- 1Departamento Química Física y Analítica, Universidad de Oviedo, Oviedo, Spain
- 2Instituto de Investigación Sanitaria del Principado de Asturias, Oviedo, Spain
- 3Dipartimento di Chimica Ugo Schiff, Università degli Studi di Firenze, Florence, Italy
Latest technological advancement has tremendously expanded the knowledge on the composition of body fluids and the cancer-associated changes, which has fueled the replacement of invasive biopsies with liquid biopsies by using appropriate specific receptors. DNA emerges as a versatile analytical reagent in electrochemical devices for hybridization-based or aptamer-based recognition of all kind of biomarkers. In this mini review, we briefly introduce the current affordable targets (tumor-derived nucleic acids, circulating tumor cells and exosomes) in body fluids, and then we provide an overview of selected electrochemical methods already applied in clinical samples by dividing them into three large categories according to sample type: red (blood), yellow (urine), and white (saliva and sweat) diagnostics. This review focuses on the hurdles of the complex matrices rather than a comprehensive and detailed revision of the format schemes of DNA-based electrochemical sensing. This diverse perspective compiles some challenges that are often forgotten and critically underlines real sample analysis or clinical validation assays. Finally, the needs and trends to reach the market are briefly outlined.
Introduction
Only 30 years after the discovery of circulating tumor DNA (ctDNA) as a type of circulating free DNA (cfDNA) (Stroun et al., 1989) the dream of interrogating the dynamic molecular profile of solid tumors from a blood test is being enthusiastically pursued. Liquid biopsy, the detection of tumor or tumor-derived material in body fluids, has emerged as a minimally invasive subrogate of tissue biopsy. Body fluids are complex matrices containing representative molecules that correlate with the pathological status and progression of disease. The ultimate goal is early detection of cancer and its recurrence through longitudinal studies that allow better patient stratification, but also to observe the response to therapy and drug-resistance earlier than clinical and imaging diagnostics (Crowley et al., 2013; Keller and Pantel, 2019). This attractive concept has bloomed in less than a decade to be integrated in cancer management, which would be impossible without extremely sensitive and selective analytical methodology and the recently available bioinformatic tools.
Besides circulating tumor cells (CTCs), tumor materials released to biological fluids comprise of cell products, mainly nucleic acids (NA), and extracellular vesicles (EV). High throughput and genomic wide analysis of these biomarkers requires sophisticated instrumentation and handling huge amounts of data. Though invaluable, implementation of these technologies is not straightforward, especially in low-resources settings. To reduce the elevated expenditure of health systems in in-vitro diagnostics, biosensors-based devices have appeared as a point-of-care (POC), decentralized, rapid, and low-cost alternative. Among them, electrochemical platforms—the focus of this perspective—are emerging in the clinical field due to miniaturization and multianalyte detection capability in untreated body fluids. Smart measurement strategies provide real-time signal drift correction and adjusted time-resolution, allowing for continuous therapeutic drug monitoring (Aller Pellitero et al., 2019). These promising applications based on self-reporting DNA receptors have not yet been applied to humans or to detect tumor biomarkers.
It is fascinating that the analysis of known NA point mutations and the detection of cells, EV, and their cargo can be accomplished using a single type of probe: DNA, the most versatile molecule (Figure 1A). It recognizes either NA through complementary hybridization or protein biomarkers on EV and CTCs surface through specific affinity interactions (aptamer-based detection), similarly to antigen-antibody recognition. Herein, after a description of the main targets and their challenges as biomarkers, we focus on selected methods applied to blood (red diagnostics), urine (yellow diagnostics), and saliva and sweat (white diagnostics) following the requirements for in-vitro diagnostics with POC devices.
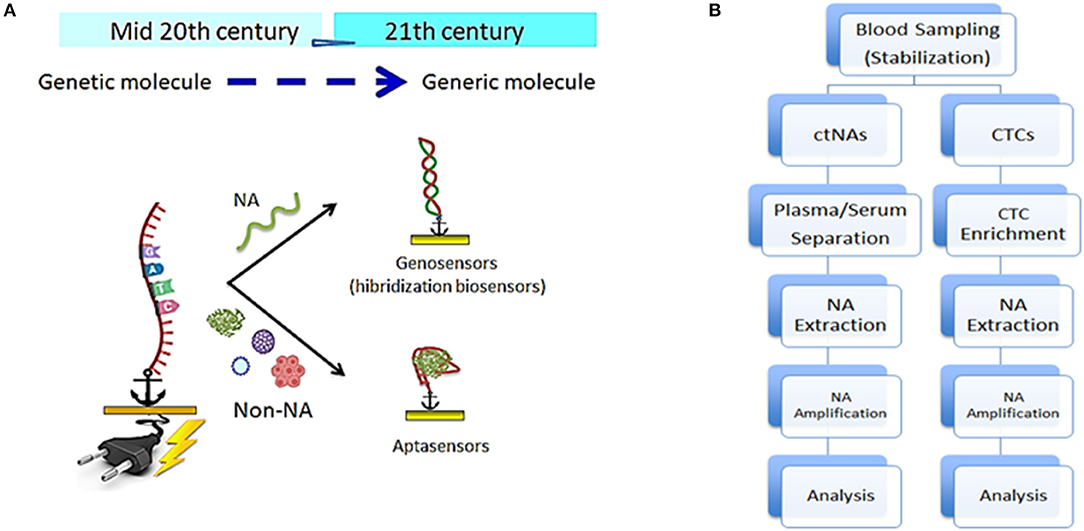
Figure 1. (A) Versatility of DNA-based sensors schematics; genosensors based on hybridization reaction to detect any nucleic acid and aptasensors based on conformation and chemical affinity to detect non-nucleic acid analytes. (B) Comparative step by step pre-analytical workflow for the analysis of clinical blood samples to detect circulating tumor nucleic acid (left) and circulating tumor cells (right).
Targets in Liquid Biopsies
Correlation between levels of CTCs or ctDNA and disease progression/remission/recurrence is the rationale behind their potential use in guiding cancer care. However, their extremely low amount at early disease stages and the high prevalence of tumor-associated mutations in a healthy aged population imposes a boundary to apply them as early-detection biomarkers (Keller and Pantel, 2019).
CtDNA appears in body fluids as even shorter fragments than cfDNA (<200 bp), though there are longer strands that are poorly recovered by current extraction methods (Wan et al., 2017). The short length facilitates the hybridization reaction on biosensor surface and their half-life, <2.5 h, provides a real-time picture of the disease stage and evolution but also imposes challenges in sample collection that needs to be standardized (Rossi and Ignatiadis, 2019). The ctDNA-to-cfDNA ratio varies on disease stage and tumor type (0.1–5%) (Keller and Pantel, 2019), which means the cfDNA is much more abundant than the target complicating the detection. Most cfRNA in body fluids are non-coding RNA and mRNA. Unlike ctDNA the mRNA copy number is larger than two, up to thousands copies, so their concentration in fluids is higher (Siravegna et al., 2017). Non-coding RNAs, formerly known as dark matter, are now regarded as crucial molecules in cancer biology (Miranda-Castro et al., 2019). The shorter RNAs (mi-RNA) have been studied more intensively than the longer counterparts (lncRNA) but both are promising markers in spite of the presence of ribonucleases because they used to be encapsulated in EV or linked to other macromolecules (Wang et al., 2019a).
Beyond free NA, other structures carrying tumor-related components such as aberrant proteins or NAs are regarded as promising biomarkers. CTCs presence in body fluids is associated to poor prognosis and recurrence. Their isolation from an ocean of blood cells is challenging, though not always required. Antigen-dependent enrichment uses antibodies against cell surface markers, but these signatures can be lost during malignant transformation. Cocktails of antibodies could alleviate this issue (Siravegna et al., 2017). As an alternative, cell-SELEX provides a convenient tool to select DNA-based receptors customized to the aberrant features of tumor cells and there are already commercial kits using specific aptamers for this purpose.
EVs are membranous structures secreted by several tissues through different mechanisms originating three distinct vesicle types. Of these, exosomes stand out because their cargo (cfNA and proteins) might mirror the cell of origin. For analytical purposes, exosome isolation through antigen-dependent methods that can be integrated in analytical platforms are preferable over physical methods that are technically demanding and cannot discriminate between EV subtypes containing more relevant information (Wang et al., 2019a).
The nucleic acid nature of capturing aptamers provides an easy way of recovering intact CTCs or exosomes for downstream applications (Zhang et al., 2019). Besides isolation, specific CTC and exosome detection using aptamers is an attractive alternative to immunoassays under active investigation.
DNA-Based Red Diagnostics
Smart and very sensitive (fM and aM) electrochemical strategies have been proposed for the determination of NAs (Bettazzi and Palchetti, 2018; Tavallaie et al., 2018; Das and Kelley, 2019; Soda et al., 2019). However, to the best of our knowledge, so far no examples of electrochemical DNA-based platforms have been proposed for the analysis of ctNAs in untreated human blood samples, just after venipuncture. Thus, electrochemical red diagnostics for ctNA monitoring faces the same sample pre-analytical issues as the ones reported for other analytical techniques. In fact, monitoring of ctNAs is generally performed by using pre-analytical steps consisting of blood collection, sample stabilization, sample processing, NA extraction, and amplification. A schematic of a possible multistep workflow is reported in Figure 1B, including an additional cell enrichment step followed by NA extraction or amplification by cell lysis when the target is NA contained in CTCs.
Blood collection is mainly performed in collection vessels containing EDTA where leucocyte lysis occurs, leading to cellular genomic contamination; therefore, stabilizing blood collection is important in obtaining reproducible and sensitive analytical data. Nowadays, collection devices with preservative reagents are commercially available, and some recent reports review the performance of these collection devices (Toro et al., 2015; Salvianti et al., 2019). Blood storage conditions are another crucial step that should be carefully designed, since degradation and fragmentation of ctDNAs is possible during storage. Blood processing is mainly performed by centrifugation, and procedures have been developed to obtain reproducible results (i.e., double centrifugation steps at known velocity and time) and to obtain stable plasma and serum samples. Owing to the low abundance of ctNAs, NA extraction and amplification are generally performed. Both manual and automated extraction procedures are currently in use, with commercially available extraction kits. These kits operate via liquid-phase isolation methods, solid-phase extraction methods based on silica gel spin columns, and magnetic beads based separation methods. In comparison to ctDNAs, ctRNAs are more fragile and easily prone to degradation due to the presence of ubiquitous RNAses, however, similar protocols can also be applied for RNA extraction and analysis (Haentzsch et al., 2014; El-Khoury et al., 2016).
Many gold standard techniques for ctNA monitoring require a sample-amplification step, generally performed by PCR. Biosensing platforms hold great promise for a simple and rapid detection of ctNAs, since they are claimed to skip the time-consuming extraction and PCR amplification step, and thus, allow for the possibility to be used as screening tests for POC analysis (Finotti et al., 2018). Nano-structuration of the electrochemical platforms, obtaining improved surfaces in terms of higher electroactive surface area and oriented capture probe immobilization (Voccia et al., 2017; Ingrosso et al., 2019), and the development of reliable signal-amplification techniques are important factors to explain the improved detection performance of the electrochemical devices (Voccia et al., 2016). Plasma and serum are the matrices where electrochemical platforms are mainly tested. A PCR-free biosensing approach capable of detecting KRAS and BRAF mutations in the serum of patients with lung cancer and melanoma with an electrochemical clamp assay was proposed by Kelley's group (Das et al., 2015). The clamp chip detected mutated sequences of both ctDNAs and ctRNAs directly in the patient serum, without any previous extraction procedure. The serum sample was mixed with many oligonucleotide sequences that sequester the wild-type sequence and all the mutants except the detection target. The sample was then applied to a PNA probe-modified nanostructured chip, and only the target mutant hybridizes to the immobilized PNA capture probes. The other mutants and wild-type nucleic acids are prevented from binding. Differential pulse voltammetry, using an electrocatalytic reporter system (i.e., Ru(NH3)63+ and Fe(CN)63−), is used for monitoring the hybridization reaction. The same group reported a similar approach for the monitoring of all 40 somatic mutations of the EGFR gene directly in patient serum by using a nanostructured electrochemical chip modified with 7 capture probes (Das et al., 2018).
Enzymes are also frequently used to improve the signal amplification in a PCR-free analytical scheme (Bettazzi et al., 2013; Voccia et al., 2016). Recently, a PCR-free electrochemical approach has been reported that detects the most frequent DNA methylation markers (5-methylcytosine, 5-mC, and/or 5-hydroxymethylcytosine, 5-hmC) directly in serum samples from cancer patients (Povedano et al., 2019).
Analysis of plasma samples other than serum samples is also quite frequently performed. Recently, extremely low amounts of ctDNA (KRAS G12DM) have been achieved in plasma with a label-free biosensor based on double amplification strategy (namely target recycling and isothermal non-templated DNA elongation), though it still requires previous ctDNA extraction (Wang et al., 2018). Cancer-associated epigenetic modifications such as cytosine methylation leading to changes in DNA properties on Au surfaces were also explored in order to detect cancerous genomes in treated plasma samples (Sina et al., 2018).
Regarding the analysis of NAs present in CTCs, an analytical platform integrating electrical lysis and release of cellular targets, isothermal NA amplification and nanozyme-mediated electrochemical detection has been proposed by Trau's group (Koo et al., 2018).
The detection of specific features of CTCs (aberrant protein expression) or tumor-specific protein released into the bloodstream has benefited from the search for novel therapeutic aptamers. Magnetic particles functionalized with aptamers allows for the decoupling of biorecognition and electrochemical transduction, thus minimizing electrode biofouling. This effect could be monitored through for example, the enzymatic hydrolysis of a nitrocellulose film covering the electrode (Malecka et al., 2019), or the on-surface hybridization of a DNA probe acting as a surrogate for the protein biomarker (Liu et al., 2019). Even so, analysis of diluted serum samples is still required, thus demanding more sensitive approaches to detect clinically relevant protein levels. Aptamer-based assays challenged to clinical serum samples (from healthy individuals and cancer patients) showed satisfactory agreement with the immunomethod routinely used in hospitals (Wen et al., 2016; Ma et al., 2018a; Liu et al., 2019; Wang et al., 2019b). Nevertheless, their complexity in steps and reagents (Wen et al., 2016; Ma et al., 2018a; Liu et al., 2019) could discourage their implementation in clinical practice. Of greater simplicity are signal-off tests (Wang et al., 2019b), although they require very strict selectivity controls.
The obvious replacement of antibodies with aptamers to detect the few cancer biomarkers in clinical usage cannot improve the limited clinical utility of those biomarkers. Accumulated evidence shows that novel approaches targeting abnormal glycosylation of proteins among other posttranslational modifications associated to tumorigenesis can be more informative than the total amount of a biomarker (Díaz-Fernández et al., 2018). These strategies are feasible by rational selection of aptamers as recently reported for the detection of PSA in clinical serum samples with a sandwich-type aptasensor. Combining a protein-binding anti-PSA aptamer with an aptamer directed to the glycan moiety enhanced specificity compared to commercial ELISA was achieved when considering final medical diagnosis (Díaz-Fernández et al., 2019).
Regarding the numerous aptasensors for cancer cells, the great performance of nanomaterials is a common feature (Sun et al., 2019) that was brought to light with multiplexing in whole blood (Dou et al., 2019). Interestingly, the release of viable CTCs for downstream analyses has been accomplished by an enzymatic digestion step of a uracil-containing aptamer sequence partially hybridized with a complementary sequence attached to the electrode. The resulting faradaic impedance cytosensor is reusable up to eight times (Shen et al., 2016). An attractive feature of aptamers is the possibility of exploiting DNA amplification techniques to improve sensitivity, which is of particular interest due to the low clinically relevant levels of exosomes or CTCs. A microfluidic platform, ExoPC D-chip, designed for isolation and detection of exosomes in human serum (just 30 μL required), discriminates healthy individuals from patients with hepatic carcinoma in <4 h (Xu et al., 2018). The chip has two differentiated areas for magnetic capture of exosomes, with capture efficiency about 3-times higher than that of a commercial kit, and downstream electrochemical detection onto an ITO electrode. Specific exosome quantification is accomplished by using an in-solution hairpin DNA probe harboring CD63 aptamer and DNAzyme sequences, which is open in the presence of CD63-positive exosomes, giving rise to a catalytic signal.
A more general detection strategy is proposed by (An et al., 2019). An aptamer is immobilized on the surface of a nanostructured electrode surface for capturing exosomes. Isothermal signal amplification by hybridization chain reaction (HCR) is used for signal amplification, by attaching an initiator DNA strand to the captured exosomes via click chemistry. The main drawback of this approach is the longer assay time without substantial gain in sensitivity.
In spite of the advantages of aptamer capture—such as the easy to disrupt aptamer-exosome binding, either by displacement with a complementary oligonucleotide or by DNA-cutting enzymes—immunoaffinity capture of exosomes is still predominant. (Huang et al., 2019) combined this strategy with an aptamer for detection (sandwich approach) so they can benefit from amplification strategies not amenable to antibodies pushing down the LOD of these elusive targets.
In sum, liquid biopsy in blood is advancing quickly toward PCR-free, extraction-free strategies for cfNA detection by means of nanostructuring, and signal amplification strategies. The latter is suitable for concatenation (cascades) but it must be kept to a minimum to be viable in POC employed by non-specialized staff. CTCs and exosome detection with aptamers is still in its infancy and needs to face clinical samples instead of the dominant spiked serum/plasma to be seriously considered for clinical usage. The availability of novel aptamers for features specifically expressed under several disease stages is the bottleneck that needs to be surpassed to boost the development of aptamer-based in-vitro diagnostic devices.
DNA-Based Yellow Diagnostics
Unlike blood, urine-based liquid biopsy is completely non-invasive (home sample collection is indeed feasible), and it allows for more frequent monitoring independently of patient's health status and without restrictions in the collected specimen volume. In addition to these practical advantages favorable to point-of-care testing, the use of urine-based tests can also promote the early detection of urologic cancers (namely prostate, bladder, and kidney) due to the proximity of this fluid to the primary tumor; while blood tests tend to exhibit poorer sensitivity since vascular invasion would occur at an advanced stage of the disease (Peng et al., 2017).
Despite this promising atmosphere, just a few electrochemical DNA-based biosensors have been developed for urine-based biopsy so far, and they mainly employ spiked synthetic urine for validation. As an example, a microfluidic electrochemical device with multiplexing capability for three methylation DNA biomarkers associated with bladder cancer exhibited femtomolar level detection within 20 min, but it was only tested in an enriched urine-mimicking matrix with unknown content in other NA (Pursey et al., 2017). Unspecific protein adsorption and matrix effects appear when using patient samples with label-free techniques. In an impedimetric hybridization-based biosensor for miRNA-21 reporting fM LOD, urine was digested with proteinase K and then filtrated (Smith et al., 2017). That way, an antifouling strategy to protect the surface is unnecessary while miRNA integrity is preserved, though direct detection is not viable. Even when the access to the electrode of the redox probe is restricted to nanochannels within an aptamer-modified silica film, matrix effects preclude direct detection of the target PSA (Argoubi et al., 2018).
These rather simple approaches contrast with an aptamer-based assay for the determination of epithelial cell adhesion molecule (EpCAM), where an enzyme-free strand displacement reaction is combined with isothermal DNA amplification (Chen et al., 2018). The utility of this apta-test was evaluated in different spiked body fluids, including urine, after dilution with a suitable buffer solution to fix the pH and the ionic strength.
It is apparent that yellow diagnostics is less explored and the lower availability of well-studied tumor biomarkers is reflected in the much fewer number of approaches sampling urine. The fact that less effort is invested here delays the development of strategies to fully achieve the direct measurement.
DNA-Based White Diagnostics
Saliva is 94–99% water, and easy to collect in large amounts (humans generate up to 1 L/day), but is poorer than blood in analytes, except for ctDNA from oral cancer (Wang et al., 2015). The advent of more sensitive techniques has boosted the interest in both this fluid and sweat (“white diagnostics”) as a source of cancer biomarkers entering from the blood.
The electrochemical technology EFIRM (Electric Field-Induced Release and Measurement), commercially exploited by Ezlife, was used to validate salivary biomarkers in oral (Wei et al., 2009) and lung (Wei et al., 2014) cancers. The innovative cycling square wave electric field method works by speeding up the target-probe interaction from 30 to 60 min to <5 min and also permits the cargo release from exosomes (Wei et al., 2013). The main advantage is the simultaneous determination of targets of a different nature, i.e., proteins and NA in the same sample but separate electrodes of a single chip without needing individual assay optimization. Saliva analysis is performed after minimal pretreatment in 10 min with a LOD of 3.9 fM for mRNA (Wei et al., 2009). The use of undiluted saliva requires proper antifouling strategies while admitting high target binding capacity as demonstrated with a hybrid nanomaterial composed of reduced graphene oxide and carboxymethylcellulose (Esteban-Fernandez de Avila et al., 2015). Discrimination of a single central mismatched TP53 sequence was possible in spiked saliva, but at relatively high concentrations.
Low detectability is claimed for DNA (12.8 fM) (Ma et al., 2018b) with exonuclease assisted target recycling. To decrease the LOD, avoidance of direct contact between biofluids and the electrode is an alternative approach. This is straightforward on magnetic beads with final MB capture on the electrode surface. Ultrasensitivity of miRNA (0.22 aM) was achieved with enzymatic signal amplification (Wang et al., 2013) but all these approaches were only tested in spiked saliva.
As an ultrafiltrated fluid, saliva has extremely low protein content and aptasensors are currently mainly directed to non-protein targets, such as drug of abuse, according to Scopus database. Nonetheless a prototype of a portable field-effect transistor based on graphene with wireless capability for remote diagnosis has been recently reported to detect a pancreatic cancer biomarker with a remarkable LOD (12 pM), though centrifugation and saliva dilution is still needed (Hao et al., 2019).
The other white fluid, sweat, has found higher applicability in sports medicine until now, because the clinical utility of tumor biomarkers needs to be fully addressed. Nonetheless, an aptamer-based assay has been reported for interleukin-6, a potential biomarker of cancer but also inflammation in artificial sweat (Kumar et al., 2016).
The potential of saliva for liquid biopsy is just finding its way accompanied by a demonstration of its clinical validity with an increasing number of biomarkers (Kaczor-Urbanowicz et al., 2019). Investment in commercial development would suggest that the interest in this fluid will grow in the next decade. On the contrary, sweat seems to be less suited for tumor biomarker detection unless a breakthrough takes places in the near future. Table 1 summarizes the main characteristics of the above commented methods.
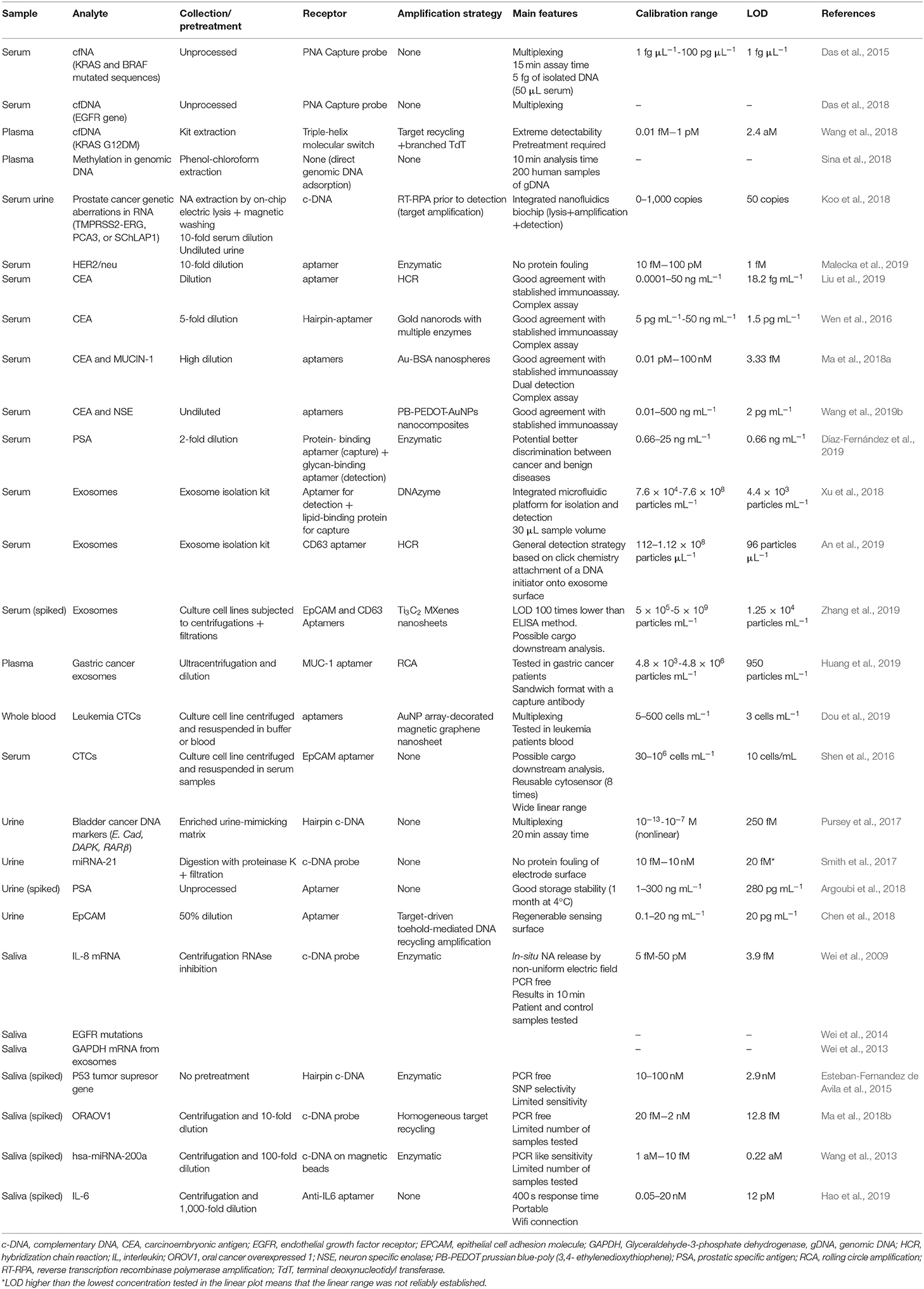
Table 1. Main characteristics of selected electrochemical methods using a nucleic acid as a receptor for the detection of tumor biomarkers in biological fluids (liquid biopsy).
Translation to the Clinical Practice
The creativity of researchers to combine the wide variety of nanomaterials with specifically-designed DNA probes and amplification schemes is endless. However, simplicity is a compulsory feature of POC devices, along with non-marginal improvement over current technology, to attract investors who will translate them into commercial devices and make viable the analytical validation. This step requires the analysis of a huge number of samples and is lacking in many academic works.
Meeting the strict regulations for medical use of in-vitro diagnostic devices is also essential in order to reach the market. Clinical validity depends on biomarker clinical usefulness. It relies on extensive years-long clinical trials that lag behind the active biosensing research field. Thus, the paradoxical scenario of developing tools for non-fully validated targets is a critical barrier for commercialization of electrochemical and other kinds of biosensors. Concurrently, low-cost, and rapid tools as biosensors can speed up the target validation.
Electrochemical DNA-biosensors are reaching the capability of detecting ctNA with minimal or no sample pretreatment but at the expense of correcting the sensor drift due to surface fouling, using disposable platforms (increasing waste) or developing smart schemes to protect the sensing layer (Campuzano et al., 2019; Yang et al., 2019). This also holds for aptasensors that use thiol-PEG (Salimian et al., 2017) or zwitterionic peptides (Cui et al., 2017) as blocking agents, as well as mixed-self assembled monolayers for subsequent covalent immobilization of the receptor (Díaz-Fernández et al., 2019). In any case, sample collection needs harmonization. As long as this is achieved, more biosensors will pass the administration scrutiny.
Microfluidic technology reduces the conventional multistep workflow so it is becoming essential in pursuing the integration of isolating rare cells and exosomes with analysis, commonly in conjunction with magnetic particles (Contreras-Naranjo et al., 2017). Though they rely on immunocapture (Chang et al., 2019), one can envision the successful use of aptamers for an identical purpose.
Companies are investing heavily in hand-held platforms with mobile-phone connectivity or smartphone-based devices to decentralize the expensive clinical analysis requiring large benchtop instrumentation and specialized staff. In tumor biomarker detection, this technology is less explored than for drug abuse analysis or other widespread disorders. It is worth noting that electrochemical detection is the choice for quantitative in-vitro diagnostics (Shin et al., 2018).
Conclusions
The development of electrochemical DNA-based devices for cancer biomarkers is growing at a good pace but is somehow slower than other transduction techniques. The outstanding features they offer cannot be taken aside with the excuse of the relatively complex theoretical foundation of some smart electrochemical measurement schemes. The advances are more visible in blood testing but they must be adapted to other fluids, especially urine, with a huge potential in liquid biopsy. Innovation in format schemes is needed to meet the extreme sensitivity liquid biopsy demands but complex cascades of amplification schemes may not be the route if POC devices are in the spotlight. Otherwise, paying more attention to sample issues is desirable.
Many hybridization assays are now commercial so it is expected that their translation to a low-cost biosensor format will attract investors' attention. On the contrary, aptamers are mainly unknown by a medical community accustomed to antibodies as the reagent of choice for affinity tests. In recent years, a higher interest in them is apparent but their excellent features and versatility needs to be reinforced with reliable clinical applications. The antibody “industry” should not be threatened by aptamers. On the contrary, they should consider them as a complementary technology to fill the gap where antibodies fail, such as directing the selection to specific regions (often low immunogenic or hindered) of the targets. In clinical settings, microfluidics and biosensors with direct connectivity are already reality and these trends will coexist with future smartphone-based devices, provided that they overpass the current limitations in accuracy and reproducibility. We also envision that multiplexing capability will be crucial in future biosensors because of the superior specificity/sensitivity of combination of biomarkers in early detection of cancer.
Author Contributions
RM-C, IP, and NS-Á conceived the work, wrote, and revised the manuscript.
Funding
We acknowledge the financial support from the Spanish Ministerio de Ciencia y Universidades (RTI-2018-095756-B-I00), Principado de Asturias Government (IDI2018-000217), co-financed by FEDER funds, ERA-NET COFUND Photonicsensing, Safe-water GA n. 688735 and Fondazione CR Firenze ID 2018.0944.
Conflict of Interest
The authors declare that the research was conducted in the absence of any commercial or financial relationships that could be construed as a potential conflict of interest.
References
Aller Pellitero, M., Shaver, A., and Arroyo-Currá, N. (2019). Review—Approaches for the electrochemical interrogation of DNA-based sensors: a critical review. J. Electrochem. Soc. 167:037529. doi: 10.1149/2.0292003JES
An, Y., Jin, T., Zhu, Y., Zhang, F., and He, P. (2019). An ultrasensitive electrochemical aptasensor for the determination of tumor exosomes based on click chemistry. Biosens. Bioelectron. 142:111503. doi: 10.1016/j.bios.2019.111503
Argoubi, W., Sanchez, A., Parrado, C., Raouafi, N., and Villalonga, R. (2018). Label-free electrochemical aptasensing platform based on mesoporous silica thin film for the detection of prostate specific antigen. Sens. Actuators B Chem. 255, 309–315. doi: 10.1016/j.snb.2017.08.045
Bettazzi, F., Hamid-Asl, E., Esposito, C. L., Quintavalle, C., Formisano, N., Laschi, S., et al. (2013). Electrochemical detection of miRNA-222 by use of a magnetic bead-based bioassay. Anal. Bioanal. Chem. 405, 1025–1034. doi: 10.1007/s00216-012-6476-7
Bettazzi, F., and Palchetti, I. (2018). Photoelectrochemical genosensors for the determination of nucleic acid cancer biomarkers. Curr. Opin. Electrochem. 12, 51–59. doi: 10.1016/j.coelec.2018.07.001
Campuzano, S., Pedrero, M., Yañez-Sedeño, P., and Pingarrón, J. M. (2019). Antifouling (bio)materials for electrochemical (bio)sensing. Int. J. Mol. Sci. 20:423. doi: 10.3390/ijms20020423
Chang, L., Ni, J., Zhu, Y., Pang, B., Graham, P., Zhang, H., et al. (2019). Liquid biopsy in ovarian cancer: recent advances in circulating extracellular vesicle detection for early diagnosis and monitoring progression. Theranostics 9, 4130–4140. doi: 10.7150/thno.34692
Chen, Q., Hu, W., Shang, B., Wei, J., Chen, L., Guo, X., et al. (2018). Ultrasensitive amperometric aptasensor for the epithelial cell adhesion molecule by using target-driven toehold-mediated DNA recycling amplification. Mikrochim. Acta 185:202. doi: 10.1007/s00604-018-2739-0
Contreras-Naranjo, J. C., Wu, H. J., and Ugaz, V. M. (2017). Microfluidics for exosome isolation and analysis: enabling liquid biopsy for personalized medicine. Lab. Chip 17, 3558–3577. doi: 10.1039/C7LC00592J
Crowley, E., Di Nicolantonio, F., Loupakis, F., and Bardelli, A. (2013). Liquid biopsy: monitoring cancer-genetics in the blood. Nat. Rev. Clin. Oncol. 10, 472–484. doi: 10.1038/nrclinonc.2013.110
Cui, M., Wang, Y., Jiao, M., Jayachandran, S., Wu, Y., Fan, X., et al. (2017). Mixed self-assembled aptamer and newly designed zwitterionic peptide as antifouling biosensing interface for electrochemical detection of alpha-fetoprotein. ACS Sens. 2, 490–494. doi: 10.1021/acssensors.7b00103
Das, J., Ivanov, I., Montermini, L., Rak, J., Sargent, E. H., and Kelley, S. O. (2015). An electrochemical clamp assay for direct, rapid analysis of circulating nucleic acids in serum. Nat. Chem. 7, 569–575. doi: 10.1038/nchem.2270
Das, J., Ivanov, I., Safaei, T. S., Sargent, E. H., and Kelley, S. O. (2018). Combinatorial probes for high-throughput electrochemical analysis of circulating nucleic acids in clinical samples. Angew. Chem. Int. Ed. Engl. 57, 3711–3716. doi: 10.1002/anie.201800455
Das, J., and Kelley, S. O. (2019). High-performance nucleic acid sensors for liquid biopsy applications. Angew. Chem. Int. Ed. Engl. 59, 2554–2564. doi: 10.1002/ange.201905005
Díaz-Fernández, A., Miranda-Castro, R., de-los-Santos-Álvarez, N., and Lobo-Castañón, M. J. (2018). Post-translational modifications in tumor biomarkers: the next challenge for aptamers? Anal. Bioanal. Chem. 410, 2059–2065. doi: 10.1007/s00216-018-0861-9
Díaz-Fernández, A., Miranda-Castro, R., de-los-Santos-Álvarez, N., Rodríguez, E. F., and Lobo-Castañón, M. J. (2019). Focusing aptamer selection on the glycan structure of prostate-specific antigen: toward more specific detection of prostate cancer. Biosens. Bioelectron. 128, 83–90. doi: 10.1016/j.bios.2018.12.040
Dou, B., Xu, L., Jiang, B., Yuan, R., and Xiang, Y. (2019). Aptamer-functionalized and gold nanoparticle array-decorated magnetic graphene nanosheets enable multiplexed and sensitive electrochemical detection of rare circulating tumor cells in whole blood. Anal. Chem. 91, 10792–10799. doi: 10.1021/acs.analchem.9b02403
El-Khoury, V., Pierson, S., Kaoma, T., Bernardin, F., and Berchem, G. (2016). Assessing cellular and circulating miRNA recovery: the impact of the RNA isolation method and the quantity of input material. Sci. Rep. 6:19529. doi: 10.1038/srep19529
Esteban-Fernandez de Avila, B., Araque, E., Campuzano, S., Pedrero, M., Dalkiran, B., Barderas, R., et al. (2015). Dual functional graphene derivative-based electrochemical platforms for detection of the TP53 gene with single nucleotide polymorphism selectivity in biological samples. Anal. Chem. 87, 2290–2298. doi: 10.1021/ac504032d
Finotti, A., Allegretti, M., Gasparello, J., Giacomini, P., Spandidos, D. A., Spoto, G., et al. (2018). Liquid biopsy and PCR-free ultrasensitive detection systems in oncology. Int. J. Oncol. 53, 1395–1434. doi: 10.3892/ijo.2018.4516
Haentzsch, M., Tolios, A., Beutner, F., Nagel, D., Thiery, J., Teupser, D., et al. (2014). Comparison of whole blood RNA preservation tubes and novel generation RNA extraction kits for analysis of mRNA and miRNA profiles. PLoS ONE 9:e113298. doi: 10.1371/journal.pone.0113298
Hao, Z., Pan, Y., Shao, W., Lin, Q., and Zhao, X. (2019). Graphene-based fully integrated portable nanosensing system for on-line detection of cytokine biomarkers in saliva. Biosens. Bioelectron. 134, 16–23. doi: 10.1016/j.bios.2019.03.053
Huang, R., He, L., Xia, Y., Xu, H., Liu, C., Xie, H., et al. (2019). A sensitive aptasensor based on a hemin/G-quadruplex-assisted signal amplification strategy for electrochemical detection of gastric cancer exosomes. Small 15:1900735. doi: 10.1002/smll.201900735
Ingrosso, C., Corricelli, M., Bettazzi, F., Konstantinidou, E., Bianco, G. V., Depalo, N., et al. (2019). Au nanoparticle in situ decorated RGO nanocomposites for highly sensitive electrochemical genosensors. J. Mater. Chem. B 7, 768–777. doi: 10.1039/C8TB02514B
Kaczor-Urbanowicz, K.-E., Wei, F., Liu Rao, S., Kim, J., Shin, H., Cheng, J., et al. (2019). Clinical validity of saliva and novel technology for cancer detection. Biochim. Biophys. Acta Rev. Cancer 1872, 49–59. doi: 10.1016/j.bbcan.2019.05.007
Keller, L., and Pantel, K. (2019). Unravelling tumour heterogeneity by single-cell profiling of circulating tumour cells. Nat. Rev. Cancer 19, 553–567. doi: 10.1038/s41568-019-0180-2
Koo, K. M., Dey, S., and Trau, M. (2018). A sample-to-targeted gene analysis biochip for nanofluidic manipulation of solid-phase circulating tumor nucleic acid amplification in liquid biopsies. ACS Sens. 3, 2597–2603. doi: 10.1021/acssensors.8b01011
Kumar, L. S. S., Wang, X., Hagen, J., Naik, R., Papautsky, I., and Heikenfeld, J. (2016). Label free nano-aptasensor for interleukin-6 in protein-dilute bio fluids such as sweat. Anal. Meth. 8, 3440–3444. doi: 10.1039/C6AY00331A
Liu, Z., Lei, S., Zou, L., Li, G., Xu, L., and Ye, B. (2019). A label-free and double recognition-amplification novel strategy for sensitive and accurate carcinoembryonic antigen assay. Biosens. Bioelectron. 131, 113–118. doi: 10.1016/j.bios.2019.02.020
Ma, C., Liu, H., Zhang, L., Li, H., Yan, M., Song, X., et al. (2018a). Multiplexed aptasensor for simultaneous detection of carcinoembryonic antigen and mucin-1 based on metal ion electrochemical labels and electronic wires. Biosens. Bioelectron. 99, 8–13. doi: 10.1016/j.bios.2017.07.031
Ma, R.-N., Wang, L.-L., Wang, H.-F., Jia, L.-P., Zhang, W., Shang, L., et al. (2018b). Highly sensitive ratiometric electrochemical DNA biosensor based on homogeneous exonuclease III-assisted target recycling amplification and one-step triggered dual-signal output. Sens. Actuators B Chem. 269, 173–179. doi: 10.1016/j.snb.2018.04.143
Malecka, K., Pankratov, D., and Ferapontova, E. E. (2019). Femtomolar electroanalysis of a breast cancer biomarker HER-2/neu protein in human serum by the cellulase-linked sandwich assay on magnetic beads. Anal. Chim. Acta 1077, 140–149. doi: 10.1016/j.aca.2019.05.052
Miranda-Castro, R., de-los-Santos-Álvarez, N., and Lobo-Castañón, M. J. (2019). Long noncoding RNAs: from genomic junk to rising stars in the early detection of cancer. Anal. Bioanal. Chem. 411, 4265–4275. doi: 10.1007/s00216-019-01607-6
Peng, M., Chen, C., Hulbert, A., Brock, M. V., and Yu, F. (2017). Non-blood circulating tumor DNA detection in cancer. Oncotarget 8, 69162–69173. doi: 10.18632/oncotarget.19942
Povedano, E., Montiel, V. R., Valverde, A., Navarro-Villoslada, F., Yañez-Sedeño, P., Pedrero, M., et al. (2019). Versatile electroanalytical bioplatforms for simultaneous determination of cancer-related dna 5-methyl- and 5-hydroxymethyl-cytosines at global and gene-specific levels in human serum and tissues. ACS Sens. 4, 227–234. doi: 10.1021/acssensors.8b01339
Pursey, J. P., Chen, Y., Stulz, E., Park, M. K., and Kongsuphol, P. (2017). Microfluidic electrochemical multiplex detection of bladder cancer DNA markers. Sens. Actuators B Chem. 251, 34–39. doi: 10.1016/j.snb.2017.05.006
Rossi, G., and Ignatiadis, M. (2019). Promises and pitfalls of using liquid biopsy for precision medicine. Cancer Res. 79, 2798–2804. doi: 10.1158/0008-5472.CAN-18-3402
Salimian, R., Kékedy-Nagy, L., and Ferapontova, E. E. (2017). Specific picomolar detection of a breast cancer biomarker HER-2/neu protein in serum: electrocatalytically amplified electroanalysis by the aptamer/PEG-modified electrode. Chem. Electro. Chem. 4, 872–879. doi: 10.1002/celc.201700025
Salvianti, F., Gelmini, S., Costanza, F., Mancini, I., Sonnati, G., Simi, L., et al. (2019). The pre-analytical phase of the liquid biopsy. New Biotechnol. 55, 19–29. doi: 10.1016/j.nbt.2019.09.006
Shen, H., Yang, J., Chen, Z., Chen, X., Wang, L., Hu, J., et al. (2016). A novel label-free and reusable electrochemical cytosensor for highly sensitive detection and specific collection of CTCs. Biosens. Bioelectron. 81, 495–502. doi: 10.1016/j.bios.2016.03.048
Shin, J., Chakravarty, S., Choi, W., Lee, K., Han, D., Hwang, H., et al. (2018). Mobile diagnostics: next-generation technologies for in vitro diagnostics. Analyst 143, 1515–1525. doi: 10.1039/C7AN01945A
Sina, A. A., Carrascosa, L. G., Liang, Z., Grewal, Y. S., Wardiana, A., Shiddiky, M. J. A., et al. (2018). Epigenetically reprogrammed methylation landscape drives the DNA self-assembly and serves as a universal cancer biomarker. Nat. Commun. 9:4915. doi: 10.1038/s41467-018-07214-w
Siravegna, G., Marsoni, S., Siena, S., and Bardelli, A. (2017). Integrating liquid biopsies into the management of cancer. Nat. Rev. Clin. Oncol. 14, 531–548. doi: 10.1038/nrclinonc.2017.14
Smith, D. A., Newbury, L. J., Drago, G., Bowen, T., and Redman, J. E. (2017). Electrochemical detection of urinary microRNAs via sulfonamide-bound antisense hybridisation. Sens. Actuators B Chem. 253, 335–341. doi: 10.1016/j.snb.2017.06.069
Soda, N., Rehm, B. H. A., Sonar, P., Nguyen, N. T., and Shiddiky, M. J. A. (2019). Advanced liquid biopsy technologies for circulating biomarker detection. J. Mater. Chem. B 7, 6670–6704. doi: 10.1039/C9TB01490J
Stroun, M., Anker, P., Maurice, P., Lyautey, J., Lederrey, C., and Beljanski, M. (1989). Neoplastic characteristics of the DNA found in the plasma of cancer patients. Oncology 46, 318–322. doi: 10.1159/000226740
Sun, D., Lu, J., Zhang, L., and Chen, Z. (2019). Aptamer-based electrochemical cytosensors for tumor cell detection in cancer diagnosis: a review. Anal. Chim. Acta 1082, 1–17. doi: 10.1016/j.aca.2019.07.054
Tavallaie, R., McCarroll, J., Le Grand, M., Ariotti, N., Schuhmann, W., Bakker, E., et al. (2018). Nucleic acid hybridization on an electrically reconfigurable network of gold-coated magnetic nanoparticles enables microRNA detection in blood. Nat. Nanotechnol. 13, 1066–1071. doi: 10.1038/s41565-018-0232-x
Toro, P. V., Erlanger, B., Beaver, J. A., Cochran, R. L., VanDenBerg, D. A., Yakim, E., et al. (2015). Comparison of cell stabilizing blood collection tubes for circulating plasma tumor DNA. Clin. Biochem. 48, 993–998. doi: 10.1016/j.clinbiochem.2015.07.097
Voccia, D., Bettazzi, F., Fratini, E., Berti, D., and Palchetti, I. (2016). Improving impedimetric nucleic acid detection by using enzyme-decorated liposomes and nanostructured screen-printed electrodes. Anal. Bioanal. Chem. 408, 7271–7281. doi: 10.1007/s00216-016-9593-x
Voccia, D., Sosnowska, M., Bettazzi, F., Roscigno, G., Fratini, E., De Franciscis, V., et al. (2017). Direct determination of small RNAs using a biotinylated polythiophene impedimetric genosensor. Biosens. Bioelectron. 87, 1012–1019. doi: 10.1016/j.bios.2016.09.058
Wan, J. C. M., Massie, C., Garcia-Corbacho, J., Mouliere, F., Brenton, J. D., Caldas, C., et al. (2017). Liquid biopsies come of age: towards implementation of circulating tumour DNA. Nat. Rev. Cancer 17, 223–238. doi: 10.1038/nrc.2017.7
Wang, H. F., Ma, R. N., Sun, F., Jia, L. P., Zhang, W., Shang, L., et al. (2018). A versatile label-free electrochemical biosensor for circulating tumor DNA based on dual enzyme assisted multiple amplification strategy. Biosens. Bioelectron. 122, 224–230. doi: 10.1016/j.bios.2018.09.028
Wang, Y., Luo, J., Liu, J., Sun, S., Xiong, Y., Ma, Y., et al. (2019b). Label-free microfluidic paper-based electrochemical aptasensor for ultrasensitive and simultaneous multiplexed detection of cancer biomarkers. Biosens. Bioelectron. 136, 84–90. doi: 10.1016/j.bios.2019.04.032
Wang, Y., Springer, S., Mulvey, C. L., Silliman, N., Schaefer, J., Sausen, M., et al. (2015). Detection of somatic mutations and HPV in the saliva and plasma of patients with head and neck squamous cell carcinomas. Sci. Transl. Med. 7:293ra104. doi: 10.1126/scitranslmed.aaa8507
Wang, Y.-M., Trinh, M. P., Zheng, Y., Guo, K., Jimenez, L. A., and Zhong, W. (2019a). Analysis of circulating non-coding RNAs in a non-invasive and cost-effective manner. Trac Trends Anal. Chem. 117, 242–262. doi: 10.1016/j.trac.2019.07.001
Wang, Z., Zhang, J., Guo, Y., Wu, X., Yang, W., Xu, L., et al. (2013). A novel electrically magnetic-controllable electrochemical biosensor for the ultra sensitive and specific detection of attomolar level oral cancer-related microRNA. Biosens. Bioelectron. 45, 108–113. doi: 10.1016/j.bios.2013.02.007
Wei, F., Lin, C. C., Joon, A., Feng, Z., Troche, G., Lira, M. E., et al. (2014). Noninvasive saliva-based EGFR gene mutation detection in patients with lung cancer. Am. J. Respir. Crit. Care Med. 190, 1117–1126. doi: 10.1164/rccm.201406-1003OC
Wei, F., Patel, P., Liao, W., Chaudhry, K., Zhang, L., Arellano-Garcia, M., et al. (2009). Electrochemical sensor for multiplex biomarkers detection. Clin. Cancer Res. 15, 4446–4452. doi: 10.1158/1078-0432.CCR-09-0050
Wei, F., Yang, J., and Wong, D. T. (2013). Detection of exosomal biomarker by electric field-induced release and measurement (EFIRM). Biosens. Bioelectron. 44, 115–121. doi: 10.1016/j.bios.2012.12.046
Wen, W., Huang, J. Y., Bao, T., Zhou, J., Xia, H. X., Zhang, X. H., et al. (2016). Increased electrocatalyzed performance through hairpin oligonucleotide aptamer-functionalized gold nanorods labels and graphene-streptavidin nanomatrix: highly selective and sensitive electrochemical biosensor of carcinoembryonic antigen. Biosens. Bioelectron. 83, 142–148. doi: 10.1016/j.bios.2016.04.039
Xu, H., Liao, C., Zuo, P., Liu, Z., and Ye, B. C. (2018). Magnetic-based microfluidic device for on-chip isolation and detection of tumor-derived exosomes. Anal. Chem. 90, 13451–13458. doi: 10.1021/acs.analchem.8b03272
Yang, Y., Huang, Y., and Li, C. (2019). A reusable electrochemical sensor for one-step biosensing in complex media using triplex-forming oligonucleotide coupled DNA nanostructure. Anal. Chim. Acta 1055, 90–97. doi: 10.1016/j.aca.2018.12.031
Keywords: cancer, liquid biopsy, nucleic acids, electrochemical biosensors, ctDNA, exosomes, CTCs, aptamer
Citation: Miranda-Castro R, Palchetti I and de-los-Santos-Álvarez N (2020) The Translational Potential of Electrochemical DNA-Based Liquid Biopsy. Front. Chem. 8:143. doi: 10.3389/fchem.2020.00143
Received: 19 December 2019; Accepted: 17 February 2020;
Published: 20 March 2020.
Edited by:
Nicole J. Jaffrezic-Renault, Université Claude Bernard Lyon 1, FranceReviewed by:
Lingxin Chen, Yantai Institute of Coastal Zone Research (CAS), ChinaJafar Soleymani, Tabriz University of Medical Sciences, Iran
Copyright © 2020 Miranda-Castro, Palchetti and de-los-Santos-Álvarez. This is an open-access article distributed under the terms of the Creative Commons Attribution License (CC BY). The use, distribution or reproduction in other forums is permitted, provided the original author(s) and the copyright owner(s) are credited and that the original publication in this journal is cited, in accordance with accepted academic practice. No use, distribution or reproduction is permitted which does not comply with these terms.
*Correspondence: Noemí de-los-Santos-Álvarez, c2FudG9zbm9lbWlAdW5pb3ZpLmVz