- College of Chemistry and Pharmaceutical Sciences, Qingdao Agricultural University, Qingdao, China
A facile hydrothermal approach was adopted to the direct synthesis of bimetallic sulfide (CuCo2S4) on carbon cloth (CC) without binders for the supercapacitor's electrodes. A possible formation mechanism was proposed. The prepared bimetallic electrode exhibited a high specific capacitance (Csp) of 1,312 F·g−1 at 1 A·g−1, and an excellent capacitance retention of 94% at 5 A·g−1 over 5,000 cycles. In addition, the asymmetric supercapacitor (CuCo2S4/CC//AC/CC) exhibited energy density (42.9 wh·kg−1 at 0.8 kW·kg−1) and outstanding cycle performance (80% initial capacity retention after 5,000 cycles at 10 A·g−1). It should be noted that the electrochemical performance of a supercapacitor device is quite stable at different bending angles. Two charged devices in series can light 28 red-colored LEDs (2.0 V) for 5 min. All of this serves to indicate the potentially high application value of CuCo2S4.
Introduction
Supercapacitors are extremely valuable for energy storage, owing to their rapid rechargeable ability and extended life cycle (Gao et al., 2015b; He and Chen, 2015). In recent years, they have been widely applied in many fields, such as power backup devices, hybrid electric vehicles, and portable and wearable electronic products (Dong et al., 2015). While flexible electronic products have experienced explosive growth, all electronic devices require flexible and long-life energy storage systems (Hu et al., 2010; Shen et al., 2014; Liu G. et al., 2018). Currently, the main commercial supercapacitors use carbon materials as electrode materials and thus suffer from low-energy density (Dong et al., 2016). Therefore, designing advanced nanostructures with high-energy density and outstanding performance at high-current densities is still given a large amount of attention and holds great appeal (Bao and Li, 2012; Wang et al., 2014; Huang et al., 2016; Xing et al., 2018).
Transition metal compounds, including oxides and sulfides, can provide superior specific capacitance (Csp) due to the rapid faraday redox reaction (Guo et al., 2014; Padmanathan and Selladurai, 2014; Pu et al., 2014; Gao et al., 2015b; Moosavifard et al., 2016). However, the maximum capacitance and energy density of these materials are severely limited by the lower electrical conductivity. Among them, transitional bimetallic sulfides can provide a richer redox reaction, resulting in a generally superior electrochemical performance (Xiong et al., 2015; Sun et al., 2017; Wang J. et al., 2018; You et al., 2018; Dong et al., 2019). For example, Li et al. supported NiCo2S4 nanorods on Ni foam, which displayed a 94.96% capacitive retention over 10,000 cycles (Li M. L. et al., 2018; Li X.-X et al., 2018). At the same time, it has been found that transition bimetallic sulfides also possess rich redox couples and enhance the electronic conductivity (Ai et al., 2016; Wang et al., 2017). In recent years, CuCo2S4 as electrode material has been found to have excellent electronic conductivity, good electrochemical stability, and higher conductivity when compared to its oxide counterparts. In addition, the synergistic effects due to the interaction between diverse metal compounds leads to an enhanced electrochemical energy storage performance, as compared with monometallic sulfides (Shinde et al., 2019). For example, Tian et al. anchored CuCo2S4 to graphene aerogel through solvent thermal reaction with 668 F·g−1 at 1A·g−1. Asymmetrical supercapacitors based on CuCo2S4/GA exhibit a maximum of 22 Wh·kg−1 (Tian et al., 2018). Guo et al. prepared Co2CuS4 on graphene nanosheets, which exhibited Csp of 1,005 F·g−1 at 1A·g−1 (Guo et al., 2016). In addition, designing nanostructures with large surface areas is important for promoting ion transport in electrochemical processes (Wang et al., 2016; Shang et al., 2018).
In order to adapt to the trend of wearable and flexible supercapacitors, others found it desirable to prepare electrode materials on flexible substrates (Dingshan et al., 2015; He et al., 2018). According to previous reports, carbon fiber, nickel foam, and copper foam are widely applied as conductive substrate (Martti et al., 2009; Yang et al., 2014; Xiao et al., 2015; Sun et al., 2016). Among them, the carbon fiber cloth is considered to be an ideal conductive substrate due to its flexibility, electrical conductivity, and low cost. At the same time, directly growing nanostructured materials on carbon cloth avoids the use of binders, resulting in rapid electro transport (Jiang et al., 2011; Liu et al., 2011; Yuan et al., 2012; Zhang et al., 2012, 2016; Wang et al., 2013).
Here, we confirm that carbon cloth is a conductive substrate that can be used to grow a novel 3D needle-flowerlike CuCo2S4 nanostructure. As a result, the flowerlike CuCo2S4 nanostructure exhibits excellent performance (1,312 F·g−1 at 1 A·g−1). Beyond that, an asymmetric solid-state device was manufactured. As a result, it's provided a significant energy density of 42.9 Wh·kg−1 with an initial capacitance retention rate of 80%–even after 5,000 cycles in the 1.6 V-wide working potential window, showing excellent cycling stability.
Materials and Methods
Preparation of CuCo2S4/CC
All reagents were analytical-grade and used without any additional purification. The carbon cloth was purchased from Ce Tech WOS1009. First, a piece of carbon cloth was refluxed at 100°C for 1 h with HNO3 and subsequently rinsed with deionized water and ethanol. As is typical, CuCl2·6H2O (2 mmol), CoCl2·2H2O (4 mmol), urea (30 mmol), and NH4F (20 mmol) were dissolved into 60 mL DI water and stirred for 10 min. Then, the mixture solution and the treated CC were transferred into 100 mL autoclave. After the hydrothermal reaction (140°C for 6 h), the precursors on carbon cloth were washed with deionized water and ethanol, and then dried at 60°C for 12 h.
Next, thioacetamide (TAA, 5 mmol) was dissolved in 60 mL of absolute ethanol with stirring. Then, the solution and the as-obtained precursor were transferred together to the 100 ml autoclave (140°C for 6 h). Finally, after being washed and dried at 60°C overnight, the carbon cloth of uniform load CuCo2S4 was obtained. The loading mass of CuCo2S4 was about 2.3 mg·cm−2. For comparison, CuCo2S4 was directly obtained without the placement of a carbon cloth and the CC without the loaded sample is named blank-CC.
Characterization
X-ray diffraction (XRD) patterns were recorded on a Bruker D8. The morphology of samples was studied by scanning electron microscopy (SEM) (JEOL-7500F, Japan) and transmission electron microscopy (TEM) (HITACHI-7650, Japan). Cycling voltammetry (CV), galvanostatic charge-discharge (GCD), and electrochemical impedance spectroscopy (EIS) were performed with a CHI760E (Shanghai CH, China) electrochemical workstation. Additionally, the cycle life was tested on a CT2001A LAND Battery Test System (LAND, Wuhan, China).
Electrochemical Measurements
The electrochemical measurements were carried out in a three-electrode system by using 3 M KOH aqueous solution as the electrolyte, where the CuCo2S4/CC was directly used as the working electrode, Pt foil was used as the counter electrode, and a saturated calomel electrode (SCE.) was used as the reference electrode. The Csp of materials was calculated through the equation Csp = I × Δt / m × ΔV, where I, ρt, m, and V are the discharge current (A), discharge time (s), loading mass (g), and voltage range.
The flexible solid-state asymmetric supercapacitor (FSASC) device was assembled with a CuCo2S4/CC cathode, active carbon (AC)/CC anode, and PVA/KOH gel electrolyte. The mass ratio between CuCo2S4/CC and AC/CC was m+/m− = 0.19, according to charge conservation (Yuan et al., 2018). The AC/CC electrode was produced by mixing AC 80 wt%, acetylene black 10 wt%, and PTFE 10 wt% and then coated onto carbon cloth. The PVA/KOH gel electrolyte was maintained by mixing KOH (3 g) and PVA (3 g) in 30 ml DI water at 80°C for 1 h with stirring. The energy and power densities were measured by using equation E (Wh·kg−1) = Csp × ΔV2 / 2 and P (W·kg−1) = E / Δt.
Results and Discussion
As illustrated in Scheme 1, the CuCo2S4/CC was successfully synthesized through a simple two-step process. First, the Cu-Co precursor was directly grown on the CC via a hydrothermal process. Subsequently, the Cu-Co precursor is converted into CuCo2S4/CC via a sulfurization strategy.
The chemical composition is recorded by XRD (Figure 1). The different peaks of 16, 26, 31.2, 37.9, 46.9, 49.9, and 54.8° are identical to the standard diffraction data of CuCo2S4 (JCPDS No.42-1450) (You et al., 2018). And the broad peaks at 25 and 45° are derived from the CC substrate (Liu and Wu, 2018).
Figures 2A–H shows the topographical changes of CuCo2S4 on carbon cloth. In Figure 2A, the CuCo2S4 was uniformly adhered to the carbon fiber at a reaction time of 2 h. As seen in Figure 2E, the flowerlike structure, composed of acicular CuCo2S4, is supported on the carbon fiber. When the reaction time was extended to 4 h (Figures 2B,F), the acicular structure of CuCo2S4 began to aggregate. When the reaction time was 6 h (Figures 2C,G), the acicular structure was further aggregated to form a flowerlike structure. The acicular structure provides more active sites, providing abundant pores, while the surface that is initially aggregated by the needle structure provides a larger reaction area. When the reaction time was extended to 8 h, the flowerlike structure supported on the carbon fiber is composed of agglomerated sheets, which reduces the reactive sites and electrochemical performance (Figures 2D,H). The morphological structure of the CoCu-pre and CuCo2S4 is shown in Figure S1. In Figure S1A, the needle CuCo-pre constitutes a flowerlike structure and uniformly grows on the carbon fibers. Figure S1B clearly shows the flowerlike CuCo2S4 structure on the carbon fiber; the exchange of anions during vulcanization does not destroy the overall morphology. In contrast, the CuCo-pre and CuCo2S4 were obtained without adding a carbon cloth substrate (Figures S1C,D). It can be seen that both exhibit similar morphologies as those loaded on carbon cloth.
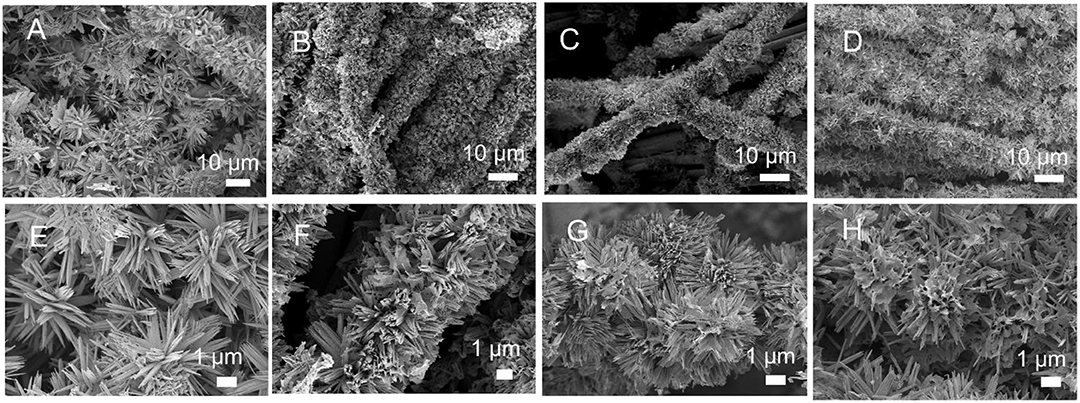
Figure 2. SEM images for CuCo2S4/CC at different reaction times: 2 h (A,E); 4 h (B,F); 6 h (C,G), and 8 h (D,H).
In addition, the microstructure of the CuCo2S4/CC shown by TEM (Figure 3A) further demonstrates that the acicular structure of CuCo2S4 is attached to the carbon fibers. A HRTEM image shows clear lattice fringes with lattice spacing corresponding to the (113) and (004) planes (Figure 3B). The SAED in inset indicates the polycrystalline of the materials. Meanwhile, the TEM and SAED image of CuCo2S4 shows that it is composed of many primary nano-needles and its polycrystalline (Figures S1E,F).
Through the above observations, the growth mechanism of flowerlike CuCo2S4 on carbon cloth can be preliminarily obtained. First, in an alkaline environment, Cu2+ and Co2+ ions form a CuCo(OH)x precursor (Wan et al., 2015; Jayaraman et al., 2017). At this time, the solution becomes a supersaturated solution with a large amount of Cu-Co precursor particles. Due to the high surface energy and thermodynamic instability, the nanoparticles adhere to the carbon fibers to reduce the surface energy. Based on the hydrolysis rate control of NH4F, the precursors will be oriented to grow into the acicular structure and further assemble into a flowerlike structure (Liu S. et al., 2018). The second step is the vulcanization process. The S2− released by TAA undergoes anion exchange with OH− to form a CuCo2S4 flowerlike structure.
The electrochemical properties of CuCo2S4/CC were measured. Figure 4A shows the CV curve of CuCo2S4/CC, CuCo2S4 and blank-CC at 10 mV·s−1. The curve area of CuCo2S4/CC is larger than that of CuCo2S4 and blank-CC, which means that CuCo2S4/CC has a higher specific capacitance. Figure 4B is a CuCo2S4/CC and CuCo2S4 GCD curves at 1 A·g−1. Similarly, the discharge time of the CuCo2S4/CC material is much higher than that of CuCo2S4. And, the capacitance contribution of blank-CC is negligible. Figure 4C shows typical CV curves for CuCo2S4/CC at 0–0.6 V. Each curve shows a significant redox peak, indicating that the Cu(Co)-S-O/Cu(Co)-S-OH-related faraday reaction was carried out (Jayaraman et al., 2017). As the scan rate increased, the redox peak appeared to shift due to the presence of polarization (Ratha et al., 2017). The possible electrochemical reaction equations are as follows (Kaverlavani et al., 2017; Zhu et al., 2017):
In order to accurately compare the specific capacitances, the exact Csp was calculated from GCD measures. Figure 4D shows the GCD curves of CuCo2S4/CC from 1 to 10 A·g−1. From the comparison of Csp graphs of CuCo2S4/CC and CuCo2S4 (Figure 4E), the discharge-specific capacitance of CuCo2S4/CC is 1,312, 1,228, 1,116, 1,010, 864, and 820 F·g−1 at 1, 2, 3, 5, 8, 10 A·g−1, which is much larger than CuCo2S4. The specific capacity values were compatible relative to those previously reported (Table S1). On the other hand, lower charge transfer resistance (Rct) and Warburg impedance (W) also means that CuCo2S4/CC can show superior electrochemical performance compared to CuCo2S4 (Figure S2). It is noteworthy that the capacities decrease with an increase in current density, which may be due to the fact that the transport and/or diffusion of electrolyte ions are suppressed in high current density (Brousse et al., 2015). The CuCo2S4/CC electrode maintained an initial capacity of 94% after cycling 5,000 times at 5 A·g−1, while the Coulomb efficiency remained almost 100%, indicating excellent cycling stability (Figure 4F).
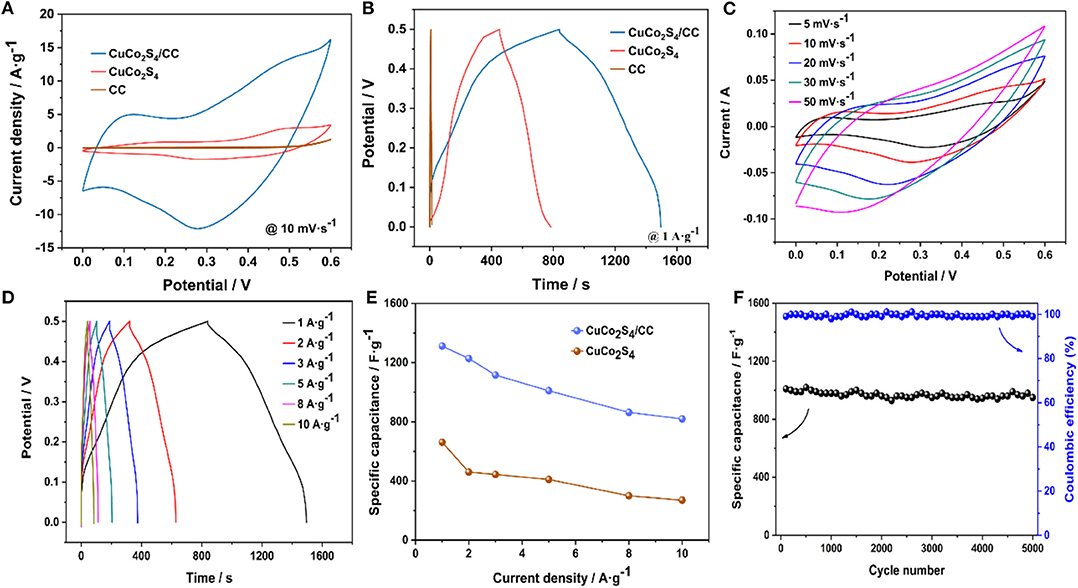
Figure 4. CV curves of CuCo2S4/CC, CuCo2S4, and blank-CC at 10 mV·s−1 (A); GCD curves of CuCo2S4/CC, CuCo2S4, and blank-CC at 1A·g−1 (B); CV curves of CuCo2S4/CC at 5–50 mV·s−1 (C); GCD curves of CuCo2S4/CC at 1–10A·g−1 (D); the rate capability of the as-prepared two electrodes (E); Cycling performance at 5A·g−1 (F).
To prove the application potential, the FSASC device was made of CuCo2S4/CC and AC/CC as the positive and negative electrodes, respectively. Figure 5A is the CV curves of CuCo2S4/CC and AC/CC at 20 mV·s−1. The detailed electrochemical data of the AC is shown in Figure S3. The electrochemical properties of the assembled FSASC were evaluated. The CV and GCD measurements of the ASC device are shown in Figures 5B,C, respectively. At the same time, the charging time of the device is higher than the discharge time in GCD curves, which may be due to the fact that transport of the electrolyte ion cannot be fully carried out at a low current density (Figure 5C). This phenomenon stems from the nature of the gel electrolyte and the inherent properties of the material (Qi et al., 2015; Wang P. et al., 2018). When the current density is 10 A·g−1, a high Csp of 106.3 F·g−1 can be delivered, which shows a retention of 88.1% (Figure 5D).
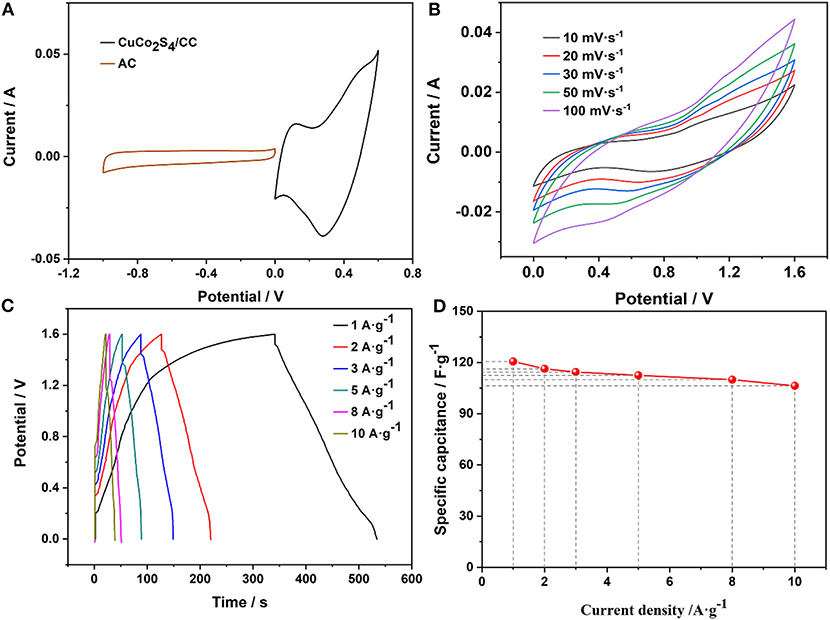
Figure 5. CV curves of the positive and negative (A); CV curves (B); GCD curves (C); and the rate characteristics (D) of the device.
The cycle properties of the ASC device were evaluated by cycling 5,000 times at 10 A·g−1 (Figure 6A). The Csp decreases from 110 to 87.5 F·g−1, which exhibits an excellent retention of 80%. Meanwhile, the coulombic efficiency is almost maintained at 100%, which indicates that the prepared device has excellent performance. Figure 6B shows a maximum energy density of 42.9 Wh·kg−1 at 0.8 kW·kg−1. And it's still has 37.8 Wh·kg−1 at 8 kW·kg−1. In addition, the assembled FSASC device exhibits outstanding flexibility—the CV curves at different angles proving that the bending has little effect on the device (Figures 6C,D).
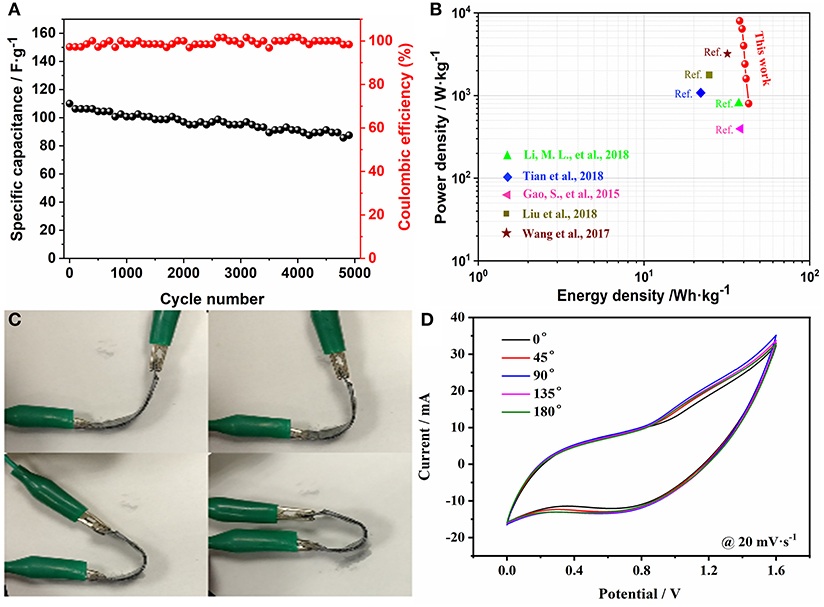
Figure 6. Cycling properties of the device at 10 A·g−1 (A); Ragone plot (B); images of asymmetric device at different bending states (C); and the corresponding CV curves (D).
To demonstrate the practical application potential of the equipment, 28 red LEDs assembled in parallel can be illuminated for more than 5 min (Figure 7), which indicates that CuCo2S4 possess excellent energy storage potential.
Conclusions
In short, we directly grew needle flower structure CuCo2S4 on carbon cloth by means of a hydrothermal reaction and vulcanization process; the prepared CuCo2S4/CC shows an excellent electrochemical performance (1,312 F·g−1 at 1 A·g−1 and 94% retention over 5,000 cycles). When used as an electrode material for flexible asymmetric supercapacitors, the device provides higher energy density (maximum of 42.9 Wh·kg−1) and power density (maximum of 8 kW·kg−1), long-term cycling stability (80% retention over 5,000 cycles), and superior flexibility. The needle flower structure promotes the transport of electrolyte ions, providing a higher specific capacitance. The rapid transport of ions/electrons on carbon fibers imparts high-rate characteristics to flexible solid-state supercapacitors. This indicates that CuCo2S4/CC is promising as a flexible supercapacitor electrode material.
Data Availability Statement
All datasets generated for this study are included in the article/Supplementary Material.
Author Contributions
The manuscript was written through contributions of all authors. All authors have given their approval of the final version of the manuscript.
Funding
This work was financially supported by the Natural Science Foundation of Shandong Province (ZR2017LB031).
Conflict of Interest
The authors declare that the research was conducted in the absence of any commercial or financial relationships that could be construed as a potential conflict of interest.
Acknowledgments
The authors thank the Analytical and Testing Center of Qingdao Agricultural University for allowing them to use its facilities.
Supplementary Material
The Supplementary Material for this article can be found online at: https://www.frontiersin.org/articles/10.3389/fchem.2020.00062/full#supplementary-material
References
Ai, Z., Hu, Z., Liu, Y., Fan, M., and Liu, P. (2016). Novel 3D flowerlike CoNi2S4/carbon nanotube composites as high-performance electrode materials for supercapacitors. N. J. Chem. 40, 340–347. doi: 10.1039/C5NJ02279G
Bao, L., and Li, X. (2012). Towards textile energy storage from cotton T-shirts. Adv. Mater. 24, 3246–3252. doi: 10.1002/adma.201200246
Brousse, T., Bélanger, D., and Long, J. W. (2015). To be or not to be pseudocapacitive? J. Electrochem. Soc. 162, A5185–A5189. doi: 10.1149/2.0201505jes
Dingshan, Y., Shengli, Z., Wenchao, J., Kunli, G., Li, W., Xudong, C., et al. (2015). Transforming pristine carbon fiber tows into high performance solid-state fiber supercapacitors. Adv. Mater. 27, 4895–4901. doi: 10.1002/adma.201501948
Dong, D., Wu, Z., Wang, J., Fu, G., and Tang, Y. (2019). Recent progress in Co9S8-based materials for hydrogen and oxygen electrocatalysis. J. Mater. Chem. A 7, 16068–16088. doi: 10.1039/C9TA04972J
Dong, L., Xu, C., Li, Y., Huang, Z.-H., Kang, F., Yang, Q.-H., et al. (2016). Flexible electrodes and supercapacitors for wearable energy storage: a review by category. J. Mater. Chem. A 4, 4659–4685. doi: 10.1039/C5TA10582J
Dong, L., Xu, C., Yang, Q., Fang, J., Li, Y., and Kang, F. (2015). High-performance compressible supercapacitors based on functionally synergic multiscale carbon composite textiles. J. Mater. Chem. A 3, 4729–4737. doi: 10.1039/C4TA06494A
Gao, S., Liao, F., Ma, S., Zhu, L., and Shao, M. (2015a). Networklike mesoporous NiCo2O4 grown on carbon cloth for high-performance pseudocapacitors. J. Mat. Chem. A 3, 16520–16527. doi: 10.1039/C5TA02876K
Gao, Z., Yang, W., Wang, J., Song, N., and Li, X. (2015b). Flexible all-solid-state hierarchical NiCo2O4/porous graphene paper asymmetric supercapacitors with an exceptional combination of electrochemical properties. Nano Energy 13, 306–317. doi: 10.1016/j.nanoen.2015.02.036
Guo, D., Luo, Y., Yu, X., Li, Q., and Wang, T. (2014). High performance NiMoO4 nanowires supported on carbon cloth as advanced electrodes for symmetric supercapacitors. Nano Energy 8, 174–182. doi: 10.1016/j.nanoen.2014.06.002
Guo, M., Balamurugan, J., Thanh, T. D., Kim, N. H., and Lee, J. H. (2016). Facile fabrication of Co2CuS4 nanoparticle anchored N-doped graphene for high-performance asymmetric supercapacitors. J. Mater. Chem. A 4, 17560–17571. doi: 10.1039/C6TA07400F
He, S., and Chen, W. (2015). Application of biomass-derived flexible carbon cloth coated with MnO2 nanosheets in supercapacitors. J. Power Sources 294, 150–158. doi: 10.1016/j.jpowsour.2015.06.051
He, Z., Jiang, Y., Li, Y., Zhu, J., Zhou, H., Meng, W., et al. (2018). Carbon layer-exfoliated, wettability-enhanced, SO3H− functionalized carbon paper: a superior positive electrode for vanadium redox flow battery. Carbon 127, 297–304. doi: 10.1016/j.carbon.2017.11.006
Hu, L., Pasta, M., Mantia, F. L., Cui, L., Jeong, S., Deshazer, H. D., et al. (2010). Stretchable, porous, and conductive energy textiles. Nano Lett. 10, 708–714. doi: 10.1021/nl903949m
Huang, Z., Zhang, Z., Qi, X., Ren, X., Xu, G., Wan, P., et al. (2016). Wall-like hierarchical metal oxide nanosheet arrays grown on carbon cloth for excellent supercapacitor electrodes. Nanoscale 8, 13273–13279. doi: 10.1039/C6NR04020A
Jayaraman, B., Chao, L., Peera, S. G., Kim, N.H., and Lee, J. H. (2017). High-energy asymmetric supercapacitors based on free-standing hierarchical Co-Mo-S nanosheets with enhanced cycling stability. Nanoscale 9, 13747–13759. doi: 10.1039/C7NR03763E
Jiang, H., Zhao, T., Ma, J., Yan, C., and Li, C. (2011). Ultrafine manganese dioxide nanowire network for high-performance supercapacitors. Chem. Commun. 47, 1264–1266. doi: 10.1039/C0CC04134C
Kaverlavani, S. K., Moosavifard, S. E., and Bakouei, A. (2017). Designing graphene-wrapped nanoporous CuCo2O4 hollow spheres electrodes for high-performance asymmetric supercapacitors. J. Mater. Chem. A 5, 14301–14309. doi: 10.1039/C7TA03943C
Li, M. L., Xiao, K., Su, H., Li, N., Cai, Y. P., Liu, Z. Q., et al. (2018). CuCo2S4 nanosheets coupled with carbon nanotube heterostructures for highly efficient capacitive energy storage. ChemElectroChem 5, 2496–2502. doi: 10.1002/celc.201800329
Li, X.-X., Wang, X.-T., Xiao, K., Ouyang, T., Li, N., and Liu, Z.-Q. (2018). In situ formation of consubstantial NiCo2S4 nanorod arrays toward self-standing electrode for high activity supercapacitors and overall water splitting. J. Power Sources 402, 116–123. doi: 10.1016/j.jpowsour.2018.09.021
Liu, C., and Wu, X. (2018). NiCo2S4 nanotube arrays grown on flexible carbon fibers as battery-type electrodes for asymmetric supercapacitors. Mater. Res. Bull. 103, 55–62. doi: 10.1016/j.materresbull.2018.03.014
Liu, G., Wang, B., Liu, T., Wang, L., Luo, H., Gao, T., et al. (2018). 3D self-supported hierarchical core/shell structured MnCo2O4@CoS arrays for high-energy supercapacitors. J. Mat. Chem. A 6, 1822–1831. doi: 10.1039/C7TA10140F
Liu, R., Duay, J., and Lee, S. B. (2011). Heterogeneous nanostructured electrode materials for electrochemical energy storage. Chem. Commun. 47, 1384–1404. doi: 10.1039/C0CC03158E
Liu, S., Yin, Y., Hui, K. S., Hui, K. N., Lee, S. C., and Jun, S. C. (2018). High-performance flexible quasi-solid-state supercapacitors realized by molybdenum dioxide@nitrogen-doped carbon and copper cobalt sulfide tubular nanostructures. Adv. Sci. 5:1800733. doi: 10.1002/advs.201800733
Martti, K., Chan, C. K., Ma, J., Yi, C., and George, G. (2009). Printable thin film supercapacitors using single-walled carbon nanotubes. Nano Lett. 9, 1872–1876. doi: 10.1021/nl8038579
Moosavifard, S. E., Fani, S., and Rahmanian, M. (2016). Hierarchical CuCo2S4 hollow nanoneedle arrays as novel binder-free electrodes for high-performance asymmetric supercapacitors. Chem. Commun. 52, 4517–4520. doi: 10.1039/C6CC00215C
Padmanathan, N., and Selladurai, S. (2014). Controlled growth of spinel NiCo2O4 nanostructures on carbon cloth as a superior electrode for supercapacitors. RSC Adv. 4:8341. doi: 10.1039/c3ra46399k
Pu, J., Wang, T., Wang, H., Tong, Y., Lu, C., Kong, W., et al. (2014). Direct growth of NiCo2S4 nanotube arrays on nickel foam as high-performance binder-free electrodes for supercapacitors. Chempluschem 79, 577–583. doi: 10.1002/cplu.201300431
Qi, J. L., Wang, X., Lin, J. H., Zhang, F., Feng, J. C., and Fei, W.-D. (2015). A high-performance supercapacitor of vertically-oriented few-layered graphene with high-density defects. Nanoscale 7, 3675–3682. doi: 10.1039/C4NR07284G
Ratha, S. K., Samantara, A., Singha, K. K., Sadashiv, A., Gangan Chakraborty, B., et al. (2017). Urea-assisted room temperature stabilized metastable beta-NiMoO4 experimental and theoretical insights into its unique bifunctional activity toward oxygen evolution and supercapacitor. ACS Appl. Mater. Interfaces 9, 9640–9653. doi: 10.1021/acsami.6b16250
Shang, Y., Xie, T., Ma, C., Su, L., Gai, Y., Liu, J., et al. (2018). Synthesis of hollow ZnCo2O4 microspheres with enhanced electrochemical performance for asymmetric supercapacitor. Electrochim. Acta 286, 103–113. doi: 10.1016/j.electacta.2018.08.025
Shen, L., Che, Q., Li, H., and Zhang, X. (2014). Mesoporous NiCo2O4 nanowire arrays grown on carbon textiles as binder-free flexible electrodes for energy storage. Adv. Funct. Mater. 24, 2630–2637. doi: 10.1002/adfm.201303138
Shinde, S. K., Ramesh, S., Bathula, C., Ghodake, G. S., Kim, D. Y., Jagadale, A. D., et al. (2019). Novel approach to synthesize NiCo2S4 composite for high-performance supercapacitor application with different molar ratio of Ni and Co. Sci. Rep. 9, 1–10. doi: 10.1038/s41598-019-50165-5
Sun, H., Xie, S., Li, Y., Jiang, Y., Sun, X., Wang, B., et al. (2016). Large-area supercapacitor textiles with novel hierarchical conducting structures. Adv. Mater 28, 8431–8438. doi: 10.1002/adma.201602987
Sun, W., Wang, Y., Wu, H., Wang, Z., Rooney, D., and Sun, K. (2017). 3D free-standing hierarchical CuCo2O4 nanowire cathodes for rechargeable lithium-oxygen batteries. Chem. Commun. 53, 8711–8714. doi: 10.1039/C7CC02621H
Tian, Z., Wang, X., Li, B., Li, H., and Wu, Y. (2018). High rate capability electrode constructed by anchoring CuCo2S4 on graphene aerogel skeleton toward quasi-solid-state supercapacitor. Electrochem. Acta 298, 321–329. doi: 10.1016/j.electacta.2018.12.103
Wan, H., Liu, J., Ruan, Y., Lv, L., Peng, L., Ji, X., et al. (2015). Hierarchical configuration of NiCo2S4 nanotube@Ni-Mn layered double hydroxide arrays/three-dimensional graphene sponge as electrode materials for high-capacitance supercapacitors. ACS Appl. Mater. Interfaces 7, 15840–15847. doi: 10.1021/acsami.5b03042
Wang, G., Wang, H., Lu, X., Ling, Y., Yu, M., Zhai, T., et al. (2014). Solid-state supercapacitor based on activated carbon cloths exhibits excellent rate capability. Adv. Mater. 26, 2676-2682, 2615. doi: 10.1002/adma.201304756
Wang, J., Liang, J., Wu, J., Xuan, C., Wu, Z., and Guo, X. (2018). Coordination effect of network NiO nanosheet and a carbon layer on the cathode side in constructing a high-performance lithium-sulfur battery. J. Mater. Chem. A 6, 6503–6509. doi: 10.1039/C8TA00270C
Wang, J.-G., Jin, D., Zhou, R., Shen, C., Xie, K., and Wei, B. (2016). One-step synthesis of NiCo2S4 ultrathin nanosheets on conductive substrates as advanced electrodes for high-efficient energy storage. J. Power Sources 306, 100–106. doi: 10.1016/j.jpowsour.2015.12.014
Wang, P., Zhang, Y., Yin, Y., Fan, L., Zhang, N., and Sun, K. (2018). In Situ synthesis of CuCo2S4@N/S-doped graphene composites with pseudocapacitive properties for high-performance lithium-ion batteries. ACS Appl. Mater. Interfaces 10, 11708–11714. doi: 10.1021/acsami.8b00632
Wang, Q., Wang, X., Liu, B., Yu, G., Hou, X., Chen, D., et al. (2013). NiCo2O4 nanowire arrays supported on Ni foam for high-performance flexible all-solid-state supercapacitors. J. Mat. Chem. A 1:2468. doi: 10.1039/c2ta01283a
Wang, Y., Yang, D., Zhou, T., Pan, J., Wei, T., and Sun, Y. (2017). Oriented CuCo2S4 nanograss arrays/Ni foam as an electrode for a high-performance all-solid-state supercapacitor. Nanotechnology 28:465402.
Xiao, F., Yang, S., Zhang, Z., Liu, H., Xiao, J., Wan, L., et al. (2015). Scalable synthesis of freestanding sandwich-structured graphene/polyaniline/graphene nanocomposite paper for flexible all-solid-state supercapacitor. Sci. Rep. 5:9359. doi: 10.1038/srep09359
Xing, L., Dong, Y., Hu, F., Wu, X., and Umar, A. (2018). Co3O4 nanowire@NiO nanosheet arrays for high performance asymmetric supercapacitors. Dalton Trans. 47, 5687–5694. doi: 10.1039/C8DT00750K
Xiong, X., Waller, G., Ding, D., Chen, D., Rainwater, B., Zhao, B., et al. (2015). Controlled synthesis of NiCo2S4 nanostructured arrays on carbon fiber paper for high-performance pseudocapacitors. Nano Energy 16, 71–80. doi: 10.1016/j.nanoen.2015.06.018
Yang, Y., Zhiwei, P., Gunuk, W., Gedeng, R., Xiujun, F., Lei, L., et al. (2014). Three-dimensional thin film for lithium-ion batteries and supercapacitors. ACS Nano 8, 7279–7287. doi: 10.1021/nn502341x
You, H., Zhang, L., Jiang, Y., Shao, T., Li, M., and Gong, J. (2018). Bubble-supported engineering of hierarchical CuCo2S4 hollow spheres for enhanced electrochemical performance. J. Mater. Chem. A 6, 5265–5270. doi: 10.1039/C7TA07890K
Yuan, C., Li, J., Hou, L., Zhang, X., Shen, L., and Lou, X. W. D. (2012). Ultrathin mesoporous NiCo2O4 nanosheets supported on Ni foam as advanced electrodes for supercapacitors. Adv. Funct. Mater. 22, 4592–4597. doi: 10.1002/adfm.201200994
Yuan, X., Tang, B., Sui, Y., Huang, S., Qi, J., Pu, Y., et al. (2018). CuCo2S4 nanotubes on carbon fiber papers for high-performance all-solid-state asymmetric supercapacitors. J. Mat. Sci. Mat. Elect. 29, 8636–8648. doi: 10.1007/s10854-018-8878-6
Zhang, G. Q., Wu, H. B., Hoster, H. E., Chan-Park, M. B., and Lou, X. W. (2012). Single-crystalline NiCo2O4 nanoneedle arrays grown on conductive substrates as binder-free electrodes for high-performance supercapacitors. Energy Environ. Sci. 5:9453. doi: 10.1039/c2ee22572g
Zhang, Y., Hu, Z., An, Y., Guo, B., An, N., Liang, Y., et al. (2016). High-performance symmetric supercapacitor based on manganese oxyhydroxide nanosheets on carbon cloth as binder-free electrodes. J. Power Sources 311, 121–129. doi: 10.1016/j.jpowsour.2016.02.017
Zhu, J., Tang, S., Wu, J., Shi, X., Zhu, B., and Meng, X. (2017). Wearable high-performance supercapacitors based on silver-sputtered textiles with FeCo2S4-NiCo2S4 composite nanotube-built multitripod architectures as advanced flexible electrodes. Adv. Ene. Mat. 7:1601234. doi: 10.1002/aenm.201601234
Keywords: flexible electrode, nanoneedles, CuCo2S4, pseudocapacitance, supercapacitors
Citation: Xie T, Xu J, Wang J, Ma C, Su L, Dong F and Gong L (2020) Freestanding Needle Flower Structure CuCo2S4 on Carbon Cloth for Flexible High Energy Supercapacitors With the Gel Electrolyte. Front. Chem. 8:62. doi: 10.3389/fchem.2020.00062
Received: 19 December 2019; Accepted: 20 January 2020;
Published: 27 February 2020.
Edited by:
Tang Wei, Xi'an Jiaotong University, ChinaReviewed by:
Bingbing Tian, Shenzhen University, ChinaChengxin Peng, University of Shanghai for Science and Technology, China
Copyright © 2020 Xie, Xu, Wang, Ma, Su, Dong and Gong. This is an open-access article distributed under the terms of the Creative Commons Attribution License (CC BY). The use, distribution or reproduction in other forums is permitted, provided the original author(s) and the copyright owner(s) are credited and that the original publication in this journal is cited, in accordance with accepted academic practice. No use, distribution or reproduction is permitted which does not comply with these terms.
*Correspondence: Liangyu Gong, bHlnb25nQDE2My5jb20=