- Department of Applied Chemistry, College of Arts and Sciences, Northeast Agricultural University, Harbin, China
A novel “turn-on” fluorescent probe (PCN) was designed, synthesized, and characterized with perylene tetracarboxylic disimide as the fluorophore and Schiff base subunit as the metal ion receptor. The probe demonstrated a considerable fluorescence enhancement in the presence of Al3+ in DMF with high selectivity and sensitivity. Furthermore, the considerably “off–on” fluorescence response simultaneously led to the apparent color change from colorless to brilliant yellow, which could also be identified by naked eye easily. The sensing capability of PCN to Al3+ was evaluated by the changes in ultraviolet–visible, fluorescence, Fourier transform–infrared, proton nuclear magnetic resonance, and high-resolution mass spectrometry spectroscopies. The linear concentration range for Al3+ was 0–63 μM with a detection limit of 0.16 μM, which allowed for the quantitative determination of Al3+.
Introduction
Aluminum is the third most prevalent metal in the lithosphere, and it is toxic to living organisms due to its potential neurotoxicity in excessive amounts (Frantzios et al., 2000; Abeywickrama et al., 2019). Excess Al3+ will induce a wide range of diseases such as microcytic hypochromic anemia, osteoporosis, muscular atrophy, and Alzheimer's disease (Cronan et al., 1986; Fasman, 1996; Nayak, 2002; Walton, 2007; Zhang et al., 2020). According to the recommendation of the World Health Organization, the tolerable value of average human intake of Al3+ ions is around 3.0–10.0 mg/day (Valeur and Leray, 2000; Qin et al., 2014). Moreover, the environment may be polluted due to high level of Al3+ in the ecosystem (Godbold et al., 1988; Sade et al., 2016; Ye et al., 2019a). Fluorescence techniques for detecting various ions have become an optimal choice due to their high sensitivity and selectivity (Suresh et al., 2018; Ye et al., 2019b; Bai et al., 2020; Wu et al., 2020; Zhao et al., 2020). In the past few years, various fluorescent chemosensors including coumarin, naphthalimide, pyrene, BODIPY, anthracene, and rhodamine were exploited for detection of Al3+ (Samanta et al., 2014; Hossain et al., 2017; Kozlov et al., 2019; Prabhu et al., 2019; Roy et al., 2019; Li et al., 2020).
Perylene tetracarboxylic disimide derivatives (PDIs), as a representative of strong fluorescence and superior functional organic dyes, display exceptional optical, redox, and electrochemical properties and high thermal stability (Ahrens et al., 2003; Wurthner, 2004; Wasielewski, 2006; Xu et al., 2008; Luo and Chen, 2013; Gao et al., 2020). In addition to their industrial application as pigment, many PDIs also exhibit unique spectroscopical, near-unity fluorescence quantum yields, and strong electron-deficient nature. Owing to the delocalization effect and rigid plane, PDIs are endowed with high fluorescence quantum yields and have been widely utilized as a chromophore (Miyake et al., 2006; Soh et al., 2006; He et al., 2007; Kirschning et al., 2007; Yan et al., 2009; Ruan et al., 2010; Nanashima et al., 2012; Zhang et al., 2013). Fluorescent chemosensors based on modified perylene dye have been used to monitor various ions. PDI-based 2-thiophenamine derivative was reported for the selective determination of Hg2+ with a detection limit of 2.2 μM in DMF–H2O (1:1, v/v; Malkondu and Erdemir, 2015). A new water-soluble fluorescent probe was given by the condensation polymerization between 2,2′:6′,2″-terpyridine-containing dibromide and perylene diimide–diamines, which was used to determine Fe3+ and monitors the Fe3+/Fe2+ transition after the addition of a reducing agent such as ascorbic acid (Vc; Jin et al., 2016).
Herein, a novel perylenebisimide-based fluorescent sensor for monitoring Al3+ had been designed (Scheme 1; Erdemir and Kocyigit, 2018; Kashyap et al., 2019; Kumar et al., 2019; Erdemir et al., 2020). N,N′-bis[(2-p-chlorobenzaldehyde)-ethyl] perylene-3,4,9,10-tetracarboxylic diimide (PCN) was obtained via the polycondensation reaction of amino functionalized perylene-3,4,9,10-tetracarboxylic diimide and p-chlorobenzaldehyde (Scheme 2) and characterized by Fourier transform–infrared (FT-IR), proton nuclear magnetic resonance (1H NMR), carbon-13 nuclear magnetic resonance (13C NMR), and high-resolution mass spectrometry (HRMS) spectroscopies. It showed drastic fluorescence enhancement and obvious color change toward its binding of Al3+ in DMF solution with excellent selectivity and sensitivity.
Experimental
Materials and Instruments
All chemicals and solvents were purchased from commercial providers and used without purifying. FT-IR spectra were measured using a Bruker ALPHA-T spectrometer (KBr, Bruker, Germany). The 1H NMR and 13C NMR spectra were recorded on a Bruker AVANVE 400 MHz system (Bruker, Germany) using CF3COOD as the solvent. The HRMS was carried out on an FTMS Ultral Apex MS spectrometer (Bruker Daltonics Inc., USA). The ultraviolet–visible (UV-vis) spectra were gained on a UV-2550 ultraviolet spectrophotometer (Shimadzu, Japan). The fluorescence spectra were obtained through the PerkinElmer LS55 fluorescence spectrometer (PerkinElmer, UK). All pH values were made with PHS-3C pH meter (Inesa, China).
Synthesis of N,N′-bis(ethylenediamine)Perylene-3,4,9,10-Tetracarboxylic Bisimide (2)
The N,N′-bis(ethylenediamine)perylene-3,4,9,10-tetracarboxylic bisimide (2) was synthesized according to the reported literatures (Cheng et al., 2009; Chang et al., 2012). A solution of ethylene diamine (3.0 g, 10 mmol) and perylene-3,4,9,10-tetracarboxylic acid dianhydride (1; 1.0 g, 0.5 mmol) in 1-Butanol (100 mL) was refluxed with continuous stirring at 90°C. After refluxing for 24 h, the resulting mixture was treated with 0.2 M sodium acetate–acetic acid buffer solution (pH = 5.3, 50 mL). Then, 1 M NaOH was added to the filtrate until pH = 13. The residue was filtered and washed with water and methanol, and dried to give compound 2. Yield: 46 %. m.p. > 300°C. All spectra of structural characterization of compounds are in the Supporting Information. FT-IR data in KBr (cm−1): 3,360, 3,296 (v N-H), 2,933, 2,894, 2,841 (v C-H), and 1,674(v C=O). 1H NMR [δH in parts per million (ppm) in CF3COOD (TMS), 400 MHz]: 8.94–9.01 (m, 8H, Ar-H), 4.96 (br, 4H, -CH2-), and 3.98 (br, 4H, -CH2-). 13C NMR [δC in CF3COOD (TMS), 100 MHz]: 166.38, 136.31, 133.09, 129.33, 126.33, 124.34, 121.56, 116.19, 115.15, 113.38, 112.34, 40.57, and 38.40.
Synthesis of N,N′-bis[(2-p-Chlorobenzaldehyde)-ethyl]Perylene-3,4,9,10-Tetracarboxylic Diimide
The final product PCN was synthesized on the basis of the previous literatures (Malkondu and Erdemir, 2015; Fu et al., 2019a). Compound 2 (0.46 g, 1 mmol) was suspended first in MeOH/CHCl3 (1:1, 160 mL) followed by adding 0.7 g, 5 mmol p-chlorobenzaldehyde, and then the mixture was stirred at 50°C for 48 h. After cooling to room temperature, the precipitated solid was obtained and washed with methanol. The residue was recrystallized from MeOH/CHCl3 (v/v, 4:1) to obtain the dark-red solid. Yield: 84 %. m.p. > 300°C; FT-IR data in KBr (cm−1): 2,932, 2,822 (v C-H), 1,682, and 1,646 (v C=O); 1H NMR [δH in ppm in CF3COOD (TMS), 400 MHz]:8.99 (s, 2H, CH=N), 8.78 (s, 8H, Ar-H), 7.98–7.96 (d, 4H, Ar-H), 7.69–7.67 (d, 4H, Ar-H), 4.92 (br, 4H, -CH2-), and 4.57 (br, 4H, -CH2-). 13C NMR [δC in CF3COOD (TMS), 100 MHz]: 172.16, 165.69, 147.91, 136.05, 132.93, 132.79, 130.81, 129.10, 126.08, 124.25, 123.74, 121.38, 51.63, and 39.09. HRMS (ESI): calcd. for C42H26N4O4Cl2 ([M+H]+) 721.5862 found 721.1407.
Spectrophotometric Studies
The stock solution of PCN (10−3 M) was dissolved in DMF and then diluted to 10−5 M for the spectroscopic measurements of Al3+. The stock solutions of 10−2 mol L−1 concentration of metal ions were provided from AlCl3, FeCl3, CrCl3, MgCl2, PbCl2, ZnCl2, Hg(OAc)2, CaCl2, CuCl2, CoCl2, MnCl2, SnCl2, NiCl2, BaCl2, NaCl, KCl, and AgNO3 using ultrapure water. In the selectivity measurement, 10 mL of the PCN solution (1 × 10−5 M) and 50 μL of each metal ion stock solution (10−2 M) were added to volumetric flasks. The probe PCN stock solution (10 mL) was mixed with gradual incremental Al3+ solution separately for the titration experiments. These resulting solutions were well mixed, and then the spectral properties were recorded after 6 h. The excitation was set at 500 nm for the measurement of fluorescence, and slit width of the excitation was 10 nm. In spectral experiment, the concentration titration was measured at least twice to ensure consistent results. All the measurements were obtained at 25°C.
Results and Discussion
UV-Vis and Fluorescence Spectral Characteristics Studies
The solvent effect of PCN has been studied through fluorescence measurement in different solvents (Supplementary Figure 8). The probe exhibits weak fluorescence properties in almost all the investigated solvents except EtOH. The compound exhibits weak yellow fluorescence emission with peaks from 541 nm (MeCN) to 558 nm (DMSO) in most solvents, but much no fluorescence in EtOH was observed. Based on the response mode of the fluorescent molecular probe's “turn on” and “turn off,” the solvent was selected for further study. The probe had failed to show the good selectivity toward various ions in DMSO and other solvents. The photophysical property of the fabricated fluorescent chemosensor in DMF was investigated. Free probe (PCN) demonstrated weak fluorescence at about 550 and 590 nm. To estimate the selectivity and sensitivity of PCN (10 μM), the UV-vis and fluorescence spectra of PCN toward different metal cations (such as Al3+, Fe3+, Cr3+, Mg2+, Pb2+, Zn2+, Hg2+, Ca2+, Cu2+, Co2+, Mn2+, Sn2+, Ni2+, Ba2+, Na+, K+, and Ag+) have been investigated. UV-vis spectra of PCN were obtained in the existence of 5 equiv. of the tested cations. The absorption of PCN increased significantly in the presence of Al3+ at 490 and 525 nm, and the colorless solution of PCN changed to yellow under natural light, indicating that the “naked eye” is visible (Figure 1). The fluorescence responses of PCN were measured in the presence of fivefold excess of various metal ions (Figure 2). Upon the addition of different cations (5 equiv.), only when added the Al3+ into the solution can it induce a significant fluorescence enhancement at 550 and 590 nm. Moreover, a yellow-colored visual fluorescence change was observed after adding Al3+ ion to the PCN solution. In contrast, most of the other metal ions, including some mono-, di-, and trivalent metal ions (Ag+, Na+, K+, Mg2+, Pb2+, Zn2+, Hg2+, Ca2+, Cu2+, Co2+, Mn2+, Sn2+, Ni2+, Ba2+, Cr3+, and Fe3+), were unresponsive to this system. The imine bond in PCN was hydrolyzed by the addition of Al3+ due to the Lewis acid character of Al3+, and compound 2 with strong fluorescent was released. It resulted to a prominent “light-on” yellow solution and fluorescence emission of PCN, which allowed for naked-eye detection of Al3+ under natural light and UV light of 365 nm. This mechanism was proven by FT-IR, 1H NMR, and HRMS experiments. Therefore, PCN showed “off–on” response to Al3+ ions in the DMF solution. All these showed the good selectivity of PCN toward Al3+ over other cations.
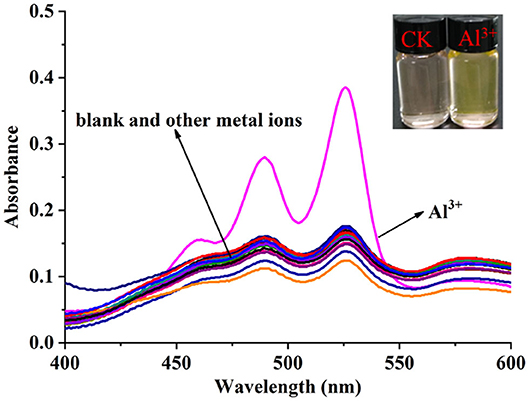
Figure 1. UV-vis spectra changes of the PCN solution (10 μM) after different metal ions (50 μM) were added in DMF. Inset: color changes of PCN solution before and after adding Al3+ (50 μM) under natural light.
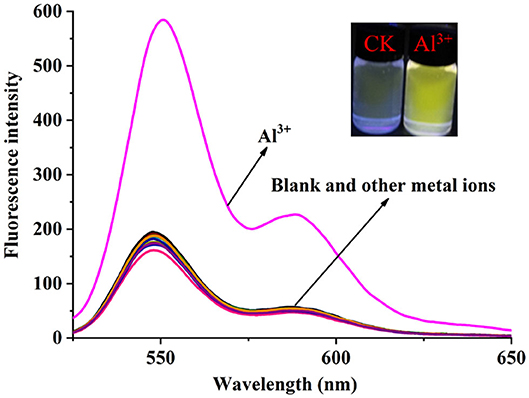
Figure 2. Fluorescence spectra changes of the PCN solution (10 μM) after different metal ions (50 μM) were added in DMF (λex = 500 nm). Inset: color changes of PCN solution before and after adding Al3+ (50 μM) under UV light of 365 nm.
UV-Vis and Fluorescence Titration Experiments
The UV-vis and fluorescence titrations were measured by increasing the amount of Al3+ (0–70 μM) to PCN in DMF. The intensity of absorbance at 490 and 525 nm increased gradually after the addition of an increasing amount of Al3+ (Figure 3). The absorbance of PCN became steady at 525 nm with the Al3+ concentration being 63 μM. The change of UV-vis spectral could attribute to the binding affinity of PCN. As depicted in Figure 4, the independent PCN exhibited a weak emission in DMF. However, a distinct increase in fluorescence emission was observed after Al3+ was added and a plateau with the addition of 63 μM of Al3+ was achieved at 550 nm. The weak fluorescence may correspond to the photoinduced electron transfer (PET) process resulted from the N atom of imine (C=N) to the luminescent perylene unit (Upadhyay et al., 2018; Fu et al., 2019b). With the increase of Al3+, the PET effect of the sensor PCN is inhibited, and thereby the intense fluorescence of PDI units is restored. Also, it clearly indicates the structural change of PCN by the interaction of Al3+ with PCN. As shown in Figure 5A, the fluorescence intensity showed a linear relationship (R2 = 0.9956) with concentration of Al3+ in the range of 0–63 μM, indicating that PCN could be used to determine the Al3+ quantitatively. The detection limit of PCN for sensing Al3+was 0.16 μM based on applying equation LOD = 3σ/k, which was calculated from the linear regression curve of the fluorescence intensity and the Al3+ concentration. This indicated that PCN had high fluorescence selectivity to Al3+. In addition, the binding constant, K, could also be obtained from the Stern–Volmer equation I0/(I0 − I) = 1/A + 1/KA · 1/[Q]. The K for Al3+ was calculated to be 7.75 × 104 M−1 in the DMF solution, as increased from the fluorescence titration curves of probe PCN with Al3+ (Figure 5B). The comparison of probe PCN with other Al3+ chemical sensors based on Schiff's base was summarized in Table 1 with different sensing mechanisms (Gan et al., 2017; Roy et al., 2017; Tajbakhsh et al., 2017; Shen et al., 2018; Wang et al., 2018; Zhang et al., 2018; Fu et al., 2019). Compared with other sensors, the advantage of probe PCN was its lower detection limit, but its insolubility in water was its shortcoming, which might limit its application in biological and environmental chemistry to some extent.
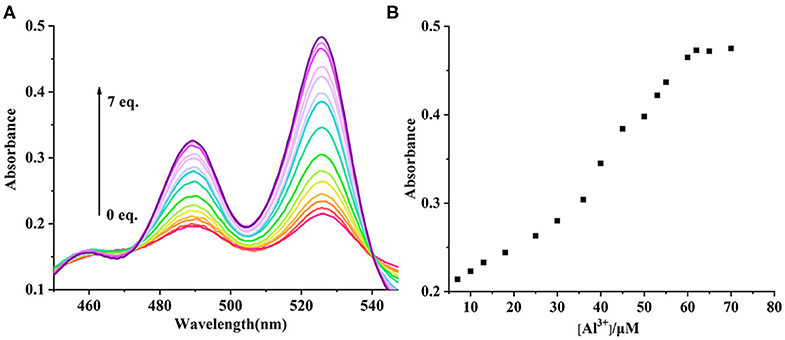
Figure 3. (A) UV-vis spectra recorded for probe PCN (10 μM) in DMF upon spectrometric titration with Al3+ (0–70 μM). (B) The relationship between the absorbance (525 nm) and the concentration of Al3+.
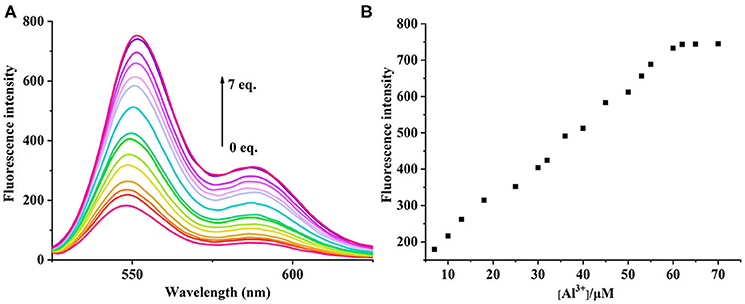
Figure 4. (A) Fluorescence spectra recorded for probe PCN (10 μM) in DMF upon spectrometric titration with Al3+ (0–70 μM). (B) The relationship between the fluorescence intensity (550 nm) and the concentration of Al3+.
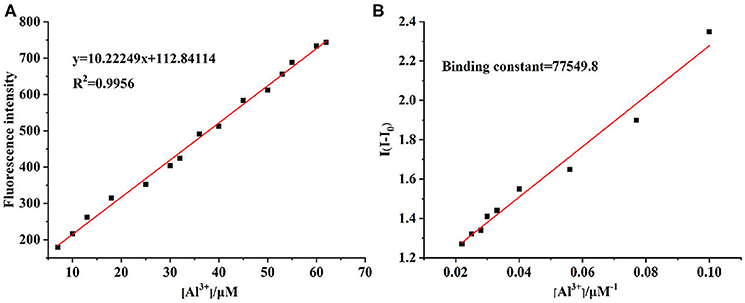
Figure 5. (A) The linear responses of fluorescence intensity (550 nm) with Al3+ concentrations. (B) Benesie–Hildebrand plot of PCN between PCN and Al3+ in DMF.
The Competition Experiments Studies
The fluorescence spectra of PCN were investigated to examine its selectivity in the existence of other metal ions. First, 10 equiv. of background metal ions (100 μM) was added to the solution of PCN (10 μM) to form a PCN/Mn+ system, and then, 5 equiv. of Al3+ (50 μM) was added into the solution. As shown in Figure 6, Al3+ detection by compound PCN was not influenced by the selected background metal cations. Therefore, the combined results clearly indicated that PCN exhibited remarkable Al3+ signaling behavior and can function as a high selectivity and disturbance-free Al3+ fluorescent probe even in the existence of most competing metal cations.
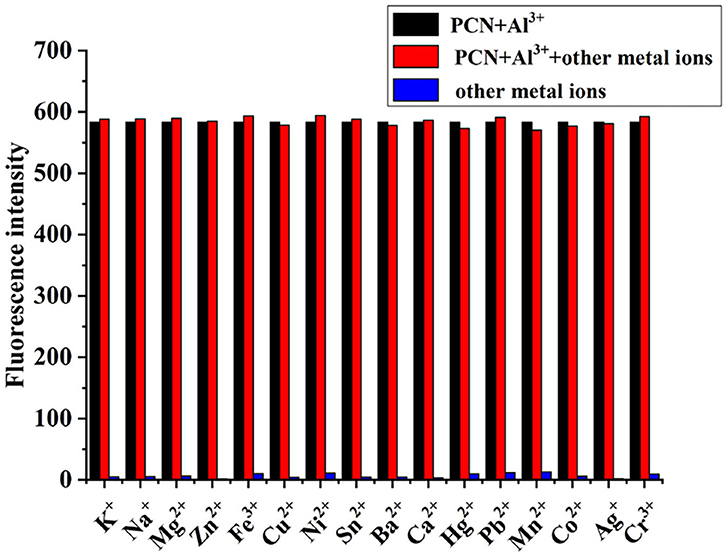
Figure 6. Competition selectivity of PCN (10 μM) toward Al3+ (50 μM) in the presence of other competition metal ions (100 μM) with emission at 550 nm.
Sensing Mechanism of PCN for Al3+
To elucidate the sensing mechanism, the IR, NMR, and HRMS spectra of PCN-Al3+ were performed. The FT-IR spectroscopic analysis of PCN and PCN–Al3+ complexes is shown in Figure 7. Compared with the FT-IR spectra of PCN, the peaks appeared at 3,386 and 3,323 cm−1 in the FT-IR spectra after the addition of 5 equiv. of Al3+, which is consistent with the amino peak of intermediate 2. The result suggested that intermediate 2 might be regenerated, which is consistent with the previous spectral analysis. The 1H NMR spectroscopic analysis of PCN and the reaction product of PCN with Al3+ are shown in Figure 8. The addition of Al3+ resulted in different peak profiles, the signal of aldimine protons (H4) at 8.99 ppm completely disappeared, and these peaks at 7.67–7.98 ppm corresponding to aromatic protons (H5) also disappeared comparing with 1H NMR spectra, which also confirmed that probe PCN was hydrolyzed. Moreover, by comparing the HRMS spectra in Figure 9, it was found that the original peak at [M+H]+ 721.1407 for free PCN disappeared, and a new peak at [M+H]+ 477.1552 emerged after the addition of Al3+. All results clearly delineated that Al3+ induced the cleavage of imine. In order to get full insight into the mechanism, the fluorescence spectra of compound 2 and PCN in the absence and presence of Al3+ were recorded separately in Figure 10. Upon the spectral changes of PCN induced by Al3+, it was found that the spectral data were nearly identical with those of compound 2, which clearly confirmed the cleavage of the C=N of PCN in the presence of Al3+(Scheme 3).
In order to understand the sensing mechanism in the probe PCN in the absence or presence of Al3+ ion, density functional theory (DFT) quantum mechanical approach was performed. A Gaussian program (Frisch et al., 2009) was employed for DFT calculations at the B3LYP-D3BJ/def2-SV(P) level (Stephens et al., 1994; Weigend and Ahlrichs, 2005; Frisch et al., 2009; Grimme et al., 2010). The S1 state geometry was optimized, and the corresponding molecular orbitals were recorded by isosurfaces with the isovalue at 0.02. In the excite state of PCN, HOMO-1 (−6.62 eV) of the receptor unit is close to fluorophore HOMO (−6.16 eV) and located above the fluorophore HOMO-9 (−7.67eV). Hence, the electron of the HOMO-1 will be transferred to fluorophore regime through the reductive PET mechanism (Maity et al., 2019; Dos Santos Carlos et al., 2020). Significantly, the fluorescent “off” state of PCN is observed. After Al3+ ion hydrolyzed the probe PCN, the HOMO-2 (−6.44 eV) energy levels of the primary amine are decreased than that of the fluorophore HOMO-1 (−6.18 eV), as also observed. Hence, PET could not efficiently operate from the HOMO-2 of the primary amine to the fluorophore's HOMO-1 upon the removal of imine moiety, resulting in the fluorescent “on” state (Figure 11). Thus, the present calculation demonstrated that the electron donor imine leads to a highly efficient PET process.
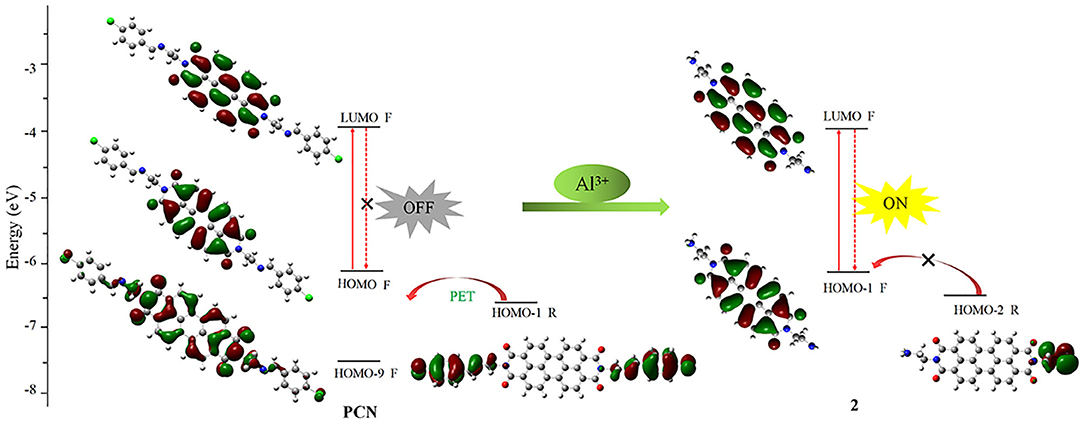
Figure 11. Frontier orbital energy diagram and electron transfer path in PCN and compound 2 (F, fluorophore; R, receptor segment).
Conclusion
In summary, a novel PDI-based Schiff base derivative PCN was synthesized and utilized as a fluorescent probe. PCN exhibited a selective turn-on response to Al3+ over other coexisting competitive metal ions in DMF. DFT calculations showed that coordination of PCN to Al3+ inhibits the PET process. The C=N of PCN was hydrolyzed by Al3+, leading to the return to the intermediate compound, which resulted naked-eye visible color changes from colorless to yellow and nonfluorescent to yellow fluorescent. The detection limit was sufficiently low to determine the micromolar levels of Al3+. This sensor is valuable for Al3+ analysis in environmental samples.
Data Availability Statement
The original contributions presented in the study are included in the article/Supplementary Material, further inquiries can be directed to the corresponding author/s.
Author Contributions
YL and SG constructed the workflow, performed the data analysis, and wrote the manuscript. X-ML synthesized and purified the compound. LY and Y-LL contributed to data analysis. FY and YF contributed to the conception of the study, revised the manuscript, and approved the final version. All authors contributed to the article and approved the submitted version.
Conflict of Interest
The authors declare that the research was conducted in the absence of any commercial or financial relationships that could be construed as a potential conflict of interest.
Acknowledgments
This work was supported by the Natural Science Foundation of China (51903032), the Natural Science Foundation of Heilongjiang Province (LH2019B002), Postdoctoral Science Foundation of Heilongjiang Province (No. LBH-Z19045), and Young Talents Project of Northeast Agricultural University (No. 18QC64). The authors are grateful to Prof. Min Zhang (Northeast Normal University) for the assistance with the DFT calculation and analysis.
Supplementary Material
The Supplementary Material for this article can be found online at: https://www.frontiersin.org/articles/10.3389/fchem.2020.00702/full#supplementary-material
References
Abeywickrama, C. S., Bertman, K. A., and Pang, Y. (2019). A bright red-emitting flavonoid for Al3+ detection in live cells without quenching ICT fluorescence. Chem. Commun. 55, 7041–7044. doi: 10.1039/C9CC02322D
Ahrens, M. J., Fuller, M. J., and Wasielewski, M. R. (2003). Cyanated Perylene-3,4-dicarboximides and Perylene-3,4:9,10-bis(dicarboximide): facile chromophoric oxidants for organic photonics and electronics. Chem. Mater. 15, 2684–2686. doi: 10.1021/cm034140u
Bai, C. B., Wang, W. G., Zhang, J., Wang, C., Qiao, R., Wei, B., et al. (2020). A Fluorescent and colorimetric chemosensor for Hg2+ based on rhodamine 6G with a two-step reaction mechanism. Front. Chem. 8:14. doi: 10.3389/fchem.2020.00014
Chang, T. J., Liu, X. J., Cheng, X. H., Qi, C., Mei, H. C., and Shangguan, D. H. (2012). Selective isolation of G-quadruplexes by affinity chromatography. J. Chromatogr. A. 1246, 62–68. doi: 10.1016/j.chroma.2012.02.026
Cheng, X. H., Liu, X. J., Bing, T., Cao, Z. H., and Shangguan, D. H. (2009). General peroxidase activity of G-quadruplex-hemin complexes and its application in ligand screening. Biochemistry 48, 7817–7823. doi: 10.1021/bi9006786
Cronan, C. S., Walker, W. J., and Bloom, P. R. (1986). Predicting aqueous aluminium concentrations in natural waters. Nature 324, 140–143. doi: 10.1038/324140a0
Dos Santos Carlos, F., Monteiro, R. F., da Silva, L. A., Zanlorenzi, C., and Nunes, F. S. (2020). A highly selective acridine-based fluorescent probe for detection of Al3+ in alcoholic beverage samples. Spectrochim. Acta A. 231:118119. doi: 10.1016/j.saa.2020.118119
Erdemir, S., Karakurt, S., and Malkondu, S. (2020). Unusual “OFF-ON” fluorescent sensor including a triazole unit for Al3+ detection via selective imine hydrolysis and its cell image study. Analyst. 145, 3725–3731. doi: 10.1039/c9an02500f
Erdemir, S., and Kocyigit, O. (2018). Dual recognition of Zn2+ and Al3+ ions by a novel probe containing two fluorophore through different signaling mechanisms. Sensor. Actuat. B-Chem. 273, 56–61. doi: 10.1016/j.snb.2018.06.019
Fasman, G. D. (1996). Aluminum and Alzheimer's disease: model studies. Coordin. Chem. Rev. 149, 125–165. doi: 10.1016/S0010-8545(96)90020-X
Frantzios, G., Galatis, B., and Apostolakos, P. (2000). Aluminium effects on microtubule organization in dividing root-tip cells of Triticum turgidum. I. Mitotic cells. New Phytol. 145, 211–224. doi: 10.1046/j.1469-8137.2000.00580.x
Frisch, M., Trucks, G. W., Schlegel, H. B., Scuseria, G. E., Robb, M. A., Cheeseman, J. R., et al. (2009). Others, Gaussian 09, revision D. 01. Wallingford, CT: In Gaussian, Inc.
Fu, J. X., Yao, K., Chang, Y. X., Li, B., Yang, L., and Xu, K. X. (2019). A novel colorimetric-fluorescent probe for Al3+ and the resultant complex for F− and its applications in cell imaging. Spectrochim. Acta A. 222:7234. doi: 10.1016/j.saa.2019.117234
Fu, Y., Pang, X. X., Wang, Z. Q., Chai, Q., and Ye, F. (2019a). A highly sensitive and selective fluorescent probe for determination of Cu (II) and application in live cell imaging. Spectrochim. Acta. A. 208, 198–205. doi: 10.1016/j.saa.2018.10.005
Fu, Y., Zhang, D., Zhang, S. Q., Liu, Y. X., Guo, Y. Y., Wang, M. X., et al. (2019b). Discovery of N-aroyl diketone/triketone derivatives as novel 4-hydroxyphenylpyruvatedioxygenase inhibiting-based herbicides. J. Agr. Food Chem. 67, 11839–11847. doi: 10.1021/acs.jafc.9b01412
Gan, X. P., Li, W., Li, C. X., Wu, Z. C., Liu, D., Huang, B., et al. (2017). Two analogously structural triphenylamine-based fluorescent “off-on” probes for Al3+ via two distinct mechanisms and cell imaging application. Sensor. Actuat. B-Chem. 239, 642–651. doi: 10.1016/j.snb.2016.08.042
Gao, S., Jiang, J. Y., Li, X. M., Liu, Y. Y., Zhao, L. X., Fu, Y., et al. (2020). Enhanced physicochemical properties and herbicidal activity of an environment-friendly clathrate formed by β-cyclodextrin and herbicide cyanazine. J. Mol. Liq. 305:112858. doi: 10.1016/j.molliq.2020.112858
Godbold, D. L., Fritz, E., and Huttermann, A. (1988). Aluminum toxicity and forest decline. Proc. Natl. Acad. Sci. U.S.A. 85, 3888–3892. doi: 10.1073/pnas.85.11.3888
Grimme, S., Antony, J., Ehrlich, S., and Krieg, H. (2010). A consistent and accurate ab initio parametrization of density functional dispersion correction (DFT-D) for the 94 elements H-Pu. J. Chem. Phys. 132:154104. doi: 10.1063/1.3382344
He, X. R., Zhong, Z. F., Guo, Y. B., Lv, J., Xu, J. L., Zhu, M., et al. (2007). Gold nanoparticle-based monitoring of the reduction of oxidized to reduced glutathione. Langmuir. 23, 8815–8819. doi: 10.1021/la7011028
Hossain, S. M., Singh, K., Lakma, A., Pradhan, R. N., and Singh, A. K. (2017). A schiff base ligand of coumarin derivative as an ICT-Based fluorescence chemosensor for Al3+. Sensor. Actuat. B-Chem. 239, 1109–1117. doi: 10.1016/j.snb.2016.08.093
Jin, L., Liu, C., An, N. Q., Zhang, Q., Wang, J., Zhao, L. L., et al. (2016). Fluorescence turn-on detection of Fe3+ in pure water based on a cationic poly(perylene diimide) derivative. RSC Adv. 6, 58394–58400. doi: 10.1039/c6ra08267j
Kashyap, K. S., Kumar, A., Hira, S. K., and Dey, S. (2019). Recognition of Al3+ through the off-on mechanism as a proficient driving force for the hydrolysis of BODIPY conjugated Schiff base and its application in bio-imaging. Inorg. Chim. Acta. 498:9157. doi: 10.1016/j.ica.2019.119157
Kirschning, A., Taft, F., and Knobloch, T. (2007). Total synthesis approaches to natural product derivatives based on the combination of chemical synthesis and metabolic engineering. Org. Biomol. Chem. 5:3245. doi: 10.1039/b709549j
Kozlov, M. A., Uvarov, D. Y., Gorbatov, S. A., Kolotirkina, N. G., Averin, A. D., Kachala, V. V., et al. (2019). Pseudo-crown ethers as a novel scaffold for the development of Al3+-selective fluorescent probes. Eur. J. Org. Chem. 26, 4196–4206. doi: 10.1002/ejoc.201900315
Kumar, G., Rani, R., Paul, K., and Luxami, V. (2019). Single molecular platform displaying PET and hydrolysis sensing mechanism for differential detection of metal ions. J. Photoch. Photobio. A. 380, 111845. doi: 10.1016/j.jphotochem.2019.05008
Li, Z., Chen, W., Dong, L., Song, Y., Li, R., Li, Q., et al. (2020). A novel ratiometric and reversible fluorescent probe based on naphthalimide for the detection of Al3+ and pH with excellent selectivity. New J. Chem. 44, 3261–3267. doi: 10.1039/C9NJ06309A
Luo, M. H., and Chen, K. Y. (2013). Asymmetric perylene bisimide dyes with strong solvatofluorism. Dyes Pigments. 99, 456–464. doi: 10.1016/j.dyepig.2013.05033
Maity, A., Ghosh, U., Giri, D., Mukherjee, D., Maiti, T. K., and Patra, S. K. (2019). A water-soluble BODIPY based “OFF/ON” fluorescence probe for the detection of Cd2+ ions with high selectivity and sensitivity. Dalton T. 48, 2108–2118. doi: 10.1039/c8dt04016h
Malkondu, S., and Erdemir, S. (2015). A novel perylene-bisimide dye as “turn on” fluorescent sensor for Hg2+ ion found in DMF/H2O. Dyes Pigments. 113, 763–769. doi: 10.1016/j.dyepig.2014.10.020
Miyake, Y., Togashi, H., Tashiro, M., Yamaguchi, H., Oda, S., Kudo, M., et al. (2006). MercuryII-mediated formation of thymine-Hg-II-thymine base pairs in DNA duplexes. J. Am. Chem. Soc. 128, 2172–2173. doi: 10.1021/ja056354d
Nanashima, Y., Yokoyama, A., and Yokozawa, T. (2012). Synthesis of well-defined Poly(2-alkoxypyridine-3,5-diyl) via Ni-catalyst-transfer condensation polymerization. Macromolecules. 45, 2609–2613. doi: 10.1021/ma202756y
Nayak, P. (2002). Aluminum: impacts and disease. Environ. Res. 89, 101–115. doi: 10.1006/enrs.2002.4352
Prabhu, J., Velmurugan, K., Raman, A., Duraipandy, N., Kiran, M. S., Easwaramoorthi, S., et al. (2019). Pyrene-phenylglycinol linked reversible ratiometric fluorescent chemosensor for the detection of aluminium in nanomolar range and its bio-imaging. Anal. Chim. Acta. 1090, 114–124. doi: 10.1016/j.aca.2019.09.008
Qin, J. C., Li, T. R., Wang, B. D., Yang, Z. Y., and Fan, L. (2014). A sensor for selective detection of Al3+ based on quinoline Schiff-base in aqueous media. Synthetic Met. 195, 141–146. doi: 10.1016/j.synthmet.2014.06.002
Roy, A., Das, S., Sacher, S., Mandal, S. K., and Roy, P. (2019). Rhodamine based biocompatible chemosensor for Al3+, Cr3+ and Fe3+ ions: extraordinary fluorescence enhancement and precursor for future chemosensors. Dalton T. 47, 17594–17604. doi: 10.1039/C9DT03833G
Roy, A., Dey, S., Halder, S., and Roy, P. (2017). Development of a new chemosensor for Al3+ ion: tuning of properties. J. Lumin. 192, 504–512. doi: 10.1016/j.jlumin.2017.07.020
Ruan, Y. B., Li, A. F., Zhao, J. S., Shen, J. S., and Jiang, Y. B. (2010). Specific Hg2+-mediated perylene bisimide aggregation for highly sensitive detection of cysteine. Chem. Commun. 46:4938. doi: 10.1039/c0cc00630k
Sade, H., Meriga, B., Surapu, V., Gadi, J., Sunita, M. S. L., Suravajhala, P., et al. (2016). Toxicity and tolerance of aluminum in plants: tailoring plants to suit to acid soils. Biometals 29, 187–210. doi: 10.1007/s10534-016-9910-z
Samanta, S., Goswami, S., Ramesh, A., and Das, G. (2014). A new fluorogenic probe for solution and intra-cellular sensing of trivalent cations in model human cells. Sensor. Actuat. B-Chem. 194, 120–126. doi: 10.1016/j.snb.2013.12.049
Shen, K. S., Mao, S. S., Shi, X. K., Wang, F., Xu, Y. L., and Aderinto, S. O. (2018). Characterization of a highly Al3+-selective fluorescence probe based on naphthalimide-Schiff base and its application to practical water samples. Luminescence. 33, 54–63. doi: 10.1002/bio.3372
Soh, N., Ariyoshi, T., Fukaminato, T., Nakano, K., Irie, M., and Imato, T. (2006). Novel fluorescent probe for detecting hydroperoxides with strong emission in the visible range. Bioorg. Med. Chem. Lett. 16, 2943–2946. doi: 10.1016/j.bmcl.2006.02.078
Stephens, P. J., Devlin, F. J., Chabalowski, C. F., and Frisch, M. J. (1994). Ab initio calculation of vibrational absorption and circular dichroism spectra using density functional force fields. J. Phys. Chem. 98, 11623–11627. doi: 10.1021/j100096a001
Suresh, S., Bhuvanesh, N., Prabhu, J., Thamilselvan, A., Rajkumar, S. R. J., Kannan, K., et al. (2018). Pyrene based chalcone as a reversible fluorescent chemosensor for Al3+ ion and its biological applications. J. Photoch. Photobio. A. 359, 172–182. doi: 10.1016/j.jphotochem.2018.04.009
Tajbakhsh, M., Chalmardi, G. B., Bekhradnia, A., Hosseinzadeh, R., Hasani, N., and Amiri, M. A. (2017). A new fluorene-based Schiff-base as fluorescent chemosensor for selective detection of Cr3+ and Al3+. Spectrochim. Acta A. 189, 22–31. doi: 10.1016/j.saa.2017.08.007
Upadhyay, Y., Anand, T., Babu, L. T., Paira, P., Kumar Sk, A., Kumar, R., et al. (2018). Combined use of spectrophotometer and smartphone for the optical detection of Fe3+, using a vitamin B 6, cofactor conjugated pyrene derivative and its application in live cells imaging. J. Photoch. Photobio A. 361, 34–40. doi: 10.1016/j.jphotochem.2018.05.002
Valeur, B., and Leray, I. (2000). Design principles of fluorescent molecular sensors for cation recognition. Coordin. Chem. Rev. 205, 3–40. doi: 10.1016/S0010-8545(00)00246-0
Walton, J. R. (2007). An aluminum-based rat model for Alzheimer's disease exhibits oxidative damage, inhibition of PP2A activity, hyperphosphorylated tau, and granulovacuolar degeneration. J. Inorg. Biochem. 101, 1275–1284. doi: 10.1016/j.jinorgbio.2007.06.001
Wang, Q., Wen, X. Y., and Fan, Z. F. (2018). A Schiff base fluorescent chemsensor for the double detection of Al3+ and PPi through aggregation induced emission in environmental physiology. J. Photoch. Photobio. A. 358, 92–99. doi: 10.1016/j.jphotochem.2018.03.004
Wasielewski, M. R. (2006). Energy, charge, and spin transport in molecules and self-assembled nanostructures inspired by photosynthesis. J. Org. Chem. 71, 5051–5066. doi: 10.1021/jo060225d
Weigend, F., and Ahlrichs, R. (2005). Balanced basis sets of split valence, triple zeta valence and quadruple zeta valence quality for H to Rn: design and assessment of accuracy. Phys. Chem. Chem. Phys. 7, 3297. doi: 10.1039/b508541a
Wu, N., Zhao, L. X., Jiang, C. Y., Li, P., Liu, Y. L., Fu, Y., et al. (2020). A naked-eye visible colorimetric and fluorescent chemosensor for rapid detection of fluoride anions: Implication for toxic fluorine-containing pesticides detection. J. Mol. Liq. 302, 112549. doi: 10.1016/j.molliq.2020.112549
Wurthner, F. (2004). Perylene bisimide dyes as versatile building blocks for functional supramolecular architectures. Chem. Commun. 14, 1564–1579. doi: 10.1039/b401630k
Xu, W., Chen, H., Wang, Y., Zhao, C., Li, X., Wang, S., et al. (2008). photoinduced electron and energy transfer in dyads of porphyrin dimer and perylene tetracarboxylic diimide. Chem. Phys. Chem. 9, 1409–1415. doi: 10.1002/cphc.200800028
Yan, L. W., Yang, L., Lan, J. B, and You, J. S. (2009). A new perylene diimide-based colorimetric and fluorescent sensor for selective detection of Cu2+ cation. Sci. China Ser. B. 52, 518–522. doi: 10.1007/s11426-009-0041-z
Ye, F., Liang, X. M., Xu, K. X., Pang, X. X., Chai, Q., and Fu, Y. (2019a). A novel dithiourea-appended naphthalimide “on-off” fluorescent probe for detecting Hg2+ and Ag+ and its application in cell imaging. Talanta. 200, 494–502. doi: 10.1016/j.talanta.2019.03.076
Ye, F., Zhai, Y., Guo, K. L., Liu, Y. X., Li, N., Gao, S., et al. (2019b). Safeners improve maize tolerance under herbicide toxicity stress by increasing the activity of enzymes in vivo. J. Agr. Food Chem. 67, 11568–11576. doi: 10.1021/acs.jafc.9b03587
Zhang, L., Wang, Y. F., Yu, J. J., Zhang, G. J., Cai, X. F., Wu, Y., et al. (2013). A colorimetric and fluorescent sensor based on PBIs for palladium detection. Tetrahedron Lett. 54, 4019–4022. doi: 10.1016/j.tetlet.2013.05.076
Zhang, X., Sun, P., Li, F., Li, H., Zhou, H. P., Wang, H., et al. (2018). A tissue-permeable fluorescent probe for Al (III), Cu (II) imaging in vivo. Sensor. Actuat. B-Chem. 255, 366–373. doi: 10.1016/j.snb.2017.07.196
Zhang, Y. Y., Gao, S., Liu, Y. X., Wang, C., Jiang, W., Zhao, L. X., et al. (2020). Design, synthesis and biological activity of novel diazabicyclo derivatives as safeners. J. Agr. Food Chem. 68, 3403–3414. doi: 10.1021/acs.jafc.9b07449
Keywords: fluorescence, perylened derivatives, Al3+, turn on, hydrolysis
Citation: Liu Y, Gao S, Yang L, Liu Y-L, Liang X-M, Ye F and Fu Y (2020) A Highly Selective Perylenediimide-Based Chemosensor: “Naked-Eye” Colorimetric and Fluorescent Turn-On Recognition for Al3+. Front. Chem. 8:702. doi: 10.3389/fchem.2020.00702
Received: 15 May 2020; Accepted: 07 July 2020;
Published: 11 September 2020.
Edited by:
Xiaoming He, Shaanxi Normal University, ChinaReviewed by:
Lokesh K. Kumawat, Maynooth University, IrelandNarayanan Selvapalam, Kalasalingam University, India
Copyright © 2020 Liu, Gao, Yang, Liu, Liang, Ye and Fu. This is an open-access article distributed under the terms of the Creative Commons Attribution License (CC BY). The use, distribution or reproduction in other forums is permitted, provided the original author(s) and the copyright owner(s) are credited and that the original publication in this journal is cited, in accordance with accepted academic practice. No use, distribution or reproduction is permitted which does not comply with these terms.
*Correspondence: Fei Ye, yefei@neau.edu.cn; Ying Fu, fuying@neau.edu.cn
†These authors have contributed equally to this work