- Department of Chemistry, Physics and Atmospheric Sciences, Jackson State University, Jackson, MS, United States
Two rationally designed 4-nitrophenyl-based molecular clefts functionalized with thiourea (L1) and urea (L2) have been synthesized and studied for a variety of anions by UV-Vis and colorimetric techniques in DMSO. Results from the binding studies suggest that both L1 and L2 bind halides showing the order: fluoride > chloride > bromide > iodide; and oxoanions showing the order: dihydrogen phosphate > hydrogen sulfate > nitrate > perchlorate. Each receptor has been shown to form a 1:1 complex with an anion via hydrogen bonding interactions, displaying distinct color change for fluoride and dihydrogen phosphate in solution. As compared to the urea-based receptor L2, the thiourea-based receptor L1 exhibits stronger affinity for anions due the presence of more acidic thiourea functional groups.
Introduction
Because of the fundamental role played by anions in biological, industrial, and environmental processes, selective binding and sensing of anions by synthetic receptors has become one of the most extensively studied areas in supramolecular chemistry (Sessler et al., 2008; Bowman-James, 2005). As anions are vital in a wide range of chemical research such as functional materials, transmembrane transports, and catalysis, the anion binding chemistry has been proven to be an immense area of research interest to the scientific community (Wiskur et al., 2001; Martinez-Manez et al., 2003; Gale et al., 2008; Caltagirone et al., 2009; Gale et al., 2010; Wenzel et al., 2012).
Among the various anions, halides and oxoanions have a great impact on both human health and environment. For example, an elevated amount of fluoride in drinking water causes dental and skeletal fluorosis (Ayoob and Gupta, 2006). Fluoride has an adverse effect in human body, resulting in a bone cancer known as osteosarcoma (Ayoob and Gupta, 2006). Sulfate and phosphate anions are known to bind to proteins in the neutral environment of respective sulfate and phosphate binding proteins (Pflugrath and Quiocho, 1985; Wang et al., 1997). Dihydrogen phosphate becomes the most abundant species among the three forms of inorganic phosphates (H2PO4−, HPO42− and PO43−) and shows donor-acceptor properties similar to those of the water molecule. Hence, the recognition and detection of these key inorganic anions by efficient artificial sensors is much anticipated. In a recent report, it was shown that C3v-symmetric receptors possessing both H-bond donor and acceptor exhibited high selectivity for H2PO4− over other common inorganic anions (Park et al., 2016; Kim, et al., 2018; Kim, et al., 2019). It is now documented that a urea or thiourea group is capable to chelate an anion via two directional H-bonds (Jia et al., 2016). Therefore, the molecules containing (thio)urea moieties with flexible (Nishizawa et al., 1995; Nishizawa et al., 1998; Emgenbroich et al., 2008; Duke et al., 2012; Caltagirone et al., 2013; Olivari et al., 2015; Gillen et al., 2018) or rigid (Manna et al., 2016; Manna and Das 2017; Khansari et al., 2017b; Manna et al., 2017; Gale and Gunnlaugsson, 2010) linkers have been successfully applied as a promising class of receptors for anion recognition purpose in recent past years. Nishizawa et al. (1995) reported bis(thio)urea receptors containing n-butyl terminals with a flexible meta-xylylenediamine-based linker, exhibiting preferable binding for H2PO4− and SO42− over other anions as evaluated by NMR studies. The same group also synthesized phenyl-substituted ureas as an ionophore for sulfate selective electrode (Nishizawa et al., 1998). Emgenbroich et al. (2008) synthesized a meta-xylylenediamine-based vinylphenyl urea, showing good interactions for tyrosine phosphorylated peptides. Caltagirone et al. (2013) described pyrophosphate optical sensing properties of meta-xylylenediamine derivatives bearing naphthalene and 2-nitrobenzene moieties. Further studies on similar types of receptors indicated that such receptors can be useful for selective anion transports (Olivari et al., 2015). Li et al. (2013) reported ortho-phenylene connected bis-thio(urea) receptors exhibiting interactions with oxoanions, while both bisurea and thiourea were shown to form tris-chelate complexes with phosphate ion (PO43−) in the solid state. C3v-symmetric tripodal receptors with various flexible and rigid frameworks have been shown to exhibit for the chromogenic and fluorogenic sensing of anions through the formation of hydrogen bonded host-guest complex followed by the deprotonation of amide-NH protons (Kim et al., 2015; Kim et al., 2016; Sahoo et al., 2016).
In an effort to develop new colorimetric chemosensors for naked eye detection of anions (Basaran et al., 2014; Haque et al., 2015; Rhaman et al., 2018; Rhaman et al., 2020; Portis et al., 2020), we have synthesized two simple meta-xylylene-based molecular clefts: one containing thiourea (L1) and other containing urea (L2) functionalities (Scheme 1). Herein we report the synthesis of these receptors and their binding behavior for halides and oxoanions, which exhibit preferential binding as well as visible color change for fluoride and phosphate in DMSO. Due to the presence of more acidic thiourea groups in L1 as compared to its urea analogue L2, the former exhibits stronger interactions for anions than its counterpart.
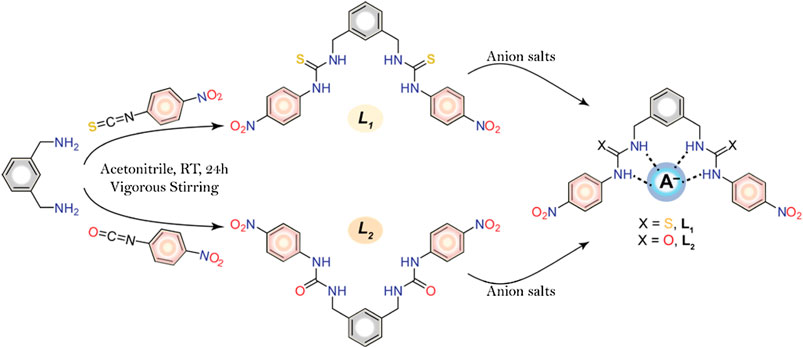
SCHEME 1. Synthetic route of the receptors and schematic diagram of anion-receptor binding through probable H-bonding interactions.
Material and Methods
Materials
All reagents and solvents were purchased from commercial suppliers and used without further purification. Nuclear magnetic resonance (NMR) spectra were recorded at 25 °C on a Varian Unity INOVA 500 FT-NMR instrument and the chemical shifts were recorded in parts per million (ppm) using tetramethylsilane as a reference. All NMR data were processed and analyzed with MestReNova software. The following abbreviations in 1H NMR characterization are used to describe spin multiplicities: s = singlet; d = doublet; t = triplet; and m = multiplet. The absorption spectra for UV-Vis screening and titrations were performed in DMSO at room temperature using a UV-2600 spectrophotometer (SHIMADZU). Elemental analysis was carried out using an ECS 4010 Analytical Platform (Costech Instrument) at Jackson State University.
Synthesis
The receptors were successfully synthesized (Scheme 1) by the reaction of m-xylylenediamine (0.5 ml, 3.7 mmol) with 4-nitrophenyl isothiocyanate (1.3 g, 7.7 mmol) for L1 or 4-nitrophenyl isocyanate (1.2 g, 7.7 mmol) for and L2 in dry acetonitrile under constant stirring at room temperature. After overnight stirring, the yellowish precipitate was filtered off and washed with acetonitrile and THF. The residues were dried under vacuum to give L1 (88% yield) and L2 (92% yield).
L1: 1H NMR (500 MHz, DMSO-d6) δ (ppm): 4.77 (s, 4H, xylyl–CH2), 5.76 (s, 1H, Ar–H), 7.26–7.27 (d, 2H, ∼7.5 Hz, Ar–H), 7.33–7.36 (t, 1H, ∼7.0 Hz, Ar–H), 7.82–7.84 (d, 4H, ∼9.0 Hz, Ar–H), 8.15 (d, 4H, ∼9.0 Hz, Ar–H), 8.71 (s, 2H, NHa), 10.26 (s, 2H, NHb).
13C NMR (125 MHz, DMSO-d6) δ (ppm): 47.5 (xylyl–CH2), 120.0 (Ar–C), 124.9 (Ar–C), 126.9 (Ar–C), 128.9 (Ar–C), 138.9 (Ar–C), 180.7 (C=S). IR (KBr, ν/cm−1): 3,334, 3,190, 2,930, 1,594, 1,533, 1,506, 1,326, 1,303, 1,245. Anal. Calcd. for C22H20N6O4S2: C, 53.21; H, 4.06; N, 16.92. Found: C, 53.25; H, 4.07; N, 16.88.
L2: 1H NMR (500 MHz, DMSO-d6) δ (ppm): 4.774 (s, 4H, Xylyl–CH2), 5.760 (s, 1H, Ar–H), 7.259–7.272 (d, 2H, ∼6.5 Hz, Ar–H), 7.335–7.356 (t, 1H, ∼6.0 Hz, Ar–H), 7.825–7.838 (d, 4H, ∼6.5 Hz, Ar–H), 8.149–8.162 (d, 4H, ∼6.5 Hz, Ar–H), 8.717 (s, 2H, NHa), 10.270 (s, 2H, NHb).
13C NMR (125 MHz, DMSO-d6) δ (ppm): 44.5 (xylyl–CH2), 118.2.0 (Ar–C), 125.69 (Ar–C), 128.0 (Ar–C), 141.8 (Ar–C), 146.2 (Ar–C) 152.2 (C=S). IR (KBr, ν/cm−1): 3360, 3340, 2,940, 1734, 1,594, 1,495, 1,250, 1,207, 1,177. Anal. Calcd. for C22H20N6O6: C, 56.89; H, 4.34; N, 18.10. Found: C, 56.85; H, 4.37; N, 18.08.
UV-Vis Titration Studies
Stock solutions of various anions (1 × 10−1 M) and receptors (5 × 10−3 M) were prepared separately in DMSO. In order to obtain an optimal absorption, the solutions of L1 and L2 were diluted to 2.5 × 10−5 M. In the UV-Vis screening experiment, the samples were prepared by mixing 10 equivalents of the stock solutions of different anions into a quartz optical cell of 1 cm path length filled with 2.0 ml of individual receptor solution. In a typical titration experiment, UV abortion spectra were recorded from the gradual addition (up to 35 equivalents) of a respective anionic solution (2.5 × 10−3 M) to L1 or L2. The receptor-anion binding constant values (log K) were determined from UV-Visible titration studies using a 1:1 binding model (Hynes 1993). The error limit was less than 10%.
Results and Discussion
Synthetic Design and Binding Studies
The synthesis of molecular clefts L1 and L2 was accomplished in a single-step reaction (Scheme 1) as a pure form, providing a good yield (88–92%). The principle of designing a chemosensor is based on the fundamental features that a probe within a single framework should possess anion binding sites for recognizing a particular anion through noncovalent interactions and suitable chromophores for providing a desired absorption band at a UV-Vis wavelength. We also attempted to study the anion binding behavior of these receptors using NMR titration studies. However, the addition of fluoride or phosphate resulted into the broadening of NH resonances, thus hampering the determination of binding constants. For other anions, NMR resonances were not significant enough to calculate the binding constants.
UV-Vis Spectroscopic Studies
The presence of two nitrophenyl units as chromophores in both receptors L1 and L2 with anion binding sites, allowed us to use them as potential colorimetric probes for anions. Earlier work has proven that the nitrophenyl groups functionalized to certain receptors could act as effective chromophores for anion sensing (Jia et al., 2016; Khansari et al., 2017a). Hence, extensive UV-Vis screening experiments and colorimetric studies were performed to examine the sensitivity of the receptors for anions. Furthermore, the binding interactions of the probes for different anions were evaluated by UV-Vis titration experiments in DMSO.
As shown in Figure 1, the probes L1 and L2 in their free states showed intense bands at 355 and 357 nm, respectively in DMSO. After the addition of different anions (10 equivalents) including F−, Cl−, Br−, I−, HSO4−, H2PO4−, NO3− and ClO4− as their salts of [n-Bu4N]+ to the probes, we observed remarkable spectral changes of L1 and L2 in terms of both intensity and wavelength with fluoride and dihydrogen phosphate, as compared to other anions included in our study. This observation suggests that these two anions (fluoride and dihydrogen phosphate) are capable to induce a colorimetric response in DMSO due to the formation of a strong anion complex. The corresponding colorimetric experiments, as performed with the addition of 10 equivalents of the respective anions to L1 and L2 (2 × 10−3 M) are shown in Figure 2, displaying a sharp color change for fluoride (dark red) and dihydrogen phosphate (red), agreeing with the results of UV-Vis screening studies.
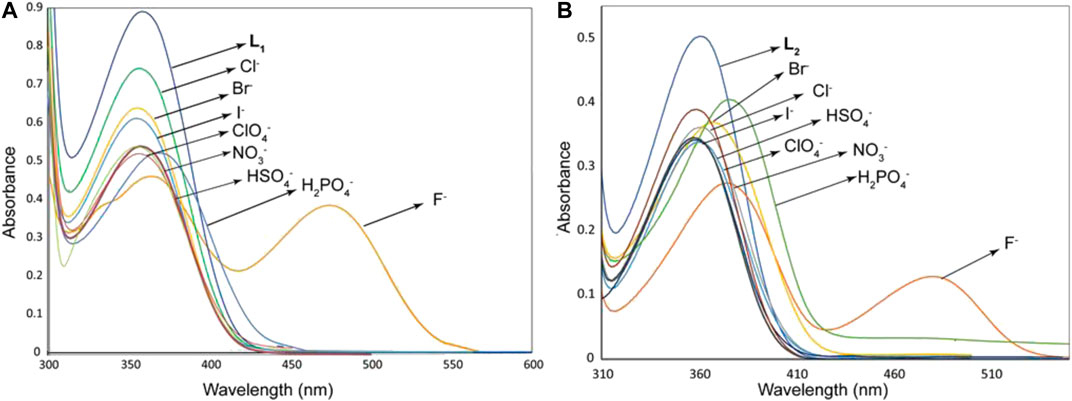
FIGURE 1. UV-Vis screening experiments of L1 (2.5 × 10−5 M) (A) and L2 (2.5 × 10−5 M) (B) with different anions (1.5 × 10−3 M) in DMSO, showing changes in the absorption bands.
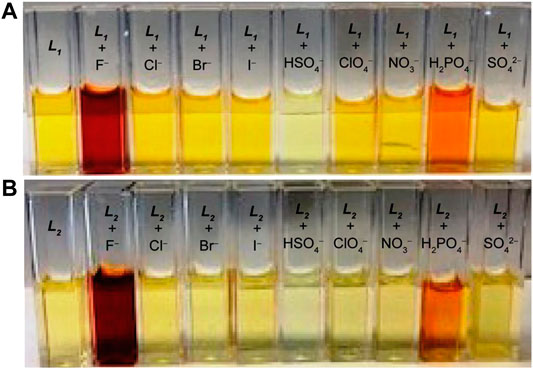
FIGURE 2. Colorimetric studies of (A) L1 and (B) L2 in the presence of different anions in DMSO, showing a color change for fluoride and dihydrogen phosphate ([host]0 = 2 × 10−3 M, [anion]0/[host]0 = 10).
The anion binding strengths of the receptors were evaluated by UV-Vis titration studies in DMSO. The titration profile of L1 with fluoride, as shown in Figure 3A, demonstrates a systematic decrease (hypochromic shift) in the absorption band at 355 nm with a red shift (Δλmax = 6–7 nm), yielding an isosbestic point at around 395 nm. A new peak appears in the visible range at around 478 nm, showing an increase in intensity (hyperchromic shift) with the concentration of fluoride, suggesting the formation of a receptor-fluoride complex. This spectral change is consistent with the visual color change (from pale yellow to deep red, Figure 2A) due to the complex formation, offering the scope for the naked eye detection of fluoride in solution. The relative absorbance I/I0 of the peak at 355 nm (where, I0 and I represent the absorbance of L1 before and after the addition of fluoride, respectively) due to the gradual addition of fluoride provided the best fit to a 1:1 binding mode, yielding a binding constant of 3.70 (in log K, Figure 3A). The acidic NH groups within the host molecule allow to bind F− within the cavity of L1, thereby forming a [L1·F]- complex through hydrogen-bonding interactions (Scheme 1). This binding constant is comparable to that reported previously with a dipodal thiourea (log K = 3.69, Khansari et al., 2014) or a tris(3-aminopropyl) amine-based tripodal thiourea (log K = 3.81, Khansari et al., 2015); however, somewhat lower than that with a tris(2-aminoethyl) amine-based tripodal thiourea (log K = 5.1, Khansari et al., 2017a).
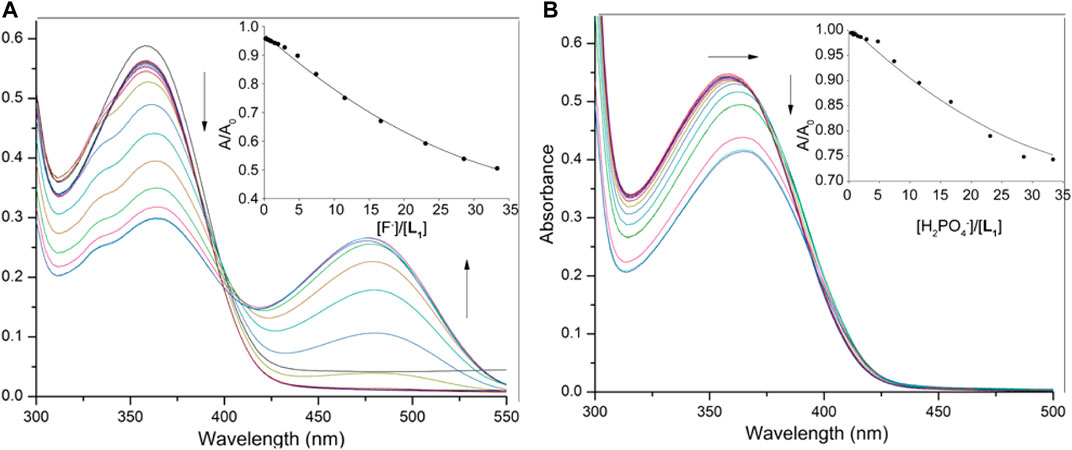
FIGURE 3. UV-Vis titration spectra of L1 (2.5 × 10−5 M) in DMSO with varying concentration of (A) fluoride (1.5 × 10−3 M), and (B) dihydrogen phosphate (1.5 × 10−3 M); titration fitting curve showing changes in the absorbance with incremental addition of F− (at λmax = 355 nm) and H2PO4− (at λmax = 358 nm) anions are shown in insets.
On titration with dihydrogen phosphate, the receptor L1 showed a similar decrease in the absorption band (λmax at 355 nm), while the peak was shifted to about λmax at 363 nm at the end of the titration (Figure 3B). However, no new peak was observed on the visible range. The change of the relative absorbance I/I0 at λmax at 355 nm was consistent with a 1:1 complex, providing a binding constant of log K = 2.82. A closely related rigid bis-thiourea receptor reported by Li et al. was shown to bind dihydrogen phosphate ion with higher affinity (log K = 3.49) may be because of more converging dipodal arms (Li et al., 2013). Continuing titrations with other anions including Cl−, Br−, I−, HSO4−, NO3− and ClO4− showed a systematic decrease in the absorption band (λmax at 355 nm). Notably, no shifting of λmax was found (Supplementary Material) which is consistent with the colorimetric results, displaying no apparent color change due to the addition of these anions to the receptor. The binding data (Table 1), as obtained from non-linear regression analysis (Hynes, 1993), suggest that the receptor binds strongly toward F− anion (log K = 3.70). Under the same condition, it binds other anions (Br−, I−, HSO4−, NO3− and ClO4−) weakly, agreeing with the reported binding trend for other neutral receptors (Khansari et al., 2017a). The higher affinity for F− is due to the fact that this anion is more basic as compared to other anions, thereby forming strong anion-complexes with the acidic thiourea groups of L1 through H-bonding interactions.
In order to assess and compare the binding constants of the urea analogue L2, we also performed UV-Vis titrations with anions, showing almost similar spectral changes as observed in the case of L1 under the same conditions (Figure 4A). The titration profile of receptor L2 with fluoride revealed a systematic decrease in the absorption band (λmax) at 357 nm followed by a red shift (Δλmax = 10–12 nm), while a new peak appeared at around 480 nm was shown to increase with the incremental addition of fluoride to the receptor. With dihydrogen phosphate, it showed a red shift (Δλmax = 15 nm) of the absorption band (λmax) at 357 nm at the end of the titration (Figure 4B). The corresponding colorimetric results, showing visible color change are again in accord with these observations (Figure 2B). The binding constants, as listed in Table 1, clearly demonstrate that both receptors exhibited a similar trend of binding for anions, showing the higher affinity for F− and H2PO4− with a binding trend of F− > H2PO4− > Cl− > HSO4− > Br− > I− > ClO4− > NO3−. It is noted that the thiourea-based receptor L1 shows an improved binding for an anion as compared to its urea analogue, accounting the enhanced acidity of NHs in L1 offered by the thiourea groups (Custelcean et al., 2005; Li et al., 2013).
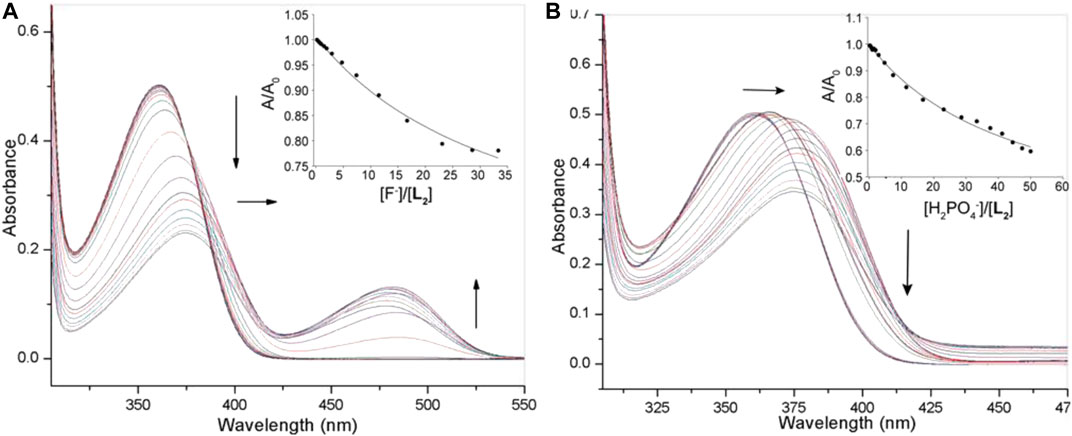
FIGURE 4. UV-Vis titration spectra of L2 (2.5 × 10−5 M) in DMSO with varying concentration of (A) fluoride (1.5 × 10−3 M), (B) dihydrogen phosphate (1.5 × 10−3 M); Titration fitting curve showing changes in the absorbance with the incremental addition of F− (at λmax = 355 nm) and H2PO4− (at λmax = 360 nm) anions are shown in insets.
Conclusion
In summary, we have synthesized two simple dipodal urea/thiourea clefts; each appended on a meta-xylyl based molecular framework and investigated their affinities toward a variety of inorganic anions. The inclusion of a nitro group as a chromophore allows the molecules to use them as effective probes for spectroscopic and visual discrimination of fluoride (from light yellow to dark red) and dihydrogen phosphate (from light yellow to red) in solution. In particular, each of the receptors exhibited strong affinities for fluoride with the binding trend for halides in the order: fluoride > chloride > bromide > iodide; and for oxoanions in the order: dihydrogen phosphate > hydrogen sulfate > nitrate > perchlorate, forming 1:1 complexes with anions by virtue of hydrogen bonding interactions. The thiourea-based receptor as compared to its urea analogue, exhibits an increased affinity for anions due the presence of enhanced acidity of thiourea units. This study could serve as an insight for the rational design of new functional chemosensors for selective sensing of anions in an application oriented field.
Data Availability Statement
The original contributions presented in the study are included in the article/Supplementary Material, further inquiries can be directed to the corresponding author.
Author Contributions
MH conceived the project idea. UM, BP, and MN synthesized and studied the compounds. TE assisted in titration studies. UM prepared the initial manuscript. All authors approved the manuscript.
Funding
The project described was supported by the US Department of Defense (Grant No. W911NF-19-1-0006).
Conflict of Interest
The authors declare that the research was conducted in the absence of any commercial or financial relationships that could be construed as a potential conflict of interest.
Acknowlegments
Analytical core facility at Jackson State University was supported by the National Institutes of Health (G12RR013459).
Supplementary Material
The Supplementary Material for this article can be found online at: https://www.frontiersin.org/articles/10.3389/fchem.2020.575701/full#supplementary-material.
References
Ayoob, S., and Gupta, A. K. (2006). Fluoride in Drinking Water: a review on the status and stress effects. Crit. Rev. Environ. Sci. Technol. 36, 433–487. doi:10.1080/10643380600678112
Basaran, I., Khansari, M. E., Pramanik, A., Wong, B. M., and Hossain, A. 2014). An exclusive fluoride receptor: fluoride-induced proton transfer to a quinoline-based thiourea. Tetrahedron Lett. 55, 1467–1470. doi:10.1016/j.tetlet.2014.01.054
Bowman-James, K. (2005). Alfred Werner revisited: the coordination chemistry of anions. Acc. Chem. Res. 38, 671–678. doi:10.1021/ar040071t
Caltagirone, C., Bazzicalupi, C., Isaia, F., Light, M. E., Lippolis, V., Montis, R., et al. (2013). A new family of bis-ureidic receptors for pyrophosphate optical sensing. Org. Biomol. Chem. 11, 2445–2451. doi:10.1039/C3OB27244C
Caltagirone, C., and Gale, P. A. (2009). Anion receptor chemistry: highlights from 2007. Chem. Soc. Rev. 38, 520–563. doi:10.1039/B806422A
Custelcean, R., Delmau, L. H., Moyer, B. A., Sessler, J. L., Cho, W. S., Gross, D., et al. (2005). Calix[4]pyrrole: an old yet new ion-pair receptor. Angew Chem. Int. Ed. Engl. 44, 2537–2542. doi:10.1002/anie.200462945
Duke, R. M., McCabe, T., Schmitt, W., and Gunnlaugsson, T. (2012). Recognition and sensing of biologically relevant anions in alcohol and mixed alcohol-aqueous solutions using charge neutral cleft-like glycol-derived pyridyl-amidothiourea receptors. J. Org. Chem. 77, 3115–3126. doi:10.1021/jo202332h
Emgenbroich, M., Borrelli, C., Shinde, S., Lazraq, I., Vilela, F., Hall, A. J., et al. (2008). A phosphotyrosine-imprinted polymer receptor for the recognition of tyrosine phosphorylated peptides. Chemistry 14, 9516–9529. doi:10.1002/chem.200801046
Gale, P. A., García-Garrido, S. E., and Garric, J. (2008). Anion receptors based on organic frameworks: highlights from 2005 and 2006. Chem. Soc. Rev. 37, 151–190. doi:10.1039/B715825D
Gale, P. A., and Gunnlaugsson, T. (2010). Preface: supramolecular chemistry of anionic species themed issue. Chem. Soc. Rev. 39, 3595–3596. doi:10.1039/C0CS90020F
Gillen, D. M., Hawes, C. S., and Gunnlaugsson, T. (2018). Solution-state anion recognition, and structural studies, of a series of electron-rich meta-phenylene bis(phenylurea) receptors and their self-assembled structures. J. Org. Chem. 83, 10398 − 10408. doi:10.1021/acs.joc.8b01481
Haque, S. A., Bolhofner, R. L., Wong, B. M., and Hossain, A. (2015). Colorimetric and optical discrimination of halides by a simple chemosensor. RSC Adv. 5, 38733–38741. doi:10.1039/C5RA02372F
Hynes, M. J. (1993). EQNMR: a computer program for the calculation of stability constants from nuclear magnetic resonance chemical shift data. Dalton Trans. 1993, 311–312. doi:10.1039/DT9930000311
Jia, C., Zuo, W., Zhang, D., Yang, X. J., and Wu, B. (2016). Anion recognition by oligo-(thio)urea-based receptors. Chem. Commun. 52, 9614–9627. doi:10.1039/C6CC03761E
Khansari, M. E., Johnson, C. R., Basaran, I., Nafis, A., Wang, J., Leszczynski, J., et al. (2015). Synthesis and anion binding studies of tris(3-aminopropyl)amine-based tripodal urea and thiourea receptors: proton transfer-induced selectivity for hydrogen sulfate over sulfate. RSC Adv. 5, 17606–17614. doi:10.1039/C5RA01315A
Khansari, M. E., Hasan, M. H., Johnson, C. R., Williams, N. A., Wong, B. M., Powell, D. R., et al. (2017a). Anion complexation studies of 3-nitrophenyl-substituted tripodal thiourea receptor: a naked-eye detection of sulfate via fluoride displacement assay. ACS Omega 2, 9057–9066. doi:10.1021/acsomega.7b01485
Khansari, M. E., Mirchi, A., Pramanik, A., Johnson, C. R., Leszczynski, J., and Hossain, M. A. (2017b). Remarkable hexafunctional anion receptor with operational urea-based inner cleft and thiourea-based outer cleft: novel design with high-efficiency for sulfate binding. Sci. Rep. 7, 6032. doi:10.1038/s41598-017-05831-x
Khansari, M. E., Wallace, K. D., and Hossain, M. A. (2014). Synthesis and anion recognition studies of a dipodal thiourea-based sensor for anions. Tetrahedron Lett. 55, 438–440. doi:10.1016/j.tetlet.2013.11.050
Kim, G.-D., Bothra, S., Sahoo, S. K., and Choi, H.-J. (2018). A novel C3v -symmetric molecular clip with tris(diamide) recognition sites on trindane platform for H2PO4− recognition. Tetrahedron Lett. 59, 1679–1682. doi:10.1016/j.tetlet.2018.03.055
Kim, G.-D., Bothra, S., Sahoo, S. K., and Choi, H.-J. (2019). Tripodal tris(diamide) receptor having H-bond donors and acceptors on trindane platform for H2PO4− recognition. J. Inclusion Phenom. Macrocycl. Chem. 95, 215–221. doi:10.1007/s10847-019-00937-3
Kim, W., Sahoo, S. K., Kim, G.-D., and Choi, H.-J. (2016). C3v -symmetric anion receptors with guanidine recognition motifs for ratiometric sensing of fluoride. RSC Adv. 6 (10), 7872–7878. doi:10.1039/c5ra26039f
Kim, W., Sahoo, S. K., Kim, G.-D., and Choi, H.-J. (2015). Novel C3v -symmetric trindane based tripodal anion receptor with tris(coumarin-urea) extension for optical sensing of bioactive anions. Tetrahedron 71 (42), 8111–8116. doi:10.1016/j.tet.2015.08.043
Li, R., Zhao, Y., Li, S., Yang, P., Huang, X., Yang, X. J., et al. (2013). Tris chelating phosphate complexes of bis(thio)urea ligands. Inorg. Chem. 52, 5851–5860. doi:10.1021/ic3028012
Manna, U., Chutia, R., and Das, G. (2016). Entrapment of cyclic fluoride–water and sulfate–water–sulfate cluster within the self-assembled structure of linear meta-phenylenediamine based bis-urea receptors: positional isomeric effect. Cryst. Growth Des. 16, 2893–2903. doi:10.1021/acs.cgd.6b00205
Manna, U., and Das, G. (2017). Anion binding consistency by influence of aromatic meta-disubstitution of a simple urea receptor: regular entrapment of hydrated halide and oxyanion clusters. CrystEngComm. 19, 5622–5634. doi:10.1039/C7CE01155E
Manna, U., Kayal, S., Samanta, S., and Das, G. (2017). Fixation of atmospheric CO2 as novel carbonate–(water)2–carbonate cluster and entrapment of double sulfate within a linear tetrameric barrel of a neutral bis-urea scaffold. Dalton Trans. 46, 10374–10386. doi:10.1039/C7DT01697B
Martínez-Máñez, R., and Sancenón, F. (2003). Fluorogenic and chromogenic chemosensors and reagents for anions. Chem. Rev. 103, 4419–4476. doi:10.1021/cr010421e
Nishizawa, S., Bühlmsnn, P., Iwaho, M., and Umezawa, Y. (1995). Anion recognition by urea and thiourea groups: remarkably simple neutral receptors for dihydrogenphosphate. Tetrahedron Lett. 36, 6483–6486. doi:10.1016/0040-4039(95)01296-T
Nishizawa, S., Bühlmsnn, P., Xiao, K. P., and Umezawa, Y. (1998). Application of a bis-thiourea ionophore for an anion selective electrode with a remarkable sulfate selectivity. Anal. Chim. Acta 358, 35–44. doi:10.1016/S0003-2670(97)00569-2
Olivari, M., Montis, R., Karagiannidis, L. E., Horton, P. N., Mapp, L. K., Coles, S. J., et al. (2015). Anion complexation, transport and structural studies of a series of bis-methylurea compounds. Dalton Trans. 44, 2138–2149. doi:10.1039/C4DT02893G
Park, J. O., Sahoo, S. K., and Choi, H. J. (2016). Design, synthesis and ¹H NMR study of C3v-symmetric anion receptors with urethane-NH as recognition group. Spectrochim. Acta Mol. Biomol. Spectrosc. 153, 199–205. doi:10.1016/j.saa.2015.08.015
Pflugrath, J. W., and Quiocho, F. A. (1985). Sulphate sequestered in the sulphate-binding protein of Salmonella typhimurium is bound solely by hydrogen bonds. Nature 314, 257–260. doi:10.1038/314257a0
Portis, B., Mirchi, A., Hasan, M. H., Khansari, M. E., Johnson, C. R., Leszczynski, J., et al. (2020). Cleft-induced ditopic binding of spherical halides with a hexaurea receptor. ChemistrySelect 5, 1401–1409. doi:10.1002/slct.201903950
Rhaman, M. M., Hasan, M. H., Alamgir, A., Xu, L., Powell, D. R., Wong, B. M., et al. (2018). Highly selective and sensitive macrocycle-based dinuclear foldamer for fluorometric and colorimetric sensing of citrate in water. Sci. Rep. 8, 286. doi:10.1038/s41598-017-18322-w
Rhaman, M. M., Hasan, M. H., Ali, Z. A., Powell, D. R., Tandon, R., Wong, B. M., et al. (2020). Charge-density induced discrimination of halides with a rigid dinuclear copper(II) complex. Mol. Syst. Des. Eng. 5, 996. doi:10.1039/d0me00025f
Sahoo, S. K., Kim, G.-D., and Choi, H.-J. (2016). Optical sensing of anions using C3v-symmetric tripodal receptors. J. Photochem. Photobiol. C. 27, 30–53. doi:10.1016/j.jphotochemrev.2016.04.004
Sessler, J. L., Gale, P. A., and Cho, W. S. (2008). Anion receptor chemistry. Cambridge, United Kingdom: The Royal Society of Chemistry.
Wang, Z., Luecke, H., Yao, N., and Quiocho, F. A. (1997). A low energy short hydrogen bond in very high resolution structures of protein receptor--phosphate complexes. Nat. Struct. Biol. 4, 519–522. doi:10.1038/nsb0797-519
Wenzel, M., Hiscock, J. R., and Gale, P. A. (2012). Anion receptor chemistry: highlights from 2010. Chem. Soc. Rev. 41, 480–520. doi:10.1039/C1CS15257B
Keywords: molecular cleft, urea, thiourea, anion binding, chemosensor
Citation: Manna U, Portis B, Egboluche TK, Nafis M and Hossain MA (2021) Anion Binding Studies of Urea and Thiourea Functionalized Molecular Clefts. Front. Chem. 8:575701. doi: 10.3389/fchem.2020.575701
Received: 24 June 2020; Accepted: 21 December 2020;
Published: 29 January 2021.
Edited by:
Mosae Selvakumar Paulraj, Asian University for Women, BangladeshReviewed by:
Suban K. Sahoo, Sardar Vallabhbhai National Institute of Technology Surat, IndiaChitra Varadaraju, St. Joseph’s College for Women, India
Copyright © 2021 Manna, Portis, Egboluche, Nafis and Hossain. This is an open-access article distributed under the terms of the Creative Commons Attribution License (CC BY). The use, distribution or reproduction in other forums is permitted, provided the original author(s) and the copyright owner(s) are credited and that the original publication in this journal is cited, in accordance with accepted academic practice. No use, distribution or reproduction is permitted which does not comply with these terms.
*Correspondence: Md. Alamgir Hossain, YWxhbWdpci5ob3NzYWluQGpzdW1zLmVkdQ==