- 1School of Environmental Science and Engineering, Huazhong University of Science and Technology, Wuhan, China
- 2School of Architecture & Urban Planning, Huazhong University of Science and Technology (HUST), Wuhan, China
- 3Center for the Environmental Implications of Nanotechnology (CEINT), Durham, NC, United States
- 4Hubei Provincial Engineering Research Center for Water Quality Safety and Pollution Control, Huazhong University of Science and Technology, Wuhan, China
- 5Key Laboratory of Water & Wastewater Treatment (MOHURD), Huazhong University of Science and Technology, Wuhan, China
UV/persulfate (UV/PS) could effectively degrade algal cells and micro-organic pollutants. This process was firstly applied to remove Microcystis aeruginosa (M. aeruginosa) and 2,4,6-trichlorophenol (TCP) simultaneously in bench scale. Algal cells can be efficiently removed after 120 min reaction accompanied with far quicker removal of the coexisted TCP, which could be totally removed within 5 min in the UV/PS process. Both and HO• were responsible for algal cells and TCP degradation, while and HO• separately dominated TCP degradation and algal cells removal. Apart from the role of radicals ( and HO•) for algal cells and TCP degradation, UV also played a role to some extent. Increased PS dose (0–4.5 mM) or UV intensity (2.71–7.82 mW/cm2) could enhance the performance of the UV/PS process in both TCP and algae removal. Although some intracellular organic matters can be released to the outside of algal cells due to the cell lysis, they can be further degraded by UV/PS process, which was inhibited by the presence of TCP. This study suggested the good potential of the UV/PS process in the simultaneous removal of algal cells and micro-organic pollutants.
Introduction
Cyanobacterial blooms in lakes, urban ponds, and reservoirs have been frequently reported in recent years (Wang and Wei, 2008; de la Cruz et al., 2017; Keith et al., 2018). Bloom-forming cyanobacterial taxa are harmful to the environment and human health via consuming oxygen in water and releasing algal organic matter (AOM), such as toxins and taste & odor substances (Wang et al., 2016; Chen et al., 2017a). Additionally, the proliferation of algal cells can interrupt the supply of drinking water systems such as clogging filters and increasing the usages of treatment agent (Paerl and Otten, 2013; Xie et al., 2016). Due to the electrostatic repulsion, surface hydrophilicity, and steric effects of algal cells (Chow et al., 1999; Teixeira and Rosa, 2006; Shen et al., 2011), the conventional drinking water systems posed limited efficiency on the cells removal, causing serious deterioration in water quality (Rajasekhar et al., 2012). It is known that the discharge of municipal, industrial and agricultural wastewater which contains large amounts of micro-organic pollutants (MPs) can result in the occurrence of eutrophication and algal blooms (Liu et al., 2012). Therefore, natural water may be contaminated by algae and MPs at the same time, and it is meaningful to develop treatment methods to simultaneously remove algal cells and MPs.
Advanced oxidation processes (AOPs) could effectively degrade numerous MPs due to the production of high amounts of reactive radicals such as hydroxyl and sulfate radicals (HO• and ) (Olmez-Hanci et al., 2015; Matzek and Carter, 2016). Due to the efficient destruction of algal cells by the formed reactive radicals, AOPs also perform well in removing algal cells (Wang et al., 2016; Wacławek et al., 2017; Chen et al., 2020). Consequently, the application of AOPs would simutaneously achieve good removal of algal cells and MPs in therory.
Among the reported AOPs, activation of persulfate (PS) by ultraviolet (UV) radiation can high-efficiently generate with a quantum yield of 1.4 mol E s−1 at 254 nm (Equation 1), accompanied with generating HO• via Equation (2) (Mark et al., 1990; Xie et al., 2015). Both HO• and are reactive radicals in the UV/PS process and account for the degradation of numerous MPs (Olmez-Hanci et al., 2015; Xie et al., 2015; Matzek and Carter, 2016). Furthermore, our previous studies have revealed that ultraviolet/persulfate (UV/PS) process is one of the most effective AOPs in the removal of algal cells and AOM (Wang et al., 2016; Chen et al., 2017a). So, the UV/PS process is expected to be a good choice in the simultaneous treatment of algal cells and MPs. Additionally, the released intracellular organic matter (IOM) during the treatment also shows high reactivity with reactive radicals with the second reaction rate constant between IOM and HO• (kIOM) reaching (4.02~7.95) × 108 Mc−1 s−1 (Lee et al., 2018), which would also scavenge the formed reactive radicals in the UV/PS process. Therefore, from a practical point of view, comprehensively investigating the treatability of co-existed algal cells and MPs by UV/PS would be of interest.
In this study, the UV/PS was firstly applied in removing algal cells and MPs simultaneously. The objective of this study is to evaluate the UV/PS performance on the removal of algae and MPs simultaneously by choosing the widely detective Microcystis aeruginosa (M. aeruginosa) and 2,4,6-trichlorophenol (TCP) as examples of typical algal cells and MP, respectively. The removal efficiencies of algal cells and TCP under different conditions were studied, and the roles of reactive radicals (i.e., HO• and ) were evaluated. The variation of cells integrity and typical AOM characteristics were investigated. The interaction between algae and TCP, and some influencing factors were also assessed.
Materials and Methods
Materials
TCP (98%) was purchased from Aladdin (Shanghai, China), and its stock solution of 12 mM was prepared by pure water (Micropure UV, Thermo Fisher Scientific, USA) containing 0.01 mM NaOH. M. aeruginosa (No. FACHB-909) was obtained from the Institute of Hydrobiology, Chinese Academy of Sciences and cultured in BG-11 media according to our previous research (Wang et al., 2016). Cells were harvested by centrifugation at 4,500 rpm for 10 min and re-suspended with 15 mM NaClO4 solution twice to remove residual BG-11 media. Then the algae solution was diluted to 1 × 106 cells/mL by adding 15 mM NaClO4 solution (Liu et al., 2018). All the other chemical reagents of analytical grade at least were obtained from Sinopharm Chemical Reagent Co., Ltd. (Shanghai, China).
Experimental Procedures
For the UV/PS system, a 600 mL cylindrical glass vessel equipped with a low-pressure mercury UV lamp (254 nm, GPH135T5 L/4, Heraeus Noblelight) was used following our previous research (Supplementary Figure 1; Wang et al., 2016). Three different UV intensities (2.71, 5.37, and 7.82 mW/cm2) were obtained through wrapping the UV lamp with copper wire cloth and measured by the photolysis of H2O2 (Li et al., 2012). The UV reactor was thermo-stated (DC-0510, Hannuo, China) at 25 ± 1°C and switched on at least 15 min before the reaction. Na2S2O8 powder was added to the prepared solution containing both algae and TCP, then the solution was quickly transferred into the UV reactor after adjusting the pH by 0.1 M HClO4 or NaOH. After the reaction, samples were collected at predetermined time intervals and quenched using excess sodium thiosulfate for further measurement.
Analytical Methods
The absorbance of M. aeruginosa at 680 nm (OD680) was read by a UV-vis spectrophotometer (U-3100PC, Mapada, Shanghai, China), which was linear with cell density with R2 of 0.996 in this study (Supplementary Figure 2; Wang et al., 2016). TCP was first acetylated with acetic anhydride in the presence of K2CO3 and then measured by a gas chromatography (GC-2014C, Shimadzu, Japan) equipped with a ZB-5 column (30 m × 0.25 mm, ID 0.25 μm) and an electron capture detector (ECD) (Rodríguez et al., 1996). Chromatographic parameters include 200°C injector temperature, 290°C ECD temperature, and 160°C oven temperature.
Other parts of the samples were filtered through 0.45 μm cellulose acetate membranes and characterized by fluorescence excitation-emission matrix (EEM) using an F-4600 fluorescence spectrophotometer (Hitachi, Japan). The PS concentration was measured by a rapid spectrophotometric method according to a previous research (Liang et al., 2008). A flow cytometer (FCM) (Guava easyCyte5, Amnis Merck Millipore, USA) at a fixed wavelength of 488 nm was employed to evaluate the cell breakage during reaction. SYTOX Green nucleic acid stain (Life Technologies, US) was used to determine the percentages of viable and non-viable cells (Xie et al., 2013; Sun et al., 2018). In detail, 0.5 mL sample was stained with 50 nM SYTOX Green nucleic acid stain and incubated for 10 min at room temperature. The settings used for FCM were: flow rate mode = very low, FSC = 100 – 105, SSC = 100 – 105, Number of events = 5,000. The Green fluorescence (530 nm) and the red fluorescence (630 nm) were recorded in channel Green-B and Red-B, respectively.
Results and Discussion
Comparison of UV, PS, and UV/PS Processes on Algae and TCP Removal
Figure 1 shows the removal of algae and TCP under UV irradiation, PS oxidation, and UV/PS oxidation. Negligible removal of algae or TCP was observed in the presence of 4.5 mM PS, indicating that PS could not effectively remove algae or TCP through direct oxidation. When the solution was treated by UV irradiation, 37.8% of algae was removed after 120 min, and the coexisted TCP was rapidly degraded by 94.1% within 10 min. Among all the selected processes, UV/PS showed the best performance on algae and TCP removal as 97.9% of algal cells and 98.7% of the coexisted TCP were removed after reaction. The coexisted TCP was removed much faster (98.7% removal within 10 min) than algal cells in the UV/PS process, indicating that TCP was more vulnerable when it coexisted in algae solution. Although both UV and UV/PS processes can efficiently degrade TCP, the TCP degradation rate in the UV/PS process was far quicker than that in the UV alone. The aforementioned results suggest that the formed reactive radicals in the UV/PS process were believed to account for the removal of algal cells and TCP, while direct UV radiation would also play an important role in TCP degradation.
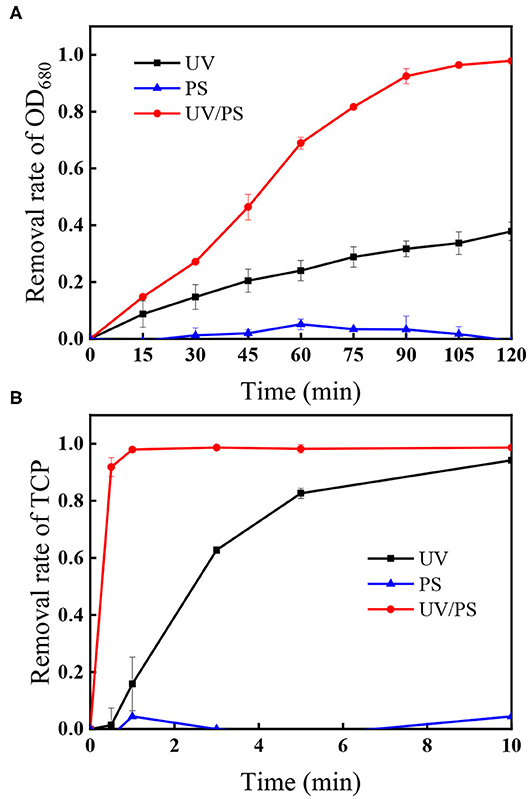
Figure 1. The removal of (A) OD680 and (B) TCP under different treatment processes. Conditions: initial algal cell density: (1.00 ± 0.05) × 106 cells/mL, initial TCP concentration: 20 μM, initial pH: 7.6 ± 0.2, temperature: 25 ± 1°C, UV intensity: 7.82 mW/cm2, PS dose: 4.5 mM. The error bars represent the standard deviations from duplicate tests.
Roles of Sulfate and Hydroxyl Radicals in the UV/PS Process
In the UV/PS process, the photolysis of PS generates and HO• via Equation (1) and (2) (Mark et al., 1990; Xie et al., 2015). Thus, HO• and are usually the main reactive radicals in the UV/PS process, which would be responsible for the degradation of algal cells and TCP. To confirm the roles of HO• and , tert-butyl alcohol (TBA) and methanol (MeOH) were applied as specific scavengers. MeOH can scavenge both HO• and with the second reaction rate constant of 1.1 × 107 M−1s−1 for and 9.7 × 108 M−1s−1 for HO•, respectively (Buxton et al., 1988; Neta et al., 1988). As for TBA, the second reaction rate constant for HO• (6.0 × 108 M−1s−1) is 1,500-fold greater than that for (4.0 × 105 M−1s−1), suggesting that it is only a good scavenger of HO• (Buxton et al., 1988; Neta et al., 1988). Figure 2A displays the changes of algae within 120 min in the UV/PS process, and the removal efficiencies of algae in the presence of TBA or MeOH were 47.8 and 39.5%, respectively. Comparing with the removal rate without the presence of scavengers (control in Figure 2A), it could be concluded that the algae removal is mainly attributed to HO• and UV irradiation (Figure 1A). Although also took part in the removal of algal cells, it was not the dominant reactive species in algae oxidation, which could be explained by the fact that shows lower reactivity toward microbes compared to HO• (Sun et al., 2016). Furthermore, the zeta potential of M. aeruginosa ranges from −25 to −30 mV at the selected pH (7.0), which would decrease the contact possibility between the algal cells and negatively charged (Henderson et al., 2008; Chen et al., 2017a; Liu et al., 2017).
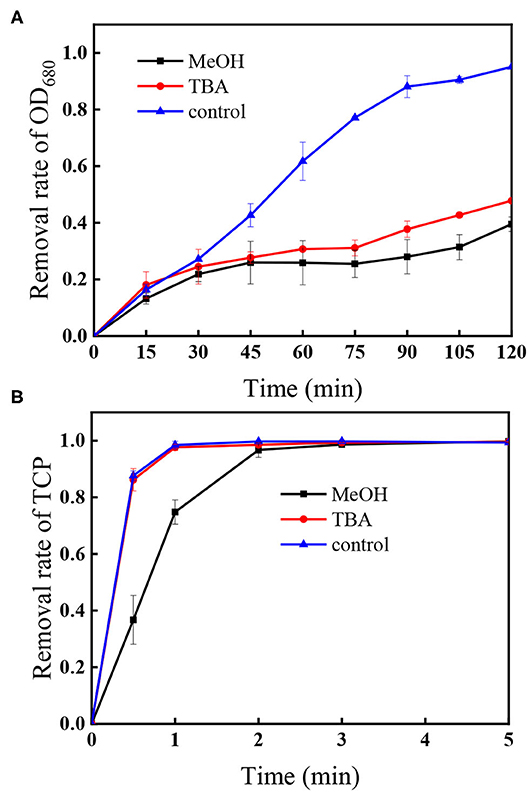
Figure 2. Impacts of TBA and MeOH on the removal of (A) OD680 and (B) TCP. Conditions: initial algal cell density: (1.00 ± 0.05) × 106 cells/mL, initial TCP concentration: 20 μM, initial pH: 7.6 ± 0.2, temperature: 25 ± 1°C, UV intensity: 7.82 mW/cm2, PS dose: 1.5 mM, [MeOH] = [TBA] = 22.5 mM. The error bars represent the standard deviations from duplicate tests.
As shown in Figure 2B, the degradation of TCP in the presence of TBA in the UV/PS process followed the same trend as that without TBA (control in Figure 2B), while the addition of MeOH can efficiently slow down the degradation rate of TCP in the process. As TBA can scavenge HO• but has negligible effect on (Chen et al., 2017b), this result suggests that the coexisted TCP could be degraded by and direct UV irradiation.
Interaction Between Algae and TCP
As discussed above, the main reactive radicals oxidizing algal cells and TCP were HO• and , respectively, which left the question of the possible interaction of these radicals with TCP and algal cells. Supplementary Figure 3A shows the removal of TCP under different initial TCP concentrations in the UV/PS process. As expected, an increase in the initial TCP concentration causes a decrease in TCP degradation. When TCP concentration increased from 5 to 20 μM, the degradation efficiencies decreased from 97.5 to 64.2% in 0.5 min. Furthermore, the presence of TCP could decrease algae removal somewhat, with the removal of algae decreased by about 13.3% after 60 min in the presence of 20 μM TCP compared to the sample in the absence of TCP (Supplementary Figure 3B). The scavenging effect of TCP on and further inhibiting the generation of HO• via Equation (2) were expected to be responsible for the inhibition of algae removal (Xie et al., 2015).
Supplementary Figure 4A shows similar final algal cell density after reaction for 120 min in the presence of 0.75 mM PS even the initial algal cells were doubled with the initial OD680 increasing from 0.02 to 0.04, suggesting that higher initial algae concentration could accelerate the removal of algal cells in the system. The possible reason was that higher concentration of algal cells would compete more reactive radicals. However, the different initial algal density showed little effect on TCP degradation, which could be explained by the fact that the initial generated only had small reactivity toward algal cells (Figure 2).
EEM Spectra of Released IOM and Cell Integrity
The fluorescence EEM spectra of released IOM under different treatment processes including UV alone, PS and UV/PS oxidation are shown in Supplementary Figure 5. IOM was not be released into solutions in both untreated and PS oxidation processes as there were no obvious fluorescence signal observed in Supplementary Figures 5A,B. After UV irradiation for 30 min, four EEM peaks including peak I (Ex/Em ~ 350/432 nm, represents humic acid-like organics), peak II (Ex/Em ~ 270/442 nm, represents fulvic acid-like materials), peak III (Ex/Em ~ 230/330 nm, represents simple aromatic proteins), and peak IV (Ex/Em ~ 280/330 nm, represents soluble microbial byproduct-like materials) suggested that IOM were released from cells by UV treatment (Supplementary Figure 5C; Chen et al., 2003). All of these peaks disappeared after UV/PS oxidation (Supplementary Figure 5D), suggesting that the released IOM would be further oxidized by the generated radicals. Additionally, the presence of 20 μM TCP did not affect the fluorescence of IOM in the UV/PS process after 30 min (Supplementary Figure 5E), which might attribute to the fast removal of TCP with a relatively high dose of PS (1.5 mM).
To further understand the performance of EEMs spectra of IOM in the presence of TCP, PS was decreased to 0.05 mM. Figure 3 depicts the EEMs spectra of the released IOM as a function of reaction time in the UV/PS process with or without TCP, and no obvious peaks were observed in the absence of TCP (Figure 3A). It has reported that the IOM from M. aeruginosa could be fast oxidized by reactive radicals (Lee et al., 2018), which can explain the no obvious peaks during the treatment by UV/PS in the absence of TCP. In contrast, when TCP coexisted in the algae solution, peaks IV and III were first observed to be enhanced after 5 min reaction and then began to disappear when the oxidation time was over 10 min, suggesting that the presence of TCP could retard the oxidation of the released IOM in the UV/PS process (Figure 3A). Additionally, although no peaks in areas I and II were observed after oxidation for 5 min, significant peaks appeared in the two areas after treatment for 10 min, and their intensities followed a decreasing trend with further prolonging treatment time. The aforementioned results suggested that the released IOM contained numerous protein-like fractions which can be transferred to humic and fulvic acid-like compounds by UV/PS treatment. Then the formed humic and fulvic acid-like compounds can be further oxidized or even mineralized by the reactive radicals in the UV/PS process. These results are partly in line with some previous studies majoring in the treatment of algae-containing water by UV-based advanced oxidation processes (Wang et al., 2016; Sun et al., 2018; Chen et al., 2020).
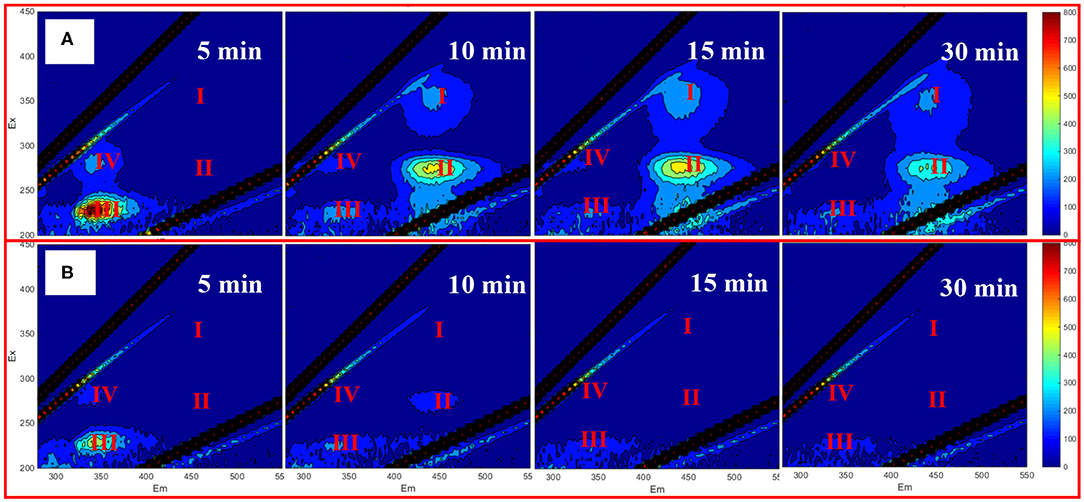
Figure 3. EEM of extracellular AOM with 20 μM TCP (A) and without TCP (B). Conditions: initial algal cell density: (1.00 ± 0.05) × 106 cells/mL, initial pH: 7.6 ± 0.2, temperature: 25 ± 1°C, UV intensity: 7.82 mW/cm2, PS dose: 0.05 mM. The error bars represent the standard deviations from duplicate tests.
Figure 4 presents the flow cytometry results under UV and UV/PS treatment, and SYTOX green nucleic acid stain was used to distinguish the viable and non-viable cells. The RED-B and Green-B represent chlorophyll auto-fluorescence and cell permeability, respectively (Daly et al., 2007; Xie et al., 2013). Region I (SYTOX Green positive) and Region II (SYTOX green negative) were associated with the percentages of the non-viable and viable cells. As shown in Figure 4a, most of the algae are viable cells in the untreated sample as 88.87% of cells showed SYTOX green negative in Region II. Compared with the untreated sample, the proportion of viable cells decreased from 88.87 to 29.65% after UV irradiation for 5 min and further decreased to 0.94% within 10 min, indicating that UV irradiation played significant roles in cell destruction. However, the proportion of cells in Region II increased again (98.36% in 5 min and 99.08% in 10 min) in the UV/PS process (Figures 4d,e), which was similar to a previous research (Wang et al., 2016). This phenomenon could be explained by the fact that the nucleic acid in cells was further oxidized by the generated and HO• as no signals from nucleic acid stained by SYTOX were found in Region I (Figures 4d,e; Daly et al., 2007). Additionally, there are no signals in Region I when TCP was added in the solution in the UV/PS process (Figures 4f,g), which suggested that the presence of TCP cannot retard the nucleic acid oxidation at the given reaction conditions.
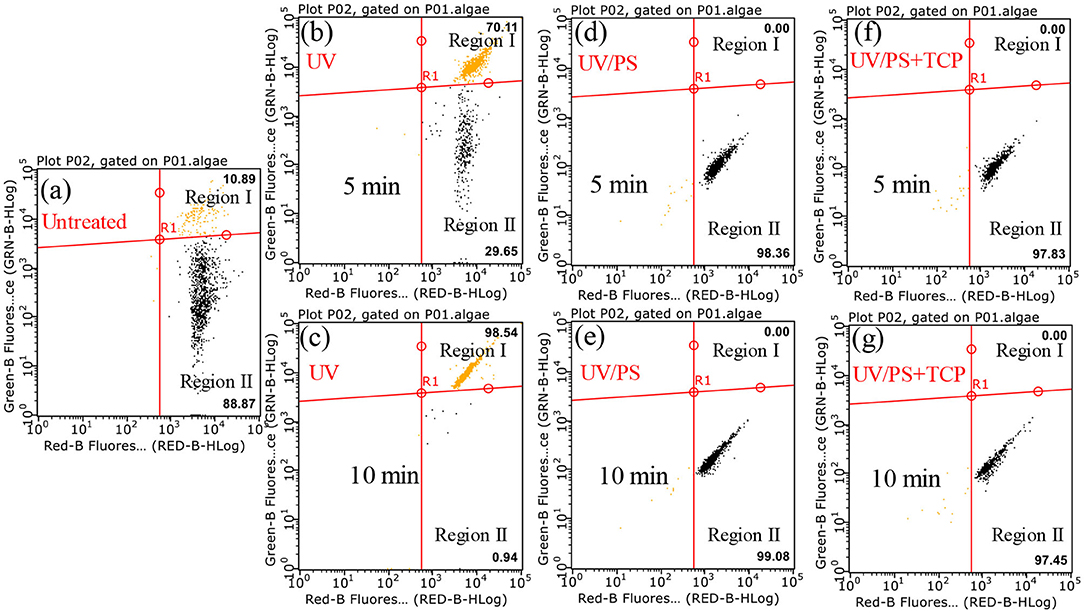
Figure 4. Flow cytometry results after treatment by different processes. Conditions: initial algal cell density: (1.00 ± 0.05) × 106 cells/mL, initial TCP concentration: 0 μM for (a–e) and 20 μM for (f,g), initial pH: 7.6 ± 0.2, temperature: 25 ± 1°C, UV intensity: 7.82 mW/cm2, PS dose: 1.5 mM. The error bars represent the standard deviations from duplicate tests.
Effects of PS Dose and UV Intensity
Figure 5 presents the effect of PS concentration (0.75–4.5 mM) on algal cells and TCP degradation in the UV/PS process, and the removal efficiency of algal cells and TCP increased from 75.5 to 97.9% after 120 min (Figure 5A) and from 46.8 to 97.9% within 1 min (Figure 5B), respectively. In the selected PS range, the coexisted TCP was quickly degraded within 5 min (removal rate >97%). Initial PS concentration is critical in UV/PS process as PS is the source of (Equation 1), as a result to promoting the removal of algae and the degradation of micro-organic pollutants with elevating PS dosage (Xie et al., 2015; Chen et al., 2017b). As nearly all the cells showed non-viable after 10 min UV irradiation and nucleic acid was totally destroyed within 5 min in the UV/PS process (Figure 4), the algal cells must underwent an irreversible rupture by UV/PS oxidation, and deposed to fragments after that.
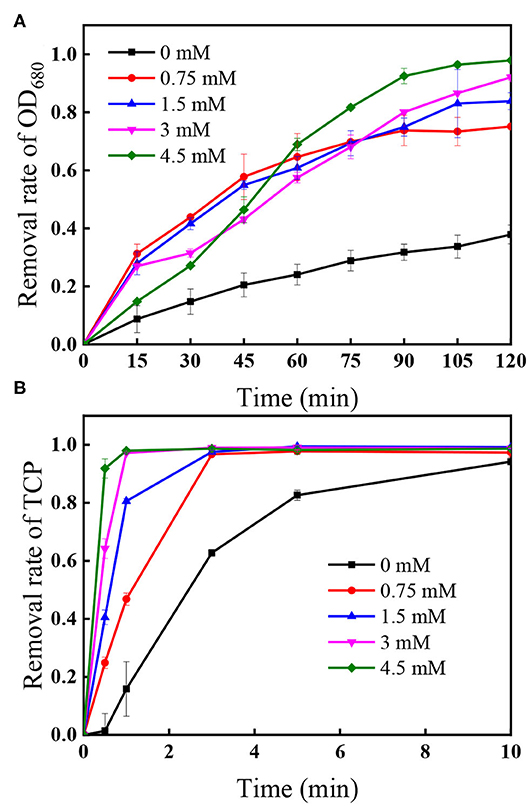
Figure 5. Effect of PS dose on the removal of (A) OD680 and (B) TCP. Conditions: initial algal cell density: (1.00 ± 0.05) × 106 cells/mL, initial TCP concentration: 20 μM, initial pH: 7.6 ± 0.2, temperature: 25 ± 1°C, UV intensity: 7.82 mW/cm2. The error bars represent the standard deviations from duplicate tests.
Supplementary Figure 6 shows the PS decomposition under different initial PS concentration followed the first-order kinetics in the selected PS range, indicating that the presence of algal cells and TCP would not affect the first-order photolysis of PS significantly. Additionally, the residual PS after 120 min UV irradiation was <2% in each selected initial PS dose, suggesting that few PS would left after applying UV/PS to simultaneously treat algae and TCP.
Figure 6 shows that the removal of algal cells increased from 62.2 to 95.0% when UV intensity increased from 2.71 to 7.82 mW/cm2 within 120 min, accompanied with rapid decomposition efficiencies of the coexisted TCP increasing from 83.3 to 98.4% within 1 min. As the radical quantum yields of PS under UV254 activation is 1.4 (Crittenden et al., 1999), more reactive radicals including and HO• would be generated under higher UV intensity (Xie et al., 2015). Additionally, Figure 1 shows that direct photolysis also played important role in the removal of algae and the TCP degradation, meaning that higher photon intensity can also improve the direct photolysis efficiency.
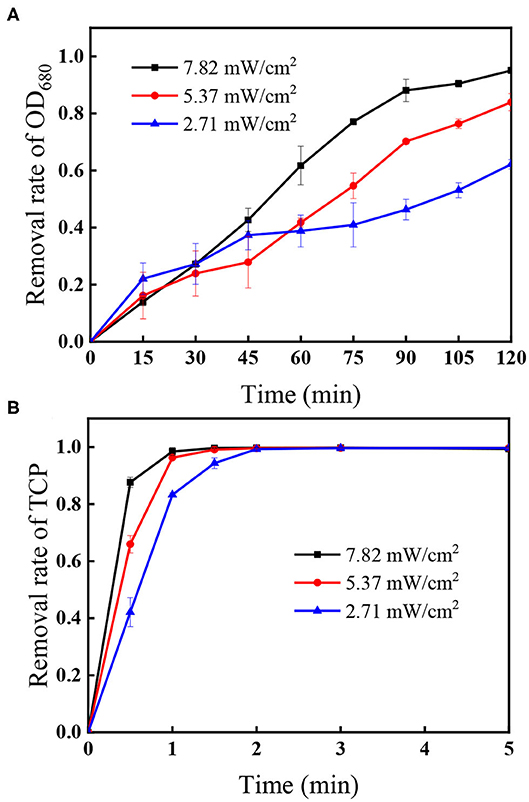
Figure 6. Effect of UV intensity on the removal of (A) OD680 and (B) TCP. Conditions: initial algal cell density: (1.00 ± 0.05) × 106 cells/mL, initial TCP concentration: 20 μM, initial pH: 7.6 ± 0.2, temperature: 25 ± 1°C, PS dose: 1.5 mM. The error bars represent the standard deviations from duplicate tests.
Conclusion
In this study, UV/PS process was firstly applied to simultaneously treat algal cells (M. aeruginosa) and TCP. and direct UV photolysis dominated TCP degradation, while HO• and UV direct photolysis were evidenced to play the primary roles in algae removal. Increasing PS dose and UV intensity could enhance both TCP and algae removal. Higher initial TCP concentration (20 μM) can significantly slow down the removal of algal cells and TCP, but high final treatment efficiency was always achieved. In the selected algal densities (OD680 = 0.02, 0.04), initial algae densities played little role in the remained OD680 and TCP concentration after UV/PS treatment. Although IOM were released from the lysed algal cells in the UV/PS process, they could be further oxidized by the generated reactive radicals. Due to the competition of generated reactive radicals, the presence of TCP could inhibit IOM removal in the UV/PS process. However, the inhibitory effect could be neglected at high PS dose (>1.5 mM). After reaction for 120 min in the selected experimental conditions, the residual PS was <2%, ensuring the safety of UV/PS treatment strategy to degrade TCP and algae.
Data Availability Statement
All datasets generated for this study are included in the article/Supplementary Material.
Author Contributions
JW: conceptualization, data curation, writing-original draft preparation, formal analysis, and investigation. JD, SY, and YW: writing-reviewing. PX: conceptualization, co-supervision, writing-reviewing and editing, project administration, formal analysis, funding acquisition, and resources. ZW: co-supervision, writing-reviewing, formal analysis, and resources. All authors approved it for publication.
Funding
This study was supported by the Natural Science Foundation of China (grants 51878308 and 51578259).
Conflict of Interest
The authors declare that the research was conducted in the absence of any commercial or financial relationships that could be construed as a potential conflict of interest.
Acknowledgments
We gratefully acknowledge the HUST Training Program for Excellent Young Teachers for the support of PX to do research at CEINT, Duke University. The analysis offered by Analytical and Testing Center of HUST and the valuable work of editor and reviewers are also appreciated.
Supplementary Material
The Supplementary Material for this article can be found online at: https://www.frontiersin.org/articles/10.3389/fchem.2020.591641/full#supplementary-material
References
Buxton, G. V., Greenstock, C. L., Helman, W. P., and Ross, A. B. (1988). Critical review of rate constants for reactions of hydrated electrons, hydrogen atoms and hydroxyl radicals (•OH/•O−) in aqueous solution. J. Phys. Chem. Ref. Data 17, 513–886. doi: 10.1063/1.555805
Chen, W., Westerhoff, P., Leenheer, J. A., and Booksh, K. (2003). Fluorescence excitation-emission matrix regional integration to quantify spectra for dissolved organic matter. Environ. Sci. Technol. 37, 5701–5710. doi: 10.1021/es034354c
Chen, Y., Bai, F., Li, Z., Xie, P., Wang, Z., Feng, X., et al. (2020). UV-assisted chlorination of algae-laden water: cell lysis and disinfection byproducts formation. Chem. Eng. J. 383, 123165. doi: 10.1016/j.cej.2019.123165
Chen, Y., Deng, P., Xie, P., Shang, R., Wang, Z., and Wang, S. (2017b). Heat-activated persulfate oxidation of methyl-and ethyl-parabens: effect, kinetics, and mechanism. Chemosphere 168, 1628–1636. doi: 10.1016/j.chemosphere.2016.11.143
Chen, Y., Xie, P., Wang, Z., Shang, R., and Wang, S. (2017a). UV/persulfate preoxidation to improve coagulation efficiency of Microcystis aeruginosa. J. Hazard. Mater. 322, 508–515. doi: 10.1016/j.jhazmat.2016.10.017
Chow, C. W. K., Drikas, M., House, J., Burch, M. D., and Velzeboer, R. M. A. (1999). The impact of conventional water treatment processes on cells of the cyanobacterium Microcystis aeruginosa. Water Res. 33, 3253–3262. doi: 10.1016/S0043-1354(99)00051-2
Crittenden, J. C., Hu, S., Hand, D. W., and Green, S. A. (1999). A kinetic model for H2O2/UV process in a completely mixed batch reactor. Water Res. 33, 2315–2328. doi: 10.1016/S0043-1354(98)00448-5
Daly, R. I., Ho, L., and Brookes, J. D. (2007). Effect of chlorination on Microcystis aeruginosa cell integrity and subsequent microcystin release and degradation. Environ. Sci. Technol. 41, 4447–4453. doi: 10.1021/es070318s
de la Cruz, A., Logsdon, R., Lye, D., Guglielmi, S., Rice, A., and Kannan, M. (2017). Harmful algae bloom occurrence in urban ponds: relationship of toxin levels with cell density and species composition. J. Earth Environ. Sci. 25, 704–726. doi: 10.29011/JEES-148.100048
Henderson, R., Parsons, S. A., and Jefferson, B. (2008). The impact of algal properties and pre-oxidation on solid-liquid separation of algae. Water Res. 42, 1827–1845. doi: 10.1016/j.watres.2007.11.039
Keith, D., Rover, J., Green, J., Zalewsky, B., Charpentier, M., Thursby, G., et al. (2018). Monitoring algal blooms in drinking water reservoirs using the Landsat-8 operational land imager. Int. J. Remote Sens. 39, 2818–2846. doi: 10.1080/01431161.2018.1430912
Lee, D., Kwon, M., Ahn, Y., Jung, Y., Nam, S. N., Choi, I., et al. (2018). Characteristics of intracellular algogenic organic matter and its reactivity with hydroxyl radicals. Water Res. 144, 13–25. doi: 10.1016/j.watres.2018.06.069
Li, X., Ma, J., Liu, G., Fang, J., Yue, S., Guan, Y., et al. (2012). Efficient reductive dechlorination of monochloroacetic acid by sulfite/UV process. Environ. Sci. Technol. 46, 7342–7349. doi: 10.1021/es3008535
Liang, C., Huang, C.-F., Mohanty, N., and Kurakalva, R. M. (2008). A rapid spectrophotometric determination of persulfate anion in ISCO. Chemosphere 73, 1540–1543. doi: 10.1016/j.chemosphere.2008.08.043
Liu, B., Qu, F., Liang, H., Gan, Z., Yu, H., Li, G., et al. (2017). Algae-laden water treatment using ultrafiltration: individual and combined fouling effects of cells, debris, extracellular and intracellular organic matter. J. Memb. Sci. 528, 178–186. doi: 10.1016/j.memsci.2017.01.032
Liu, B., Qu, F., Yu, H., Tian, J., Chen, W., Liang, H., et al. (2018). Membrane fouling and rejection of organics during algae-laden water treatment using ultrafiltration: a comparison between in situ pretreatment with Fe(II)/persulfate and ozone. Environ. Sci. Technol. 52, 765–774. doi: 10.1021/acs.est.7b03819
Liu, Z., Cui, F., Ma, H., Fan, Z., Zhao, Z., Hou, Z., et al. (2012). Bio-reaction of nitrobenzene with Microcystis aeruginosa: characteristics, kinetics and application. Water Res. 46, 2290–2298. doi: 10.1016/j.watres.2012.01.049
Mark, G., Schuchmann, M. N., Schuchmann, H.-P., and von Sonntag, C. (1990). The photolysis of potassium peroxodisulphate in aqueous solution in the presence of tert-butanol: a simple actinometer for 254 nm radiation. J. Photochem. Photobiol. A Chem. 55, 157–168. doi: 10.1016/1010-6030(90)80028-V
Matzek, L. W., and Carter, K. E. (2016). Activated persulfate for organic chemical degradation: a review. Chemosphere 151, 178–188 doi: 10.1016/j.chemosphere.2016.02.055
Neta, P., Huie, R. E., and Ross, A. B. (1988). Rate constants for reactions of inorganic radicals in aqueous solution. J. Phys. Chem. Ref. Data 17, 1027–1284. doi: 10.1063/1.555808
Olmez-Hanci, T., Dursun, D., Aydin, E., Arslan-Alaton, I., Girit, B., Mita, L., et al. (2015). S2/UV-C and H2O2/UV-C treatment of bisphenol A: assessment of toxicity, estrogenic activity, degradation products and results in real water. Chemosphere 119, S115–S123. doi: 10.1016/j.chemosphere.2014.06.020
Paerl, H. W., and Otten, T. G. (2013). Harmful cyanobacterial blooms: causes, consequences, and controls. Microb. Ecol. 65, 995–1010. doi: 10.1007/s00248-012-0159-y
Rajasekhar, P., Fan, L., Nguyen, T., and Roddick, F. A. (2012). A review of the use of sonication to control cyanobacterial blooms. Water Res. 46, 4319–4329. doi: 10.1016/j.watres.2012.05.054
Rodríguez, I., Turnes, M. I., Mejuto, M. C., and Cela, R. (1996). Determination of chlorophenols at the sub-ppb level in tap water using derivatization, solid-phase extraction and gas chromatography with plasma atomic emission detection. J. Chromatogr. A 721, 297–304. doi: 10.1016/0021-9673(95)00795-4
Shen, Q., Zhu, J., Cheng, L., Zhang, J., Zhang, Z., and Xu, X. (2011). Enhanced algae removal by drinking water treatment of chlorination coupled with coagulation. Desalination 271, 236–240. doi: 10.1016/j.desal.2010.12.039
Sun, J., Bu, L., Deng, L., Shi, Z., and Zhou, S. (2018). Removal of Microcystis aeruginosa by UV/chlorine process: Inactivation mechanism and microcystins degradation. Chem. Eng. J. 349, 408–415. doi: 10.1016/j.cej.2018.05.116
Sun, P., Tyree, C., and Huang, C. H. (2016). Inactivation of Escherichia coli, bacteriophage MS2, and bacillus spores under UV/H2O2 and UV/Peroxydisulfate advanced disinfection conditions. Environ. Sci. Technol. 50, 4448–4458. doi: 10.1021/acs.est.5b06097
Teixeira, M. R., and Rosa, M. J. (2006). Comparing dissolved air flotation and conventional sedimentation to remove cyanobacterial cells of Microcystis aeruginosa: Part I: The key operating conditions. Sep. Purif. Technol. 52, 84–94. doi: 10.1016/j.seppur.2006.03.017
Wacławek, S., Lutze, H. V., Grübel, K., Padil, V. V. T., Cerník, M., and Dionysiou, D.D. (2017). Chemistry of persulfates in water and wastewater treatment: a review. Chem. Eng. J. 330, 44–62. doi: 10.1016/j.cej.2017.07.132
Wang, M., and Wei, S. (2008). Satellite-observed algae blooms in China's Lake Taihu. Eos Trans. Am. Geophys. Union. 89, 201–208. doi: 10.1029/2008EO220001
Wang, Z., Chen, Y., Xie, P., Shang, R., and Ma, J. (2016). Removal of Microcystis aeruginosa by UV-activated persulfate: performance and characteristics. Chem. Eng. J. 300, 245–253. doi: 10.1016/j.cej.2016.04.125
Xie, P., Chen, Y., Ma, J., Zhang, X., Zou, J., and Wang, Z. (2016). A mini review of preoxidation to improve coagulation. Chemosphere 155, 550–563. doi: 10.1016/j.chemosphere.2016.04.003
Xie, P., Ma, J., Fang, J., Guan, Y., Yue, S., Li, X., and Chen, L. (2013). Comparison of permanganate preoxidation and preozonation on algae containing water: cell integrity, characteristics, and chlorinated disinfection byproduct formation. Environ. Sci. Technol. 47, 14051–14061. doi: 10.1021/es4027024
Keywords: Microcystis aeruginosa, 2,4,6-trichlorophenol, ultraviolet/persulfate, advanced oxidation process, cell integrity
Citation: Wang J, Wan Y, Yue S, Ding J, Xie P and Wang Z (2020) Simultaneous Removal of Microcystis aeruginosa and 2,4,6-Trichlorophenol by UV/Persulfate Process. Front. Chem. 8:591641. doi: 10.3389/fchem.2020.591641
Received: 05 August 2020; Accepted: 07 September 2020;
Published: 04 November 2020.
Edited by:
Liangliang Wei, Harbin Institute of Technology, ChinaCopyright © 2020 Wang, Wan, Yue, Ding, Xie and Wang. This is an open-access article distributed under the terms of the Creative Commons Attribution License (CC BY). The use, distribution or reproduction in other forums is permitted, provided the original author(s) and the copyright owner(s) are credited and that the original publication in this journal is cited, in accordance with accepted academic practice. No use, distribution or reproduction is permitted which does not comply with these terms.
*Correspondence: Pengchao Xie, cGVuZ2NoYW9feGllJiN4MDAwNDA7aHVzdC5lZHUuY24=; Zongping Wang, em9uZ3Bpbmd3JiN4MDAwNDA7aHVzdC5lZHUuY24=