- Department of Mathematics, Physics and Electrical Engineering, Northumbria University, Newcastle upon Tyne, United Kingdom
Emitters that exhibit thermally activated delayed fluorescence (TADF) are of interest for commercial applications in organic light-emitting diodes (OLEDs) due to their ability to achieve internal quantum efficiency of 100%. However, beyond the intrinsic properties of these materials it is important to understand how the molecules interact with each other and when these interactions may occur. Such interactions lead to a significant red shift in the photoluminescence and electroluminescence, making them less practicable for commercial use. Through summarizing the literature, covering solid-state solvation effects and aggregate effects in organic emitters, this mini review outlines a framework for the complete study of TADF emitters formed from the current-state-of-the-art techniques.
Introduction
Increasing the efficiency and stability of organic light-emitting diodes (OLEDs) is a focus of significant attention for researchers and one aspect of improving these systems is to produce novel emitters that have internal quantum efficiencies (IQEs) above the 25% dictated by spin statistics (Baldo et al., 1999). Thermally activated delayed fluorescence (TADF) (Uoyama et al., 2012; Dias et al., 2013) is one phenomenon that is used to achieve 100% IQE (Lin et al., 2016; Liu et al., 2018b; Zeng et al., 2018). This significant increase arises from the molecule being able to promote non-emissive triplet states to the emissive singlet state via reverse intersystem crossing (rISC). The rISC process requires a small energy gap between the singlet and triplet state and this can be achieved in a variety of ways and through a variety of molecular designs: exciplex systems where the donor (D) and acceptor (A) are non-identical molecules;(Sarma and Wong, 2018; Colella et al., 2019; Tang et al., 2020) organometallic systems (Di et al., 2017; Conaghan et al., 2018; Yersin et al., 2018; Mahoro et al., 2020) metal halide perovskites;(Zhou and Yan, 2019; Qin et al., 2020) and fully organic systems where the D and A are covalently bonded. (Uoyama et al., 2012; Dias et al., 2013) The subjects of this review are fully organic covalently bonded donor–acceptor (D–A) systems or donor–acceptor–donor (D–A–D) systems. D–A and D–A–D systems have the highest occupied molecular orbital (HOMO) and lowest unoccupied molecular orbital (LUMO) localized on the D and A units respectively to produce charge-transfer (CT) states and the required small singlet–triplet gap. This localization is achieved through control of the dihedral angle between the D and A moieties and the relative electron donating and accepting strengths of the D and A units.
TADF systems typically emit from singlet charge-transfer states (1CT), which are almost isoenergetic with triplet charge-transfer states (3CT), however intersystem crossing, and rISC, between these two states is prohibited (Lim and Kedzierski, 1973). This limitation can be overcome by coupling to a third state that does not have the same character. For most TADF systems this third state is a close-lying locally excited triplet state (3LE). Through spin-vibronic coupling of the 3LE state with the 3CT state the rISC process between 3CT and 1CT is enhanced. This was demonstrated theoretically by Gibson et al. (2016) and experimentally by Etherington et al. (2016). The need for proximity of the 3LE state with the 3CT state provides a new criterion for the molecular design of TADF systems. One major impact of this result is that to design a TADF emitter with a particular emission wavelength, the choice of D and A units is now limited by their 3LE energy.
Most work on TADF systems, through photophysical studies and within devices, is performed either in the solution state or dilute concentrations within small molecule or polymer hosts, meaning that the measurements are in an ideal scenario and relate predominantly to a single, isolated molecule. However, the CT states are also susceptible to solvent polarity or solid-state host rigidity, which can influence the emissive and functional behavior of the compound and there are many research articles and reviews on this topic (Etherington et al., 2016; Santos et al., 2016a,b, 2018; Haseyama et al., 2017; Wong and Zysman-Colman, 2017; Chatterjee and Wong, 2019; Hung et al., 2019). Although it must be noted that there is an extensive field of research into exciplex systems and controlling fluorescence behavior through host–guest interactions, where intermolecular interactions are embraced. This literature considers the interactions between non-identical molecules to achieve the small exchange energies and high rISC rates required for TADF (Kim et al., 2016; Nakanotani et al., 2016; Sarma and Wong, 2018; Chatterjee and Wong, 2019; Tang et al., 2020) and it has been observed that host–guest interactions can also imbue functional properties (Matsunaga and Yang, 2015; Feng et al., 2017; Ono et al., 2018).
Equally important is a molecule's interaction with identical molecules. There is extensive literature concerning aggregation-induced emission (AIE) of organic systems (Luo et al., 2001; Hong et al., 2009; Mei et al., 2014) and these effects need to be considered for TADF systems. This review will give an overview of the phenomena that occur when we consider TADF emitters beyond the ideal of the single molecule as well as the techniques used to study them. This review will focus on novel compounds that are designed or used for their behavior in aggregates and how studying the molecules' mechanochromism, thermochromism and concentration effects in doped films facilitate the understanding of new functional behaviors. The review will also reflect on the discovery of functional behavior in existing compounds, especially 1,2,3,5-tetrakis(carbazol-9-yl)-4,6-dicyanobenzene (4CzIPN)–an archetypal TADF emitter (Uoyama et al., 2012) and how this may influence the interpretation of previous results highlighting the two effects related to the red shifting of emission with concentration: solid-state solvation effect (SSSE) and aggregation.
Concentration and Aggregation in Organic Emitters
Aggregation-caused quenching (Thomas et al., 2007) and AIE (Luo et al., 2001; Hong et al., 2009; Mei et al., 2014) are two phenomena that provide a framework for the study of organic emitters beyond the single molecule in neat films, organic crystals and doped matrices. The basis of the AIE field is to understand the interaction between the molecules in these systems and establish the causes of enhancements or reductions in emission, shifts in emission color, mechanochromism or thermochromism. Recent publications and reviews in the area include (Hong et al., 2009; Mei et al., 2014; Furue et al., 2016; Gan et al., 2016; Sturala et al., 2017; Zhang et al., 2017, 2019b, 2020; Liu et al., 2018a; Yu et al., 2020), which demonstrate that phenyl rings should not be considered as entirely inert spacer units in the design of organic emitters and that the aggregation effects can be controlled through photoexcitation. These results have far-reaching implications for molecular design of organic emitters as researchers now need to take care in their choice of moieties during synthesis.
Novel TADF Emitters Exhibiting Functional Behavior
Implementing the techniques found in the works mentioned above to TADF systems becomes somewhat more complicated as there is now not just the behavior of the prompt singlet emission to explain but also the processes and energetics underlying rISC and delayed emission. Functional phenomena in TADF systems as a result of intermolecular interactions have been observed for a range of D–A and D–A–D systems (Hladka et al., 2018; Huang et al., 2018; Klimash et al., 2018; Pashazadeh et al., 2018; Shi et al., 2018; Skuodis et al., 2018; Bhatia and Ray, 2019; Zheng et al., 2019). These works provide methodologies and insights in understanding the influence that intermolecular interactions have on TADF emitters including their photophysical properties. The compounds discussed in this review are shown in Figure 1A.
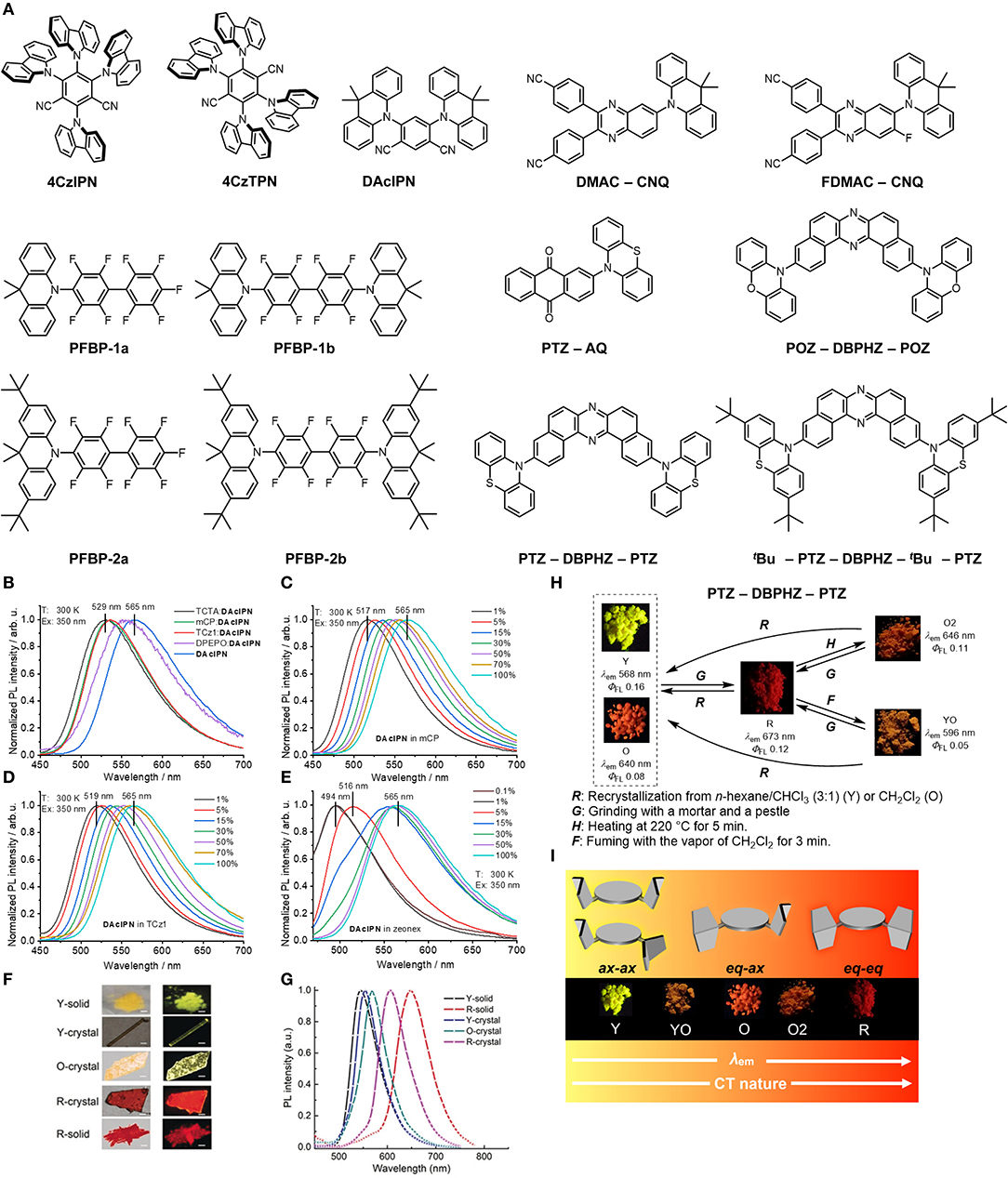
Figure 1. (A) The chemical structures of the compounds discussed in the review. (B–E) The effects of host and concentration on the emission profile of DAcIPN showing the significant red shifts observed when increasing the compound concentration. In (B) the shift in emission of DAcIPN in 15 wt% films of hosts of different polarizability showing that the red shift trend does not follow according to the polarizability of the host. (C–E) Red shifts in the emission occur across three different hosts, mCP, TCz1 (Tsai et al., 2007) and zeonex respectively with increasing concentration. Adapted with permission from Elsevier from Figure 4 in Skuodis et al. (2018) (F,G) The different emission profiles of PTZ-AQ as a function of solid-state environment from Y-solid to R-solid. Adapted with permission from John Wiley and Sons from Figure 1 in Huang et al. (2018) (H) The different forms and the methods of obtaining them for PTZ-DBPHZ-PTZ and (I) how the quasi-equatorial (eq) and quasi-axial (ax) conformers of phenothiazine influence the solid-state emission of PTZ-DBPHZ-PTZ. Adapted from Figures 4, 8 in Okazaki et al. (2017); (Published by The Royal Society of Chemistry).
Skuodis et al. (2018) observed changes in emission behavior as a function of concentration, the host molecule, mechanical force and thermal annealing in 4,6-Di(9,9-dimethylacridan-10-yl)isophthalonitrile (DAcIPN). The researchers found the photoluminescence quantum yield (PLQY) of DAcIPN decreased from 83% at 15 wt% in 1,3-Bis(N-carbazolyl)benzene (mCP) to 47% in a film of neat DAcIPN–they attribute this result to a decrease in TADF contribution in the neat films. The energy of the emission red shifted with increasing concentration and was 2.32 eV (533 nm) in the mCP matrix and 2.19 eV (565 nm) in the neat film, a trend that was observed for a variety of different hosts (Figures 1B–E). The authors considered two explanations for this red shift in emission for the compound in solid-state hosts: SSSE (Bulović et al., 1999) and aggregation. They found that the red shifts did not correlate with the solid-state polarizabilities of the hosts and therefore aggregation was the dominant effect in these systems, with concomitant changes in the dihedral angle of the DAcIPN. During thermal annealing of the films at 130°C the authors observed a significant blue shift in the emission of both the doped and non-doped films: the doped film blue shifted from 2.19 eV (565 nm) to 2.40 eV (517 nm), which was attributed to the thermal energy allowing the molecules to disaggregate. This disaggregation allowed the dihedral angle to increase, restoring the higher energy emission. The disaggregation also caused an increase in the PLQY of the neat film from 47 to 64%.
A similar study by Hladka et al. (2018) from the same group focused on these phenomena in sky-blue emitters based on perfluorobiphenyls (PFBP). The researchers studied four systems known as PFBP-1a, PFBP-1b, PFBP-2a, and PFBP-2b and showed that PFBP-2a, contrary to the other compounds, showed a blue shift in emission when a thermally-evaporated film of the compound was fumed with toluene vapor. This blue shift was attributed to polymorphism of the compound and shows that very small changes in structure between a set of similar compounds can have significant effects on their aggregation properties. As polymorphism is a common explanation for many of these observed properties in the solid-state, knowledge of polymorphism and crystallography is crucial to understanding the fundamental origins of this functional behavior (Chung and Diao, 2016; Bernstein, 2020; Levesque et al., 2020). The importance of knowing the crystal structures of the compounds for understanding the packing and intermolecular interactions is demonstrated in the work by Klimash et al. (2018) In this work they uncovered differences in the molecular crystal structures and how these relate to differences in the TADF efficiency.
Polymorphism as one of the causes of different TADF behavior in crystalline films/powders is reported by Zheng et al. (2019) in 4,4′-(6-(9,9-dimethylacridin-10(9H)-yl)quinoxaline- 2,3-diyl)dibenzonitrile (DMAC-CNQ) and 4,4′-(6-(9,9-dime- thylacridin-10(9H)-yl)-7-fluoroquinoxaline-2,3-diyl)dibenzo- nitrile (FDMAC-CNQ). The authors reported two different polymorphs for DMAC-CNQ, which they termed Y-crystal and O-crystal and three polymorphs for FDMAC-CNQ termed Y-crystal, O-crystal and R-crystal based on the emission color (Yellow, Orange and Red). The changes in emission color between the crystals are due to different conformations of the DMAC moiety that then has a resultant effect on the π-π interactions in the crystal. The trend for both systems is that, going from yellow to red, the number of π-π interactions increases as a function of the DMAC conformation. Similarly, a trend of decreasing TADF contribution and PLQY was observed going from Y-crystal to R-crystal, again related to the conformations of the compound. These single crystal studies which are linked to the molecular structure has allowed the authors to interpret the mechanochromism behavior they observed from the pristine, ground and fumed states of the compound. Single crystal X-ray diffraction and powder X-ray diffraction studies provide an important tool for the study of TADF beyond the single molecule and are a key part of the framework in determining aggregation effects.
Huang et al. (2018) demonstrated the significant accumulation of functional behavior that can be obtained in TADF D–A molecules. In the compound 2-(phenothiazine-10-yl)-anthraquinone (PTZ-AQ) they observed TADF, aggregation-induced emission and mechanochromism alongside polymorphism. They comment on the effect that aggregation has on the TADF behavior arguing that TADF can even be tuned by the aggregation state. Figures 1F,G show the different emission profiles that can be obtained as a function of solid-state environment. The crystals and solids, which the authors categorize into five different aggregation states, facilitate emission energies from the green to the red: a yellow solid (Y-solid), a red solid (R-solid), a yellow rod-like crystal (Y-crystal), an orange flake-like crystal (O-crystal) and a red flake-like crystal (R-crystal) with the R-crystal showing the smallest singlet-triplet gap and high PLQY.
In general, the idea of molecular conformation affecting the TADF and mechanochromism properties of a compound is in agreement with the work of Okazaki et al. (2017) In 2017 the researchers compared phenoxazine–dibenzo[a,j]phenazine–phenoxazine (POZ–DBPHZ–POZ) and the phenothiazine analog with tert-butyl groups (tBu-PTZ–DBPHZ–tBu-PTZ) and (PTZ–DBPHZ–PTZ) without tert-butyl groups. Although two polymorphs of PTZ–DBPHZ–PTZ were found only one of tBu-PTZ–DBPHZ–tBu-PTZ was discovered. In this study the emission of both compounds red shifted with grinding. Upon heating the emission of tBu-PTZ–DBPHZ–tBu-PTZ continued to red shift however, the emission of PTZ–DBPHZ–PTZ blue shifted (Figure 1H). The particular mechanochromic behavior of PTZ–DBPHZ–PTZ is linked to the tendency for PTZ to form as one of two conformers (Figure 1I): quasi-axial (ax) or quasi-equatorial (eq). (Malrieu and Pullman, 1964; Bodea and Silberg, 1968; Coubeils and Pullman, 1972; Etherington et al., 2017; Santos et al., 2018) The red emission comes from the highly twisted equatorial-equatorial conformer, the orange from the axial-equatorial and the yellow emission from the axial-axial conformer which allows formation of a higher energy 1LE state. This again demonstrates the importance of linking mechanochromic and TADF behaviors to the molecular structure through single crystal X-ray diffraction techniques. Mechanochromism is a useful tool and part of the framework for studying intermolecular effects in TADF systems. The work by Pashazadeh et al. (2018) shows how TADF can be turned on and off with grinding and how the intermolecular interactions helped mediate the singlet triplet gaps and control the fundamental emission phenomena.
Uncovering Intermolecular Effects in Existing Motifs
As noted in the work by Skuodis et al. (2018) there are two proposed origins for the red shift with increasing concentration in solid-state hosts: the SSSE effect (Cotts et al., 2017; Delor et al., 2017; Han and Kim, 2019) and aggregation (dimer or intermolecular) effects (Skaisgiris et al., 2019; Zhang et al., 2019a) In this section of the review, literature surrounding an existing and widely-used motif, 4CzIPN, (Uoyama et al., 2012) will be used to contextualize this red shift in emission.
In 2017 Kim et al. (2017) studied the effects of concentration on the emission profile and TADF characteristics of 4CzIPN. They observed a significant redshift in the emission with increasing concentration (Figures 2A–E) and a decrease in the TADF lifetime. These red shifts, both in photoluminescence and electroluminescence, have been observed by a range of researchers (Nakanotani et al., 2013; Kim and Lee, 2014; Komatsu et al., 2015; Wang et al., 2016; Zhu et al., 2017; Li et al., 2018; Niwa et al., 2018). Kim et al. (2017) attributed these red shifts and the consequential change in TADF behavior to the changing dipole moment of the surrounding matrix as the host molecules are subsequently replaced with more 4CzIPN molecules (Figure 2A). 4CzIPN is more polar than the host 4,4′-Bis(N-carbazolyl)-1,1′-biphenyl (CBP) and therefore as the concentration increases, the dipole moment and polarizability of the environment increases producing a red shift in the emission. This is the effect known as SSSE (Bulović et al., 1999).
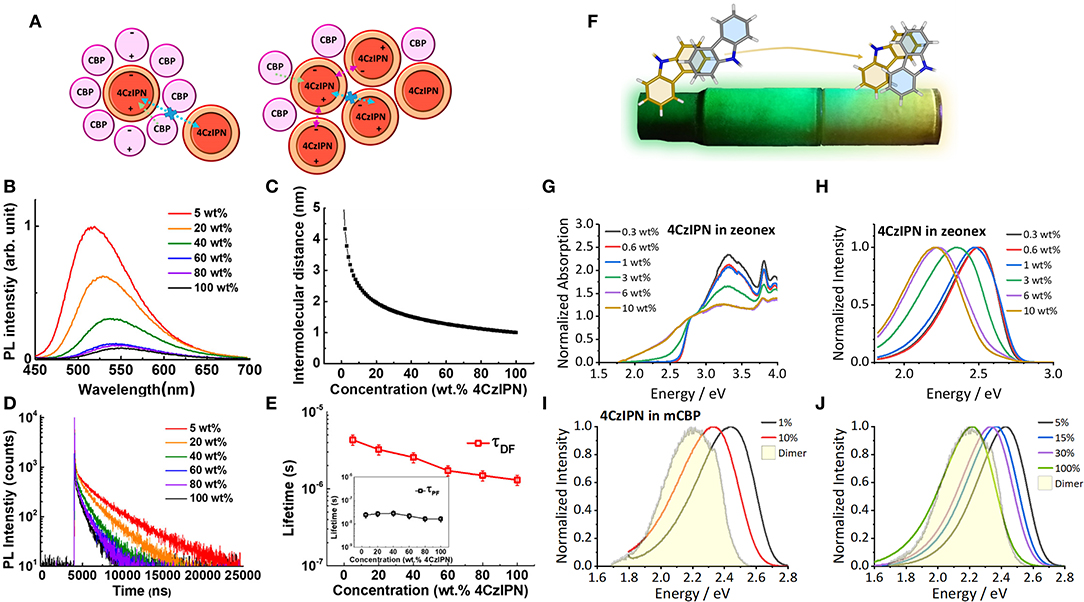
Figure 2. (A) A diagram showing the effect of increasing concentration on the processes of 4CzIPN. The dipole moment of 4CzIPN is larger than CBP and affects the emission color of neighboring 4CzIPN molecules through SSSE. (B–E) Photophysical studies of 4CzIPN in evaporated films of CBP with increasing concentration. (B) There is a reduction in intensity and a bathochromic shift in the emission color and (C) a reduction in the intermolecular distance that leads to (D,E) a reduction in the delayed fluorescence lifetime (τDF). Figures adapted with permission from Kim et al. (2017). “Concentration Quenching Behavior of Thermally Activated Delayed Fluorescence in a Solid Film.” The Journal of Physical Chemistry C 121 (26): 13986–13997. https://doi.org/10.1021/acs.jpcc.7b02369. Copyright 2017 American Chemical Society (Kim et al., 2017) (F). The effects of dimers on the emission color of 4CzIPN that was demonstrated under UV illumination in a sublimation tube. (G) The appearance of the absorption band of the dimer species with increasing concentration in a zeonex film and (H) the resultant effect on photoluminescence. The presence of the new absorption band allows direct excitation of the dimer species, which has characteristic emission (shaded area) that appears in (I) the photoluminescence of evaporated films of 3,3′-Di(9H-carbazol-9-yl)-1,1′-biphenyl (mCBP) and (J) the electroluminescence of devices using a proprietary material from Merck as the host. Figures adapted from Etherington et al. (2019).
Performing measurements in solid-state hosts with different polarities like Skuodis et al. (2018) is one way of deconvoluting SSSE and aggregation effects, however to completely characterize these systems a diverse framework of measurements is required. In 2019, Etherington et al. (2019) performed a study on 4CzIPN, incorporating techniques used in the majority of investigations mentioned in section Novel TADF Emitters Exhibiting Functional Behavior, including mechanochromism, thermochromism, single-crystal X-ray diffraction and combined them with time-resolved photoluminescence spectroscopy. This framework uncovered the dimer species of 4CzIPN present in certain environments. The effects of these intermolecular interactions were seen during sublimation of 4CzIPN and Etherington et al. observed that crystals and solid-state powders with different emission colors were produced along the sublimation tubing (See Figure 2F). This emission color was found to be changeable through thermal annealing and the introduction of mechanical energy. The fact that in neat films there were changes in emission color without a change in polarizability of the surrounding media suggests that SSSE is not the sole, or even dominant, effect in determining the emission properties of 4CzIPN. Indeed, the related material 2,3,5,6-Tetra(carbazol-9-yl)benzene-1,4-dicarbonitrile (4CzTPN) (Uoyama et al., 2012) is known to give emission of orange-red within the solid-state but only yellow emission in one of the most polar solvents dimethyl sulfoxide (DMSO). This result strongly suggests that polarizability is not the most crucial effect for the emission properties of these materials in the solid-state. Theoretical work by Northey et al. (2017) shows that the SSSE, while present in TADF systems, can be restricted due to rigidity of the host molecule in a solid-state environment.
The dimer/aggregate species observed in 4CzIPN through excitation at 2.33 eV (532 nm), significantly below the band gap of the monomer absorption band (Figure 2G), has an emission profile that almost exactly matches the higher concentration zeonex films and the neat film (Figures 2H–J). This gives unequivocal evidence that intermolecular interactions play a key role in determining the emission properties, color purity and TADF efficiency of these systems. The framework Etherington et al. established (Etherington et al., 2019), which builds upon techniques in the wider literature (section Novel TADF Emitters Exhibiting Functional Behavior) provides the means to deconvolute SSSE from intermolecular effects. This is an important aspect to build the complete picture of TADF behavior beyond the single molecule while highlighting the need for researchers to be acutely aware of intermolecular interactions in future studies.
Conclusion and Outlook
While there has been vast development of new TADF emitters and our understanding of the fundamental processes of rISC and the spin states has developed, it is now time to look towards understanding these processes in non-ideal situations using the techniques and framework discussed above. This framework will facilitate the development and understanding of functional materials that are sensitive to mechanical and thermal energy while opening up pathways to AIE-based, non-doped OLEDs (Furue et al., 2016; Liu et al., 2018a; Cai et al., 2020; Yang et al., 2020). It will also help unify the study of intramolecular TADF emitters with the studies of polymorphism (Chung and Diao, 2016; Bernstein, 2020; Levesque et al., 2020) exciplexes (Kim et al., 2016; Sarma and Wong, 2018; Tang et al., 2020) and host–guest interactions (Matsunaga and Yang, 2015; Feng et al., 2017; Ono et al., 2018).
The framework should continually incorporate new experimental techniques that are sensitive to the environmental changes underpinning this behavior to be controlled. So far, the framework includes single crystal studies and controlled mechanochromic and thermochromic analyses. This framework will then allow for the control and use of these properties as demonstrated in the work by Bhatia and Ray (2019) The researchers utilized the dimer/aggregate state, not to improve TADF but rather, to introduce room temperature phosphorescence. A combination of the emission profiles of the monomer, dimer and aggregated species produces a wide band white afterglow.
Future work will specifically link molecular structure to the desired properties. Sussardi et al. have begun this progress linking the crystal structure of a compound directly to the emission profile as a function of pressure (Sussardi et al., 2020). This work allows a systematic way to study and correlate the intermolecular interactions and the photophysics. Etherington et al. (2019) showed that the addition of tert-butyl groups do little to prevent the intermolecular interactions and thus we must look to bulkier spacer units or alternative methods to control these effects. High-pressure crystallography, similar to the work of Sussardi et al. (2020) would be able to test the limit of these bulky units. The techniques and studies mentioned in this review provide a basis to develop future frameworks and criteria for inhibiting or enhancing intermolecular interactions. This will help to provide high color purity compounds for OLED commercialization and unlock new functional behavior for applications beyond OLEDs.
Author Contributions
MKE devised the idea for the topic of this review, collated the literature and wrote the review. The author confirms being the sole contributor of this work and has approved it for publication.
Conflict of Interest
The author declares that the research was conducted in the absence of any commercial or financial relationships that could be construed as a potential conflict of interest.
References
Baldo, M. A., O'Brien, D. F., and Forrest, S. R. (1999). Excitonic singlet-triplet ratio in a semiconducting organic thin film. Phys. Rev. B 60, 14422–14428. doi: 10.1103/PhysRevB.60.14422
Bernstein, J. (2020). Polymorphism in Molecular Crystals. 2nd Edn. New York, NY: Oxford University Press. doi: 10.1093/oso/9780199655441.001.0001
Bhatia, H., and Ray, D. (2019). Use of dimeric excited states of the donors in d4-a systems for accessing white light emission, afterglow, and invisible security ink. J. Phys. Chem. C 123, 22104–22113. doi: 10.1021/acs.jpcc.9b07762
Bodea, C., and Silberg, I. (1968). Recent advances in the chemistry of phenothiazines. Chimia Intern. J. Chem. 66, 321–460. doi: 10.1016/S0065-2725(08)60375-X
Bulović, V., Deshpande, R., Thompson, M. E., and Forrest, S. R. (1999). Tuning the color emission of thin film molecular organic light emitting devices by the solid state solvation effect. Chem. Phys. Lett. 308, 317–322. doi: 10.1016/S0009-2614(99)00580-1
Cai, Z., Chen, H., Guo, J., Zhao, Z., and Tang, B. Z. (2020). Efficient aggregation-induced delayed fluorescence luminogens for solution-processed oleds with small efficiency roll-off. Front. Chem. 8:193. doi: 10.3389/fchem.2020.00193
Chatterjee, T., and Wong, K. T. (2019). Perspective on host materials for thermally activated delayed fluorescence organic light emitting diodes. Adv. Optical Mater. 7, 1–34. doi: 10.1002/adom.201800565
Chung, H., and Diao, Y. (2016). Polymorphism as an emerging design strategy for high performance organic electronics. J. Mater. Chem. C 4, 3915–3933. doi: 10.1039/C5TC04390E
Colella, M., Danos, A., and Monkman, A. P. (2019). Less is more: dilution enhances optical and electrical performance of a TADF exciplex. J. Phys. Chem. Lett. 10, 793–798. doi: 10.1021/acs.jpclett.8b03646
Conaghan, P. J., Matthew Menke, S., Romanov, A. S., Jones, S. T. E., Pearson, A. J., Evans, E. W., et al. (2018). Efficient vacuum-processed light-emitting diodes based on carbene–metal–amides. Adv. Mater. 30:35. doi: 10.1002/adma.201802285
Cotts, B. L., McCarthy, D. G., Noriega, R., Penwell, S. B., Delor, M., Devore, D. D., et al. (2017). Tuning thermally activated delayed fluorescence emitter photophysics through solvation in the solid state. ACS Energy Lett. 2, 1526–1533. doi: 10.1021/acsenergylett.7b00268
Coubeils, J. L., and Pullman, B. (1972). Molecular orbital study of the conformational properties of phenothiazines. Theor. Chim. Acta 24, 35–41. doi: 10.1007/BF00528308
Delor, M., McCarthy, D. G., Cotts, B. L., Roberts, T. D., Noriega, R., Devore, D. D., et al. (2017). Resolving and controlling photoinduced ultrafast solvation in the solid state. J. Phys. Chem. Lett. 8, 4183–4190. doi: 10.1021/acs.jpclett.7b01689
Di, D., Romanov, A. S., Yang, L., Richter, J. M., Rivett, J. R. H., Jones, S., et al. (2017). High-performance light-emitting diodes based on carbene-metal-amides. Science 356, 159–163. doi: 10.1126/science.aah4345
Dias, F. B., Bourdakos, K. N., Jankus, V., Moss, K. C., Kamtekar, K. T., Bhalla, V., et al. (2013). Triplet harvesting with 100% efficiency by way of thermally activated delayed fluorescence in charge transfer OLED emitters. Adv. Mater. (Deerfield Beach, Fla.) 25, 3707–3714. doi: 10.1002/adma.201300753
Etherington, M. K., Franchello, F., Gibson, J., Northey, T., Santos, J., Ward, J. S., et al. (2017). Regio- and conformational isomerization critical to design of efficient thermally-activated delayed fluorescence emitters. Nat. Commun. 8:14987. doi: 10.1038/ncomms14987
Etherington, M. K., Gibson, J., Higginbotham, H. F., Penfold, H. J., and Monkman, A. P. (2016). Revealing the spin–vibronic coupling mechanism of thermally activated delayed fluorescence. Nat. Commun. 7:13680. doi: 10.1038/ncomms13680
Etherington, M. K., Kukhta, N. A., Higginbotham, H. F., Danos, A., Bismillah, A. N., Graves, D. R., et al. (2019). Persistent dimer emission in thermally activated delayed fluorescence materials. J. Phys. Chem. C 123, 11109–11117. doi: 10.1021/acs.jpcc.9b01458
Feng, H. T., Bin Xiong, J., Luo, J., Fang Feng, W., Yang, D., and Song Zheng, Y. (2017). Selective host–guest co-crystallization of pyridine-functionalized tetraphenylethylenes with phthalic acids and multicolor emission of the co-crystals. Chem. A Eur. J. 23, 644–651. doi: 10.1002/chem.201604133
Furue, R., Nishimoto, T., Seob Park, I., Lee, J., and Yasuda, T. (2016). Aggregation-induced delayed fluorescence based on donor/acceptor-tethered janus carborane triads: unique photophysical properties of nondoped OLEDs. Angew. Chem. Intern. Edition 55, 7171–7175. doi: 10.1002/anie.201603232
Gan, S., Luo, W., He, B., Chen, L., Nie, H., Hu, R., et al. (2016). Integration of aggregation-induced emission and delayed fluorescence into electronic donor-acceptor conjugates. J. Mater. Chem. C 4, 3705–3708. doi: 10.1039/C5TC03588K
Gibson, J., Monkman, A. P., and Penfold, T. J. (2016). The importance of vibronic coupling for efficient reverse intersystem crossing in thermally activated delayed fluorescence molecules. Chem. Phys. Chem. 17, 2956–2961. doi: 10.1002/cphc.201600662
Han, H., and Kim, E. (2019). Dielectric effects on charge-transfer and local excited states in organic persistent room-temperature phosphorescence. Chem. Mater. 31, 6925–6935. doi: 10.1021/acs.chemmater.9b01364
Haseyama, S., Niwa, A., Kobayashi, T., Nagase, T., Goushi, K., Adachi, C., et al. (2017). Control of the singlet–triplet energy gap in a thermally activated delayed fluorescence emitter by using a polar host matrix. Nanos. Res. Lett. 12:268. doi: 10.1186/s11671-017-2012-1
Hladka, I., Yu Volyniuk, D., Bezvikonnyi, O., Kinzhybalo, V., Bednarchuk, T., Danyliv, Y., et al. (2018). Polymorphism of derivatives of tert-butyl substituted acridan and perfluorobiphenyl as sky-blue OLED emitters exhibiting aggregation induced-active thermally activated delayed fluorescence. J. Mater. Chem. C. 6, 13179–13189. doi: 10.1039/C8TC04867C
Hong, Y., Lam, J. W. Y., and Zhong Tang, B. (2009). Aggregation-induced emission: phenomenon, mechanism and applications. Chem. Commun. 29, 4332–4353. doi: 10.1039/b904665h
Huang, B., Chen, W.-C., Li, Z., Zhang, J., Zhao, W., Feng, Y., et al. (2018). Manipulation of molecular aggregation states to realize polymorphism, AIE, MCL, and TADF in a single molecule. Angew. Chem. Intern. Edition 57, 12473–12477. doi: 10.1002/anie.201806800
Hung, M. K., Wei Tsai, K., Sharma, S., Yi Wu, J., and An Chen, S. (2019). Acridan-grafted poly(Biphenyl germanium) with high triplet energy, low polarizability, and an external heavy-atom effect for highly efficient sky-blue TADF electroluminescence. Angew. Chem. Intern. Edition 58, 11317–11323. doi: 10.1002/anie.201904433
Kim, B. S., and Lee, J. Y. (2014). Phosphine oxide type bipolar host material for high quantum efficiency in thermally activated delayed fluorescent device. ACS Appl. Mater. Interfaces 6, 8396–8400. doi: 10.1021/am501301g
Kim, H. S., Park, S.-R., and Chul Suh, M. (2017). Concentration quenching behavior of thermally activated delayed fluorescence in a solid film. J. Phys. Chem. C 121, 13986–13997. doi: 10.1021/acs.jpcc.7b02369
Kim, K. H., Jun Yoo, S., and Kim, J. J. (2016). Boosting triplet harvest by reducing nonradiative transition of exciplex toward fluorescent organic light-emitting diodes with 100% internal quantum efficiency. Chem. Mater. 28, 1936–1941. doi: 10.1021/acs.chemmater.6b00478
Klimash, A., Pander, P., Klooster, W. T., Coles, S. J., Data, P., Dias, F. B., et al. (2018). Intermolecular interactions in molecular crystals and their effect on thermally activated delayed fluorescence of helicene-based emitters. J. Mater. Chem. C 6, 10557–10568. doi: 10.1039/C8TC03390K
Komatsu, R., Sasabe, H., Inomata, S., Pu, Y.-J., and Kido, J. (2015). High efficiency solution processed OLEDs using a thermally activated delayed fluorescence emitter. Synthetic Metals 202, 165–168. doi: 10.1016/j.synthmet.2015.02.009
Levesque, A., Maris, T., and Wuest, J. D. (2020). ROY reclaims its crown: new ways to increase polymorphic diversity. J. Am. Chem. Soc. doi: 10.1021/jacs.0c04434
Li, N., Fang, Y., Li, L., Zhao, H., Quan, Y., Ye, S., et al. (2018). A universal solution-processable bipolar host based on triphenylamine and pyridine for efficient phosphorescent and thermally activated delayed fluorescence OLEDs. J. Luminescence 199, 465–4674. doi: 10.1016/j.jlumin.2018.03.003
Lim, E. C., and Kedzierski, M. (1973). Spin-forbidden electronic transitions in non-planar aromatic amines. Chem. Phys. Lett. 20, 242–245. doi: 10.1016/0009-2614(73)85167-X
Lin, T. A., Chatterjee, T., Lung Tsai, W., Kai Lee, W., Jung Wu, M., et al. (2016). Sky-blue organic light emitting diode with 37% external quantum efficiency using thermally activated delayed fluorescence from spiroacridine-triazine hybrid. Adv. Mater. 28, 6976–6983. doi: 10.1002/adma.201601675
Liu, H., Zeng, J., Guo, J., Nie, H., Zhao, Z., and Tang, B. Z. (2018a). High-performance non-doped OLEDs with nearly 100 % exciton use and negligible efficiency roll-off. Angew. Chem. Intern. Edition 57, 9290–9294. doi: 10.1002/anie.201802060
Liu, Y., Li, C., Ren, Z., Yan, S., and Bryce, M. R. (2018b). All-organic thermally activated delayed fluorescence materials for organic light-emitting diodes. Nat. Rev. Mater. 3:18020. doi: 10.1038/natrevmats.2018.20
Luo, J., Xie, Z., Xie, Z., Lam, J. W. Y., Cheng, L., Chen, H., et al. (2001). Aggregation-induced emission of 1-methyl-1,2,3,4,5-pentaphenylsilole. Chem. Commun. 18, 1740–1741. doi: 10.1039/b105159h
Mahoro, G. U., Fernandez-Cestau, J., Renaud, J. L., Coto, P. B., Costa, R. D., and Gaillard, S. (2020). Recent advances in solid-state lighting devices using transition metal complexes exhibiting thermally activated delayed fluorescent emission mechanism. Adv. Opt. Mater. 260, 1–36. doi: 10.1002/adom.202000260
Malrieu, J.-P., and Pullman, B. (1964). Sur les propriétés électroniques de La phénothiazine et de son radical. Theor. Chim. Acta 2, 293–301. doi: 10.1007/BF00527056
Matsunaga, Y., and Yang, J. S. (2015). Multicolor fluorescence writing based on host-guest interactions and force-induced fluorescence-color memory. Angew. Chem. Intern. Edition 54, 7985–7989. doi: 10.1002/anie.201503406
Mei, J., Hong, Y., Lam, J. Y. W., Qin, A., Tang, Y., and Tang, B. (2014). Aggregation-induced emission: the whole is more brilliant than the parts. Adv. Mater. 26, 5429–5479. doi: 10.1002/adma.201401356
Nakanotani, H., Furukawa, T., Morimoto, K., and Adachi, C. (2016). Electrical conductors: long-range coupling of electron-hole pairs in spatially separated organic donor-acceptor layers. Sci. Adv. 2, 1–8. doi: 10.1126/sciadv.1501470
Nakanotani, H., Masui, K., Nishide, J., Shibata, T., and Adachi, C. (2013). Promising operational stability of high-efficiency organic light-emitting diodes based on thermally activated delayed fluorescence. Sci. Rep. 3:2127. doi: 10.1038/srep02127
Niwa, A., Haseyama, S., Kobayashi, T., Nagase, T., Goushi, K., Adachi, C., et al. (2018). Triplet-triplet annihilation in a thermally activated delayed fluorescence emitter lightly doped in a host. Appl. Phys. Lett. 113:083301. doi: 10.1063/1.5025870
Northey, T., Stacey, J. E., and Penfold, T. J. (2017). The role of solid state solvation on the charge transfer state of a thermally activated delayed fluorescence emitter. J. Mater. Chem. C 5, 11001–11009. doi: 10.1039/C7TC04099G
Okazaki, M., Takeda, Y., Data, P., Pander, P., Higginbotham, H., Monkman, A. P., et al. (2017). Thermally activated delayed fluorescent phenothiazine-dibenzo[a,j]phenazine-phenothiazine triads exhibiting tricolor-changing mechanochromic luminescence. Chem. Sci. 8, 2677–2686. doi: 10.1039/C6SC04863C
Ono, T., Taema, A., Goto, A., and Hisaeda, Y. (2018). Switching of monomer fluorescence, charge-transfer fluorescence, and room-temperature phosphorescence induced by aromatic guest inclusion in a supramolecular host. Chem. A Eur. J. 24, 17487–17496. doi: 10.1002/chem.201804349
Pashazadeh, R., Pander, P., Lazauskas, A., Dias, F. B., and Grazulevicius, J. V. (2018). Multicolor luminescence switching and controllable thermally activated delayed fluorescence turn on/turn off in carbazole–quinoxaline–carbazole triads. J. Phys. Chem. Lett. 9, 1172–1177. doi: 10.1021/acs.jpclett.8b00136
Qin, C., Matsushima, T., Potscavage, M. J., Sandanayaka, A. S. D., Leyden, M. R., Bencheikh, F., et al. (2020). Triplet management for efficient perovskite light-emitting diodes. Nat. Photonics 14, 70–75. doi: 10.1038/s41566-019-0545-9
Santos, P. L. D., Etherington, M. K., and Monkman, A. P. (2018). Chemical and conformational control of the energy gaps involved in the thermally activated delayed fluorescence mechanism. J. Mater. Chem. C 6, 4842–4853. doi: 10.1039/C8TC00991K
Santos, P. L. D., Ward, J. S., Bryce, M. R., and Monkman, A. P. (2016a). Using guest–host interactions to optimize the efficiency of TADF OLEDs. J. Phys. Chem. Lett. 7, 3341–3346. doi: 10.1021/acs.jpclett.6b01542
Santos, P. L. D., Ward, J. S., Data, P., Batsanov, A. S., Bryce, M. R., Dias, F. B., et al. (2016b). Engineering the singlet-triplet energy splitting in a TADF molecule. J. Mater. Chem. C 4, 3815–3824. doi: 10.1039/C5TC03849A
Sarma, M., and Wong, K. T. (2018). Exciplex: an intermolecular charge-transfer approach for TADF. ACS Appl. Mater. Interfaces 10, 19279–19304. doi: 10.1021/acsami.7b18318
Shi, Y. Z., Wang, K., Li, X., Le Dai, G., Liu, W., Ke, K., et al. (2018). Intermolecular charge-transfer transition emitter showing thermally activated delayed fluorescence for efficient non-doped OLEDs. Angew. Chem. Intern. Edition 57, 9480–9484. doi: 10.1002/anie.201804483
Skaisgiris, R., Serevičius, T., Kazlauskas, K., Geng, Y., Adachi, C., and Juršenas, S. (2019). Origin of dual emission in σ-bridged donor–acceptor TADF compounds. J. Mater. Chem. C 7, 12601–12609. doi: 10.1039/C9TC03548F
Skuodis, E., Bezvikonnyi, O., Tomkeviciene, A., Volyniuk, D., Mimaite, V., Lazauskas, A., et al. (2018). Aggregation, thermal annealing, and hosting effects on performances of an acridan-based TADF emitter. Org. Electron. 63, 29–40. doi: 10.1016/j.orgel.2018.09.002
Sturala, J., Etherington, M. K., Bismillah, A. N., Higginbotham, H. F., Trewby, W., Aguilar, J. A., et al. (2017). Excited-state aromatic interactions in the aggregation-induced emission of molecular rotors. J. Am. Chem. Soc. 139, 17882–17889. doi: 10.1021/jacs.7b08570
Sussardi, A., Hobday, C. L., Marshall, R. J., Forgan, R. S., Jones, A. C., and Moggach, S. A. (2020). Correlating pressure-induced emission modulation with linker rotation in a photoluminescent MOF. Angew. Chem. Intern. Edition 59, 8118–8122. doi: 10.1002/anie.202000555
Tang, X., Cui, L.-S., Li, H.-C., Gillett, A. J., Auras, F., Qu, Y.-K., et al. (2020). Highly efficient luminescence from space-confined charge-transfer emitters. Nat. Mater. doi: 10.1038/s41563-020-0710-z. [Epub ahead of print].
Thomas, S. W., Joly, G. D., and Swager, T. M. (2007). Chemical sensors based on amplifying fluorescent conjugated polymers. Chem. Rev. 107, 1339–1386. doi: 10.1021/cr0501339
Tsai, M. H., Hong, Y. H., Chang, C. H., Su, H. C., Wu, C. C., Matoliukstyte, A., et al. (2007). 3-(9-CarbazolyI)Carbazoles and 3,6-Di(9-Carbazolyl)Carbazoles as Effective Host Materials for Efficient Blue Organic Electrophosphorescence. Adv. Mater. 19, 862–866. doi: 10.1002/adma.200600822
Uoyama, H., Goushi, K., Shizu, K., Nomura, H., and Adachi, C. (2012). Highly efficient organic light-emitting diodes from delayed fluorescence. Nature 492, 234–238. doi: 10.1038/nature11687
Wang, P., Zhao, S., Xu, Z., Qiao, B., Long, Z., and Huang, Q. (2016). The electroluminescence mechanism of solution-processed TADF emitter 4CzIPN doped OLEDs investigated by transient measurements. Molecules 21:1365. doi: 10.3390/molecules21101365
Wong, M. Y., and Zysman-Colman, E. (2017). Purely organic thermally activated delayed fluorescence materials for organic light-emitting diodes. Adv. Mater. 29:22. doi: 10.1002/adma.201605444
Yang, M., Park, I. S., Miyashita, Y., Tanaka, K., and Yasuda, T. (2020). Mechanochromic delayed fluorescence switching in propeller-shaped carbazole–isophthalonitrile luminogens with stimuli-responsive intramolecular charge-transfer excited states. Angew. Chem. Int. Ed. Engl. 59, 13955–13961. doi: 10.1002/ange.202005584
Yersin, H., Czerwieniec, R., Shafikov, M. Z., and Suleymanova, A. F. (2018). “TADF material design: photophysical background and case studies focusing on Cu (I) and Ag (I) complexes,” in Highly Efficient OLEDs: Materials Based on Thermally Activated Delayed Fluorescence, ed Yersin H. (Weinheim: Wiley Periodicals), 1–60. doi: 10.1002/9783527691722.ch1
Yu, M., Huang, R., Guo, J., Zhao, Z., and Tang, B. Z. (2020). Promising applications of aggregation-induced emission luminogens in organic optoelectronic devices. PhotoniX 1, 1–33. doi: 10.1186/s43074-020-00012-y
Zeng, W., Lai, H. Y., Lee, W. K., Jiao, M., Shiu, Y. J., Zhong, C., et al. (2018). Achieving nearly 30% external quantum efficiency for orange–red organic light emitting diodes by employing thermally activated delayed fluorescence emitters composed of 1,8-naphthalimide-acridine hybrids. Adv. Mater. 30, 1–8. doi: 10.1002/adma.201704961
Zhang, D.-D., Suzuki, K., Song, X.-Z., Wada, Y., Kubo, S., Duan, L., et al. (2019a). Thermally activated delayed fluorescent materials combining intra- and intermolecular charge transfers. ACS Appl. Mater. Interfaces 11, 7192–7198. doi: 10.1021/acsami.8b19428
Zhang, H., Du, L., Wang, L., Liu, J., Wan, Q., Kwok, R. T. K., et al. (2019b). Visualization and manipulation of molecular motion in the solid state through photoinduced clusteroluminescence. J. Phys. Chem. Lett. 10, 7077–7085. doi: 10.1021/acs.jpclett.9b02752
Zhang, H., Zhao, Z., McGonigal, P. R., Ye, R., Liu, S., Lam, J. W. Y., et al. (2020). Clusterization-triggered emission: uncommon luminescence from common materials. Mater. Today 32, 275–292. doi: 10.1016/j.mattod.2019.08.010
Zhang, H., Zheng, X., Xie, N., He, Z., Liu, J., Leung, N. L. C., et al. (2017). “Why do simple molecules with ‘isolated' phenyl rings emit visible light?” J. Am. Chem. Soc. 139, 16264–16272. doi: 10.1021/jacs.7b08592
Zheng, K., Ni, F., Chen, Z., Zhong, C., and Yang, C. (2019). Polymorph-dependent thermally activated delayed fluorescence emitters: understanding TADF from a perspective of aggregation state. Angew. Chem. Int. Ed. Engl. 59, 9972–9976. doi: 10.1002/ange.201913210
Zhou, B., and Yan, D. (2019). Simultaneous long-persistent blue luminescence and high quantum yield within 2d organic–metal halide perovskite micro/nanosheets. Angew. Chem. Int. Ed. Engl. 58, 15128–145135. doi: 10.1002/anie.201909760
Zhu, F., Zhou, C., Zhou, D., and Yu, J. (2017). Improving electroluminescent efficiency and ultraviolet detectivity of optoelectronic integrated devices by doping a thermally activated delayed fluorescent material in an aggregation-induced emission material as an active layer. Opt. Mater. Express 7:3538. doi: 10.1364/OME.7.003538
Keywords: thermally activated delayed fluorescence (TADF), aggregation, solid state solvation effect, photophysics, organic light-emitting diodes (OLEDs)
Citation: Etherington MK (2020) Thermally Activated Delayed Fluorescence: Beyond the Single Molecule. Front. Chem. 8:716. doi: 10.3389/fchem.2020.00716
Received: 03 May 2020; Accepted: 13 July 2020;
Published: 18 September 2020.
Edited by:
Eli Zysman-Colman, University of St Andrews, United KingdomReviewed by:
Basem Moosa, KAUST Catalysis Center (KCC), Saudi ArabiaRadek Cibulka, University of Chemistry and Technology in Prague, Czechia
Copyright © 2020 Etherington. This is an open-access article distributed under the terms of the Creative Commons Attribution License (CC BY). The use, distribution or reproduction in other forums is permitted, provided the original author(s) and the copyright owner(s) are credited and that the original publication in this journal is cited, in accordance with accepted academic practice. No use, distribution or reproduction is permitted which does not comply with these terms.
*Correspondence: Marc K. Etherington, marc.k.etherington@northumbria.ac.uk