteroaryl)iodonium salts have been reported. Thus, this mini-review highlights the representative examples in both metal-catalyzed and metal-free reactions based on the currently available literature.
The conventional synthetic routes to heteroaryliodonium salts rely on stepwise processes using a series of organometallic nucleophiles instead of heteroarenes themselves, as outlined in Figures 1A–D. One general method for the preparation of heteroaryliodonium salts is based on the iodonium(III)-atom transfer of iodine(III) cyanides to heteroarylstannanes (Figure 1A). More specifically, (dicyano)iodonium(III) triflate [(NC)2I+−OTf] 1, generated in situ from iodosyl triflate and trimethylsilyl cyanide (TMSCN), reacts with tributyltin precursors 2 derived from furans, thiophenes, and pyrazoles, to afford the corresponding symmetrical bis(heteroaryl)iodonium triflates 3a–3d (Figure 1A; Stang et al., 1992a,b). Aryl(cyano)iodonium(III) triflates (ArI+CN−OTf) 4 could also be used as an iodine transfer agent, reacting with stannylated derivatives 5 and 6 to afford a variety of mixed thienyl(aryl)iodonium salts 7 and 8, respectively (Figure 1A; Gallop et al., 1993; Zhdankin et al., 1993; Bykowski et al., 2002).
Figure 1. Synthesis of heteroaryliodonium salts. (A) Stannyl heteroarenes. (B) Lithio heteroarenes. (C) Boryl heteroarenes. (D) Silyl heteroarenes. (E) Direct synthesis. (F) One-pot synthesis from iodoarenes.
The iodonium(III)-transfer procedure using trans-(chlorovinyl)iodonium dichloride 9 (Beringer and Nathan, 1969) was reported to access the bis(heteroaryl)iodonium chlorides 11 by the reaction of lithiated nitrogen-containing heteroarenes 10 (Figure 1B; Stang and Chen, 1995; Stang et al., 1995). Thus, the prepared bis(heteroaryl)iodonium chlorides 11 could be converted to the corresponding triflates 12 by treatment with trimethylsilyl triflate (TMSOTf). For the preparation of unsymmetrical heteroaryl(phenyl)iodonium triflate 15, the ligand-transfer reaction between vinyliodonium salt 13 with heteroaryllithium 14 was developed by Kitamura et al. (Figure 1B; Kitamura et al., 1996).
Another approach to unsymmetrical heteroaryl(phenyl)iodonium salts is the combination of readily available heteroarylboronic acids 16 with hydroxy(tosyloxy)iodobenzene (PhI(OH)OTs; Koser's reagent), which is useful for the mild and regioselective preparation of tosylate salts 17 (Figure 1C; Carroll et al., 2000).
Silylated heteroarenes can also be utilized for the preparation of heteroaryliodonium salts; phenyliodonium triflate 19 was prepared from bis(trimethylsilyl)thiophene 18 by the reaction with phenyliodine diacetate [PhI(OAc)2; PIDA] and triflic acid (Ye et al., 1996), while pyrrolyliodonium triflate 21 was obtained by the reaction of bis(trimethylsilyl)pyrroles 20 with iodosobenzene (PhIO) in the presence of BF3•Et2O (Figure 1D; Liu et al., 1999).
Straightforward approaches for the direct/one-pot preparation of heteroaryliodonium salts from heteroarenes have been elaborated as shown in Figures 1E,F, which allow expansion of the substrate scope to provide diverse types of heteroaryliodonium salts. In contrast to the aforementioned iodonium(III) salts containing fundamental heteroarene units, such as thiophene, pyridine, and furan, iodonium(III) salts bearing nucleobase and nucleoside moieties did not appear in the literature until 1998. Kim et al. reported the preparation of phenyliodonium(III)-substituted uracil nucleosides 23 by the reaction of uracil nucleosides 22 with PIDA in the presence of triflic acid, and its subsequent application in palladium-catalyzed alkenylation reaction (Figure 1E; Roh et al., 1998, 1999). Utilizing the unique character of the fluoroalcohol medium as a solvent, Kita et al. developed a facile synthesis of heteroaryliodonium salts 24 and 25 through the condensation of thiophenes and pyrroles with an iodine(III) reagent, whereby trifluoroethanol (TFE) enhanced the efficiency of this type of condensation, significantly extending the product scope (Dohi et al., 2007, 2010; Ito et al., 2010). Furthermore, this procedure could also be applied to the preparation of uracil-aryliodonium salts 26 possessing different types of aryl moieties and various counterions (Takenaga et al., 2018, 2019a).
Moreover, Olofsson et al. developed an efficient one-pot synthesis to heteroaryliodonium triflates 27 and 28 from arenes and aryl iodides using m-chloroperbenzoic acid (mCPBA) as a stoichiometric oxidant in the presence of triflic acid. In a seminal report, heteroaryliodonium tosylates could be prepared using p-toluenesulfonic acid instead of triflic acid as an additive (Figure 1F; Bielawski and Olofsson, 2007; Bielawski et al., 2007, 2014). Symmetrical di(heteroaryl)iodonium salt 29 was synthesized via a modified one-pot procedure employing elemental iodine, arenes, mCPBA, and toluenesulfonic acid under similar conditions (Figure 1F; Zhu et al., 2008). The one-pot procedure was also applied to the preparation of uracil-aryliodonium salts 30 bearing various aryl moieties (Toh et al., 2013; Modha and Greaney, 2015). As a result, recent progress in the efficient synthesis of diaryliodonium(III) salts has enabled the facile preparation of diverse types of heteroaryliodonium salts.
Various organic synthetic transformations utilizing diaryliodonium salts have emerged over the past few decades, and this research field includes diverse metal-catalyzed and metal-free arylations for a wide range of nucleophiles, in addition to benzyne generation and the dearomatization of phenols. In addition to these reactions, new synthetic applications of heteroaryliodonium salts have been investigated in recent years. Herein, we outline the strategies based on the use of heteroaryliodonium(III) salts as electrophilic aryl transfer reagents for the C-O, C-N, and C-C bond formations. The synthetic transformations of heteroaryliodonium salts are classified into the following two categories: (1) reactions utilizing the high reactivities of iodonium(III) salts, and (2) reactions based on the unique and new reactivities of heteroaryliodonium salts. The former category of transformation has been applied to C-O and C-N bond formation reactions (Figures 2A,B), while the latter has been employed in C-C bond formation processes (Figure 2C).
Figure 2. New synthetic application of heteroaryliodonium salts. (A) C-O bond formation [metal-free: (a,b), metal-catalyzed: (c,d)]. (B) C-N bond formation [metal-catalyzed: (e,f), metal-free: (g)]. (C) C-C bond formation [metal-free: (h,i), metal-catalyzed: (j), aryne analog precursor: (k)].
Over the past few decades, symmetrical heteroaryliodonium salts (Ar1Ar2I+X−; Ar1 = Ar2) have found numerous applications as electrophilic arylating agents for nucleophiles. In contrast, unsymmetrical salts (Ar1Ar2I+X−; Ar1 ≠ Ar2) have been less frequently employed since the presence of two different aromatic moieties in the salts could potentially give mixtures of two arylated products (i.e., Ar1-Nu and Ar2-Nu). Recently however, by the introduction of a nontransferable dummy group, unsymmetrical iodonium salts participate in a wide range of transformations. The representative examples of dummy groups are p-anisyl (Ma et al., 2015; Xiong et al., 2015), mesityl (Matsuzaki et al., 2015; Sundalam and Stuart, 2015), and 2,4,6-trimethoxyphenyl (TMP) (Malmgren et al., 2013; Seidl et al., 2016) groups, and these dummy groups were utilized in reactions of the conventional diaryliodonium salts.
Selected examples of C-O bond formation reactions by the electrophilic arylation of phenols using heteroaryliodonium salts are shown in Figure 2A, Equation a–c. In such cases, phenols were efficiently arylated to afford industrially important aryl(heteroaryl) ether compounds. In 2012, Olofsson et al. studied the metal-free arylation of phenols under basic conditions. They confirmed the chemoselective reactivity pattern of unsymmetrical diaryliodonium salts (Jalalian et al., 2012; Bielawski et al., 2014) (Figure 2A, Equation a). It was revealed that the electronic effect of the aryl rings was dominant during the reaction of p-anisyl (pyridyl) triflate 31 with phenols 32; the phenol nucleophiles selectively coupled to the electron-deficient pyridyl group to yield pyridyl ether 33 in 65–88% yield. This chemoselectivity trend has been further applied by other researchers to transfer the electron-poor aryl group of unsymmetrical iodonium salts, and it was noted that the TMP group serves as an improved dummy ligand for aryl transfer processes from heteroaryl(TMP)iodonium salts (Seidl et al., 2016; Dohi et al., 2017). In 2020, Stuart et al. reported a convenient method for phenol arylation using the in-situ-generated aryl(TMP)iodonium salts 34 (Gallagher et al., 2020) (Figure 2A, Equation b). Thus, in metal-free reactions, nucleophiles would preferentially react with the more electron-deficient aromatic ring out of the two aryl moieties in unsymmetrical diaryliodonium salts. The results are however different in case of metal-catalyzed reactions. Suna et al. demonstrated that the presence of a Cu(I) catalyst directed the selectivity of the arylation to the more electron-rich heteroarene moiety during the reaction between heteroaryliodonium salts 35 and phenols (Sokolovs and Suna, 2016) (Figure 2A, Equation c). This Cu(I)-catalyzed synthesis of diaryl ethers using diaryliodonium salts is a complementary strategy to the previously mentioned metal-free arylation of phenols. The arylation of oxygen nucleophiles using heteroaryliodonium salts was not limited to the preparation of aryl(heteroaryl) ethers; as in the cases of other oxygen nucleophiles, it was demonstrated that benzoic acid was also arylated to produce quinolin-3-yl benzoate via the in-situ-generated iodonium salt bearing a 3-quinolyl group (Dohi et al., 2017). Further, the chemistry of cyclic iodonium salts has recently experienced growing development (Chatterjee and Goswami, 2017). As for just an emerging interesting compound, Nachtsheim et al. investigated that the direct oxidative cyclization of 3-(2-iodophenyl)-1H-pyrazole to novel iodolopyrazolium salt 36 via in-situ formed N-heterocyclic stabilized hydroxy- λ3-iodane (Boelke et al., 2020) (Figure 2A, Equation d). They also demonstrated that the copper-catalyzed ring opening of 36 with acetate followed by hydrolysis produced functionalized heteroaromatic biaryl 37 in 70% yield.
Various heteroaryliodonium salt-mediated C-N bond formation processes are summarized in Figure 2B; these reactions include the azidation, amination, and nitration of heteroarenes. In 2012, Suna et al. reported the Cu(I)-catalyzed transformation of C–H to C–N in electron-rich heteroaromatics (i.e., pyrroles, pyrrolopyridines, thienopyrroles, pyrrolopyrimidines, and uracil), which involves the reaction of in-situ prepared heteroaryl(phenyl)iodonium tosylates 38 with sodium azide, whereby complete regiocontrol is accomplished (Lubriks et al., 2012) (Figure 2B, Equation e). Based on this study, they further demonstrated the C–H amination of electron-rich heteroarenes, comprising the in-situ formation of heteroaryliodonium salts 39 bearing sterically hindered mesityl (Mes) or triisopropylphenyl (TIPP) group (Sokolovs et al., 2014; Berzina et al., 2015) (Figure 2B, Equation f). The electronic effects observed for the aryl transfer are consistent with those mentioned earlier for O-nucleophiles. Thus, the electron-rich heteroarene moieties of these salts were transferred in Cu(I)-catalyzed arylations, while N-nucleophiles were preferentially coupled with the electron-deficient heteroaromatic ring under metal-free conditions. Sodium nitrite was recently used as a nucleophile for diaryliodonium salts as a convenient approach to access synthetically useful nitroarenes. In 2016, Olofsson et al. reported the metal-free nitration of p-anisyl(pyridyl) salt 40 to afford nitropyridine 41 (Reitti et al., 2016) (Figure 2B, Equation g). Other metal-free reactions with N-nucleophiles include application of the TMP group as an extremely electron-rich aryl dummy ligand to transfer electron-deficient pyridyl groups to alicyclic amines or the azide ion (Sandtorv and Stuart, 2016; Seidl and Stuart, 2017).
Figure 2C lists the versatile C-C bond forming reaction based on the use of heteroaryliodonium salts as aryl sources and reaction intermediates. For example, in 2009, Kita et al. demonstrated the metal-free oxidative cross-coupling of heteroarenes with electron-rich arenes by direct C–H transformations. The reaction proceeded through the in-situ formation of thienyliodonium salt 42 from an electron-rich thiopene and Koser's reagent in hexafluoroisopropanol (HFIP). It was proposed that the intermediate thienyliodonium species was activated by the addition of trimethylsilyl bromide, followed by its coupling with electron-rich arenes to provide a wide range of useful mixed biaryl products 43 (Kita et al., 2009, 2011; Morimoto et al., 2010, 2011; Dohi et al., 2013) (Figure 2C, Equation h). In 2013, Gaunt et al. developed a new acyl coupling reaction that merges the aryl transfer ability of diaryliodonium salts with carbonyl umpolung using an N-heterocyclic carbene (NHC) catalyst 44. They also established the transfer of a pyridyl group from several pyridyliodonium salts to provide di(hetero)aryl ketones 46, and exclusive pyridyl transfer was observed when a uracil-derived salt 45 was used. This is an interesting example of utilization of the uracil moiety as a nontransferable dummy group for the organocatalytic reactions of diaryliodoniuim(III) salts, with more frequently used dummy groups for arylations being the Mes, TMP, and TIPP groups (Toh et al., 2013) (Figure 2C, Equation i). In general, arylation using diaryiodonium salts produces one equivalent of iodoarene as a waste byproduct, which is problematic in the context of atom economy. Thus, Greaney et al. reported the very atom-economical transformation of heteroaryliodonium salts (Modha and Greaney, 2015) (Figure 2C, Equation j). They showed that uracil-iodonium salts 47 could undergo Cu-catalyzed tandem C-H/N-H arylation reactions to produce double-arylated indoles 48 by incorporation of both the uracil and aryl groups. As another copper-catalyzed tandem reaction, Fañanás-Mastral et al. described an interesting example, carboarylation/cyclization of alkynyl phosphonate with a mesityl thienyl iodonium salt (Pérez-Saavedra et al., 2017). The recent report by Takenaga et al. highlighted the synthesis of bicyclic uracil systems and the vicinal functionalization of uracils with the basic activation of salts 49 being stabilized by the o-trifluorophenyl group. The reactive intermediate of this reaction is believed to involve the generation of a highly strained heterocyclic alkyne species, uracilyne 50, a non-reported heteroaryne analog (Takenaga et al., 2019a,b) (Figure 2C, Equation k).
In the twentieth century, the synthetic scope of heteroaryliodonium(III) salts was limited, and thus only relatively simple heteroaryliodonium salts, such as symmetrical bis(heteroaryl)iodonium salts and unsymmetrical phenyl(heteroaryl)iodonium salts, were previously reported. With recent advances in efficient synthetic procedures, heteroaryliodonium salts bearing sterically hindered mesityl, trimethoxyphenyl, and triisopropylphenyl groups were efficiently synthesized. These designer heteroaryliodonium salts have been found to control the chemoselectivity of aryl transfer in a wide range of reactions. The metal-free and metal-catalyzed reactions show significant selectivity toward aryl/heteroaryl group transfer and unearth an important synthetic methodology for selective synthetic purposes. The synthetic utilities of heteroaryliodonium salts highlighted in this mini-review, such as NHC-catalyzed C-H bond arylation, Cu-catalyzed tandem arylation of indoles, and vicinal functionalization, undoubtedly pave a useful pathway in this direction, and developments of new synthetic transformations in this area are highly demanded in future.
NT and TD conceived and wrote the manuscript. All authors provided comments, discussed the manuscript, and approved it for publication.
This work was supported by JSPS KAKENHI Grant Number JP20K06980. TD acknowledges support from JSPS KAKENHI Grant Number JP19K05466 and the Ritsumeikan Global Innovation Research Organization (R-GIRO) project.
The authors declare that the research was conducted in the absence of any commercial or financial relationships that could be construed as a potential conflict of interest.
Aradi, K., Tóth, B., Tolnai, G., and Novák, Z. (2016). Diaryliodonium salts in organic syntheses: a useful compound class for novel arylation strategies. Synlett 27, 1456–1485. doi: 10.1055/s-0035-1561369
Beringer, F. M., and Nathan, R. A. (1969). Diaryliodonium salts from aryllithium reagents with trans-chlorovinyliodoso dichloride. J. Org. Chem. 34, 685–689. doi: 10.1021/jo01255a044
Berzina, B., Sokolovs, I., and Suna, E. (2015). Copper-catalyzed para-selective C–H amination of electron-rich arenes. ACS Catal. 5, 7008–7014. doi: 10.1021/acscatal.5b01992
Bielawski, M., Malmgren, J., Pardo, L. M., Wikmark, Y., and Olofsson, B. (2014). One-pot synthesis and applications of N-heteroaryl iodonium salts. Chem. Open. 3, 19–22. doi: 10.1002/open.201300042
Bielawski, M., and Olofsson, B. (2007). High-yielding one-pot synthesis of diaryliodonium triflates from arenes and iodine or aryl iodides. Chem. Commun. 2521–2523. doi: 10.1039/b701864a
Bielawski, M., Zhu, M., and Olofsson, B. (2007). Efficient and general one-pot synthesis of diaryliodonium triflates: optimization, scope and limitations. Adv. Synth. Catal. 349, 2610–2618. doi: 10.1002/adsc.200700373
Boelke, A., Kuczmera, T. J., Caspers, L. D., Lork, E., and Nachtsheim, B. J. (2020). Iodolopyrazolium salts: synthesis, derivatizations, and applications. Org. Lett. 22, 7261–7266. doi: 10.1021/acs.orglett.0c02593
Bykowski, D., McDonald, R., Hinkle, R. J., and Tykwinski, R. R. (2002). Structural and electronic characteristics of thienyl(aryl)iodonium triflates. J. Org. Chem. 67, 2798–2804. doi: 10.1021/jo015910t
Carroll, M. A., Pike, V. W., and Widdowson, D. A. (2000). New synthesis of diaryliodonium sulfonates from arylboronic acids. Tetrahedron Lett. 41, 5393–5396. doi: 10.1016/S0040-4039(00)00861-3
Chatterjee, N., and Goswami, A. (2017). Synthesis and application of cyclic diaryliodonium salts: a platform for bifunctionalization in a single step. Eur. J. Org. Chem. 2017, 3023–3032. doi: 10.1002/ejoc.201601651
Dohi, T., Ito, M., Morimoto, K., Minamitsuji, Y., Takenaga, N., and Kita, Y. (2007). Versatile direct dehydrative approach for diaryliodonium(III) salts in fluoroalcohol media. Chem. Commun. 4152–4154. doi: 10.1039/b708802g
Dohi, T., Koseki, D., Sumida, K., Okada, K., Mizuno, S., Kato, A., et al. (2017). Metal-free O-arylation of carboxylic acid by active diaryliodonium(III) intermediates generated in situ from iodosoarenes. Adv. Synth. Catal. 359, 3503–3508. doi: 10.1002/adsc.201700843
Dohi, T., Yamaoka, N., and Kita, Y. (2010). Fluoroalcohols: versatile solvents in hypervalent iodine chemistry and syntheses of diaryliodonium(III) salts. Tetrahedron 66, 5775–5785. doi: 10.1016/j.tet.2010.04.116
Dohi, T., Yamaoka, N., Nakamura, S., Sumida, K., Morimoto, K., and Kita, Y. (2013). Efficient synthesis of a regioregular oligothiophene photovoltaic dye molecule, MK-2, and related compounds: a cooperative hypervalent iodine and metal-catalyzed synthetic route. Chem. Eur. J. 19, 2067–2075. doi: 10.1002/chem.201203503
Fañanás-Mastral, M. (2017). Copper-catalyzed arylation with diaryliodonium salts. Synthesis 49, 1905–1930. doi: 10.1055/s-0036-1589483
Gallagher, R. T., Basu, S., and Stuart, D. R. (2020). Trimethoxyphenyl (TMP) as a useful auxiliary for in situ formation and reaction of aryl(TMP)iodonium salts: synthesis of diaryl ethers. Adv. Synth. Catal. 362, 320–325. doi: 10.1002/adsc.201901187
Gallop, P. M., Paz, M. A., Fluckiger, R., Stang, P. J., Zhdankin, V. V., and Tykwinski, R. R. (1993). Highly effective PQQ inhibition by alkynyl and aryl mono- and diiodonium salts. J. Am. Chem. Soc. 115, 11702–11704. doi: 10.1021/ja00078a007
Ito, M., Ogawa, C., Yamaoka, N., Fujioka, H., Dohi, T., and Kita, Y. (2010). Enhanced reactivity of [hydroxy(tosyloxy)iodo]benzene in fluoroalcohol media. Efficient direct synthesis of thienyl(aryl)iodonium salts. Molecules 15, 1918–1931. doi: 10.3390/molecules15031918
Jalalian, N., Petersen, T. B., and Olofsson, B. (2012). Metal-free arylation of oxygen nucleophiles with diaryliodonium salts. Chem. Eur. J. 18, 14140–14149. doi: 10.1002/chem.201201645
Kita, Y., Dohi, T., and Morimoto, K. (2011). Hypervalent iodine induced metal-free C-H cross couplings to biaryls. J. Synth. Org. Chem. Jpn. 69, 1241–1250. doi: 10.5059/yukigoseikyokaishi.69.1241
Kita, Y., Morimoto, K., Ito, M., Ogawa, C., Goto, A., and Dohi, T. (2009). Metal-free oxidative cross-coupling of unfunctionalized aromatic compounds. J. Am. Chem. Soc. 131, 1668–1669. doi: 10.1021/ja808940n
Kitamura, T., Kotani, M., and Fujiwara, Y. (1996). An efficient ligand exchange reaction of β-(trifyloxy)vinyliodonium triflates with aryllithium reagents leading to diaryliodonium triflates. Tetrahedron Lett. 37, 3721–3722. doi: 10.1016/0040-4039(96)00668-5
Liu, J. H., Chan, H. W., Xue, F., Wang, Q. G., Mak, T. C., and Wong, H. N. (1999). Generation and Trapping reactions of 1-tert-butoxycarbonyl-3,4-didehydro-1H-pyrrole. J. Org. Chem. 64, 1630–1634. doi: 10.1021/jo982129l
Lubriks, D., Sokolovs, I., and Suna, E. (2012). Indirect C-H azidation of heterocycles via copper-catalyzed regioselective fragmentation of unsymmetrical λ3-iodanes. J. Am. Chem. Soc. 134, 15436–15442. doi: 10.1021/ja305574k
Ma, X. P., Shi, W. M., Mo, X. L., Li, X. H., Li, L. G., Pan, C. X., et al. (2015). Synthesis of alpha,beta-unsaturated N-aryl ketonitrones from oximes and diaryliodonium salts: observation of a metal-free N-arylation process. J. Org. Chem. 80, 10098–10107. doi: 10.1021/acs.joc.5b01716
Malmgren, J., Santoro, S., Jalalian, N., Himo, F., and Olofsson, B. (2013). Arylation with unsymmetrical diaryliodonium salts: a chemoselectivity study. Chem. Eur. J. 19, 10334–10342. doi: 10.1002/chem.201300860
Martín-Santamaría, S., Carroll, M. A., Carroll, C. M., Carter, C. D., Pike, V. W., Rzepa, H. S., et al. (2000). Fluoridation of heteroaromatic iodonium salts—experimental evidence supporting theoretical prediction of the selectivity of the process. Chem. Commun. 649–650. doi: 10.1039/b000868k
Matsuzaki, K., Okuyama, K., Tokunaga, E., Saito, N., Shiro, M., and Shibata, N. (2015). Synthesis of diaryliodonium salts having pentafluorosulfanylarenes and their application to electrophilic pentafluorosulfanylarylation of C-, O-, N-, and S-nucleophiles. Org. Lett. 17, 3038–3041. doi: 10.1021/acs.orglett.5b01323
Merritt, E. A., and Olofsson, B. (2009). Diaryliodonium salts: a journey from obscurity to fame. Angew. Chem. Int. Ed. 48, 9052–9070. doi: 10.1002/anie.200904689
Modha, S.G., and Greaney, M.F. (2015). Atom-economical transformation of diaryliodonium salts: tandem C-H and N-H arylation of indoles. J. Am. Chem. Soc. 137, 1416–1419. doi: 10.1021/ja5124754
Morimoto, K., Nakae, T., Yamaoka, N., Dohi, T., and Kita, Y. (2011). Metal-free oxidative coupling reactions via σ-iodonium intermediates: the efficient synthesis of bithiophenes using hypervalent iodine reagents. Eur. J. Org. Chem. 2011, 6326–6334. doi: 10.1002/ejoc.201100969
Morimoto, K., Yamaoka, N., Ogawa, C., Nakae, T., Fujioka, H., Dohi, T., et al. (2010). Metal-free regioselective oxidative biaryl coupling leading to head-to-tail bithiophenes: reactivity switching, a concept based on the iodonium(III) intermediate. Org. Lett. 12, 3804–3807. doi: 10.1021/ol101498r
Pérez-Saavedra, B., Vázquez-Galiñanes, N., Saá, C., and Fañanás-Mastral, M. (2017). Copper(I)-catalyzed tandem carboarylation/cyclization of alkynyl phosphonates with diaryliodonium salts. ACS Catal. 7, 6104–6109. doi: 10.1021/acscatal.7b02434
Reitti, M., Villo, P., and Olofsson, B. (2016). One-pot C-H functionalization of arenes by diaryliodonium salts. Angew. Chem. Int. Ed. 55, 8928–8932. doi: 10.1002/anie.201603175
Roh, K.R., Kim, J.Y., and Kim, Y.H. (1998). Novel synthesis of 5-phenyliodonium triflate substituted uracil nucleosides. Chem. Lett. 27, 1095–1096. doi: 10.1246/cl.1998.1095
Roh, K.R., Kim, J.Y., and Kim, Y.H. (1999). Palladium catalyzed alkenylation or alkynylation at C-5 of uracil nucleosides using novel phenyliodonium triflate. Tetrahedron Lett. 40, 1903–1906. doi: 10.1016/S0040-4039(98)02491-5
Sandtorv, A. H., and Stuart, D. R. (2016). Metal-free synthesis of aryl amines: beyond nucleophilic aromatic substitution. Angew. Chem. Int. Ed. 55, 15812–15815. doi: 10.1002/anie.201610086
Seidl, T. L., and Stuart, D. R. (2017). An admix approach to determine counter anion effects on metal-free arylation reactions with diaryliodonium salts. J. Org. Chem. 82, 11765–11771. doi: 10.1021/acs.joc.7b01599
Seidl, T. L., Sundalam, S. K., MiCullough, B., and Sruart, D. R. (2016). Unsymmetrical aryl(2,4,6-trimethoxyphenyl)iodonium salts: one-pot synthesis, scope, stability, and synthetic studies. J. Org. Chem. 81, 1998–2009. doi: 10.1021/acs.joc.5b02833
Sokolovs, I., Lubriks, D., and Suna, E. (2014). Copper-catalyzed intermolecular C-H amination of (hetero)arenes via transient unsymmetrical λ3-iodanes. J. Am. Chem. Soc. 136, 6920–6928. doi: 10.1021/ja502174d
Sokolovs, I., and Suna, E. (2016). Para-selective Cu-catalyzed C-H aryloxylation of electron-rich arenes and heteroarenes. J. Org. Chem. 81, 371–379. doi: 10.1021/acs.joc.5b02728
Stang, P. J., and Chen, K. (1995). Hybrid, iodonium-transition metal, cationic tetranuclear macrocyclic squares. J. Am. Chem. Soc. 117, 1667–1668. doi: 10.1021/ja00110a035
Stang, P. J., Olenyuk, B., and Chen, K. (1995). Preparation of nitrogen-containing bis(heteroaryl)iodonium salts. Synthesis 937–938. doi: 10.1055/s-1995-4023
Stang, P. J., Tykwinski, R., and Zhdankin, V. V. (1992a). Preparation of bis(heteroaryl)iodonium saltsviaan iodonium transfer reaction between di(cyano)iodonium triflate and organostannes. J. Heterocycl. Chem. 29, 815–818. doi: 10.1002/jhet.5570290424
Stang, P. J., and Zhdankin, V. V. (1996). Organic polyvalent iodine compounds. Chem. Rev. 96, 1123–1178. doi: 10.1021/cr940424+
Stang, P. J., Zhdankin, V. V., Tykwinski, R., and Zefirov, N. S. (1992b). (Dicyano)iodonium triflate- novel iodonium species and a versatile reagent for the preparation of iodonium salts via an iodonium transfer reaction with organostannanes. Tetrahedron Lett. 33, 1419–1422. doi: 10.1016/S0040-4039(00)91636-8
Stuart, D. R. (2017). Aryl transfer selectivity in metal-free reactions of unsymmetrical diaryliodonium salts. Chem. Eur. J. 23, 15852–15863. doi: 10.1002/chem.201702732
Sundalam, S. K., and Stuart, D. R. (2015). Base mediated synthesis of alkyl-aryl ethers from the reaction of aliphatic alcohols and unsymmetric diaryliodonium salts. J. Org. Chem. 80, 6456–6466. doi: 10.1021/acs.joc.5b00907
Takenaga, N., Hayashi, T., Ueda, S., Satake, H., Yamada, Y., Kodama, T., et al. (2019a). Synthesis of uracil-iodonium(III) salts for practical utilization as nucleobase synthetic modules. Molecules 24, 3034–3046. doi: 10.3390/molecules24173034
Takenaga, N., Ueda, S., Hayashi, T., Dohi, T., and Kitagaki, S. (2018). Facile synthesis of stable uracil-iodonium(III) salts with various counterions Heterocycles 97, 1248–1256. doi: 10.3987/COM-18-S(T)80
Takenaga, N., Ueda, S., Hayashi, T., Dohi, T., and Kitagaki, S. (2019b). Vicinal functionalization of uracil heterocycles with base activation of iodonium(III) salts. Heterocycles 99, 865–874. doi: 10.3987/COM-18-S(F)93
Toh, Q.Y., McNally, A., Vera, S., Erdmann, N., and Gaunt, M.J. (2013). Organocatalytic C-H bond arylation of aldehydes to bis-heteroaryl ketones. J. Am. Chem. Soc. 135, 3772–3775. doi: 10.1021/ja400051d
Xiong, W., Qi, C., Peng, Y., Guo, T., Zhang, M., and Jiang, H. (2015). Base-promoted coupling of carbon dioxide, amines, and diaryliodonium salts: a phosgene- and metal-free route to O-aryl carbamates. Chem. Eur. J. 21, 14314–14318. doi: 10.1002/chem.201502689
Ye, X.-S., Li, W.-K., and Wong, H. N. C. (1996). 3,4-Didehydrothiophene: generation, trappingreactions, andab initiostudy. J. Am. Chem. Soc. 118, 2511–2512. doi: 10.1021/ja953747b
Yoshimura, A., and Zhdankin, V. V. (2016). Advances in synthetic applications of hypervalent iodine compounds. Chem. Rev. 116, 3328–3435. doi: 10.1021/acs.chemrev.5b00547
Yusubov, M. S., Maskaev, A. V., and Zhdankin, V. V. (2011). Iodonium salts in organic synthesis. Arkivoc. 2011, 370–409. doi: 10.3998/ark.5550190.0012.107
Zhdankin, V. V., Scheuller, M. C., and Stang, P. J. (1993). A general approach to aryl(cyano)iodonium triflates - versatile iodonium transfer reagents. Tetrahedron. Lett. 34, 6853–6856. doi: 10.1016/S0040-4039(00)91812-4
Zhdankin, V. V., and Stang, P. J. (2002). Recent developments in the chemistry of polyvalent iodine compounds. Chem. Rev. 102, 2523–2584. doi: 10.1021/cr010003+
Zhdankin, V. V., and Stang, P. J. (2008). Chemistry of polyvalent iodine. Chem. Rev. 108, 5299–5358. doi: 10.1021/cr800332c
Zheng, Z., Zhang-Negrerie, D., Du, Y. F., and Zhao, K. (2014). The applications of hypervalent iodine(III) reagents in the construction of heterocyclic compounds through oxidative coupling reactions. Sci. China: Chem. 57, 189–214. doi: 10.1007/s11426-013-5043-1
Keywords: iodine, hypervalent compound, diaryliodonium(III) salt, aromatic substitution, arylation, heteroaromatic compound
Citation: Takenaga N, Kumar R and Dohi T (2020) Heteroaryliodonium(III) Salts as Highly Reactive Electrophiles. Front. Chem. 8:599026. doi: 10.3389/fchem.2020.599026
Received: 26 August 2020; Accepted: 16 October 2020;
Published: 20 November 2020.
Edited by:
Gwilherm Evano, Université Libre de Bruxelles, BelgiumReviewed by:
Martín Fañanás-Mastral, Universidad de Santiago de Compostela, SpainCopyright © 2020 Takenaga, Kumar and Dohi. This is an open-access article distributed under the terms of the Creative Commons Attribution License (CC BY). The use, distribution or reproduction in other forums is permitted, provided the original author(s) and the copyright owner(s) are credited and that the original publication in this journal is cited, in accordance with accepted academic practice. No use, distribution or reproduction is permitted which does not comply with these terms.
*Correspondence: Naoko Takenaga, bnRha2VuYWdAbWVpam8tdS5hYy5qcA==; Toshifumi Dohi, dGQxMjAzQHBoLnJpdHN1bWVpLmFjLmpw
Disclaimer: All claims expressed in this article are solely those of the authors and do not necessarily represent those of their affiliated organizations, or those of the publisher, the editors and the reviewers. Any product that may be evaluated in this article or claim that may be made by its manufacturer is not guaranteed or endorsed by the publisher.
Research integrity at Frontiers
Learn more about the work of our research integrity team to safeguard the quality of each article we publish.