- 1Institute of Chemistry, Academia Sinica, Taipei, Taiwan
- 2Sree Dattha Institute of Engineering and Science, Hyderabad, India
The process of selective oxy-functionalization of hydrocarbons using peroxide, O3, H2O2, O2, and transition metals can be carried out by the reactive oxygen species such as hydroxyl/hydroperoxyl radical and/or metal oxygenated species generated in the catalytic reaction. Thus, a variety of mechanisms have been proposed for the selective catalytic oxidation of various hydrocarbons including light alkanes, olefins, and simple aromatics by the biological metalloproteins and their biomimetics either in their homogeneous or heterogeneous platforms. Most studies involving these metalloproteins are Fe or Cu monooxygenases. The pathways carried out by these metalloenzymes in the oxidation of C–H bonds invoke either radical reaction mechanisms including Fenton's chemistry and hydrogen atom transfer followed by radical rebound reaction mechanism or electrophilic oxygenation/O-atom transfer by metal-oxygen species. In this review, we discuss the metal oxide nano-catalysts obtained from metal salts/molecular precursors (M = Cu, Fe, and V) that can easily form in situ through the oxidation of substrates using H2O2(aq) in CH3CN, and be facilely separated from the reaction mixtures as well as recycled for several times with comparable catalytic efficiency for the highly selective conversion from hydrocarbons including aromatics to oxygenates. The mechanistic insights revealed from the oxy-functionalization of simple aromatics mediated by the novel biomimetic metal oxide materials can pave the way toward developing facile, cost-effective, and highly efficient nano-catalysts for the selective partial oxidation of simple aromatics.
Introduction
The technology of “advanced oxidation processes” essentially applies Fenton's chemistry for wastewater treatment (Andreozzi et al., 1999; Pignatello et al., 2006). The primary oxidants including O3, H2O2, O2, transition metal oxo-species, light, and electrical energy are employed to remove the organic (and sometimes inorganic) wastes in wastewater via the reactive oxygen species (ROS) and/or UV light (Deng and Zhao, 2015). These remediation processes can also be properly controlled and applied for the selective catalytic oxidation of hydrocarbons by designing variable homo- and heterogeneous catalyst systems to achieve green and environmentally benign fine chemical production (Ottenbacher et al., 2020; Suib et al., 2020).
In addition to accumulate ROS, it has been believed that the transition metals such as M = Fe, Cu, Co, and Mn in H2O2(aq) can induce the metal oxygenates for the hydrocarbon activation (Sawyer et al., 1996). In fact, Fenton's chemistry can both possibly occur with the Haber–Weiss reaction mechanism (Equation 1) (Walling, 1975; Pignatello et al., 2006) for the accumulation of HO·/HOO· and for the formation ferryl-oxo species via Bray–Gorin mechanisms (Equation 2) (Bossmann et al., 1998; Enami et al., 2014). Interestingly, recent reports indicated the observation of Fenton's chemistry at the aqueous interface by mass spectrometry where there was no HO· observed but several ferryl-oxo species including dimeric forms were detected (Enami et al., 2014). The dimeric ferryl-oxo species are actually more reactive than the monomeric ferryl-oxo species for O-atom transfer (OAT) reaction. These further progresses in Fenton's chemistry have provided a significant insight to indicate the importance of the electronic coupling interplaying among the iron oxide clustering sites (Enami et al., 2014).
For the selective oxidation of sp2- vs. sp3-carbons, “toluene” can be considered as a great example to illustrate the reaction behaviors mediated either by ROS or transition metal oxygenated species. If the reactions are mediated by ROS of HO· or HOO·, it may proceed either by the hydrogen atom transfer (HAT) of C–H bond in the methyl group and then radical rebound to form benzyl alcohol/benzyl hydroperoxide or direct addition of HO· to the sp2 carbon center of o- or p-positions of the methyl substituent (Figure 1).
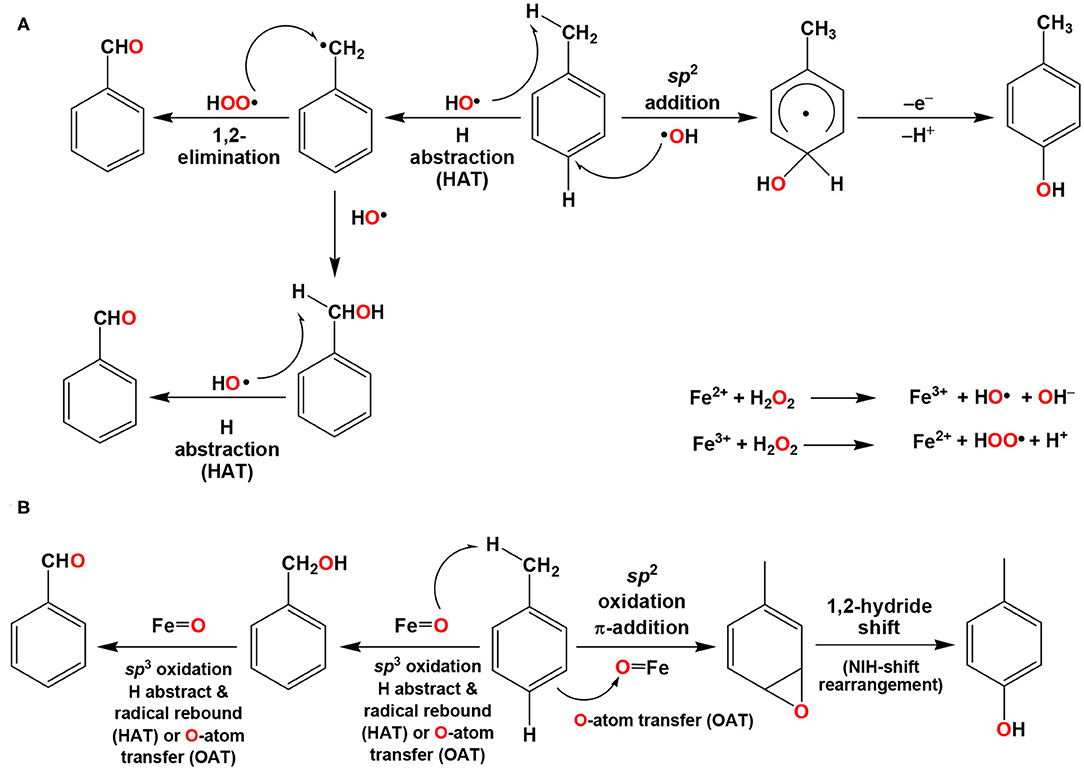
Figure 1. Ring or chain activation of toluene by (A) ROS accumulated by Fenton's reactions or (B) high valent metal oxygenate species in metalloproteins. The mechanism (B) can also be proceeded by the Bray–Gorin mechanism. Reproduced from Ramu et al. (2017). Copyright 2017 Elsevier B.V.
On the other hand, it has been long considered that the aromatic ring (sp2 carbon) oxygenation mediated by the metalloproteins such as cytochrome P450 and toluene monooxygenase (ToMO) are proceeded with the formation of arene oxide and/or σ-complex intermediates via OAT then 1,2-hydride shift (NIH shift rearrangement) toward the formation of phenolate products (Guroff et al., 1967; Boyd et al., 1972; Jerina and Daly, 1974; Bassan et al., 2003; de Visser and Shaik, 2003; Mitchell et al., 2003). With respect to sp3 C–H bond activation, the oxidation may directly be conducted by the high valent iron oxo or ferryl-oxo species either through HAT or OAT reaction mechanism (Bassan et al., 2003; de Visser and Shaik, 2003). In any case, the formation of benzaldehyde can be subsequently produced by oxidation through HAT and radical rebound or OAT toward the methylene C–H bond of benzyl alcohol (Figure 1). The bond dissociation energy (BDE = 86–89 kcal/mol) of C–H bond in methyl substituent of toluene is much lower than the ring activation of toluene (BDE = 109–112 kcal/mol) (Bassan et al., 2003; de Visser and Shaik, 2003).
The significant mechanistic feature for OAT ring oxidation can be easily determined by enriched aromatics with a deuterium atom at the site/position for oxidation (Ramu et al., 2017). For toluene oxidation using H2O2(aq), the sp2 activated site usually appeared either at the o- or p-position; therefore, [4-2H0, 1] toluene can be synthesized from 4-bromo-toluene in D2O (Morimoto et al., 2015) and used as a mechanistic probe to evidence the OAT mechanism if there is significant proportion of deuterium that remained in the molecules (Figure 2). Usually, the NIH shift ratios for metalloproteins can be up to 60–80% (Guroff et al., 1967; Boyd et al., 1972; Siegmund and Kaufman, 1991; Mitchell et al., 2003).

Figure 2. The para-hydrogen/deuterium in [4-2H0, 1]toluene can be activated by metalloprotein via 1,2-hydride shift after OAT to have significant deuterium remaining in the p-cresol product.
It is worth noting that in the past study, the addition of CH3CN to Fenton's reaction mixtures can enhance the NIH shift ratios from <5%, for FeII in aqueous solution, i.e., Fenton's oxidation, up to 50% (Castle et al., 1980; Kurata et al., 1988). These phenomena have made us wonder whether the corresponding reaction conditions can significantly accumulate any metal oxygenated species like metalloproteins of cytochrome P450 or ToMO. If so, then, how can we control the accumulated reactive oxygenated species for selective sp3 C–H vs. sp2 C–H bond oxidation via HAT or π-bond activation via OAT?
Selective Oxidation of sp2 and sp3 C–H Bond Oxidation of Toluene by Nano-Iron Oxide Particles
Molecular iron complexes including polycyclic cage-like compounds, biomimetic model complexes, and encapsulated within zeolite cages have been shown with high catalytic activity in the oxidation of hydrocarbons including alkanes and aromatics (Mori et al., 2008; Bilyachenko et al., 2016; Yalymov et al., 2017; Zhang et al., 2017). Recently, we indicated that Fe(ClO4)2 salt using H2O2(aq) in CH3CN can conduct selective oxidation of toluene either for sp2 C–H bond oxidation to cresols or sp3 C–H bond oxidation to benzyl alcohol/benzaldehyde (Ramu et al., 2017). Interestingly, the slow addition of H2O2(aq) to the reaction mixtures led to π-activation (~70%) for cresols/methyl-p-benzoquinone (BQ) formation, whereas the fast addition of H2O2(aq) resulted in the major production sp3 C–H bond oxidation at the methyl substituent (Ramu et al., 2017). Further scrutinizing the reaction mixture by the electron microscopic (EM) study revealed small nanoparticle formation with the sizes of 2–10 nm in the first 2 h with the slow addition of H2O2(aq) (Supplementary Figure 1A, Supplementary Information) and relatively larger particles of >1 μm were observed within 2 h after the fast addition of H2O2(aq) (Supplementary Figure 1B, Supplementary Information). These particles were evidenced to be consisted of iron oxides from transmission electron microscopy (TEM), energy-dispersive X-ray (Supplementary Figure 2, Supplementary Information), and elemental analysis (Supplementary Table 1, Supplementary Information) study. It is not surprising that with the fast addition of H2O2(aq), exothermic heat accumulated for the micro-size iron oxide nanoparticle formation, substantial amounts of HO·/HOO· were generated and the overall sp3 oxidation products can achieve >95%. To further examine the production of benzyl hydroperoxide, the treatment of PPh3 can further assist for its quantification (Shul'pin et al., 2010). Once the reaction is harvested, a very small amount of benzyl hydroperoxide species was observed in rapid addition of H2O2(aq). However, with slow addition of H2O2(aq), ~45% sp3 C–H bond oxidation products were observed, and the ratios for benzyl hydroperoxide, benzaldehyde, and benzyl alcohol were identified to be 18, 25, and 2%, respectively (Supplementary Table 2, Supplementary Information). Nevertheless, the selectivity for sp2 carbon oxidation to cresols/methyl-p-BQ formation, with and without treatment of PPh3 after the reactions, did not vary much with rapid or slow additions of H2O2(aq).
The study using [4-2H0, 1]toluene for its oxidation catalyzed by Fe(ClO4)2 catalyst using H2O2 in CH3CN exhibited high NIH-shift ratios of 83–86% (Ramu et al., 2017). On further examination using the resulted iron nanoparticles, the selectivity for sp2 C–H bond oxidation in cresols/p-BQ formations can still reach 65% (Supplementary Table 3, Supplementary Information) where the obtained NIH-shift ratio of deuterium remaining for p-cresol product is 81% (Supplementary Information). The outcome here implicated that the activation of aromatic π -bonds for arene oxide or σ-complex may be mediated by iron-based oxygenated species (Kudrik and Sorokin, 2008; Thibon et al., 2012; Raba et al., 2014) that was presumably arisen from the resultant particle surface of the iron nanoparticle.
Highly Selective Oxidation of Benzene to p-Benzoquinone (p-BQ) by Copper oxide Nano-catalyst
Multi-copper complexes including cage compounds were employed for selective oxidation of alkanes and aromatics with H2O2(aq) in CH3CN (You et al., 2014; Kulakova et al., 2017, 2019). Shul'pin and co-workers further reported a double oxidation from benzene to p-BQ with molar ratio of ~1:1 (PhOH:p-BQ) using Cu(ClO4)2 (Shul'pina et al., 2008). Similarly, a series of tri-copper cluster complexes achieved an efficient conversion of benzene to p-hydroquinone with percentage selectivity of 60–98% (Nagababu et al., 2012). Recently, we have discovered and studied copper oxide–based nano-catalysts for the efficient and selective double oxidation from benzene to p-BQ. During the process, the addition of optimized amount of H2O in the CH3CN solution with copper nanoparticles yielded higher overall catalytic efficiency yield based on consumed H2O2 value (98%), and better selectivity for p-BQ (77%), than other volumes of water (Wanna et al., 2019b). Most importantly, we were able to recycle the catalyst at least three times without significantly losing activity and p-BQ selectivity.
The EPR spectrum of copper oxide nanoparticles at 298 K indicated an isotropic signal, reminiscent of a previous study of pMMO with trinuclear copper cluster feature (Nguyen et al., 1994), which was significantly broadened and presented relatively poor signal-to-noise ratio than the one at 77 K (Figure 3B) (Wanna et al., 2019b). The result here reveals substantial dynamic motions around the metal centers, which are behaving like metal active sites in the metallo-monooxygenases (Chen et al., 2014; Wang et al., 2017; Yang et al., 2017). In addition, the corresponding EXAFS data fittings (k3-weighted) using two structural models of copper oxide and trinuclear copper cluster model (Cu2.8O4.6), respectively, can both give reasonable fits of Rfit = 0.027 and 0.15%, respectively (Wanna et al., 2019b), which indicated that a trinuclear copper cluster feature is manifested from the obtained oxide materials (Figure 3). Especially, the specific Cu–Cu scattering in a distance of ~3.7 Å would presumably result in Cu2+(μ-(η2:η2)-peroxo)Cu2+ (Cu–Cu distance ~3.6 Å) that can potentially reach an equilibrium of a Cu3+(μ-O)2Cu3+ structure (Lewis and Tolman, 2004; Mirica et al., 2004; Ottenwaelder et al., 2006). The formed possible intermediate of Cu2+Cu2+(μ-O)2Cu3+ can exhibit much lower activation energy for a facile oxo-transfer toward the inert C–H bond (Chen and Chan, 2006). It is not surprising that a benzene molecule can therefore easily possess an arene oxide intermediate via OAT followed by subsequent NIH rearrangement to obtain PhOH from benzene. In fact, the recycled copper nanoparticles performed with reasonable efficiency in toluene oxidation. The NIH-shift ratios obtained from the deuterated p-cresol products was 54%, which displayed that the solid-state surface of copper nanoparticles can in general oxidize ortho- or para- (sp2) C–H bonds of toluene via the formation of arene oxide intermediate followed by an NIH rearrangement process.
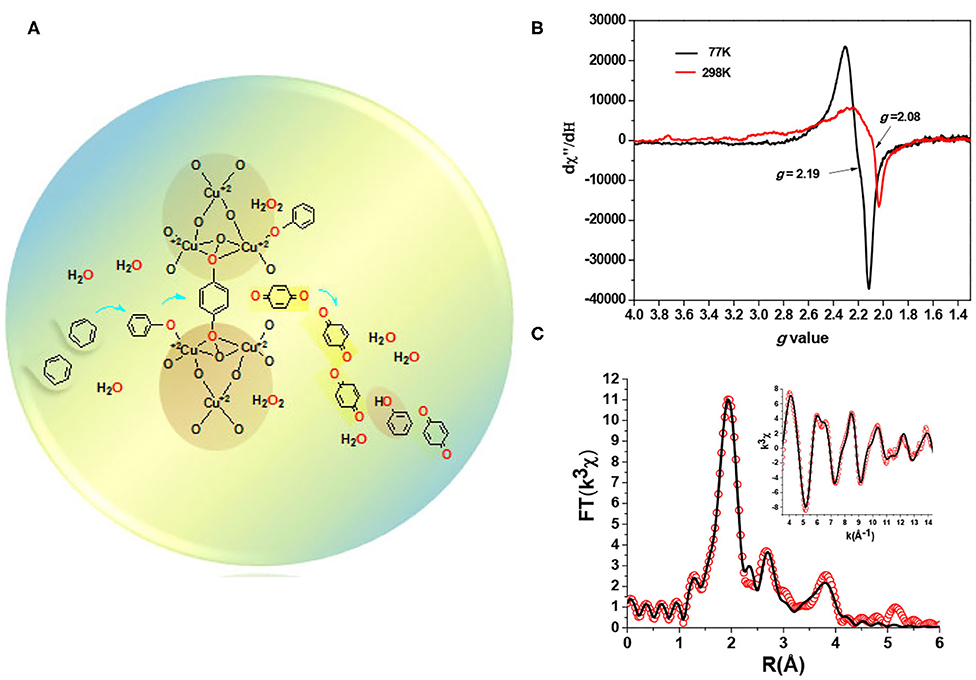
Figure 3. (A) The proposed reaction sphere in recyclable copper nano-catalyst with the active site for selective oxidation of benzene to p-benzoquinone (p-BQ) using H2O2(aq) in CH3CN; (B) EPR spectra of copper oxide nanoparticles in KBr measured at 77 and 298 K, respectively; (C) The Cu EXAFS (inset, red circle) and their Fourier transforms (red circle) as well as the corresponding best fits (black solid lines) of copper oxide nanoparticles using trinuclear copper cluster as the structural model (Chan et al., 2013). Reproduced with permission from Wanna et al. (2019b). Copyright 2019 Elsevier B.V.
The Mixed Valence Sites of V4+/V5+ Stabilized by PCA in the V2O5 for Selective Oxidation of Benzene to Phenol With High Efficiency
Vanadium-based polyoxometalates have been extensively used as green heterogeneous catalysts with high catalytic activity for selective oxidation of styrene to benzaldehyde (Zhou et al., 2020), benzene to PhOH (Li et al., 2020), and mono-substituted benzene to p-PhOH (Kamata et al., 2012). Kirillov and Shul'pin (2013) established a variety of vanadium complexes to catalyze aerobic and/or H2O2 hydroxylation of benzene to phenol and toluene to a mixture of cresols in CH3CN. Mandatory promoters such as pyridine, pyrazine-2-carboxylic acid (PCA), and acetic acid were used as co-catalysts to prompt the oxidation reactions. We recently reported a unique vanadium nanorod (Vnr) catalyst (Figure 4B) for selective oxidation of benzene to PhOH (>87%) with minor p-BQ formation in CH3CN. The presence of PCA, interacting V+4/V+5 in Vnr, can serve as Brønsted acid site to improve activity for PhOH production (Liu et al., 2017; Zhao et al., 2018). In fact, PCA ligand can serve as a stabilizer of the transition state involving V4+ species for the H-transfer (Kirillova et al., 2009) and the reduction of V5+ to V4+ by PCA with H2O2(aq) assists in accumulating more redox active centers (Shul'pin et al., 1999). During the detailed characterization of V2O5-related materials, including the Vnr catalyst prepared from VCl3, we found that lower pre-edge peak intensity of XAS data at ~5,470 eV together with XPS data analysis indicated Vnr catalyst exhibited higher V+4/V+5 ratio than the “calcined Vnr” (Vnr(cal)) and commercial V2O5 (Figure 4A). For the efficient conversion of benzene to PhOH, assisted by PCA, the vanadium materials exhibit high dependence on V+4/V+5 ratios (Table 1).
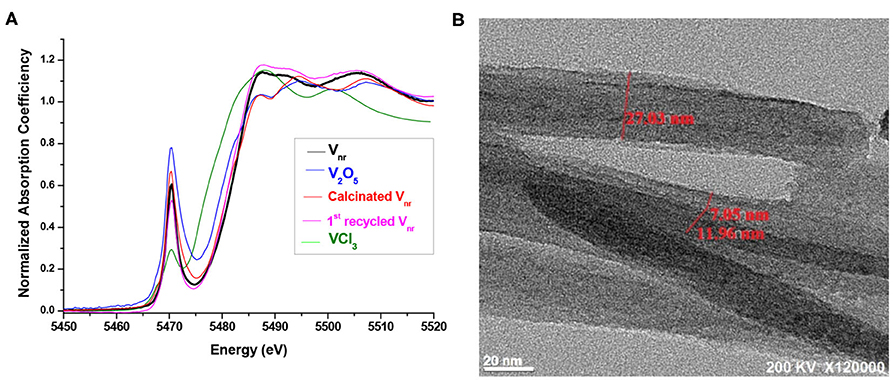
Figure 4. (A) Normalized V K-edge X-ray absorption near edge structures (XANES) of vanadium oxide nanorod (Vnr) (black line) and its calcined materials (Vnr(cal), red line), V2O5 (blue line), VCl3(aq) (green line), and the 1st recycled Vnr (magenta line) recycled from the Vnr catalyst after the catalytic reaction of benzene. (B) The high-resolution TEM image of rod-shaped Vnr. Reproduced with permission from Wanna et al. (2019a). Copyright 2019 The Royal Society of Chemistry.
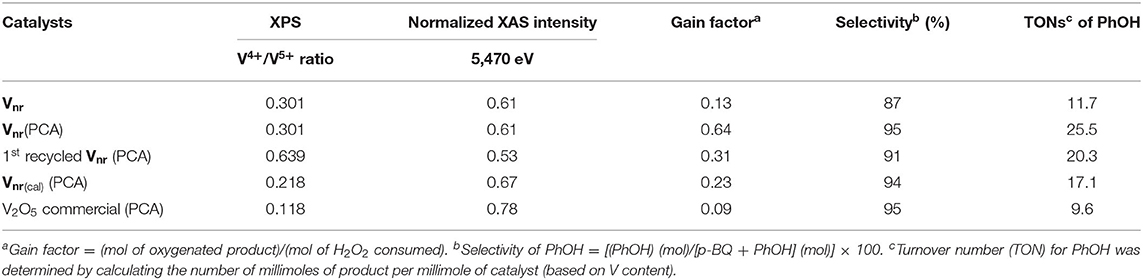
Table 1. The summary of X-ray photoelectron spectroscopy (XPS) and X-ray absorption spectroscopy (XAS) data of vanadium oxide catalysts including Vnr and its reactivity parameters.
Vnr catalyst can also conduct toluene oxidation and its selective sp2 C–H bond oxidation or π-activation for cresols/methyl-p-BQ formation up to 52% assisted by PCA (Wanna et al., 2019a). For 48% sp3 C–H bond oxidation products, in comparison with the product ratio by the addition of PPh3 after the reaction, the ratios for benzaldehyde and benzyl hydroperoxide were identified to be 23 and 25%, respectively (Supplementary Table 2, Supplementary Information). In addition, high NIH rearrangement ratio of 80% suggests that the reaction mechanism for sp2 C–H bond oxidation/π activation of toluene, presumably, also contributed partly to the catalytic oxidation of benzene to phenol, mainly via an electrophilic OAT of vanadium hydroperoxide or high valent vanadium oxo intermediates. The oxidation of benzene to PhOH catalyzed by Vnr catalyst using O2(aq) as an oxidant displayed 68% 18O-enriched PhOH; however, there was negligible enrichment using O2 and 18O2. The results implicate H2O2(aq) as the essential oxidant for OAT mediated by the vanadium oxygenated species.
Characterizations and Common Features of the Fe/Cu/V Nano-Catalysts
The Fe, Cu, and V oxide nano-catalysts as a type of metal oxide material possessed metal-oxygen/hydroxide components that can be polymerized and form active hybrid materials with oxygenated hydrocarbons (Supplementary Figures 1–7, Supplementary Information). N2 adsorption–desorption isotherms and XRD studies reveal the porous structure and crystalline feature of these catalysts that formed from the core metal oxide with the organic linker as a framework. These three catalysts similarly possessed mesoporous structure in a pore size range of 1.5–30 nm and BET surface area of each was in the range of 20–30 m2/g that can be essential for catalytic activation of the small aromatic compounds. The mesopore structure of the catalysts has a type of slit-like pore formed by the effect of substrate (benzene/toluene) acting as template while preparing in situ (Rouquerol et al., 1994; Thommes et al., 2015; Wanna et al., 2019a,b). The porous property observed in these Fe/Cu/V nano-catalysts contributed to the improvement of benzene oxidation efficiency using H2O2(aq) with additional H2O proportions in CH3CN (Ramu et al., 2017; Wanna et al., 2019b), which is consistent with the effect of water-assisted benzene oxidation catalyzed by the molecular iron complex encapsulated in the zeolite (Yamaguchi et al., 2015). In addition, the powder XRD and selected area (electron) diffraction SAD-TEM analysis for Vnr catalyst indicated that the crystal grew longer to rod shape with preferential (220) orientation that can be crucial for the related additional activities (Berenguer et al., 2017). The crystalline feature of Fe and Cu catalysts also exhibited a distinctive crystal arrangement that directly corresponded to the nature of the parental metal-oxide materials.
Thermogravimetric analysis study of the organic composition of the nano-catalysts indicates that it accounts for 10–50% and plays key roles for structural framework formation and selective catalytic activity (Supplementary Figures 3, 5, 7, Supplementary Information) (Zoubi et al., 2009; Wanna et al., 2019a,b). Calcination of these catalysts at 500°C under N2 completely decomposes the organic linker, collapses the structural framework, and changes the surface morphology that causes a decline in catalytic activity significantly. In the XPS investigation of the catalysts' surface, beside the common metal-oxide/hydroxide functional groups, we identified a hydrocarbon group that offers hydrophobic surfaces (Wanna et al., 2019a,b) that are crucial for high dispersion of the nanoparticles and surface–substrate interaction (Wu et al., 2006; Biesinger et al., 2007; Dong et al., 2016). The central metal-oxide cluster in support with the organic linker forms the surface atomic structure that can be active for catalytic application. In addition to this, XPS and XAS studies show coexistence of the mixed valance states such as Fe2+/Fe3+, Cu+/Cu2+, and V4+/V5+ that are essential for C–H or π-bond activation.
Discussion
Overall, the study provides an efficient strategy that accumulates active Fe, Cu, and V oxide species of organic–inorganic hybrid nano-catalysts, respectively, through the addition of 35% H2O2(aq) to the Fe/Cu perchlorate and VCl3 in CH3CN for the selective oxidation of simple aromatics (Ramu et al., 2017; Wanna et al., 2019a,b). Interestingly, these metal nanoparticles can be further recycled several times that can carry out the selective catalytic oxidation of benzene to PhOH or p-BQ and toluene to cresols/methyl-p-BQ (sp2 C–H bond oxidation) or benzyl alcohol/benzaldehyde (sp3 C–H bond oxidation). This indicates that, in the presence of organic residues including CH3CN, benzene, and/or toluene, the oxidant of H2O2(aq) can not only accumulate HO·/HOO· but is also essential for the self-assembly of the heterogeneous metal-oxide hybrid catalyst formation with active metal oxygenated species.
CH3CN is a polar solvent that can be miscible with polar H2O2 and H2O as well as the non-polar aromatic substrates, leading to oxygenate formation. When the metal salts dissolved in the reaction mixtures of aromatics, the free Cu/Fe/V ions presumably coordinate with CH3CN serving as a ligand to form metal complexes (Rach and Kühn, 2009; Kulakova et al., 2017). As Vnr catalyst can be directly prepared from H2O2(aq) in CH3CN without any additional substrates (Wanna et al., 2019a), we therefore surmise that these CH3CN-based metal complexes in the presence of H2O2(aq) can occur and are essential to assist the metal oxide polymerization/formation.
In addition, it has already been known that catalytic aromatic hydroxylation with H2O2(aq) is the cause of further oxidation of initially formed phenols and the appearance of tar (Olah et al., 1981; Kholdeeva, 2015). As long as we take into consideration this additional factor in a heterogeneous platform for the metal catalyzed oxidation or the reaction condition for Fenton's chemistry, the accumulated ROS exerted on the oxide surface may not be considered as diffusible radicals anymore. The Cu and Fe nanoparticles can achieve the oxidation of toluene for o,p-cresols in 75% selectivity whereas for the Vnr materials, it is ~52%. The NIH-shift ratios obtained from the mechanistic probe of [4-2H0, 1]toluene catalyzed by Fe, Cu, and V nano-catalysts are ~85, 57, and 80%, respectively (Ramu et al., 2017; Wanna et al., 2019a,b). The results further support that the oxidation using H2O2(aq) in CH3CN catalyzed by the metal oxide nano-catalysts can give rise to reactivity from the specific FeIV = O species on poly-iron oxo clusters (Enami et al., 2014) as evidenced from EM studies, tri-copper cluster moiety that existed in the copper oxide assembled nanoparticles (Wanna et al., 2019b), and crucial V4+/V5+ redox sites stabilized by PCA (Wanna et al., 2019a), which can significantly undergo OAT process for aromatics activation via the arene oxide intermediates (Guroff et al., 1967; Jerina and Daly, 1974; Bassan et al., 2003).
In summary, highly dispersed active Fe, Cu, and V nano-catalysts with H2O2/O2, in a heterogeneous platform, can drive selective oxidation of simple aromatics such as benzene and toluene. Furthermore, these unique catalysts effectively tuned sp2 vs. sp3 C–H bond hydroxylation of toluene and achieved selective double oxidation of benzene to p-BQ. In many cases, the reaction mechanism proceeds significantly through OAT process via the formation of the arene oxide intermediate, although the free radicals that initiated oxidation of C–H bond also participate. These nano-catalysts prepared in situ and composed of organic–inorganic hybrid exhibited recyclable properties, controlled selectivity, higher activity, and were greener than their bulk oxide counterparts, which make them potentially promising for large-scale application.
Author Contributions
WW, DJ, NT, and RR conducted the research of catalytic oxidative conversion of simple aromatics. WW, DJ, NT, RR, and Y-FT carried out the identification and characterization of the metal oxide materials. SY organized the research. WW, DJ, NT, and SY wrote the paper. All authors contributed to the article and approved the submitted version.
Funding
This work was supported by Academia Sinica, Taiwan (AS-iMATE-107-93), and grants from the Taiwan's Deep Decarbonization Pathways toward a Sustainable Society research program (AS-KPQ-106-DDPP) and the Ministry of Science and Technology, Taiwan (MOST 107-2113-M-001-029 and 108-2113-M-001-006).
Conflict of Interest
The authors declare that the research was conducted in the absence of any commercial or financial relationships that could be construed as a potential conflict of interest.
Acknowledgments
We are grateful to Dr. Jyh-Fu Lee, Dr. Chih-Wen Pao, Dr. Jeng-Lung Chen, and staffs of the National Synchrotron Radiation Research Center, Hsinchu, Taiwan, for their kind assistance with the X-ray absorption spectroscopy and powder X-ray diffraction studies.
Supplementary Material
The Supplementary Material for this article can be found online at: https://www.frontiersin.org/articles/10.3389/fchem.2020.589178/full#supplementary-material
References
Andreozzi, R., Caprio, V., Insola, A., and Marotta, R. (1999). Advanced oxidation processes (AOP) for water purification and recovery. Catal. Today 53, 51–59. doi: 10.1016/S0920-5861(99)00102-9
Bassan, A., Blomberg, M. R. A., and Siegbahn, P. E. M. (2003). Mechanism of aromatic hydroxylation by an activated FeIV=O core in tetrahydrobiopterin-dependent hydroxylases. Chem. Eur. J. 9, 4055–4067. doi: 10.1002/chem.200304768
Berenguer, R., Guerrero-Pérez, M. O., Guzmán, I., Rodríguez-Mirasol, J., and Cordero, T. (2017). Synthesis of vanadium oxide nanofibers with variable crystallinity and V5+/V4+ Ratios. ACS Omega 2, 7739–7745. doi: 10.1021/acsomega.7b01061
Biesinger, M. C., Hart, B. R., Polack, R., Kobe, B. A., and Smart, R. S. C. (2007). Analysis of mineral surface chemistry in flotation separation using imaging XPS. Min. Eng. 20, 152–162. doi: 10.1016/j.mineng.2006.08.006
Bilyachenko, A. N., Levitsky, M. M., Yalymov, A. I., Korlyukov, A. A., Khrustalev, V. N., Vologzhanina, A. V., et al. (2016). Cage-like Fe,Na-germsesquioxanes: structure, magnetism, and catalytic activity. Angew. Chem. Int. Ed. 55, 15360–15363. doi: 10.1002/anie.201607189
Bossmann, S. H., Oliveros, E., Göb, S., Siegwart, S., Dahlen, E. P., Payawan, L., et al. (1998). New evidence against hydroxyl radicals as reactive intermediates in the thermal and photochemically enhanced fenton reactions. J. Phys. Chem. A 102, 5542–5550. doi: 10.1021/jp980129j
Boyd, D. R., Daly, J. W., and Jerina, D. M. (1972). Rearrangement of (1-2H)- and (2-2H)naphthalene 1,2-oxides to 1-naphthol. Mechanism of the NIH shift. Biochemistry 11, 1961–1966. doi: 10.1021/bi00760a035
Castle, L., Lindsay Smith, J. R., and Buxton, G. V. (1980). Aromatic hydroxylation by oxy-radicals — a possible model for hydroxylation catalysed by cytochrome P450. J. Mol. Catal. 7, 235–243. doi: 10.1016/0304-5102(80)85021-8
Chan, S. I., Lu, Y. J., Nagababu, P., Maji, S., Hung, M. C., Lee, M. M., et al. (2013). Efficient oxidation of methane to methanol by dioxygen mediated by tricopper clusters. Angew. Chem. Int. Ed. 52, 3731–3735. doi: 10.1002/anie.201209846
Chen, P. P. Y., and Chan, S. I. (2006). Theoretical modeling of the hydroxylation of methane as mediated by the particulate methane monooxygenase. J. Inorg. Biochem. 100, 801–809. doi: 10.1016/j.jinorgbio.2005.12.014
Chen, Y. S., Luo, W. I., Yang, C. L., Tu, Y. J., Chang, C. W., Chiang, C. H., et al. (2014). Controlled oxidation of aliphatic C-H bonds in metallo-monooxygenases: mechanistic insights derived from studies on deuterated and fluorinated hydrocarbons. J. Inorg. Biochem. 134, 118–133. doi: 10.1016/j.jinorgbio.2014.02.005
de Visser, S. P., and Shaik, S. (2003). A proton-shuttle mechanism mediated by the porphyrin in benzene hydroxylation by cytochrome P450 enzymes. J. Am. Chem. Soc. 125, 7413–7424. doi: 10.1021/ja034142f
Deng, Y., and Zhao, R. (2015). Advanced oxidation processes (AOPs) in wastewater treatment. Curr. Pollut. Rep. 1, 167–176. doi: 10.1007/s40726-015-0015-z
Dong, Y., Niu, X., Song, W., Wang, D., Chen, L., Yuan, F., et al. (2016). Facile synthesis of vanadium oxide/reduced graphene oxide composite catalysts for enhanced hydroxylation of benzene to phenol. Catalysts 6:74. doi: 10.3390/catal6050074
Enami, S., Sakamoto, Y., and Colussi, A. J. (2014). Fenton chemistry at aqueous interfaces. Proc. Nat. Acad. Sci. U.S.A. 111, 623–628. doi: 10.1073/pnas.1314885111
Guroff, G., Renson, J., Udenfriend, S., Daly, J. W., Jerina, D. M., and Witkop, B. (1967). Hydroxylation-induced migration: the NIH Shift. Science 157, 1524–1530. doi: 10.1126/science.157.3796.1524
Jerina, D. M., and Daly, J. W. (1974). Arene oxides: a new aspect of drug metabolism. Science 185, 573–582. doi: 10.1126/science.185.4151.573
Kamata, K., Yamaura, T., and Mizuno, N. (2012). Chemo- and regioselective direct hydroxylation of arenes with hydrogen peroxide catalyzed by a divanadium-substituted phosphotungstate. Angew. Chem. Int. Ed. 51, 7275–7278. doi: 10.1002/anie.201201605
Kholdeeva, O. A. (2015). “Selective Oxidation of Aromatics Rings,” in Arene Chemistry: Reaction Mechanisms and Methods for Aromatic Compounds, 1st Edn, ed J. Mortier (Hoboken, NJ: John Wiley & Sons, Inc.), 365–398. doi: 10.1002/9781118754887.ch14
Kirillov, A. M., and Shul'pin, G. B. (2013). Pyrazinecarboxylic acid and analogs: highly efficient co-catalysts in the metal-complex-catalyzed oxidation of organic compounds. Coord. Chem. Rev. 257, 732–754. doi: 10.1016/j.ccr.2012.09.012
Kirillova, M. V., Kuznetsov, M. L., Romakh, V. B., Shul'pina, L. S., Fraústo Da Silva, J. J. R., Pombeiro, A. J. L., et al. (2009). Mechanism of oxidations with H2O2 catalyzed by vanadate anion or oxovanadium(V) triethanolaminate (vanadatrane) in combination with pyrazine-2-carboxylic acid (PCA): kinetic and DFT studies. J. Catal. 267, 140–157. doi: 10.1016/j.jcat.2009.08.006
Kudrik, E. V., and Sorokin, A. B. (2008). N-Bridged diiron phthalocyanine catalyzes oxidation of benzene with H2O2 via benzene oxide with NIH shift evidenced by using 1,3,5-[D3]benzene as a probe. Chem. Eur. J. 14, 7123–7126. doi: 10.1002/chem.200800504
Kulakova, A. N., Bilyachenko, A. N., Levitsky, M. M., Khrustalev, V. N., Korlyukov, A. A., Zubavichus, Y. V., et al. (2017). Si10Cu6N4 cage hexacoppersilsesquioxanes containing N ligands: synthesis, structure, and high catalytic activity in peroxide oxidations. Inorg. Chem. 56, 15026–15040. doi: 10.1021/acs.inorgchem.7b02320
Kulakova, A. N., Korlyukov, A. A., Zubavichus, Y. V., Khrustalev, V. N., Bantreil, X., Shul'pina, L. S., et al. (2019). Hexacoppergermsesquioxanes as complexes with N-ligands: synthesis, structure and catalytic properties. J. Organometal. Chem. 884, 17–28. doi: 10.1016/j.jorganchem.2019.01.004
Kurata, T., Watanabe, Y., Katoh, M., and Sawaki, Y. (1988). Mechanism of aromatic hydroxylation in the Fenton and related reactions. One-electron oxidation and the NIH shift. J. Am. Chem. Soc. 110, 7472–7478. doi: 10.1021/ja00230a032
Lewis, E. A., and Tolman, W. B. (2004). Reactivity of dioxygen–copper systems. Chem. Rev. 104, 1047–1076. doi: 10.1021/cr020633r
Li, X., Xue, H., Lin, Q., and Yu, A. (2020). Amphiphilic poly(ionic liquid)/wells–dawson-type phosphovanadomolybdate ionic composites as efficient and recyclable catalysts for the direct hydroxylation of benzene with H2O2. Appl. Organometal. Chem. 34:e5606. doi: 10.1002/aoc.5606
Liu, M. S., Su, B., Tang, Y., Jiang, X. C., and Yu, A. B. (2017). Recent advances in nanostructured vanadium oxides and composites for energy conversion. Adv. Energy Mater. 7:1700885. doi: 10.1002/aenm.201700885
Mirica, L. M., Ottenwaelder, X., and Stack, T. D. P. (2004). Structure and spectroscopy of copper–dioxygen complexes. Chem. Rev. 104, 1013–1046. doi: 10.1021/cr020632z
Mitchell, K. H., Rogge, C. E., Gierahn, T., and Fox, B. G. (2003). Insight into the mechanism of aromatic hydroxylation by toluene 4-monooxygenase by use of specifically deuterated toluene and p-xylene. Proc. Natl. Acad. Sci. U.S.A. 100, 3784–3789. doi: 10.1073/pnas.0636619100
Mori, K., Kagohara, K., and Yamashita, H. (2008). Synthesis of tris(2,2'-bipyridine)iron(II) complexes in zeolite Y cages: influence of exchanged alkali metal cations on physicochemical properties and catalytic activity. J. Phys. Chem. C 112, 2593–2600. doi: 10.1021/jp709571v
Morimoto, Y., Bunno, S., Fujieda, N., Sugimoto, H., and Itoh, S. (2015). Direct hydroxylation of benzene to phenol using hydrogen peroxide catalyzed by nickel complexes supported by pyridylalkylamine ligands. J. Am. Chem. Soc. 137, 5867–5870. doi: 10.1021/jacs.5b01814
Nagababu, P., Maji, S., Kumar, M. P., Chen, P. P. Y., Yu, S. S. F., and Chan, S. I. (2012). Efficient room-temperature oxidation of hydrocarbons mediated by tricopper cluster complexes with different ligands. Adv. Synth. Catal. 354, 3275–3282. doi: 10.1002/adsc.201200474
Nguyen, H. H. T., Shiemke, A. K., Jacobs, S. J., Hales, B. J., Lidstrom, M. E., and Chan, S. I. (1994). The nature of the copper ions in the membranes containing the particulate methane monooxygenase from Methylococcus capsulatus (Bath). J. Biol. Chem. 269, 14995–15005.
Olah, G. A., Fung, A. P., and Keumi, T. (1981). Oxyfunctionalization of hydrocarbons. 11. hydroxylation of benzene and alkylbenzenes with hydrogen peroxide in hydrogen fluoride/boron trifluoride. J. Org. Chem. 46, 4305–4306. doi: 10.1021/jo00334a046
Ottenbacher, R. V., Talsi, E. P., and Bryliakov, K. P. (2020). Recent progress in catalytic oxygenation of aromatic C–H groups with the environmentally benign oxidants H2O2 and O2. Appl. Organometal. Chem. e. 34:e5900. doi: 10.1002/aoc.5900
Ottenwaelder, X., Rudd, D. J., Corbett, M. C., Hodgson, K. O., Hedman, B., and Stack, T. D. P. (2006). Reversible O–O bond cleavage in copper–dioxygen isomers: impact of anion basicity. J. Am. Chem. Soc. 128, 9268–9269. doi: 10.1021/ja061132g
Pignatello, J. J., Oliveros, E., and Mackay, A. (2006). Advanced oxidation processes for organic contaminant destruction based on the fenton reaction and related chemistry. Crit. Rev. Environ. Sci. Technol. 36, 1–84. doi: 10.1080/10643380500326564
Raba, A., Cokoja, M., Herrmann, W. A., and Kuhn, F. E. (2014). Catalytic hydroxylation of benzene and toluene by an iron complex bearing a chelating di-pyridyl-di-NHC ligand. Chem. Commun. 50, 11454–11457. doi: 10.1039/C4CC02178A
Rach, S. F., and Kühn, F. E. (2009). Nitrile ligated transition metal complexes with weakly coordinating counteranions and their catalytic applications. Chem. Rev. 109, 2061–2080. doi: 10.1021/cr800270h
Ramu, R., Wanna, W. H., Janmanchi, D., Tsai, Y. F., Liu, C. C., Mou, C. Y., et al. (2017). Mechanistic study for the selective oxidation of benzene and toluene catalyzed by Fe(ClO4)2 in an H2O2-H2O-CH3CN system. Mol. Catal. 441, 114–121. doi: 10.1016/j.mcat.2017.08.006
Rouquerol, J., Avnir, D., Fairbridge, C. W., Everett, D. H., Haynes, J. M., Pernicone, N., et al. (1994). Recommendations for the characterization of porous solids (Technical Report). Pure Appl. Chem. 66:1739. doi: 10.1351/pac199466081739
Sawyer, D. T., Sobkowiak, A., and Matsushita, T. (1996). Metal [MLx; M = Fe, Cu, Co, Mn]/hydroperoxide-Induced activation of dioxygen for the oxygenation of hydrocarbons: oxygenated fenton chemistry. Acc. Chem. Res. 29, 409–416. doi: 10.1021/ar950031c
Shul'pin, G. B., Ishii, Y., Sakaguchi, S., and Iwahama, T. (1999). Oxidation with the “O2-H2O2–vanadium complex—pyrazine-2-carboxylic acid” reagent. Russ. Chem. Bull. 48, 887–890. doi: 10.1007/BF02494631
Shul'pin, G. B., Kozlov, Y. N., Shul'pina, L. S., and Petrovskiy, P. V. (2010). Oxidation of alkanes and alcohols with hydrogen peroxide catalyzed by complex Os3(CO)10(μ-H)2. Appl. Organometal. Chem. 24, 464–472. doi: 10.1002/aoc.1641
Shul'pina, L. S., Takaki, K., Strelkova, T. V., and Shul'pin, G. B. (2008). Benzene oxidation with hydrogen peroxide catalyzed by soluble and heterogenized copper compounds. Petro. Chem. 48, 219–222. doi: 10.1134/S0965544108030080
Siegmund, H. U., and Kaufman, S. (1991). Hydroxylation of 4-methylphenylalanine by rat liver phenylalanine hydroxylase. J. Biol. Chem. 266, 2903–2910.
Suib, S. L., Prech, J., Cejka, J., Kuwahara, Y., Mori, K., and Yamashita, H. (2020). Some novel porous materials for selective catalytic oxidations. Mater. Today 32, 244–259. doi: 10.1016/j.mattod.2019.06.008
Thibon, A., Jollet, V., Ribal, C., Sénéchal-David, K., Billon, L., Sorokin, A. B., et al. (2012). Hydroxylation of aromatics with the help of a non-haem FeOOH: a mechanistic study under single-turnover and catalytic conditions. Chem. Eur. J. 18, 2715–2724. doi: 10.1002/chem.201102252
Thommes, M., Kaneko, K., Neimark, A. V., Olivier, J. P., Rodriguez-Reinoso, F., Rouquerol, J., et al. (2015). Physisorption of gases, with special reference to the evaluation of surface area and pore size distribution (IUPAC Technical Report). Pure Appl. Chem. 87:1051. doi: 10.1515/pac-2014-1117
Walling, C. (1975). Fenton's reagent revisited. Acc. Chem. Res. 8, 125–131. doi: 10.1021/ar50088a003
Wang, V. C. C., Maji, S., Chen, P. P. Y., Lee, H. K., Yu, S. S. F., and Chan, S. I. (2017). Alkane oxidation: methane monooxygenases, related enzymes, and their biomimetics. Chem. Rev. 117, 8574–8621. doi: 10.1021/acs.chemrev.6b00624
Wanna, W. H., Janmanchi, D., Thiyagarajan, N., Ramu, R., Tsai, Y. F., Pao, C. W., et al. (2019a). Selective catalytic oxidation of benzene to phenol by a vanadium oxide nanorod (Vnr) catalyst in CH3CN using H2O2(aq) and pyrazine-2-carboxylic acid (PCA). New J. Chem. 43, 17819–17830. doi: 10.1039/C9NJ02514F
Wanna, W. H., Ramu, R., Janmanchi, D., Tsai, Y. F., Thiyagarajan, N., and Yu, S. S. F. (2019b). An efficient and recyclable copper nano-catalyst for the selective oxidation of benzene to p-benzoquinone (p-BQ) using H2O2(aq) in CH3CN. J. Catal. 370, 332–346. doi: 10.1016/j.jcat.2019.01.005
Wu, C. K., Yin, M., O'brien, S., and Koberstein, J. T. (2006). Quantitative analysis of copper oxide nanoparticle composition and structure by X-ray photoelectron spectroscopy. Chem. Mater. 18, 6054–6058. doi: 10.1021/cm061596d
Yalymov, A. I., Bilyachenko, A. N., Levitsky, M. M., Korlyukov, A. A., Khrustalev, V. N., Shul'pina, L. S., et al. (2017). High catalytic activity of heterometallic (Fe6Na7 and Fe6Na6) cage silsesquioxanes in oxidations with peroxides. Catalysts 7:101. doi: 10.3390/catal7040101
Yamaguchi, S., Ohnishi, T., Miyake, Y., and Yahiro, H. (2015). Effect of water added into acetonitrile solvent on oxidation of benzene with hydrogen peroxide over iron complexes encapsulated in zeolite. Chem. Lett. 44, 1287–1288. doi: 10.1246/cl.150479
Yang, C. L., Lin, C. H., Luo, W. I., Lee, T. L., Ramu, R., Ng, K. Y., et al. (2017). Mechanistic study of the stereoselective hydroxylation of [2-2H1,3-2H1] butanes catalyzed by cytochrome P450 BM3 variants. Chem. Eur. J. 23, 2571–2582. doi: 10.1002/chem.201603956
You, X., Wei, Z., Wang, H., Li, D., Liu, J., Xu, B., et al. (2014). Synthesis of two copper clusters and their catalysis towards the oxidation of benzene into phenol. RSC Adv. 4, 61790–61798. doi: 10.1039/C4RA12832J
Zhang, X., Zhang, T., Li, B., Zhang, G., Hai, L., Ma, X., et al. (2017). Direct synthesis of phenol by novel [FeFe]-hydrogenase model complexes as catalysts of benzene hydroxylation with H2O2. RSC Adv. 7, 2934–2942. doi: 10.1039/C6RA27831K
Zhao, X. T., Yan, Y. Y., Mao, L., Fu, M. C., Zhao, H. R., Sun, L. S., et al. (2018). A relationship between the V4+/V5+ ratio and the surface dispersion, surface acidity, and redox performance of V2O5-WO3/TiO2 SCR catalysts. RSC Adv. 8, 31081–31093. doi: 10.1039/C8RA02857E
Zhou, T., Zhang, J., Ma, Y. Y., Gao, X., Xue, Q., Gao, Y., et al. (2020). A bicadmium-substituted polyoxometalate network based on a vanadosilicate cluster for the selective oxidation of styrene to benzaldehyde. Inorg. Chem. 59, 5803–5807. doi: 10.1021/acs.inorgchem.0c00072
Keywords: benzene, phenol, p-benzoquinone, hydrogen peroxide, toluene, metal oxide, nanocatalysts
Citation: Wanna WH, Janmanchi D, Thiyagarajan N, Ramu R, Tsai Y-F and Yu SSF (2020) Selective Oxidation of Simple Aromatics Catalyzed by Nano-Biomimetic Metal Oxide Catalysts: A Mini Review. Front. Chem. 8:589178. doi: 10.3389/fchem.2020.589178
Received: 30 July 2020; Accepted: 14 September 2020;
Published: 26 October 2020.
Edited by:
Manas Sutradhar, Instituto Superior Técnico, PortugalReviewed by:
Alexey Bilyachenko, A. N. Nesmeyanov Institute of Organoelement Compounds (RAS), RussiaShilpi Ghosh, University of Marburg, Germany
Copyright © 2020 Wanna, Janmanchi, Thiyagarajan, Ramu, Tsai and Yu. This is an open-access article distributed under the terms of the Creative Commons Attribution License (CC BY). The use, distribution or reproduction in other forums is permitted, provided the original author(s) and the copyright owner(s) are credited and that the original publication in this journal is cited, in accordance with accepted academic practice. No use, distribution or reproduction is permitted which does not comply with these terms.
*Correspondence: Steve S. F. Yu, c2Z5dSYjeDAwMDQwO2dhdGUuc2luaWNhLmVkdS50dw==
†These authors have contributed equally to this work