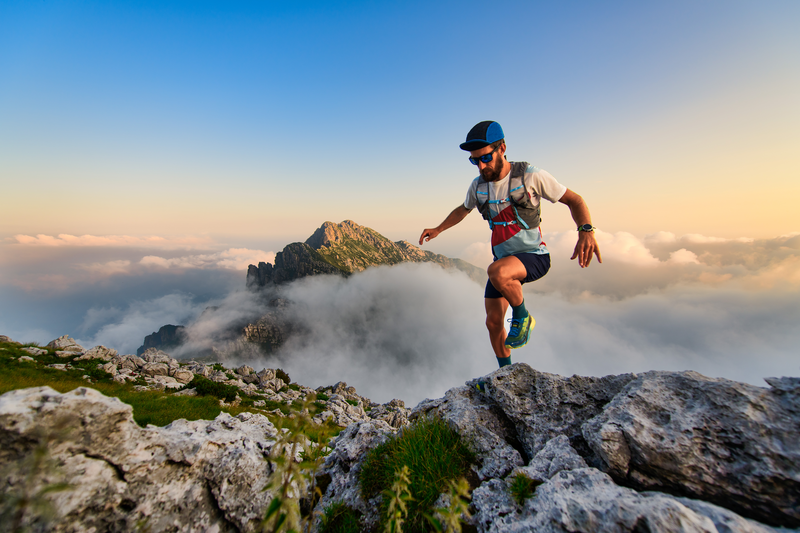
94% of researchers rate our articles as excellent or good
Learn more about the work of our research integrity team to safeguard the quality of each article we publish.
Find out more
ORIGINAL RESEARCH article
Front. Chem. , 24 July 2020
Sec. Nanoscience
Volume 8 - 2020 | https://doi.org/10.3389/fchem.2020.00611
This article is part of the Research Topic Fluorescent Carbon-based Nanostructures for Bioimaging Applications View all 6 articles
Multifunctional nanocomposites can combine multiple functions into a single nanosystem and thus have attracted extensive interest in various fields. The combination of magnetic and upconversion luminescent nanoparticles into one single nanoplatform, which have a good application in biomedical fields such as bio-magnetic separation, magnetic resonance imaging (MRI), and optical imaging, is highly desirable. Here we reported multifunctional nanocomposites by using hollow carbon sphere to integrate magnetic Fe3O4 and upconversion nanoparticles (UCNPs) into one nanosystem. The as-prepared UCNPs/Fe3O4@h-C have near-infrared (NIR) luminescence under 980 nm excitation and superparamagnetism. In addition, since the carbon layer can absorb NIR light and transfer it into heat with high efficiency, the nanocomposites can realize photo thermal (PT), upconversion luminescence (UCL) and MRI tri-mode imaging. The UCNPs/Fe3O4@h-C might be further utilized as a potential theranostic agent, including its in-depth monitoring through luminescent imaging and MRI diagnosis, as well as its direct use in tumors as a photothermal therapy (PTT) agent.
In recent years, multifunctional nanocomposite materials have attracted extensive interest because they can integrate multiple functionalities into one single nanosystem and thus endow them with great potential application in various areas (Gao et al., 2009; Liu Z. et al., 2011; Cheng et al., 2013; Jia et al., 2019; Sun et al., 2019; Liao et al., 2020). Among them, nanoparticles (NPs) with magnetism and luminescence have been extensively studied for their applications in biomedicine, for example, targeted enrichment and separation, magnetic targeted drug delivery, magnetic resonance imaging (MRI), biological luminescent probes (Cheng et al., 2016; Chen and Fu, 2018; Ding et al., 2018; Tang et al., 2018; Wang et al., 2018; Fu et al., 2019). Rare-earth doped upconversion luminescent nanomaterials (UCNPs) can emit high-energy photons of different wavelengths in visible or near-infrared (NIR) region by absorbing two or more low-energy photons in NIR region, this makes UCNPs have the prominent advantages over traditional phosphors in low autofluorescence background, shape emission bandwidths, good photochemical stability, and large tissue penetration depth (Xu et al., 2018; Zhu et al., 2018; Zhang et al., 2019). These unique properties proposed UCNPs as a new generation of optical materials in luminescent detections with high sensitivity, high resolution bioimaging, photodynamic therapies (PDT), and so on (Liu J. L. et al., 2011; Liu et al., 2017; Chen S. et al., 2018; Gu et al., 2019; Ma et al., 2019; Tang et al., 2019; Zhu et al., 2019). Magnetic nanoparticles are another kind of attractive materials for biomedicine because they can be fabricated using external magnetic fields. In consequence, the combination of magnetic nanoparticles and UCNPs into one nano-platform is highly pursued in biomedical applications, such as bio-magnetic separation, MRI and optical dual imaging, to improve the targeting efficiency and hyperthermia (Shen et al., 2010; Zhang et al., 2012; Zhong et al., 2012; Chen Z. X. et al., 2018).
Normally, UCNPs could be combined with magnetic materials in three ways. One is the SiO2-assisted synthetic strategy (Liu et al., 2008). Zhang et al. (2012) synthesized a kind of magnetic upconversion fluoride nanorattles Fe3O4@SiO2@α-NaYF4/Yb, Er (MUC-F-NR) through an ion-exchange method. The MUC-F-NR consisted a Fe3O4 magnetic core, a silica layer, a hollow space, and a NaYF4/Yb, Er shell. Further studies showed that the nanocomposites have low cytotoxicity, good cell imaging, and excellent tumor therapy efficacy in vivo when treated with an external magnetic field after loading with antitumor drug. The second is the cross-linker anchoring method. Shen et al. (2010) introduced 11-mercaptoundecanoic acid (MUA) or 1,10-decanedicarboxylic acid (DDA) as the crucial crosslinker to immobilize Fe3O4 NPs onto the surface of UCNPs, and synthesized the Fe3O4/NaYF4:Yb,Er hetero-NPs. These NPs could be well dispersed in water after ligand ozonolysis treatment, and may also acted as probes for biological imaging. The third is the seed-induced growth method (Zhong et al., 2012; Cheng et al., 2016; Qin et al., 2016). Zhong et al. (2012) first reported a seed-growth procedure to synthesize monodisperse core–shell nanoparticles Fe3O4@NaGdF4:Yb/Er@NaGdF4:Yb/Er. The Fe3O4 core enables the nanocrystals with superparamagnetic property, while the NaGdF4:Yb/Er outer shell markedly enhances the upconversion emission intensity. In spite that carbon nanomaterials have been extensively studied, the combination of carbon nanomaterials, UCNPs and Fe3O4 together to produce nanocomposites has been rarely reported. Zhu et al. (2016) constructed a carbon-coated nanocomposite, NaLuF4:Yb,Er@NaLuF4@Carbon (csUCNP@C), which can be used as a real-time monitor of the microscopic temperature in PTT. They found that the microscopic temperatures of the photothermal material upon irradiation were high enough to destroy cancer cells, while the lesions remained low enough to avoid the normal tissue from damage. Liu X. H. et al. (2011) coated a hydrophilic carbon layer on hydrophobic NaGdF4:Yb, Er nanocrystals to synthesize the core–shell structured NaGdF4:Yb,Er@Carbon nanocomposites. The prepared nanocomposites were with a uniform size of 25 nm and strong upconversion fluorescence, which can be potentially used as cell-imaging probes. They further developed NaYF4:Yb,Tm@C@CdS nanoparticles by depositing CdS on carbon-modified NaYF4:Yb,Tm nanocrystals. The nanocomposites showed good photocatalytic activity due to the efficient energy transfer from NIR light to ultraviolet-visible (UV) light of the NaYF4:Yb, Tm and the high adsorption ability of the carbon shell (Tou et al., 2016). We have combined Fe3O4 and UCNPs together by using a hollow carbon sphere to assemble a cancer theranostic agent. The nanoplatform can realize MRI-UCL dual-mode imaging and PTT for cancer with improved efficiency in vivo (Wang et al., 2019).
Inspired by the previous works mentioned above, we herein provided a kind of multifunctional nanocomposites which combine superparamagnetic Fe3O4, UCNPs and a hollow carbon sphere together. The synthesized nanocomposites were fabricated by introducing UCNPs and Fe3O4 into a cavity of hollow carbon sphere through a one-pot synthesis method, and the carbon shell wall acts as a protective shell, making it stable and free from the influence of the external environment. Since the carbon layer can convert the absorbed light into heat, the nanocomposites can realize photo thermal (PT), UCL, and MRI tri-mode imaging (Figure 1).
Figure 1. Schematic illustration of the synthesized UCNPs/Fe3O4@h-C nanocomposites with upconversion luminescence (UCL), magnetic resonance (MR), and photothermal (PT) imagings.
Cyclohexane (AR), anhydrous ethanol (AR), dichloromethane (CH2Cl2, AR), acetone (AR), iron (III) chloride hexaydrate (AR), hexamethylenetetramine (HMT, AR) were purchased from Sinopharm Chemical Reagent Co. China. Erbium (III) chloride hexaydrate (ErCl3·6H2O, 99.99%), gadolinium (III) chloride hexaydrate (GdCl3·6H2O, 99.99%), yttrium (III) chloride hexaydrate (YCl3·6H2O, 99.99%), ytterbium (III) chloride hexaydrate (YbCl3·6H2O, 99.99%), 1-octadecene (ODE), oleic acid (OA) were purchased from Sigma-Aldrich Co. Ltd. Sodium oleate (CP), n-hexane (AR), methanol (GC), sodium hydroxide (NaOH, GR), sodium oleate (NaOA, AR), ammonium fluoride (NH4F, GR), 2,4-dihydroxybenzoic acid (DA) were purchased from Shanghai Aladdin Chemistry Co., Ltd (Shanghai, China). All of the chemicals were used without further purification unless specified. A Milli-Q water system (18.2 MΩ•cm, Thermo Fisher) was used to provide the ultrapure water in the experiments.
The superparamagnetic nanoparticles Fe3O4 were synthesized according to a previous reported procedures (Park et al., 2004). Firstly, 5.4 g of FeCl3·6H2O and 18.3 g of NaOA were dissolved in a mixed solvent consisting of 40 mL of ethanol, 70 mL of hexane, and 30 mL of deionized water. The mixture was heated to 70°C and kept for 4 hours. After that, the upper organic layer containing iron oleate complex was washed three times with 15 mL of deionized water. After the hexane was evaporated off, the product iron oleate complex was obtained in a waxy solid form. Secondly, 4.5 g of the above synthesized iron oleate complexes and 0.7125 g of OA were dissolved in 32 mL of ODE. The reaction mixture was heated to 320°C and kept at this temperature for 30 min. When the reaction was completed, the solution was cooled to room temperature and 62.5 mL of ethanol was added. The nanocrystals Fe3O4 were precipitated and separated by centrifugation and dissolved in n-hexane for further usage.
The solutions of YbCl3 (1 M, 400 μL), ErCl3 (0.1 M, 400 μL), GdCl3 (1 M, 500 μL), and YCl3 (1 M, 1,100 μL) were mixed together and heated to 110°C to evaporate the water. After cooling to room temperature, OA (12.0 mL) and ODE (30.0 mL) were added and the mixture was heated to 150°C to make the solid dissolved completely. Next, 20.0 mL of NaOH (0.2 g) and NH4F (0.3 g) methanol solution was added and then the reaction solution was heated to 60°C and kept for 30 min. After the evaporating of the methanol under vacuum, the solution was heated to 300°C and maintained for 1 h in an argon (Ar) atmosphere. Then, the solution was added with equal volume of acetone, the products were precipitated and collected by centrifugation and washed three times with the acetone/cyclohexane solution.
Eight hundred microliter of GdCl3 (1 M) was heated to 110°C until the water was evaporated completely, then OA (12.0 mL) and ODE (30.0 mL) were added and the mixture was heated to 150°C to make the solid dissolved completely. Next, 5 mL of cyclohexane solution containing the obtained NaY/GdF4:Yb was added and heated to evaporate the cyclohexane completely. After that, 20.0 mL of NaOH (0.2 g) and NH4F (0.3 g) methanol solution was added and the temperature was raised to 60°C to evaporate the methanol under vacuum. The next steps were similar to the process of the synthesis of NaY/GdF4:Yb mentioned above to gain the final product NaY/GdF4:Yb,Er@NaYF4, denoted as UCNPs.
Four hundred microliter of hexane solution containing 50 mg of UCNPs and 70 mg of Fe3O4 was added into 5 mL of water solution containing 100 mg of NaOA, the mixture was kept under ultrasonic for 10 min to form a water-in-oil emulsion. After the removal of hexane by evaporation at 50°C, the solution was transfer to a 150 mL reactor containing 0.3853 g of DA and 0.0876 g of HMT with 95 mL of deionized water. The mixture was rapidly heated to 160°C and kept for 4 h. The solid was obtained by centrifugation (8,000 rpm, 10 min), washed for 3 times with deionized water, dried at 60°C for 6 h and then heated to 500°C for 2 h under H2/Ar atmosphere (5%/95%, V/V). The final product is obtained and denoted as UCNPs/Fe3O4@h-C.
The morphologies of the samples were characterized by transmission electron microscopy (JEM-2010F, JEOL). Fourier transform infrared (FTIR) spectra were measured with Nicolet AVATAR370 FTIR spectroscopy. Upconversion luminescent spectra and luminescence decay curves were acquired by Edinburgh FS5 fluorescent spectroscope with a 0–2 W adjustable 980 nm continuous wave laser. Zeta potentials were measured by Malvern Zetasizer Nano ZSE. The magnetic property of the nanoparticles was performed using a Vibrating Sample Magnetometer (7407, lakeshore) at 298 K. The concentrations of Fe3+ were determined by inductively coupled plasma atomic emission spectroscopy (ICP-AES). The MRI property was measured using a SIMENS 3T MR scanner (MAGNETOM Trio Tim). The r2 relaxivity was obtained by linear fitting of the 1/T2 relaxation time (s−1) vs. the Fe3+ concentration (mM).
0.5 mL of UCNPs/Fe3O4@h-C of different concentrations (50, 100, 200, and 400 μg mL−1, respectively) dispersed in deionized water, fetal bovine serum (FBS) with different pH value (4, 6, 8), Dulbecco's Modified Eagle's Medium (DMEM), were irradiate under an 808 nm NIR semiconductor laser. The actual output power was precisely calibrated to be 1.5 Wcm−2 by using an optical power meter. The temperatures were recorded by a thermocouple with an accuracy of 0.1°C every 20 s. All data were acquired from three independent experiments.
According to some previous reports (Roper et al., 2007; Zhu et al., 2016; Wei et al., 2017), the photothermal conversion efficiency η of UCNPs/Fe3O4@h-C nanocomposites was calculated using the following Equations (1)–(3):
In Equation (1), h is heat transfer coefficient, S is the container surface area, Tmax is the equilibrium temperature, Tsurr is ambient temperature, QDis is heat dissipated from light absorbed by the container itself, which is measured independently containing water without UCNPs/Fe3O4@h-C, A808 is the absorption intensity of UCNPs/Fe3O4@h-C at 808 nm. In Equation (2), τs is the sample system time constant, mD and CD are the mass and heat capacity of the solvent (water), respectively.
The cell viabilities were measured with a CCK-8 Kit. Human cervical carcinoma cells (HeLa cells) were used for in vitro cytotoxicity assay of UCNPs/Fe3O4@h-C nanocomposites. The cells were seeded in 96-well plates at a density of 8 × 103 cells per well and incubated overnight in DMEM containing 10% (vol·vol−1) FBS, penicillin (100 μg mL−1), and streptomycin (100 μg mL−1) at 37°C with 5% CO2, then the culture medium was replaced by fresh DMEM containing different concentrations (50, 100, 150, 200 μg/mL) of UCNPs/Fe3O4@h-C for another 24 h. The cell viability of the control group was set as 100% and that of other experimental groups were calculated based on the formula: cell viability = (Abs450nm of the treated group/Abs450nm of the control group) ×100%.
Firstly, core-shell structured NaY/GdF4:Yb,Er@NaYF4 and superparamagnetic nanoparticles Fe3O4 were synthesized by a thermal decomposition method. Secondly, acted as the precursor of polymer layer, the interaction between DA and HMT made the solution acidic with a pH of 2.98, under which NaY/GdF4:Yb,Er@NaYF4 and Fe3O4 nanoparticles were introduced by using NaOA as the surfactant and soft template to transfer oleate-capped NaY/GdF4:Yb,Er@NaYF4 and Fe3O4 nanoparticles into an aqueous phase. Under a hydrothermal condition, HMT is decomposed into NH3 and HCHO to produce cavity structure. Meanwhile, during the heating process, NaOA emulsion droplets are heated and expanded, making the cavity volume grow larger. Finally, the temperature of the mixture was raised to 500°C and kept for 2 h under the atmosphere of Ar (95%) and H2 (5%) to generate the carbon shell from DA reduction (Sun et al., 2013). As shown in Figure 2A, the Fe3O4 has monodisperse morphology, and the particle size is about 12 nm calculated from the TEM images using ImageJ software (Figure S1a, Supplementary Information). In order to improve the luminescence intensity of NaY/GdF4:Yb,Er, a shell NaYF4 was coated on its outer layer to obtain the core-shell structure upconversion nanoparticles NaY/GdF4:Yb,Er@NaYF4 (denoted as UCNPs), as shown in Figure 2B. UCNPs had uniform morphology, good dispersion, and the particle sizes ranging from 18 to 21 nm (Figure S1b, Supplementary Information). The carbon-based multifunctional nanocomposites were finally obtained by means of the hollow carbon sphere to make Fe3O4 and UCNPs locate in the hollow cavity at the same time. As shown in Figure 2C, the synthesized UCNPs/Fe3O4@h-C nanocomposites have a hollow structure with a huge cavity about 160 nm in diameter, and the carbon layer was about 60–70 nm in thickness. The total size of the UCNPs/Fe3O4@h-C is calculated to be 280–300 nm Figure S1c, Supplementary Information). Moreover, we found that UCNPs and Fe3O4 existed simultaneously in the hollow cavity of the nanocomposites, UCNPs with a relatively large particle size (denoted by the red arrow) and Fe3O4 with a relatively small particle size (denoted by the green arrow) were observed at the same time. Compared with NaY/GdF4:Yb,Er@NaYF4 NPs, the particle size of UCNPs decreased when it entered into the hollow cavity of carbon sphere, which may be caused by OA-induced dissolution of the core-shell nanocrystals (Liu et al., 2016; Tang et al., 2019). The zeta potentials of NaY/GdF4:Yb,Er, UCNPs, and UCNPs/Fe3O4@h-C are −5.2 ± 1.13 mV, −4.0 ± 0.62 mV, and −7.5 ± 1.44 mV, respectively (Figure S2, Supplementary Information). Since the nanocomposites contain carbon materials, upconversion luminescence materials and magnetic materials, it is preliminarily believed that this nanosystem can realize photothermal, UCL and MRI trimode imaging simultaneously.
Figure 2. Transmission electron microscopy (TEM) images of Fe3O4 (A), UCNPs (B), and UCNPs/Fe3O4@h-C nanocomposites (C). The red arrow indicates the UCNPs, and he green arrow indicates the Fe3O4.
The FTIR spectra of Fe3O4, UCNPs and UCNPs/Fe3O4@h-C are exhibited in Figures 3A–C, respectively. In Figure 3A, the peaks at 2,917 and 2,852 cm−1 correspond to the asymmetric and symmetric stretching vibrations of methylene (-CH2-) in the oleic acid chain, the peaks at 1,535 cm−1 correspond to the vibration of the unsaturated carbon-oxygen double bond (-C=O-) in the oleic acid, the peaks at 1,445 cm−1 correspond to the vibration of the submethyl (-CH), and the peaks at 594 cm−1 correspond to the Fe-O bond in Fe3O4. In Figure 3B, the asymmetric and symmetric stretching vibrations of methylene (-CH2-) of the UCNPs are at 2,915 and 2,860 cm−1, respectively, the vibration of the unsaturated carbon-oxygen double bond (-C=O-) and the submethyl (-CH) were corresponding to the peaks at 1,567 and 1,457 cm−1, respectively. In Figure 3C, similar peaks at 2,917, 2,850, 1,556, 1,454 cm−1 were observed, which assigned to the asymmetric and symmetric stretching vibrations of methylene (-CH2-) and the vibration of the unsaturated carbon-oxygen double bond (-C=O-). These peaks are attributed to the present of -CH2-CH2- and carbon-oxygen groups of hollow carbon nanospheres, as well as some contributions from the surface groups of UCNPs and Fe3O4 nanoparticles. Meanwhile, the peak at 602 cm−1 corresponding to the Fe-O bond suggested the existence of Fe3O4. The peak at 3,411 cm−1 corresponding to the stretching vibration of hydroxyl (OH) may due to the water molecules adsorbed and the hydroxyl on the surface of carbon spheres.
Figure 3. Fourier transform infrared (FTIR) spectra of Fe3O4 (a), UCNPs (b), and UCNPs/Fe3O4@h-C nanocomposites (c).
Due to the presence of Fe3O4 in the hollow nanocavity, the nanocomposites UCNPs/Fe3O4@h-C are expected to have good magnetic properties. The hysteresis loops of Fe3O4 (red) and UCNPs/Fe3O4@h-C (blue) are shown in Figure 4. Both Fe3O4 NPs and the UCNPs/Fe3O4@h-C nanocomposites showed superparamagnetic properties because no hysteresis was observed in the figures. The saturation magnetization of Fe3O4 is 26.68 emu/g. When Fe3O4 NPs and UCNPs were encapsulated into the hollow carbon cavity together, the saturation magnetization was markedly reduced to 0.87 emu/g, which was a little smaller than that of the samples (1.28 emu/g) prepared by Zhang et al. (2012). This may mainly because of the weight contribution from nonmagnetic NaY/GdF4:Yb,Er@NaYF4 materials and the hollow carbon materials. The magnetic separation ability of UCNPs/Fe3O4@h-C was further studied. Under the action of external magnetic field on one side of glass tube, these black nanoparticles are attracted by magnets in a short time, which proves the existence of magnetic Fe3O4 (Inset photos in Figure 4). Therefore, although the saturation magnetization value of UCNPs/Fe3O4@h-C is decreased, it still showed effective magnetic separation capability. These results demonstrated that the nanocomposites are expected for bioapplications in magnetic enrichment or separation.
Figure 4. Magnetic hysteresis loops of Fe3O4 and UCNPs/Fe3O4@h-C nanocomposites at room temperature. Inset shows the digital photographs of UCNPs/Fe3O4@h-C nanocomposites without (A) or with (B) a commercial permanent magnet.
The upconversion luminescence spectra of NaY/GdF4:Yb,Er@NaYF4 and UCNPs/Fe3O4@h-C nanocomposites were measured and shown in Figure 5. When excited by a 980 nm laser, both NaY/GdF4:Yb,Er@NaYF4 and UCNPs/Fe3O4@h-C exhibited three independent characteristic peaks located at about 524, 545 and 654 nm, they were assigned to the 2H11/2→4I15/2, 4S3/2→4I15/2 and 4F9/2→4I15/2 transitions of Er3+ ions, respectively. The characteristic emission peaks of UCNPs/Fe3O4@h-C nanoparticles are similar to those of NaY/GdF4:Yb,Er@NaYF4, suggesting that the doping of Fe3O4 NPs does not basically impeded the luminescent performance of NaY/GdF4:Yb,Er@NaYF4 despite some partial quenching caused by the black Fe4O3 and the hollow carbon layer. The visualized presentation of UCL can also be tested by the photo taken from a digital camera, as shown in inset of Figure 5, visible green luminescence can be obviously seen when UCNPs/Fe3O4@h-C nanocomposites is irradiated with 980 nm excitation light. The luminescence decay curves of Er3+: 4S3/2→4I15/2 transition in NaY/GdF4:Yb,Er, NaY/GdF4:Yb,Er@NaYF4, and UCNPs/Fe3O4@h-C under 980 nm excitation was shown in Figure S3, and the lifetimes were calculated as 0.04, 0.46, and 0.02 ms for NaY/GdF4:Yb,Er, NaY/GdF4:Yb,Er@NaYF4, and UCNPs/Fe3O4@h-C, respectively. These results indicate that the obtained nanocomposites can retain the upconversion luminescence properties from UCNPs, which can be exploited in optical imaging in vivo.
Figure 5. Upconversion luminescence spectra of UCNPs and UCNPs/Fe3O4@h-C nanocomposites. Inset shows the digital photograph of UCNPs/Fe3O4@h-C nanocomposites under 980 nm laser irradiation.
It is well known that carbon materials have good heat-absorbing properties and can convert the absorbed NIR light into heat efficiently. Herein, the temperature changes of UCNPs/Fe3O4@h-C solution at different concentrations under continuous laser irradiation at 808 nm were measured to evaluate the photothermal conversion of the nanocomposites. As indicated in Figure 6, the temperature of the solution increased by 12.2, 15.2, 25.7, and 34.6°C after 7 min of illumination when the concentrations of the sample were 50, 100, 200, and 400 μg/mL, respectively. Compared with the control group of pure water, the temperature only increased by 8°C under the same conditions (Figure 6A). The photothermal conversions of the nanocomposites in different solutions were also evaluated. As shown in Figure S4 in Supplementary Information, similar trends of temperature changes were observed when UCNPs/Fe3O4@h-C dispersed in FBS with different pH value (4, 6, 8), or cell culture medium DMEM, which indicated that the solvents had little influence on the photothermal effect of UCNPs/Fe3O4@h-C composite. We further tested the thermal stability of UCNPs/Fe3O4@h-C nanocomposites. The sample (200 μg/mL) was irradiated with 808 nm laser for 7 min and then cooled naturally to room temperature. As shown in Figure 6B, the temperature changes of the three heating and cooling experiments are similar, indicating that the samples have good thermal stability. Furthermore, the infrared thermal images of UCNPs/Fe3O4@h-C with different concentrations at the time points of 1.5, 3, 4.5, 6, and 7.5 min were recorded. As shown in Figure 6C, the temperatures of the UCNPs/Fe3O4@h-C nanocomposites increased rapidly with the increase of concentration from 0 to 400 μg/mL. In addition, time constant of heat transfer from this system is determined to be τs = 181.6 s, and the photothermal conversion efficiency (η) was calculated to be 24.07% from the obtained data (Figures 7A,B). Thus, the nanocomposites with a good photothermal conversion effect and good photothermal stability may become a promising photothermal imaging and photothermal treatment agent for tumors.
Figure 6. (A) The temperature changes of water, UCNPs/Fe3O4@h-C nanocomposites at different concentrations (50, 100, 200, 400 μg/mL, respectively) as a function of time under 808 nm laser irradiation at a power density of 1.5 W cm−2. (B) The temperature changes of UCNPs/Fe3O4@h-C nanocomposites during the three photothermal cycles. (C) Infrared thermal images of the different concentrations of UCNPs/Fe3O4@h-C nanocomposites at the time points of 1.5, 3, 4.5, 6, and 7.5 min under 808 nm laser irradiation (1.5 W cm−2).
Figure 7. (A) The temperature changes of UCNPs/Fe3O4@h-C nanocomposites (200 μg mL−1) after 808 nm laser irradiation (1.5 Wcm−2) for 7 min, following the laser being turned off. (B) Time constant for heat transfer from the system calculated to be τs = 181.6 s by using the linear time data from the cooling period (after 420 s) vs. the negative natural logarithm of the driving force temperature, which is obtained from the cooling profile in Figure 6A.
Fe3O4 is well known as a contrast agent for MRI technique, which widely applied for noninvasive biological tissues imaging with high organ resolution. Figure 8A shows relaxation rate r2 (1/T2) plotted against the different Fe3+ concentrations of UCNPs/Fe3O4@h-C nanocomposites. The r2 value of UCNPs/Fe3O4@h-C is calculated by the linear curve with a slope of 57.7 mM−1S−1, indicated that the UCNPs/Fe3O4@h-C nanocomposites have potential applications in MRI as T2-weighted contrast agents. As shown in Figure 8B, the signal intensities decrease gradually with the increase of Fe3+ concentration, which can be seen vividly in a color mapped images.
Figure 8. (A) Relaxation rate r2 (1/T2) plotted against the different Fe3+ concentrations of UCNPs/Fe3O4@h-C nanocomposites. (B) T2-weighted and color-mapped magnetic resonance (MR) images for various Fe3+ concentrations of UCNPs/Fe3O4@h-C nanocomposites.
The cytotoxicity of UCNPs/Fe3O4@h-C nanocomposites in HeLa cells was evaluated by using CCK-8 assay. As shown in Figure S5 in Supplementary Information, the cell viabilities were over 90% at all tested concentrations from 50 to 200 μg/mL, suggesting that UCNPs/Fe3O4@h-C has excellent biocompatibility.
In conclusion, monodisperse, multifunctional UCNPs/Fe3O4@h-C nanocomposites have been successfully prepared. The NaY/GdF4:Yb,Er@NaYF4 UCNPs of the nanocomposites can convert the absorbed 980 nm NIR light into visible luminescence for UCL imaging, the carbon layer of the nanocomposites can convert the absorbed 808 nm NIR light into heat to realize photothermal imaging. Furthermore, the r2 value of UCNPs/Fe3O4@h-C (57.7 mM−1S−1) indicates the nanocomposites could be used as potential T2-weighted MRI contrast agents. To the best of our knowledge, this is the first report to load UCNPs and magnetic nanoparticle simultaneously in the cavity of the hollow carbon cavity to achieve upconversion luminescence, magnetic resonance, and photothermal imaging together.
The datasets generated for this study are available on request to the corresponding author.
The manuscript was written through contributions of all authors. All authors have given their approval of the final version of the manuscript.
We acknowledge the financial support from Weifang Science and Technology Development Plan Project (No. 2018GX108), the Open Experimental Project of Shandong Peninsula Engineering Research Center of Comprehensive Brine Utilization (No. 2018LS017), National Natural Science Foundation of China (No. 31671011), and Innovative Research Team of High-Level Local Universities in Shanghai.
The authors declare that the research was conducted in the absence of any commercial or financial relationships that could be construed as a potential conflict of interest.
The Supplementary Material for this article can be found online at: https://www.frontiersin.org/articles/10.3389/fchem.2020.00611/full#supplementary-material
Chen, S., Weitemier, A. Z., Zeng, X., He, L. M., Wang, X. Y., Tao, Y. Q., et al. (2018). Near-infrared deep brain stimulation via upconversion nanoparticle-mediated optogenetics. Science 359, 679–683. doi: 10.1126/science.aaq1144
Chen, Z. X., and Fu, M. L. (2018). Recyclable magnetic Fe3O4@SiO2/beta-NaYE4:Yb3+,Tm-3(+)/TiO2 composites with NIR enhanced photocatalytic activity. Mater. Res. Bull. 107, 194–203. doi: 10.1016/j.materresbull.2018.07.016
Chen, Z. X., Fu, M. L., Huang, X. D., Yuan, B. L., and Yang, J. C. E. (2018). Magnetic infrared responsive photocatalyst: fabrication, characterization, and photocatalytic performance of beta-NaYF4:Yb3+,Tm3+/TiO2/Fe3O4@SiO2 composite. Res. Chem. Intermediat. 44, 6369–6385. doi: 10.1007/s11164-018-3495-9
Cheng, L., Wang, C., Ma, X. X., Wang, Q. L., Cheng, Y., Wang, H., et al. (2013). Multifunctional upconversion nanoparticles for dual-modal imaging-guided stem cell therapy under remote magnetic control. Adv. Funct. Mater. 23, 272–280. doi: 10.1002/adfm.201201733
Cheng, Q., Guo, H. X., Li, Y., Liu, S. X., Sui, J. H., and Cai, W. (2016). A facile one-pot method to synthesize ultrasmall core-shell superparamagnetic and upconversion nanoparticles. J. Coll. Interface Sci. 475, 1–7. doi: 10.1016/j.jcis.2016.04.040
Ding, Y. D., Hong, X., Zou, P., Liu, K., Cong, T., Zhang, H., et al. (2018). Magnetic upconversion luminescent nanocomposites with small size and strong super-paramagnetism: polyelectrolyte-mediated multimagnetic-beads embedding. ACS Appl. Nano Mater. 1, 145–151. doi: 10.1021/acsanm.7b00059
Fu, S. W., Ding, Y. D., Cong, T., Yang, X. G., Hong, X., Yu, B., et al. (2019). Multifunctional NaYF4:Yb,Er@PE3@Fe3O4 nanocomposites for magnetic-field-assisted upconversion imaging guided photothermal therapy of cancer cells. Dalton Trans. 48, 12850–12857. doi: 10.1039/C9DT02329A
Gao, J. H., Gu, H. W., and Xu, B. (2009). Multifunctional magnetic nanoparticles: design, synthesis, and biomedical applications. Acc. Chem. Res. 42, 1097–1107. doi: 10.1021/ar9000026
Gu, Y. Y., Guo, Z. Y., Yuan, W., Kong, M. Y., Liu, Y. L., Liu, Y. T., et al. (2019). High-sensitivity imaging of time-domain near-infrared light transducer. Nat. Photonics 13:525. doi: 10.1038/s41566-019-0437-z
Jia, T., Xu, J., Dong, S., He, F., Zhong, C., Yang, G., et al. (2019). Mesoporous cerium oxide-coated upconversion nanoparticles for tumor-responsive chemo-photodynamic therapy and bioimaging. Chem. Sci. 10, 8618–8633. doi: 10.1039/C9SC01615E
Liao, G., He, F., Li, Q., Zhong, L., Zhao, R., Che, H., et al. (2020). Emerging graphitic carbon nitride-based materials for biomedical applications. Progress Mater. Sci. 112:100666. doi: 10.1016/j.pmatsci.2020.100666
Liu, D., Xu, X., Du, Y., Qin, X., Zhang, Y., Ma, C., et al. (2016). Three-dimensional controlled growth of monodisperse sub-50 nm heterogeneous nanocrystals. Nat. Commun. 7:10254. doi: 10.1038/ncomms10254
Liu, J. L., Liu, Y., Liu, Q., Li, C. Y., Sun, L. N., and Li, F. Y. (2011). Iridium(III) complex-coated nanosystem for ratiometric upconversion luminescence bioimaging of cyanide anions. J. Am. Chem. Soc. 133, 15276–15279. doi: 10.1021/ja205907y
Liu, X. H., Wang, L. M., Wang, Z. Y., and Li, Z. Q. (2011). Synthesis of biocompatible and luminescent NaGdF4:Yb,Er@Carbon nanoparticles in water-in-oil microemulsion. J. Mater. Res. 26, 82–87. doi: 10.1557/jmr.2010.36
Liu, Y. J., Lu, Y. Q., Yang, X. S., Zheng, X. L., Wen, S. H., Wang, F., et al. (2017). Amplified stimulated emission in upconversion nanoparticles for super-resolution nanoscopy. Nature 543:229. doi: 10.1038/nature21366
Liu, Z., Sun, L. N., Li, F. Y., Liu, Q., Shi, L. Y., Zhang, D. S., et al. (2011). One-pot self-assembly of multifunctional mesoporous nanoprobes with magnetic nanoparticles and hydrophobic upconversion nanocrystals. J. Mater. Chem. 21, 17615–17618. doi: 10.1039/c1jm13871e
Liu, Z. Y., Yi, G. S., Zhang, H. T., Ding, J., Zhang, Y. W., and Xue, J. M. (2008). Monodisperse silica nanoparticles encapsulating upconversion fluorescent and superparamagnetic nanocrystals. Chem. Commun. 6, 694–696. doi: 10.1039/B715402J
Ma, Y. Q., Bao, J., Zhang, Y. W., Li, Z. J., Zhou, X. Y., Wan, C. L., et al. (2019). Mammalian near-infrared image vision through injectable and self-powered retinal nanoantennae. Cell 177:243. doi: 10.1016/j.cell.2019.01.038
Park, J., An, K. J., Hwang, Y. S., Park, J. G., Noh, H. J., Kim, J. Y., et al. (2004). Ultra-large-scale syntheses of monodisperse nanocrystals. Nat. Mater. 3, 891–895. doi: 10.1038/nmat1251
Qin, Z. L., Du, S. N., Luo, Y., Liao, Z. J., Zuo, F., Luo, J. B., et al. (2016). Hydrothermal synthesis of superparamagnetic and red luminescent bifunctional Fe3O4@Mn2+-doped NaYF4:Yb/Er core@shell monodisperse nanoparticles and their subsequent ligand exchange in water. Appl. Surf. Sci. 378, 174–180. doi: 10.1016/j.apsusc.2016.03.219
Roper, D. K., Ahn, W., and Hoepfner, M. (2007). Microscale heat transfer transduced by surface plasmon resonant gold nanoparticles. J. Phys. Chem. C 111, 3636–3641. doi: 10.1021/jp064341w
Shen, J., Sun, L. D., Zhang, Y. W., and Yan, C. H. (2010). Superparamagnetic and upconversion emitting Fe3O4/NaYF4:Yb,Er hetero-nanoparticles via a crosslinker anchoring strategy. Chem. Commun. 46, 5731–5733. doi: 10.1039/c0cc00814a
Sun, Q., Guo, C. Z., Wang, G. H., Li, W. C., Bongard, H. J., and Lu, A. H. (2013). Fabrication of magnetic yolk-shell nanocatalysts with spatially resolved functionalities and high activity for nitrobenzene hydrogenation. Chem. Eur. J. 19, 6217–6220. doi: 10.1002/chem.201300307
Sun, Q., He, F., Bi, H., Wang, Z., Sun, C., Li, C., et al. (2019). An intelligent nanoplatform for simultaneously controlled chemo-, photothermal, and photodynamic therapies mediated by a single NIR light. Chem. Eng. J. 362, 679–691. doi: 10.1016/j.cej.2019.01.095
Tang, M., Zhu, X., Zhang, Y., Zhang, Z., Zhang, Z., Mei, Q., et al. (2019). Near-infrared excited orthogonal emissive upconversion nanoparticles for imaging-guided on-demand therapy. ACS Nano 13, 10405–10418. doi: 10.1021/acsnano.9b04200
Tang, Y. W., Liu, H., Gao, J. W., Liu, X. Y., Gao, X., Lu, X. N., et al. (2018). Upconversion particle@Fe3O4@molecularly imprinted polymer with controllable shell thickness as high-performance fluorescent probe for sensing quinolones. Talanta 181, 95–103. doi: 10.1016/j.talanta.2018.01.006
Tou, M. J., Mei, Y. Y., Bai, S., Luo, Z. G., Zhang, Y., and Li, Z. Q. (2016). Depositing CdS nanoclusters on carbon-modified NaYF4:Yb,Tm upconversion nanocrystals for NIR-light enhanced photocatalysis. Nanoscale 8, 553–562. doi: 10.1039/C5NR06806A
Wang, J. X., Yao, C. J., Shen, B., Zhu, X. H., Li, Y., Shi, L. Y., et al. (2019). Upconversion-magnetic carbon sphere for near infrared light-triggered bioimaging and photothermal therapy. Theranostics 9, 608–619. doi: 10.7150/thno.27952
Wang, X., Xu, J., Yang, D., Sun, C., Sun, Q., He, F., et al. (2018). Fe3O4@MIL-100(Fe)-UCNPs heterojunction photosensitizer: rational design and application in near infrared light mediated hypoxic tumor therapy. Chem. Eng. J. 354, 1141–1152. doi: 10.1016/j.cej.2018.08.070
Wei, R. Y., Xi, W. S., Wang, H. F., Liu, J. L., Mayr, T., Shi, L. Y., et al. (2017). In situ crystal growth of gold nanocrystals on upconversion nanoparticles for synergistic chemo-photothermal therapy. Nanoscale 9, 12885–12896. doi: 10.1039/C7NR02280H
Xu, M., Zou, X. M., Su, Q. Q., Yuan, W., Cao, C., Wang, Q. H., et al. (2018). Ratiometric nanothermometer in vivo based on triplet sensitized upconversion. Nat. Commun. 9:7. doi: 10.1038/s41467-018-05160-1
Zhang, F., Braun, G. B., Pallaoro, A., Zhang, Y. C., Shi, Y. F., Cui, D. X., et al. (2012). Mesoporous multifunctional upconversion luminescent and magnetic “nanorattle” materials for targeted chemotherapy. Nano Lett. 12, 61–67. doi: 10.1021/nl202949y
Zhang, Z., Shikha, S., Liu, J., Zhang, J., Mei, Q., and Zhang, Y. (2019). Upconversion nanoprobes: recent advances in sensing applications. Anal. Chem. 91, 548–568. doi: 10.1021/acs.analchem.8b04049
Zhong, C. N., Yang, P. A. P., Li, X. B., Li, C. X., Wang, D., Gai, S. L., et al. (2012). Monodisperse bifunctional Fe3O4@NaGdF4:Yb/Er@NaGdF4:Yb/Er core-shell nanoparticles. RSC Adv. 2, 3194–3197. doi: 10.1039/c2ra20070h
Zhu, X., Zhang, J., Liu, J., and Zhang, Y. (2019). Recent progress of rare-earth doped upconversion nanoparticles: synthesis, optimization, and applications. Adv. Sci. 6, 1–30. doi: 10.1002/advs.201901358
Zhu, X. J., Feng, W., Chang, J., Tan, Y. W., Li, J. C., Chen, M., et al. (2016). Temperature-feedback upconversion nanocomposite for accurate photothermal therapy at facile temperature. Nat. Commun. 7:10. doi: 10.1038/ncomms10437
Keywords: superparamagnetism, upconversion nanoparticle, carbon, Fe4O3, nanocomposite
Citation: Liu F, Mou X, Song J, Li Q and Liu J (2020) Novel Carbon-Based Magnetic Luminescent Nanocomposites for Multimodal Imaging. Front. Chem. 8:611. doi: 10.3389/fchem.2020.00611
Received: 28 February 2020; Accepted: 11 June 2020;
Published: 24 July 2020.
Edited by:
Dong-Wook Han, Pusan National University, South KoreaReviewed by:
Guillermo Javier Copello, Consejo Nacional de Investigaciones Científicas y Técnicas (CONICET), ArgentinaCopyright © 2020 Liu, Mou, Song, Li and Liu. This is an open-access article distributed under the terms of the Creative Commons Attribution License (CC BY). The use, distribution or reproduction in other forums is permitted, provided the original author(s) and the copyright owner(s) are credited and that the original publication in this journal is cited, in accordance with accepted academic practice. No use, distribution or reproduction is permitted which does not comply with these terms.
*Correspondence: Fangfang Liu, YW5nZWw4MTEyMjFAMTYzLmNvbQ==; Jinliang Liu, bGl1amxAc2h1LmVkdS5jbg==
Disclaimer: All claims expressed in this article are solely those of the authors and do not necessarily represent those of their affiliated organizations, or those of the publisher, the editors and the reviewers. Any product that may be evaluated in this article or claim that may be made by its manufacturer is not guaranteed or endorsed by the publisher.
Research integrity at Frontiers
Learn more about the work of our research integrity team to safeguard the quality of each article we publish.